DOI:
10.1039/C6RA01131D
(Paper)
RSC Adv., 2016,
6, 16150-16158
Dye-sensitized solar cell based on an inclusion complex of a cyclic porphyrin dimer bearing four 4-pyridyl groups and fullerene C60†
Received
14th January 2016
, Accepted 1st February 2016
First published on 2nd February 2016
Abstract
Cyclic free-base porphyrin dimers (H4-C4-CPDPy(TEO) and H4-Ptz-CPDPy(TEO)) linked by butadiyne or phenothiazine bearing four 4-pyridyl groups and their inclusion complexes (C60⊂H4-C4-CPDPy(TEO) and C60⊂H4-Ptz-CPDPy(TEO)) with fullerene C60 have been applied to dye-sensitized solar cells (DSSCs) as a new class of porphyrin dye sensitizers with pyridyl anchoring groups for attachment on a TiO2 electrode. The FTIR spectra of the porphyrin dimers adsorbed on TiO2 nanoparticles demonstrated that these porphyrin dimers are adsorbed on the TiO2 surface through the formation of hydrogen bonding of pyridyl groups and/or pyridinium ions at Brønsted acid sites on the TiO2 surface. The adsorption amount of the porphyrin dimers adsorbed on the TiO2 electrode is 2.0 × 1017 molecules per cm2, that is, the adsorption amount of the porphyrin unit is 4.0 × 1017 cm−2, which is higher than that of dye sensitizers with pyridyl groups reported so far. The photovoltaic performance of DSSCs based on phenothiazine-bridged cyclic porphyrin dimer H4-Ptz-CPDPy(TEO) is higher than that of DSSCs based on butadiyne-linked cyclic porphyrin dimer H4-C4-CPDPy(TEO). Moreover, the photovoltaic performances of DSSCs based on cyclic free-base porphyrin dimers are higher than those of DSSCs based on their C60 inclusion complexes C60⊂H4-C4-CPDPy(TEO) and C60⊂H4-Ptz-CPDPy(TEO). On the basis of the electrochemical measurements (voltammetry and electrochemical impedance spectroscopy) and the transient absorption spectroscopy, the differences in the photovoltaic performances among these cyclic free-base porphyrin dimers are discussed from kinetic and thermodynamic considerations concerning the electron transfer processes in DSSCs.
Introduction
Dye-sensitized solar cells (DSSCs) employing dye-adsorbed TiO2 electrodes are one of the most promising new renewable photovoltaic cells utilizing the sun as a free and inexhaustible energy source because of their interesting construction and operational principles, and low cost of production, since Grätzel and co-workers produced high-performance DSSCs based on a Ru-complex dye, which showed a solar energy-to-electricity conversion yield (η) of 11%.1 To further improve the photovoltaic performance of DSSCs, many kinds of ruthenium (Ru) dyes, porphyrin dyes, phthalocyanine dyes and organic dyes bearing carboxyl groups as anchoring groups, which are adsorbed on the TiO2 electrode through the bidentate bridging linkage between the carboxyl group of the dye and Brønsted acid sites (surface-bound hydroxyl groups, Ti-OH) on the TiO2 surface, have been developed as dye sensitizers during the last two decades.2–9 In particular, porphyrin dyes have been regarded as promising candidates for photosensitizers as a result of their strong Soret (400–500 nm) and moderate Q band (500–700 nm) absorption properties, as well as their electrochemical, photochemical and thermal stabilities. Much effort in molecular design and development of porphyrin dye sensitizers having carboxyl group have been made to further improve the photovoltaic performances of DSSCs so far.10–15 Consequently, DSSCs based on donor–π–acceptor (D–π–A) porphyrin dyes bearing the diarylamino group as an electron donor and the benzothiadiazole-benzoic acid moiety as an electron acceptor, which exhibited good absorption features (bathochromic shift and broadening of the Soret and Q bands), have achieved η value of up to ca. 13%.11d,e
On the other hand, we have reported that a new type of D–π–A dye sensitizers bearing pyridyl group as electron-withdrawing anchoring group were predominantly adsorbed on the TiO2 electrode through coordinate bonding between the pyridyl group of the dye and the Lewis acid site (exposed Tin+ cations) on the TiO2 surface.16,17 It was demonstrated that the new-type of D–π–A dye sensitizers can inject electrons efficiently from the pyridyl group to the conduction band (CB) of the TiO2 electrode through the coordinate bonding, rather than the bidentate bridging linkages of conventional D–π–A dye sensitizers bearing carboxyl group. Recently, Wang et al. reported that DSSCs based on D–π–A porphyrin dye bearing a pyridyl group reached solar energy-to-electricity conversion yield (η) of 3.96%.18a On the other hand, Goutsolelos et al. reported the η value of 3.9% for DSSC based on porphyrin dye bearing four pyridyl groups.18c However, the adsorption amounts (<5.0 × 1016 molecules per cm2) of these porphyrin dye sensitizers bearing pyridyl group adsorbed on TiO2 electrode are much lower than those of porphyrin dye sensitizers bearing carboxyl group, and thus the low dye loading leads to low light-harvesting efficiency (LHE) and poor surface coverage of the TiO2 electrode, resulting in lowering of the photovoltaic performances of DSSCs.18,19 More recently, Goutsolelos et al. have designed and synthesized “spider-shaped” porphyrin dye sensitizer bearing oligophenylenevinylene moieties, long dodecyloxy chains, and four pyridyl groups, which showed high dye loading value (1.9 × 1017 molecules per cm2) due to an increase in the basicity of the pyridyl groups. As the results, the DSSC based on the “spider-shaped” porphyrin dye sensitizer reached the η value of 5.12%.18e
Recently, we have designed and prepared cyclic free-base porphyrin dimers (H4–C4-CPDPy(TEO) and H4-Ptz-CPDPy(TEO)) linked by butadiyne or phenothiazine bearing four 4-pyridyl groups and their inclusion complexes (C60⊂H4-C4-CPDPy(TEO) and C60⊂H4-Ptz-CPDPy(TEO)) with fullerene C60.20 It was found that these porphyrin dimers have favorable photochemical and electrochemical properties for DSSC through the electrochemical measurements and the transient absorption spectroscopy. Moreover, as for phenothiazine derivative H4-Ptz-CPDPy(TEO), it would be expected that the phenothiazine unit possessing electron donating ability can provide a unidirectional flow of electrons toward the pyridyl anchoring group upon photoexcitation of the porphyrin, leading to the efficient electron injection from the photoexcited dye to the CB of TiO2 electrode. Thus, in this work, to achieve high dye loading and high surface coverage of the TiO2 electrode for DSSCs based on porphyrin dye sensitizers bearing pyridyl group, the cyclic free-base porphyrin dimers H4-C4-CPDPy(TEO) and H4-Ptz-CPDPy(TEO) and their C60 inclusion complexes C60⊂H4-C4-CPDPy(TEO) and C60⊂H4-Ptz-CPDPy(TEO) have been applied to DSSCs as a new class of porphyrin dye sensitizers bearing pyridyl anchoring groups for attachment on TiO2 electrode (Scheme 1). It was demonstrated that these porphyrin dimers are adsorbed on the TiO2 surface through the formations of hydrogen bonding of pyridyl groups and/or pyridinium ion at Brønsted acid sites on the TiO2 surface. Here we reveal the photovoltaic performances of DSSCs based on these porphyrin dimers from kinetic and thermodynamic consideration concerning the electron transfer processes in DSSCs, based on the electrochemical measurements (voltammetry and electrochemical impedance spectroscopy) and the transient absorption spectroscopy.
