DOI:
10.1039/C6QO00273K
(Research Article)
Org. Chem. Front., 2016,
3, 1319-1325
Enantioselective β-alkylation of pyrroles with the formation of an all-carbon quaternary stereocenter†
Received
16th June 2016
, Accepted 17th August 2016
First published on 18th August 2016
Abstract
A substitutionally and configurationally inert octahedral chiral-at-metal iridium complex is reported to be an efficient catalyst for the enantioselective Friedel–Crafts alkylation of 2,5-disubstituted pyrroles at the β-position using nitroacrylates as electrophiles. Catalysis is mediated through the ligand sphere of the bis-cyclometalated iridium complex by forming hydrogen bonds and van-der-Waals interactions with the substrates, providing β-alkylated pyrroles regioselectively in 75–98% yield and with 86–99% enantiomeric excess (19 examples). The products are versatile chiral building blocks as demonstrated by the reduction of the nitro group with complete retention of the chiral information.
Introduction
Pyrroles are important structural motifs found in many natural products and other bioactive compounds.1 Due to the electron-rich nature of the five-membered heteroaromatic ring system, electrophilic aromatic substitutions (SEAr) serve as the predominant synthetic method for their functionalization.2 Within the family of SEAr reactions, enantioselective Friedel–Crafts alkylations have been used frequently for the introduction of adjacent stereogenic carbons. However, the vast majority of reports deal with the alkylation at the more nucleophilic α-position,3 whereas only a few publications are dedicated to the introduction of stereogenic carbons at the β-position.4–8 With respect to efficient catalytic, enantioselective Friedel–Crafts β-alkylations, Nakada and coworkers recently reported the reaction of 1,2,5-trimethyl pyrrole at the β-position with a cyclic α-alkylidene β-oxo imide using a bisoxazoline-Cu(OTf)2 catalyst,7 whereas Feng and coworkers reported the β-alkylation of 2,5-dimethyl pyrrole with β,γ-unsaturated α-ketoesters using their powerful chiral N,N′-dioxide ligands in combination with Ni(OTf)2 (Fig. 1).8 On the other hand, although some recent advances have been achieved in the enantioselective construction of all-carbon quaternary stereocenters through asymmetric Friedel–Crafts C3-alkylation of indoles,9–16 related reactions with pyrroles have not been much explored.16
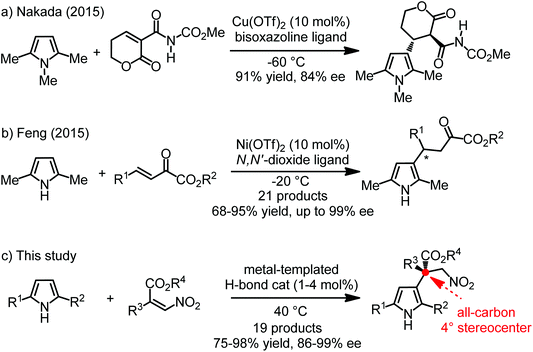 |
| Fig. 1 Catalytic enantioselective Friedel–Crafts alkylation of pyrroles at the β-position. | |
We recently introduced substitutionally inert octahedral chiral-at-metal iridium complexes as a new class of asymmetric catalysts which exploit the globular geometry, rigid nature, and stereochemical options of octahedral compounds.15,17–23 While the central transition metal serves as a structural anchor and provides metal centrochirality, catalysis is mediated solely by functional groups within the organic ligand sphere.24–26 In a noteworthy application, we developed an enantioselective Friedel–Crafts β-alkylation of indoles with α-substituted-β-nitroacrylates catalyzed by such a metal-templated catalyst (Λ-MTC, Table 1) under the enantioselective construction of an all-carbon quaternary stereocenter.15 We were wondering if this strategy would be applicable to the more challenging β-alkylation of pyrroles, and we disclose herein our successful development of a catalytic, enantioselective Friedel–Crafts alkylation of pyrroles with the formation of an all-carbon quaternary stereocenter at the β-position.
Table 1 Optimization of conditions for the chiral quaternary carbon generating β-alkylation of 2,5-dimethyl 1-H pyrrole 1a with nitroacrylate 2a catalyzed by Λ-MTCa
Results and discussion
At the outset of the study 2,5-dimethyl 1-H pyrrole 1a and nitroacrylate 2a were chosen as the model substrates, and a previously developed catalyst Λ-MTC15 was applied directly in this catalytic enantioselective Friedel–Crafts β-alkylation of the pyrroles. To our delight, in the presence of 2 mol% of Λ-MTC, the desired β-alkylated pyrrole 3a containing an all-carbon quaternary stereocenter was afforded in good enantioselectivity (93% ee) while only modest conversion (62%) was afforded at 20 °C within 30 h (Table 1, entry 1). We optimized the reaction conditions including the ratio of the substrates, temperature, concentration and catalyst loading. As shown in entries 2–5 of Table 1, when the substrate ratio of nitroacrylate 2a was increased, an obvious improvement of the reaction rates was observed. We speculated that the excess of 2a restrained self-polymerization of the pyrrole contributing to the higher conversions. Afterwards, a combination of an increased concentration (2 M) with a higher temperature (40 °C) led to the optimal conditions and enabled the reaction to be completed in 16 h by affording 3a with 94% ee (entry 8). Finally, we found that the catalyst loading could be reduced to 1 mol% while still providing a satisfactory reaction time of 24 hours for 93% conversion and 94% ee (Table 1, entry 9).
With optimized conditions in hand, we next evaluated the scope of the enantioselective Friedel–Crafts β-alkylation of pyrroles. Fig. 2 reveals that starting from the 2,5-disubstituted 1-H pyrroles 1a–e with diverse steric properties, only 1 mol% of Λ-MTC was needed to catalyze the reaction with nitroacrylate 2a providing high yields (92–98%) and good to excellent enantioselectivities (94–97%) in 15–30 hours (products 3a–e). In addition, 1f bearing an electron-deficient but bulky phenyl substituent led to a much slower reaction, however, a good yield (90%) and excellent enantioselectivity (96% ee) were still obtained after increasing the catalyst loading to 4 mol% (product 3f). It is worth noting that if unsymmetrical 2,5-disubstituted pyrroles (R1 ≠ R2, 1c–g) were used, good to excellent regioselectivities (4
:
1 to single regioisomer) were observed (products 3c–g), which were closely related to the steric difference between the two α-substituents. For example, the catalytic transformations of 1e–g containing one methyl substituent and one bulky group (cyclohexyl or aryl) afforded only single regioisomers of the β-alkylated pyrroles (products 3e–g). However, 1h containing two aromatic substituents failed to provide the desired product even at an increased catalyst loading of 4 mol%.
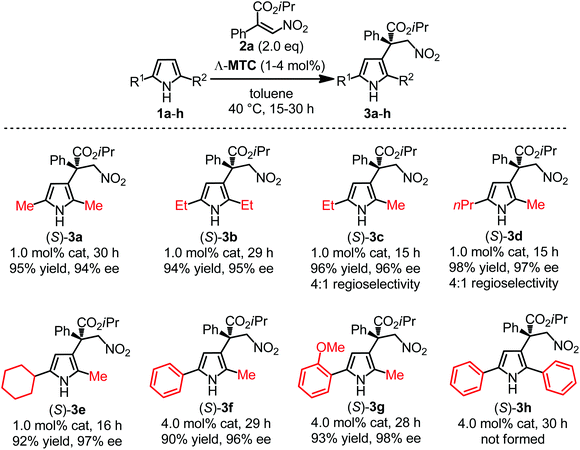 |
| Fig. 2 Substrate scope with respect to 2,5-disubstituted 1-H pyrroles. | |
Furthermore, aromatic nitroacrylates with electron donating and electron withdrawing substituents (2b–j) were well tolerated in the reactions by generating products 3i–q with 86–95% ee (Fig. 3). The reaction of aliphatic nitroacrylate 2k also afforded the desired product 3r smoothly in a yield of 96% and with 92% ee at a higher catalyst loading of 4 mol%. Additionally, besides the nitroacrylates, (E)-(3,3,3-trifluoro-1-nitroprop-1-en-2-yl)benzene 2m was also well compatible in the reaction by providing excellent yield (95%) and enantioselectivity (>99% ee) of 3t in an acceptable reaction time (30 h).