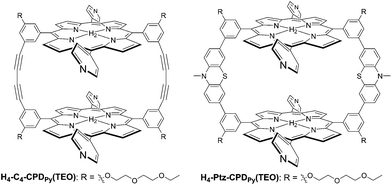 |
| Scheme 1 Cyclic free-base porphyrin dimers (H4-C4-CPDPy(TEO) and H4-Ptz-CPDPy(TEO)) bearing 4-pyridyl groups. | |
Results and discussion
Photoabsorption properties of cyclic free-base porphyrin dimers and their inclusion complexes with fullerene C60
The synthesis of H4-Ptz-CPDPy(TEO) has been reported elsewhere.20e The synthetic pathway for H4-C4-CPDPy(TEO) is shown in Scheme 2. The inclusion complexes C60⊂H4-C4-CPDPy(TEO) and C60⊂H4-Ptz-CPDPy(TEO) with C60 were prepared by slowly evaporating the mixed solution of the corresponding cyclic free-base porphyrin dimer in chloroform (0.1 mM, 5 mL) and C60 in toluene (0.1 mM, 5 mL). The UV/vis absorption spectra of cyclic free-base porphyrin dimers H4-C4-CPDPy(TEO) and H4-C4-CPDPy(TEO) in benzonitrile are shown in Fig. 1 and their spectral data are summarized in Table 1. The porphyrin dimers H4-C4-CPDPy(TEO) and H4-Ptz-CPDPy(TEO) exhibit strong Soret band at around 420 nm and relatively weak Q band in the range 500–650 nm. For the C60 inclusion complexes C60⊂H4-C4-CPDPy(TEO) and C60⊂H4-Ptz-CPDPy(TEO), it is difficult to obtain their exact absorption spectra because the 1
:
1 complex of H4-C4-CPDPy(TEO) or H4-Ptz-CPDPy(TEO) with C60 is in dissociation equilibrium in solution of 10−5 to 10−6 M concentration which is suitable for the measurement of absorption spectra of porphyrins. In our previous work, however, we have demonstrated that upon addition of C60 to the benzonitrile solution of the cyclic porphyrin dimers, their Soret bands were redshifted with a decrease in intensity, whereas their Q bands were slightly redshifted but increased in intensity.20
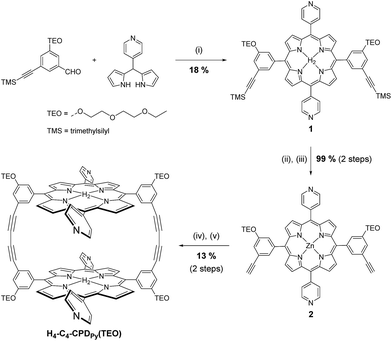 |
| Scheme 2 Synthetic pathway for H4-C4-CPDPy(TEO). (i) TFA, DDQ, CH2Cl2; (ii) Zn(OAc)·2H2O, MeOH, CH2Cl2; (iii) KF, DMF; (iv) CuCl, pyridine, air; (v) HCl aq. | |
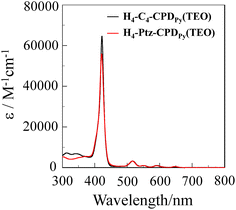 |
| Fig. 1 UV/vis absorption spectra of H4-C4-CPDPy(TEO) and H4-Ptz-CPDPy(TEO) in benzonitrile. | |
Table 1 Optical, electrochemical data and HOMO and LUMO energy levels of H4-C4-CPDPy(TEO), C60⊂H4-C4-CPDPy(TEO), H4-Ptz-CPDPy(TEO), and C60⊂H4-Ptz-CPDPy(TEO)
Porphyrin dimers |
λ
absmax/nm (ε/M−1 cm−1)a |
E
oxpa
b/V vs. Fc/Fc+ |
HOMOc/eV |
LUMOc/eV |
In benzonitrile.
Anodic (Epa) peak potentials for oxidation were recorded in benzonitrile/Bu4NPF6 (0.1 M) solution for the porphyrin unit in H4-C4-CPDPy(TEO), C60⊂H4-C4-CPDPy(TEO), H4-Ptz-CPDPy(TEO) and C60⊂H4-Ptz-CPDPy(TEO).
HOMO and LUMO energy levels of the porphyrin unit.
|
H4-C4-CPDPy(TEO)
|
422 (646 000), 517 (30 800), 551 (9800), 590 (9600), 647 (4300) |
0.71 |
−5.51 |
−3.61 |
C60⊂H4-C4-CPDPy(TEO)
|
— |
0.74 |
−5.54 |
−3.64 |
H4-Ptz-CPDPy(TEO)
|
421 (55 800), 516 (32 100), 550 (10 500), 590 (9500), 646 (5600) |
0.72 |
−5.52 |
−3.62 |
C60⊂H4-Ptz-CPDPy(TEO)
|
— |
0.75 |
−5.55 |
−3.65 |
The UV/vis absorption spectra of cyclic free-base porphyrin dimers and their C60 inclusion complexes adsorbed on TiO2 film are shown in Fig. 2. It is worth mentioning here that the adsorption amount of the porphyrin dimers adsorbed on TiO2 film is 2.0 × 1017 molecules per cm2, that is, the adsorption amount of porphyrin unit is 4.0 × 1017 cm−2, which is higher than those (<2.0 × 1017 molecules per cm2) of porphyrin dye sensitizers and D–π–A dye sensitizer bearing pyridyl groups reported so far.16,18 The Soret bands of C60⊂H4-C4-CPDPy(TEO) and C60⊂H4-Ptz-CPDPy(TEO) were redshifted compared to those of H4-C4-CPDPy(TEO) and H4-Ptz-CPDPy(TEO), although there is little difference in the Q band between the cyclic free-base porphyrin dimers and their C60 inclusion complexes. Thus, the UV/vis absorption spectra of these porphyrin dimers-adsorbed TiO2 films are in good agreement with those in benzonitrile.
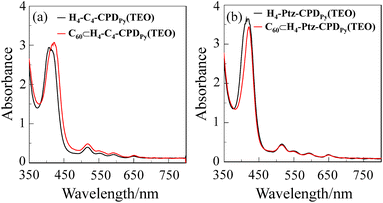 |
| Fig. 2 UV/vis absorption spectra of (a) H4-C4-CPDPy(TEO) and C60⊂H4-C4-CPDPy(TEO) and (b) H4-Ptz-CPDPy(TEO) and C60⊂H4-Ptz-CPDPy(TEO) adsorbed on TiO2 film (3 μm). | |
Electrochemical and photochemical properties of cyclic free-base porphyrin dimers and their inclusion complexes with fullerene C60
The electrochemical properties of cyclic free-base porphyrin dimers and their C60 inclusion complexes were determined by cyclic voltammetry (CV) and differential pulse voltammetry (DPV) (see Fig. S7–S10 in ESI†).20 The oxidation potential of the porphyrin unit in C60⊂H4-C4-CPDPy(TEO) (0.74 V vs. Fc/Fc+) showed anodic shift by 0.03 V, compared with that of H4-C4-CPDPy(TEO) (0.71 V) (Table 1). The oxidation potential of the porphyrin unit in C60⊂H4-Ptz-CPDPy(TEO) (0.75 V) also showed anodic shift by 0.03 V, compared with that of H4-Ptz-CPDPy(TEO) (0.72 V). The reduction potentials corresponding to the reduction of the fullerene entity of C60⊂H4-C4-CPDPy(TEO) and C60⊂H4-Ptz-CPDPy(TEO) were observed at −0.94 V and −0.96 V, respectively, which is cathodically shifted by 0.02 V and 0.04 V, respectively, compared to pristine C60 (−0.92 V).20e The small anodic shift of the oxidation potential of the porphyrin and the small cathodic shift of the reduction potential of C60 compared with their reference compounds is indicative of the charge transfer interaction between the porphyrins and C60. The HOMO and LUMO energy levels were evaluated from the oxidation wave and the singlet excited energy of these porphyrin dimers (1.90 eV) based on the Q absorption band and fluorescence band in benzonitrile (ca. 650 nm), respectively. The HOMO and LUMO energy levels of these porphyrin dimers was ca. −5.5 eV and ca. −3.6 eV, respectively (Table 1). Thus, this result shows that the HOMO energy levels are more positive than the I3−/I− redox potential (−4.9 eV), and thus this indicates that an efficient regeneration of the oxidized porphyrin dimers by electron transfer from the I3−/I− redox couple in the electrolyte is thermodynamically feasible. Evidently, the LUMO energy levels of these porphyrin dimers are higher than the energy level (Ecb) of the CB of TiO2 (−4.0 eV), suggesting that an electron injection to the CB of TiO2 is thermodynamically feasible (some researchers have proposed that an energy gap of over 0.2–0.3 eV is necessary for efficient electron injection).2–7
Time-resolved absorption spectroscopy for cyclic free-base porphyrin dimers and their C60 inclusion complexes was performed by femtosecond laser flash photolysis after photoexcitation.20 The photodynamics of these porphyrin dimers is summarized in Fig. 3. The decay of photoexcited state of C60⊂H4-C4-CPDPy(TEO) has two steps: the first step has a lifetime of 18 ps, which corresponds to the disappearance of the singlet excited state of the 1H4-C4-CPDPy(TEO)* (ca. −3.6 eV), that is, the 1H4-C4-CPDPy(TEO)* undergoes intrasupramolecular electron transfer to give a completely charge-separated state C60˙−-H4-C4-CPDPy(TEO)˙+ (ca. −3.7 eV). The C60˙−-H4-C4-CPDPy(TEO)˙+ decays with a lifetime of 470 ps to the ground state. The decay of photoexcited state of C60⊂H4-Ptz-CPDPy(TEO) also includes the charge-separated states C60˙−-H4-Ptz-CPDPy(TEO)˙+ (ca. −3.8 eV), but has multiple steps to the ground state via the triplet charge separated state 3(C60˙−-H4-Ptz˙+-CPDPy(TEO)) (ca. −4.3 eV) with a lifetime of 0.71 ms: the first step has a lifetime of 20 ps, which is similar to that (18 ps) of C60⊂H4-C4-CPDPy(TEO). On the other hand, the energy diagrams for the photochemical events in these porphyrin dimes revealed that the energy levels of charge-separated states is very close to or lower than the Ecb of the CB of TiO2 electrode. This suggests that the electron injection from the C60˙− in the charge-separated states to the CB of TiO2 electrode is thermodynamically difficult.
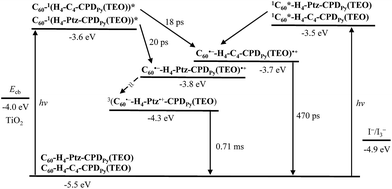 |
| Fig. 3 Energy diagrams for the photochemical events in C60⊂H4-C4-CPDPy(TEO) and C60⊂H4-Ptz-CPDPy(TEO). | |
FTIR spectra of cyclic free-base porphyrin dimers and their inclusion complexes with fullerene C60
To elucidate the adsorption states of cyclic free-base porphyrin dimers and their C60 inclusion complexes on TiO2 nanoparticles, we measured the FTIR spectra of the porphyrin dimer powders and the porphyrin dimers adsorbed on TiO2 nanoparticles (Fig. 4). For the powders of all the four porphyrin dimers H4-C4-CPDPy(TEO), H4-Ptz-CPDPy(TEO), C60⊂H4-C4-CPDPy(TEO), and C60⊂H4-Ptz-CPDPy(TEO), the C
N stretching band of pyridyl group was clearly observed at around 1590 cm−1. Interestingly, when these porphyrin dimers were adsorbed on the TiO2 surface, a new band appeared at around 1650 cm−1, which indicates the formation of a pyridinium ion with Brønsted acid sites on TiO2 surface.21 In addition, the C
N stretching band at around 1590 cm−1 is shifted by 3–5 cm−1 to higher wavenumber, that is, the resulting band can be assigned to the hydrogen-bonded pyridyl group to Brønsted acid sites on the TiO2 surface. Consequently, these observations demonstrate that these porphyrin dimers are adsorbed on the TiO2 surface through the formations of hydrogen bonding of pyridyl groups and/or pyridinium ion at Brønsted acid sites on the TiO2 surface. Thus, the UV/vis absorption and the FTIR spectra of the porphyrin dimers adsorbed on TiO2 film indicate that high dye loading and high surface coverage of the TiO2 electrode for DSSCs based on porphyrin dye sensitizers bearing pyridyl group are achieved by employing the cyclic porphyrin dimers bearing four pyridyl anchoring groups possessing the bonding ability to the two points on Brønsted acid sites on TiO2 surface.
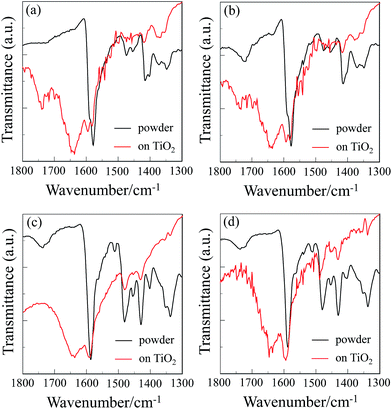 |
| Fig. 4 FTIR spectra of the porphyrin dimer powders and the porphyrin dimers adsorbed on TiO2 nanoparticles for (a) H4-C4-CPDPy(TEO), (b) C60⊂H4-C4-CPDPy(TEO), (c) H4-Ptz-CPDPy(TEO), and (d) C60⊂H4-Ptz-CPDPy(TEO). | |
Photovoltaic performances of DSSCs based on cyclic free-base porphyrin dimers and their inclusion complexes with fullerene C60
The DSSC was prepared using the dye-adsorbed TiO2 electrode (9 μm), Pt-coated glass as a counter electrode, and an acetonitrile solution with iodine (0.05 M), lithium iodide (0.1 M), and 1,2-dimethyl-3-propylimidazolium iodide (0.6 M) as an electrolyte. The photocurrent–voltage (I–V) characteristics were measured under simulated solar light (AM 1.5, 100 mW cm−2). The incident photon-to-current conversion efficiency (IPCE) spectra and the I–V curves are shown in Fig. 5. The photovoltaic performance parameters are collected in Table 2. The IPCE values corresponding to the Soret band (29% at 424 nm) and the Q band (6% at 520 nm) for the phenothiazine-bridged cyclic porphyrin dimer H4-Ptz-CPDPy(TEO) are higher than those (15% at 420 nm for the Soret band and 2% at 522 nm for the Q band) of the butadiyne-linked cyclic porphyrin dimer H4-C4-CPDPy(TEO) (Fig. 5a). The I–V curves show that the short-circuit photocurrent density (Jsc) and η values of H4-Ptz-CPDPy(TEO) (1.40 mA cm−2 and 0.35%) are higher than those of H4–C4-CPDPy(TEO) (0.79 mA cm−2 and 0.19%) (Fig. 5b). The higher photovoltaic performance for DSSC based on H4-Ptz-CPDPy(TEO) may be attributed to the efficient electron injection from the photoexcited dye (1H4-Ptz-CPDPy(TEO)*) to the CB of TiO2 electrode because the phenothiazine unit possessing electron donating ability can provide a unidirectional flow of electrons toward the pyridyl anchoring group upon photoexcitation of the porphyrin. Interestingly, the photovoltaic performances of DSSC based on the C60 inclusion complexes C60⊂H4-C4-CPDPy(TEO) and C60⊂H4-Ptz-CPDPy(TEO) are lower than those of H4-C4-CPDPy(TEO) and H4-Ptz-CPDPy(TEO). The electron injection (≈100 ps) from the photoexcited porphyrin to the CB of TiO2 electrode is in kinetically competition with the intrasupramolecular electron transfer (18–20 ps), that is, the formation of charge-separated state C60˙−-H4-C4-CPDPy(TEO)˙+ and C60˙−-H4-Ptz-CPDPy(TEO)˙+ (Fig. 3). Therefore, the lower photovoltaic performances for DSSC based on the C60 inclusion complexes would be attributed to the formation of charge-separated state, leading to low electron-injection efficiency from the photoexcited porphyrin to CB of TiO2 electrode. On the other hand, the photovoltaic performance of DSSC based on C60⊂H4-Ptz-CPDPy(TEO) is slightly lower than that of C60⊂H4-C4-CPDPy(TEO), which may be attributed to the thermodynamically difficult electron-injection due to the lower triplet charge separated state 3(C60˙−-H4-Ptz˙+- CPDPy(TEO)).
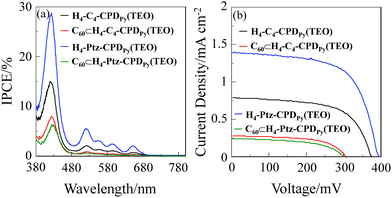 |
| Fig. 5 (a) IPCE spectra and (b) I–V curves of DSSCs based on H4-C4-CPDPy(TEO), C60⊂H4–C4-CPDPy(TEO), H4-Ptz-CPDPy(TEO), and C60⊂H4-Ptz-CPDPy(TEO). | |
Table 2 DSSC performance parameters of H4-C4-CPDPy(TEO), C60⊂H4-C4-CPDPy(TEO), H4-Ptz-CPDPy(TEO), and C60⊂H4-Ptz-CPDPy(TEO)
Porphyrin dimersa |
J
sc/mA cm−2b |
V
oc
b/mV |
ffb |
η
b (%) |
The adsorption amount of the porphyrin dimers adsorbed on TiO2 electrode is 2.0 × 1017 molecules per cm2 under the adsorption condition of 0.1 mM porphyrin dimer solution in chloroform. The amount of adsorbed dye on TiO2 electrode was determined form the calibration curve by absorption spectral measurement of the concentration change of the dye solution before and after adsorption.
Under a simulated solar light (AM 1.5, 100 mW cm−2).
|
H4-C4-CPDPy(TEO)
|
0.79 |
376 |
0.64 |
0.19 |
C60⊂H4-C4-CPDPy(TEO)
|
0.27 |
315 |
0.69 |
0.06 |
H4-Ptz-CPDPy(TEO)
|
1.40 |
396 |
0.63 |
0.35 |
C60⊂H4-Ptz-CPDPy(TEO)
|
0.24 |
304 |
0.54 |
0.04 |
Moreover, it is worth mentioning here that the open-circuit photovoltage (Voc) values of the C60 inclusion complexes C60⊂H4-C4-CPDPy(TEO) (315 mV) and C60⊂H4-Ptz-CPDPy(TEO) (304 mV) are lower than those of H4-C4-CPDPy(TEO) (376 mV) and H4-Ptz-CPDPy(TEO) (396 mV). Thus, electrochemical impedance spectroscopy (EIS) analysis was performed to study the electron recombination process in DSSCs based on these porphyrin dimers in the dark under a forward bias of −0.60 V with a frequency range of 10 mHz to 100 kHz. The large semicircle in the Nyquist plot (Fig. 6a), which corresponds to the midfrequency peaks in the Bode phase plots, represents the charge recombination between the injected electrons in TiO2 and I3− ions in the electrolyte, that is, the charge-transfer resistances at the TiO2/dye/electrolyte interface. The Nyquist plots show that the resistance values of the large semicircle for H4-C4-CPDPy(TEO) (13 Ω) and H4-Ptz-CPDPy(TEO) (14 Ω) are higher than those of C60⊂H4-C4-CPDPy(TEO) (5 Ω) and C60⊂H4-Ptz-CPDPy(TEO) (5 Ω), indicating that the electron recombination resistances for H4-C4-CPDPy(TEO) and H4-Ptz-CPDPy(TEO) are larger those of C60⊂H4-C4-CPDPy(TEO) and C60⊂H4-Ptz-CPDPy(TEO). The electron recombination lifetimes (τe) expressing the electron recombination between the injected electrons in TiO2 and I3− ions in the electrolyte, extracted from the angular frequency (ωrec) at the midfrequency peak in the Bode phase plot (Fig. 6b) using τe = 1/ωrec, increase in the order of C60⊂H4-C4-CPDPy(TEO) (0.1 ms) and C60⊂H4-Ptz-CPDPy(TEO) (0.1 ms) < H4-C4-CPDPy(TEO) (2.2 ms) < H4-Ptz-CPDPy(TEO) (2.5 ms), respectively, which are consistent with the sequence of Voc values in the DSSCs. Consequently, this is attributed to the fact that the formation of charge-separated state C60˙−-H4-C4-CPDPy(TEO)˙+ and C60˙−-H4-Ptz-CPDPy(TEO)˙+ facilitates the approach of I3− ions to the TiO2 surface because of complexation between the charge-separated state and I3− ions, leading to faster charge recombination and thus resulting in a lower Voc value.3,6
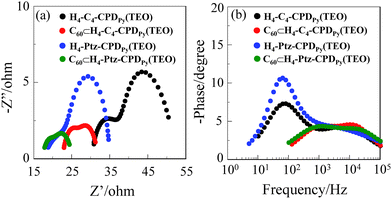 |
| Fig. 6 (a) Nyquist plots and (b) Bode phase plots of DSSCs based on H4-C4-CPDPy(TEO), C60⊂H4-C4-CPDPy(TEO), H4-Ptz-CPDPy(TEO), and C60⊂H4-Ptz-CPDPy(TEO). | |
Conclusions
In this work, to seek a direction in molecular design toward creating a new class of porphyrin dye sensitizers bearing pyridyl anchoring groups for achieving high dye loading and high surface coverage of the TiO2 electrode for dye-sensitized solar cells (DSSCs), cyclic free-base porphyrin dimers (H4-C4-CPDPy(TEO) and H4-Ptz-CPDPy(TEO)) linked by butadiyne or phenothiazine bearing 4-pyridyl groups and their C60 inclusion complexes (C60⊂H4-C4-CPDPy(TEO) and C60⊂H4-Ptz-CPDPy(TEO)) have been applied to DSSCs as the dye sensitizers. The Soret bands of C60⊂H4-C4-CPDPy(TEO) and C60⊂H4-Ptz-CPDPy(TEO) were redshifted with a decrease in intensity, whereas their Q bands were slightly redshifted but increased in intensity, compared to H4-C4-CPDPy(TEO) and H4-Ptz-CPDPy(TEO). These porphyrin dimers are adsorbed on the TiO2 surface through the formations of hydrogen bonding of pyridyl groups and/or pyridinium ion at Brønsted acid sites on the TiO2 surface. It was found that the adsorption amount of the porphyrin dimers adsorbed on TiO2 film is 2.0 × 1017 molecules per cm2, that is, the adsorption amount of porphyrin unit is 4.0 × 1017 cm−2, which is higher than those (<2.0 × 1017 molecules per cm2) of porphyrin dye sensitizers and D–π–A dye sensitizer bearing pyridyl groups reported so far. The higher photovoltaic performance for DSSC based on H4-Ptz-CPDPy(TEO) relative to H4-C4-CPDPy(TEO) may be attributed to the efficient electron injection from the photoexcited dye (1H4-Ptz-CPDPy(TEO)*) to the CB of TiO2 because the phenothiazine unit possessing electron donating ability can provide a unidirectional flow of electrons toward the pyridyl anchoring group upon photoexcitation of the porphyrin. On the other hand, the photovoltaic performances of DSSC based on C60⊂H4-C4-CPDPy(TEO) and C60⊂H4-Ptz-CPDPy(TEO) are lower than those of H4-C4-CPDPy(TEO) and H4-Ptz-CPDPy(TEO). The transient absorption spectroscopy and electrochemical measurements revealed that the lower photovoltaic performances for DSSC based on the C60 inclusion complexes would be attributed to the formation of charge-separated state between the photoexcited porphyrin and C60, leading to low electron-injection efficiency from the photoexcited porphyrin to TiO2 electrode. Consequently, this work provides that the cyclic porphyrin dimers with four pyridyl anchoring groups possessing bonding ability to the two points on Brønsted acid sites on TiO2 surface, which can achieve the high dye loading and the high surface coverage of the TiO2 electrode, would be expected to be one of the most promising classes of porphyrin dye sensitizers with pyridyl group.
Experimental
General
Infrared (IR) absorption spectra were recorded on a Perkin Elmer Spectrum One FT-IR spectrometer by ATR method and a Bio-Rad FTS6000 spectrometer by KBr disk method. Ultraviolet/visible (UV/vis) absorption spectra were observed with Shimadzu UV-3150 and UV-2500PC spectrophotometers. Nuclear magnetic resonance (NMR) spectra were recorded on a Bruker AVANCE III 600 spectrometer. The resonance frequencies are 600 MHz for 1H and 151 MHz for 13C. Chemical shifts were reported as δ values in ppm relative to tetramethylsilane. High-resolution fast atom bombardment mass spectra (HR-FAB-MS) were measured with 3-nitrobenzyl alcohol (NBA) as a matrix and recorded on a JEOL LMS-HX-110 spectrometer. Melting point (Mp) measurements were carried out on a Yanaco MP-S3 melting point meter. Cyclic voltammetry (CV) and differential pulse voltammetry (DPV) were carried out on an ALS630C (BAS, Japan) electrochemical analyzer in deaerated benzonitrile containing 0.1 M tetra-n-butylammonium hexafluorophosphate (Bu4NPF6) as a supporting electrolyte at room temperature under an Ar atmosphere. A conventional three-electrode cell was used with a platinum working electrode (surface area of 0.3 mm2) and a platinum wire as a counter electrode. The measured potentials were recorded with respect to an Ag/AgNO3 (0.01 M) reference electrode. All potentials (vs. Ag+/Ag) were converted to values with respect to that of the Fc+/Fc redox couple, which was measured under the same conditions. The highest occupied molecular orbital (HOMO) and lowest unoccupied molecular orbital (LUMO) energy levels of porphyrin dimers were evaluated from the spectral analyses and the DPV data. The HOMO energy level was evaluated from the oxidation wave for porphyrin dimers. The LUMO energy level was estimated from the singlet excited energy of these porphyrin dimers (1.90 eV) based on the Q absorption band nm and fluorescence band in benzonitrile.
Synthesis
5,15-Bis{3-[2-(2-ethoxyethoxy)ethoxy]-5-[(trimethylsilyl)ethynyl]phenyl}-10,20-di{4-pyridyl}porphine (1).
A solution of 3-[2-(2-ethoxyethoxy)ethoxy]-5-[(trimethylsilyl)ethynyl]benz-aldehyde22 (4.63 g, 13.8 mmol) and meso-(4-pyridyl)-dipyrromethane23 (3.08 g, 13.9 mmol) in CH2Cl2 (1.4 L) was degassed with a stream of N2 for 30 min. To this solution, trifluoroacetic acid (TFA) (12.3 mL, 166 mmol) was added and stirred under a N2 atmosphere. After 30 min, the resultant mixture was neutralized with NEt3 (23 mL, 0.17 mol) and then 2,3-dichloro-5,6-dicyano-1,4-benzoquinone (DDQ) (4.7 g, 21 mmol) dissolved in benzene (250 mL) was added to the mixture. After an overnight stirring, the resultant mixture was purified by column chromatography (silica-gel, CHCl3/EtOH = 50/1) then recrystallized from CHCl3/i-PrOH to give 1 as a purple solid (1.27 g, 18%). 1H NMR (CDCl3, 600 MHz): δ −2.91 (br-s, 2H, –NH), 0.27 (s, 18H, –Si(CH3)3), 1.18 (t, J = 7.2 Hz, 6H, –O(CH2CH2O)2CH2C
3), 3.52 (q, J = 7.2 Hz, 4H, –O(CH2CH2O)2C
2CH3), 3.63 (dd, J = 4.8 and 6.0 Hz, 4H, –O(CH2)2OCH2C
2OC2H5), 3.76 (t, J = 4.8 Hz, 4H, –O(CH2)2OC
2CH2OC2H5), 3.96 (t, J = 4.8 Hz, 4H, –OCH2C
2O(CH2)2OC2H5), 4.36 (t, J = 4.8 Hz, 4H, –OC
2CH2O(CH2)OC2H5), 7.47 (br-s, 2H, Ar–H), 7.75 (br-s, 2H, Ar–H), 7.93 (br-s, 2H. Ar–H), 8.17 (d, J = 5.4 Hz, 4H, Ar–H), 8.81 (d, J = 4.2 Hz, 4H, pyrrole β–H), 8.92 (d, J = 4.8 Hz, 4H, pyrrole β–H), 9.06 (d, J = 6.0 Hz, 4H, Ar–H); 13C NMR (CDCl3, 151 MHz): δ 0.1, 15.3, 66.9, 68.1, 69.9, 70.0, 71.2, 94.7, 104.9, 117.2, 117.4, 119.7, 122.6, 122.6, 129.6, 131.1, 143.1, 148.5, 150.3, 157.2; HR-FAB-MS (NBA): m/z calcd for C64H68N6O6Si2: 1072.4739; found: 1072.4718; UV/vis (CHCl3): λmax (ε, cm−1 M−1) 421 (392
000), 515 (18
200), 549 (5400), 588 (5400), 645 (2100); IR (KBr): ν = 3439, 3317, 3092, 3022, 2956, 2928, 2867, 2714, 2614, 2533, 2156, 1591, 1582, 1476, 1420, 1404, 352, 1323, 1297, 1247, 1196, 1173, 1161, 1114, 1069, 976, 927, 847, 800, 788, 760, 730, 697, 660, 563, 411 cm−1; Mp: >300 °C.
{5,15-Di{3-[2-(2-ethoxyethoxy)ethoxy]-5-ethynyl]phenyl}-10,20-di{4-pyridyl}porphinato}zinc(II) (2).
Zn(OAc)2·2H2O (2.4 g, 11 mmol) dissolved in MeOH (60 mL) was added to a solution of 1 (1.16 g, 1.08 mmol) in CH2Cl2 (1.1 L). The resultant mixture was refluxed overnight under N2 atmosphere and then cooled to room temperature. The reaction mixture was neutralized with saturated aqueous solution of NaHCO3 (200 mL), washed with water (200 mL, 2 times), dried over Na2SO4 and evaporated. The residue and KF·2H2O (0.63 g, 6.7 mmol) were dissolved in DMF (40 mL) and the mixture was stirred overnight under a N2 atmosphere. After the stirring, the resultant mixture was diluted with CHCl3 (100 mL) and washed with water (500 mL, 2 times). The organic layer was dried over Na2SO4 and evaporated to give the product as a purple solid (1.00 g, 99%). 1H NMR (pyridine-d5, 600 MHz): δ 1.00 (t, J = 7.2 Hz, 6H, –O(CH2CH2O)2CH2C
3), 3.33 (q, J = 6.6 Hz, 4H, –O(CH2CH2O)2C
2CH3), 3.52 (t, J = 4.8 Hz, 4H, –O(CH2)2OCH2C
2OC2H5), 3.67 (t, J = 4.8 Hz, 4H, –O(CH2)2OC
2CH2OC2H5), 3.87 (t, J = 4.8 Hz, 4H, –OCH2C
2O(CH2)2OC2H5), 4.15 (s, 2H, –C
CH) 4.31 (t, J = 4.8 Hz, 4H, –OC
2CH2O(CH2)OC2H5), 7.68 (d, J = 0.6 Hz, 2H, Ar–H), 8.05 (br-s, 2H, Ar–H), 8.23 (d, J = 4.2 Hz, 2H, Ar–H), 8.26 (d, J = 4.2 Hz, 4H, Ar–H), 9.02 (d, J = 4.8 Hz, 4H, pyrrole β–H), 9.11 (d, J = 5.4 Hz, 4H, Ar–H), 9.15 (d, J = 4.8 Hz, 4H, pyrrole β–H); 13C NMR (pyridine-d5, 151 MHz): δ 15.5, 66.5, 68.5, 70.0, 70.3, 71.2, 79.6, 84.5, 117.6, 118.7, 120.6, 122.0, 130.1, 131.7, 132.2, 132.9, 145.2, 148.7, 149.8, 150.3, 150.7, 151.4, 157.7; HR-FAB-MS (NBA): m/z calcd for C58H50N6O6Zn: 990.3083; found: 990.3082; UV/vis (CHCl3): λmax (ε, cm−1 M−1) 425 (418
000), 555 (16
200), 595 (2400); IR (KBr): ν = 3433, 3283, 2972, 2928, 2872, 1592, 1581, 1540, 1523, 1488, 1456, 1419, 1344, 1321, 1298, 1283, 1246, 1204, 1181, 1113, 1071, 1048, 998, 938, 874, 819, 796, 763, 719, 675, 665, 569, 545, 436, 419 cm−1; Mp: >300 °C.
H4-C4-CPDPy(TEO)
.
The free-base dimer was prepared from 2 (397 mg, 0.4 mmol) with CuCl (8.0 g, 80 mmol) in pyridine (1.2 L) according to the reported procedure.20a,d The crude product was purified by flash column chromatography (silica-gel, CHCl3/EtOH = 100/1 to 10/1) and recrystallized from CHCl3/i-PrOH to give the product as a purple powder (48 mg, 13%). 1H NMR (CDCl3, 600 MHz): δ −3.04 (br-s, 4H, –NH), 1.21 (t, J = 7.2 Hz, 12H, –O(CH2CH2O)2CH2C
3), 3.55 (q, J = 7.2 Hz, 8H, –O(CH2CH2O)2C
2CH3), 3.67 (dd, J = 4.8 and 6.0 Hz, 8H, –O(CH2)2OCH2C
2OC2H5), 3.81 (dd, J = 4.8 and 6.0 Hz, 8H, –O(CH2)2OC
2CH2OC2H5), 4.03 (t, J = 4.8 Hz, 8H, –OCH2C
2O(CH2)2OC2H5), 4.42 (t, J = 4.8 Hz, 8H, –OC
2CH2O(CH2)OC2H5), 6.88 (s, 4H, Ar–H), 7.25 (dd, J = 0.6 and 1.8 Hz, 4H, Ar–H), 7.94 (br-s, 8H, Ar–H), 8.22 (t, J = 1.8 Hz, 4H, Ar–H), 8.62 (d, J = 4.2 Hz, 8H, pyrrole β–H), 8.70 (d, J = 4.2 Hz, 8H, pyrrole β–H), 8.96 (br-s, 8H, Ar–H); 13C NMR (CDCl3, 151 MHz): δ 15.3, 66.9, 68.3, 70.0, 70.1, 71.3, 74.5, 83.1, 114.9, 117.1, 118.6, 120.4, 120.8, 129.3, 135.3, 143.0, 148.4, 149.9, 157.8; HR-FAB-MS (NBA): m/z calcd for C116H100N12O12: 1852.7584; found: 1852.7545; UV/vis (PhCN): λmax (ε, cm−1 M−1); 422 (646
000), 517 (30
800), 551 (9800), 590 (9600), 647 (4300); IR (ATR): ν = 3312, 2971, 2920, 2865, 1578, 1474, 1455, 1415, 1372, 1348, 1285, 1254, 1221, 1195, 1172, 1108, 1062, 993, 974, 930, 871, 849, 797, 728, 692, 659, 563, 540, 517, 467, 411 cm−1; Mp: >300 °C.
Preparation of DSSCs
The TiO2 paste (JGC Catalysts and Chemicals Ltd., PST-18NR) was deposited on a fluorine-doped-tin-oxide (FTO) substrate by doctor-blading, and sintered for 50 min at 450 °C. The 9 μm thick TiO2 electrode was immersed into 0.1 mM porphyrin dimer solution in chloroform for 15 hours enough to adsorb the dye sensitizers. The DSSCs were fabricated by using the TiO2 electrode (0.5 × 0.5 cm2 in photoactive area) thus prepared, Pt-coated glass as a counter electrode, and a solution of 0.05 M iodine, 0.1 M lithium iodide, and 0.6 M 1,2-dimethyl-3-propylimidazolium iodide in acetonitrile as electrolyte. The photocurrent–voltage characteristics were measured using a potentiostat under a simulated solar light (AM 1.5, 100 mW cm−2). IPCE spectra were measured under monochromatic irradiation with a tungsten-halogen lamp and a monochromator. The amount of adsorbed dye on TiO2 nanoparticles was determined form the calibration curve by absorption spectral measurement of the concentration change of the porphyrin dye solution before and after adsorption. Absorption spectra of the dyes adsorbed on TiO2 nanoparticles were recorded on the porphyrin dyes-adsorbed TiO2 film (thickness of 3 μm) in the transmission mode with a calibrated integrating sphere system. Electrochemical impedance spectroscopy (EIS) for DSSCs in the dark under a forward bias of −0.60 V with a frequency range of 10 mHz to 100 kHz was measured with a AMETEK Versa STAT 3.
Acknowledgements
This work was supported by Grants-in-Aid (No. 20108009 and 15K05432 to F. T. and No. 15H03859 to Y. O.) from Ministry of Education, Culture, Sports, Science and Technology of Japan and Research Grants to F. T. from Tokuyama Science and Technology Foundation and Iketani Science and Technology Foundation.
Notes and references
- B. O'Regan and M. Grätzel, Nature, 1991, 353, 737 CrossRef.
- A. Hagfeldt, G. Boschloo, L. Sun, L. Kloo and H. Pettersson, Chem. Rev., 2010, 110, 6595 CrossRef CAS PubMed.
-
(a) Z. Ning and H. Tian, Chem. Commun., 2009, 5483 RSC;
(b) Z. Ning, Y. Fu and H. Tian, Energy Environ. Sci., 2010, 3, 1170 RSC.
- A. Mishra, M. K. R. Fischer and P. Bäuerle, Angew. Chem., Int. Ed., 2009, 48, 2474 CrossRef CAS PubMed.
- Y. Ooyama and Y. Harima, Eur. J. Org. Chem., 2009, 18, 2903 CrossRef.
- Y. Ooyama and Y. Harima, ChemPhysChem, 2012, 13, 4032 CrossRef CAS PubMed.
- N. Manfredi, B. Cecconi and A. Abbotto, Eur. J. Org. Chem., 2014, 7069 CrossRef CAS.
-
(a) X. Wang, J. Yang, H. Yu, F. Li, L. Fan, W. Sun, Y. Liu, Z. Y. Koh, J. Pan, W.-L. Yim, L. Yan and Q. Wang, Chem. Commun., 2014, 50, 3965 RSC;
(b) S.-G. Li, K.-J. Jiang, J.-H. Huang, L.-M. Yang and Y.-L. Song, Chem. Commun., 2014, 50, 4309 RSC;
(c) D. K. Panda, F. S. Goodson, S. Ray and S. Saha, Chem. Commun., 2014, 50, 5358 RSC;
(d) K. Kakiage, Y. Aoyama, T. Yano, T. Otsuka, T. Kyomen, M. Unno and M. Hanaya, Chem. Commun., 2014, 50, 6379 RSC;
(e) A. Amacher, C. Yi, J. yang, M. P. Bircher, Y. Fu, M. Cascella, M. Grätzel, S. Decurtins and S.-X. Liu, Chem. Commun., 2014, 50, 6540 RSC;
(f) J. Yang, P. Ganesan, J. Teuscher, T. Moehl, Y. J. Kim, C. Yi, P. Comte, K. Pei, T. W. Holcombe, M. K. Nazeeruddin, J. Hua, S. K. Zakeeruddin, H. Tian and M. Grätzel, J. Am. Chem. Soc., 2014, 136, 5772 Search PubMed.
-
(a) T. Ikeuchi, H. Nomoto, N. Masaki, M. J. Griffith, S. Mori and M. Kimura, Chem. Commun., 2014, 50, 1941 RSC;
(b) R. Agosta, R. Grisorio, L. De Marco, G. Romanazzi, G. P. Suranna, G. Gigli and M. Manca, Chem. Commun., 2014, 50, 9451 RSC;
(c) A. Dessi, M. Calamante, A. Mordini, M. Peruzzin, A. Sinicropi, R. Basosi, F. F. de Biani, M. Taddei, D. Colonna, A. D. Carlo, G. Reginato and L. Zani, Chem. Commun., 2014, 50, 13952 RSC;
(d) X. Sun, Y. Wang, X. Li, H. Ågren, W. Zhu, H. Tian and Y. Xie, Chem. Commun., 2014, 50, 15609 RSC.
-
(a) H. Imahori, T. Umeyama and S. Ito, Acc. Chem. Res., 2009, 42, 1809 CrossRef CAS PubMed;
(b) S. Eu, S. Hayashi, T. Umeyama, Y. Matano, Y. Araki and H. Imahori, J. Phys. Chem. C, 2008, 112, 4396 CrossRef CAS;
(c) A. Kira, Y. Matsubara, H. Iijima, T. Umeyama, Y. Matano, S. Ito, M. Niemi, N. V. Tkachenko, H. Lemmetyinen and H. Imahori, J. Phys. Chem. C, 2010, 114, 11293 CrossRef CAS;
(d) H. Imahori, H. Iijima, H. Hayashi, Y. Toude, T. Umeyama, Y. Matano and S. Ito, ChemSusChem, 2011, 4, 797 CrossRef CAS PubMed;
(e) K. Kurotobi, Y. Toude, K. Kawamoto, Y. Fujimori, S. Ito, P. Chabera, V. Sundström and H. Imahori, Chem.–Eur. J., 2013, 19, 17075 CrossRef CAS PubMed;
(f) T. Higashino and H. Imahori, Dalton Trans., 2015, 44, 448 RSC.
-
(a) T. Bessho, S. M. Zakeeruddin, C.-Y. Yeh, E. W.-G. Diau and M. Grätzel, Angew. Chem., Int. Ed., 2010, 49, 6646 CrossRef CAS PubMed;
(b) A. Yella, H.-W. Lee, H. N. Tsao, C. Yi, A. K. Chandiran, M. K. Nazeeruddin, E. W.-G. Diau, C.-Y. Yeh, S. M. Zakeeruddin and M. Grätzel, Science, 2011, 334, 629 CrossRef CAS PubMed;
(c) L.-L. Li and E. W.-G. Diau, Chem. Soc. Rev., 2013, 42, 291 RSC;
(d) S. Mathew, A. Yella, P. Gao, R. Humphry-Baker, B. F. E. Curchod, N. Ashari-Astani, I. Tavernelli, U. Rothlisberger, M. K. Nazeeruddin and M. Grätzel, Nat. Chem., 2014, 6, 242 CrossRef CAS PubMed;
(e) A. Yella, C.-L. Mai, S. M. Zakeeruddin, S.-N. Chang, C.-H. Hsieh, C.-Y. Yeh and M. Grätzel, Angew. Chem., Int. Ed., 2014, 53, 2973 CrossRef CAS PubMed.
-
(a) M. V. Martínez-Díaz, G. de la Torre and T. Torres, Chem. Commun., 2010, 46, 7090 RSC;
(b) M. J. Griffith, K. Sunahara, P. Wagner, K. Wagner, G. G. Wallace, D. L. Officer, A. Furube, R. Katoh, S. Mori and A. J. Mozer, Chem. Commun., 2012, 48, 4145 RSC;
(c) K. Ladomenou, T. N. Kitsopoulos, G. D. Sharma and A. G. Coutsolelos, RSC Adv., 2014, 4, 21379 RSC.
-
(a) Y.-C. Chang, C.-L. Wang, T.-Y. Pan, S.-H. Hong, C.-M. Lan, H.-H. Kuo, C.-F. Lo, H.-Y. Hsu, C.-Y. Lin and E. W.-G. Diau, Chem. Commun., 2011, 47, 8910 RSC;
(b) M. Ishida, D. Hwang, Y. B. Koo, J. Sung, D. Y. Kim, J. L. Sessler and D. Kim, Chem. Commun., 2013, 49, 9164 RSC;
(c) C. Lo, S. Hsu, C. Wang, Y. Cheng, H. Lu, E. W. Diau and C. Lin, J. Phys. Chem. C, 2010, 114, 12018 CrossRef CAS;
(d) J. Lu, X. Xu, K. Cao, J. Cui, Y. Zhang, Y. Shen, X. Shi, L. Liao, Y. Cheng and M. Wang, J. Mater. Chem. A, 2013, 1, 10008 RSC;
(e) R. B. Ambre, G.-F. Chang, M. R. Zanwar, C.-F. Yao, E. W.-G. Diau and C.-H. Hung, Chem.–Asian J., 2013, 8, 2144 CrossRef CAS PubMed;
(f) R. B. Ambre, G.-F. Chang and C.-H. Hung, Chem. Commun., 2014, 50, 725 RSC.
-
(a) C.-L. Wang, Y.-C. Chang, C.-M. Lan, C.-F. Lo, E. Wei-Guang Diau and C.-Y. Lin, Energy Environ. Sci., 2011, 4, 1788 RSC;
(b) C.-H. Wu, T.-Y. Pan, S.-H. Hong, C.-L. Wang, H.-H. Kuo, Y.-Y. Chu, E. W.-G. Diau and C.-Y. Lin, Chem. Commun., 2012, 48, 4329 RSC;
(c) H.-P. Lu, C.-L. Mai, C.-Y. Tsia, S.-J. Hsu, C.-P. Hsieh, C.-L. Chiu, C.-Y. Yeh and E. W.-G. Diau, Phys. Chem. Chem. Phys., 2009, 11, 10270 RSC;
(d) C.-H. Wu, M.-C. Chen, P.-C. Su, H.-H. Kuo, C.-L. Wang, C.-Y. Lu, C.-H. Tsai, C.-C. Wu and C.-Y. Lin, J. Mater. Chem. A, 2014, 2, 991 RSC;
(e) J. M. Ball, N. K. S. Davis, J. D. Wilkinson, J. Kirkpatrick, J. Teuscher, R. Gunning, H. L. Anderson and H. J. Snaith, RSC Adv., 2012, 2, 6846 RSC;
(f) C.-L. Mai, W.-K. Huang, H.-P. Lu, C.-W. Lee, C.-L. Chiu, Y.-R. Liang, E. W.-G. Diau and C.-Y. Yeh, Chem. Commun., 2010, 46, 809 RSC.
-
(a) K. Sunahara, M. J. Griffith, T. Uchiyama, P. Wagner, D. L. Officer, G. G. Wallace, A. J. Mozer and S. Mori, ACS Appl. Mater. Interfaces, 2013, 5, 10824 CrossRef CAS PubMed;
(b) T. Hamamura, J. T. Dy, K. Tamaki, J. Nakazaki, S. Uchida, T. Kubo and H. Segawa, Phys. Chem. Chem. Phys., 2014, 16, 4551 RSC;
(c) H.-P. Wu, Z.-W. Ou, T.-Y. Pan, C.-M. Lan, W.-K. Huang, H.-W. Lee, N. M. Reddy, C.-T. Chen, W.-S. Chao, C.-Y. Yeh and E. W.-G. Diau, Energy Environ. Sci., 2012, 5, 9843 RSC;
(d) C.-L. Wang, J.-Y. Hu, C.-H. Wu, H.-H. Kuo, Y.-C. Chang, Z.-J. Lan, H.-P. Wu, E. Wei-Guang Diau and C.-Y. Lin, Energy Environ. Sci., 2014, 7, 1392 RSC;
(e) J. Luo, M. Xu, R. Li, K.-W. Huang, C. Jiang, Q. Qi, W. Zeng, J. Zhang, C. Chi, P. Wang and J. Wu, J. Am. Chem. Soc., 2014, 136, 265 CrossRef CAS PubMed.
-
(a) Y. Ooyama, S. Inoue, R. Asada, G. Ito, K. Kushimoto, K. Komaguchi, I. Imae and Y. Harima, Eur. J. Org. Chem., 2010, 92 CrossRef CAS;
(b) Y. Ooyama, S. Inoue, T. Nagano, K. Kushimoto, J. Ohshita, I. Imae, K. Komaguchi and Y. Harima, Angew. Chem., Int. Ed., 2011, 50, 7429 CrossRef CAS PubMed;
(c) Y. Ooyama, T. Nagano, S. Inoue, I. Imae, K. Komaguchi, J. Ohshita and Y. Harima, Chem.–Eur. J., 2011, 17, 14837 CrossRef CAS PubMed;
(d) Y. Ooyama, N. Yamaguchi, I. Imae, K. Komaguchi, J. Ohshita and Y. Harima, Chem. Commun., 2013, 49, 2548 RSC;
(e) Y. Ooyama, Y. Hagiwara, T. Mizumo, Y. Harima and J. Ohshita, New J. Chem., 2013, 37, 2479 RSC;
(f) Y. Ooyama, T. Sato, Y. Harima and J. Ohshita, J. Mater. Chem. A, 2014, 2, 3293 RSC.
-
(a) Y. Harima, T. Fujita, Y. Kano, I. Imae, K. Komaguchi, Y. Ooyama and J. Ohshita, J. Phys. Chem. C, 2013, 117, 16364 CrossRef CAS;
(b) N. Shibayama, H. Ozawa, M. Abe, Y. Ooyama and H. Arakawa, Chem. Commun., 2014, 50, 6398 RSC.
-
(a) J. Lu, X. Xu, Z. Li, k. Cao, J. Cui, Y. Zhang, Y. Shen, Y. Li, J. Zhu, S. Dai, W. Chjen, Y. Cheng and M. Wang, Chem.–Asian J., 2013, 8, 956 CrossRef CAS PubMed;
(b) T. Sakurada, Y. Arai and H. Segawa, RSC Adv., 2014, 4, 13201 RSC;
(c) D. Daphnomili, G. Landrou, P. Singh, A. Thomas, K. Yesudas, B. K. G. D. Sharma and A. G. Goutsolelos, RSC Adv., 2012, 2, 12899 RSC;
(d) D. Daphnomili, G. D. Sharma, S. Biswas, T. K. R. Justin and A. G. Goutsolelos, J. Photochem. Photobiol., A, 2013, 253, 88 CrossRef CAS;
(e) C. Stangel, A. Bagaki, P. A. Angaridis, G. Charalambidis, G. D. Sharma and A. G. Coutsolelos, Inorg. Chem., 2014, 53, 11871 CrossRef CAS PubMed.
-
(a) M.-D. Zhang, H.-X. Xie, X.-H. Ju, L. Qin, Q.-X. Yang, H.-G. Zheng and X.-F. Zhou, Phys. Chem. Chem. Phys., 2013, 15, 634 RSC;
(b) L. Wang, X. yang, S. Li, M. Cheng and L. Sun, RSC Adv., 2013, 3, 13677 RSC;
(c) J. Mao, D. Wang, S.-H. Liu, Y. Hang, Y. Xu, Q. Zhang, W. Wu, P.-T. Chou and J. Hua, Asian J. Org. Chem., 2014, 3, 153 CrossRef CAS;
(d) J. Massin, L. Ducasse, T. Toupance and C. Olivier, J. Phys. Chem. C, 2014, 118, 10677 CrossRef CAS;
(e) L. Zhang, J. M. Cole and C. Dai, ACS Appl. Mater. Interfaces, 2014, 6, 7535 CrossRef CAS PubMed.
-
(a) H. Nobukuni, Y. Shimazaki, H. Uno, Y. Naruta, K. Okubo, T. Kojima, S. Fukuzumi, S. Seki, H. Sakai, T. Hasobe and F. Tani, Chem.–Eur. J., 2010, 16, 11611 CrossRef CAS PubMed;
(b) H. Nobukuni, T. Kamimura, H. Uno, Y. Shimazaki, Y. Naruta and F. Tani, Bull. Chem. Soc. Jpn., 2012, 85, 862 CrossRef CAS;
(c) K. Sakaguchi, T. Kamimura, H. Uno, S. Mori, S. Ozako, H. Nobukuni, M. Ishida and F. Tani, J. Org. Chem., 2014, 79, 2980 CrossRef CAS PubMed;
(d) T. Kamimura, K. Ohkubo, Y. Kawashima, H. Nobukuni, Y. Naruta, F. Tani and S. Fukuzumi, Chem. Sci., 2013, 4, 1451 RSC;
(e) T. Kamimura, K. Ohkubo, Y. Kawashima, S. Ozako, K. Sakaguchi, S. Fukuzumi and F. Tani, J. Phys. Chem. C, 2015, 119, 25634 CrossRef CAS.
-
(a) T. J. Dines, L. D. MacGregor and C. H. Rochester, Phys. Chem. Chem. Phys., 2001, 3, 2676 RSC;
(b) M. I. Zaki, M. A. Hasan, F. A. Al-Sagheer and L. Pasupulety, Colloids Surf., A, 2001, 190, 261 CrossRef CAS;
(c) O. Kasende and T. Zeegers-Huyskens, J. Phys. Chem., 1984, 88, 2132 CrossRef CAS;
(d) H. Takahashi, K. Mamola and E. K. Plyler, J. Mol. Spectrosc., 1966, 21, 217 CrossRef CAS;
(e) M. A. Montańez, I. L. Tocón, J. C. Otero and J. I. Marcos, J. Mol. Struct., 1999, 482–483, 201 CrossRef.
- T. Kamimura, M. Komura, H. Komiyama, T. Iyoda and F. Tani, Chem. Commun., 2015, 51, 1685 RSC.
- C. Ruzié, L. Michaudet and B. Boitrel, Tetrahedron Lett., 2002, 43, 7423 CrossRef.
Footnote |
† Electronic supplementary information (ESI) available: 1H NMR and 13C NMR for compounds 1, 2 and H4-C4-CPDPy(TEO). See DOI: 10.1039/c6ra01131d |
|
This journal is © The Royal Society of Chemistry 2016 |
Click here to see how this site uses Cookies. View our privacy policy here.