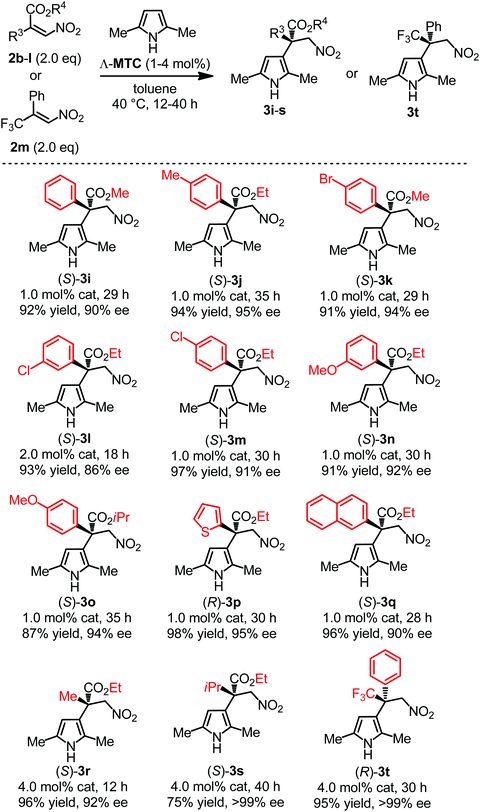 |
| Fig. 3 Substrate scope with respect to nitroacrylates. | |
The absolute stereochemistry of the all-carbon quaternary stereogenic center of the reaction product was determined by X-ray diffraction for the bromine-containing product 3u as the S-configuration (Fig. 4). Based on this assignment, a transition state is proposed as shown in Fig. 5, in which the nitroacrylate (yellow) forms a double hydrogen bond with the pyrazole amide moiety through the two oxygen atoms of the nitro group, whereas the 2,5-disubstituted pyrrole (green) forms a single hydrogen bond with one of the carboxamide moieties of the catalyst Λ-MTC (gray). This promotes a stacking of the pyrrole on top of the nitroacrylate and brings the pyrrole C-3 at a van-der-Waals distance to the β-carbon of the nitroalkene and placing the nucleophile in perfect position for the Re face attack on the electrophile, thereby rationalizing the formation of the observed S-configured product. Besides hydrogen bond interactions, we propose additional attractive weak interactions in the form of van-der-Waals contacts between one of the carbazole moieties of the catalyst and the nitroacrylate substrate, helping to stabilize the desired binding mode of the nitroacrylate. Additional steric constraints further contribute to high regio- and enantioselectivities.
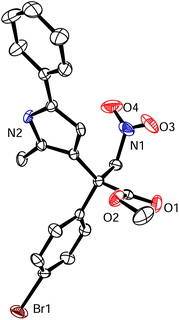 |
| Fig. 4 Crystal structure of pyrrole 3u featuring an all-carbon quaternary stereocenter. The absolute configuration was assigned as S. The compound was synthesized following the standard procedure with 4.0 mol% of Λ-MTC at 40 °C for 30 hours, affording (S)-3u in a yield of 93% with 94% ee. | |
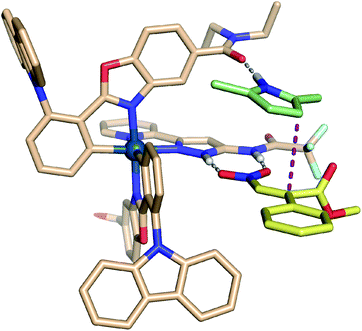 |
| Fig. 5 Proposed transition state of the hydrogen-bonded ternary complex. The distance between C-3 of the pyrrole (green) and the β-carbon of the nitroalkene (yellow) is 3.4 Å. The ternary complex was built with the molecular modeling software Scigress (Fujitsu) and represented with the PyMOL Molecular Graphics system, version 1.3 Schrödinger, LLC. | |
To further gain insight into the mechanism, control experiments were performed to probe the hydrogen bond interactions proposed in the above ternary complex. N-Methyl protected 2,5-dimethylpyrrole (1a′) without a hydrogen bond donating group was first tested in the reaction with nitroacrylate 2a under the standard conditions. As expected, 4 mol% of Λ-MTC only provide product 3a′ in 71% yield and 48% ee within 36 h (Table 2, entry 2). Furthermore, when one equivalent of ethanol or dimethylacetamide was added to the reaction 1a + 2a → (S)-3a, not only the reaction rate but also the enantioselectivity severely declined (Table 2, entries 1–3), suggesting that the hydrogen-bond-acceptor-type additive competes with the catalyst for hydrogen bonding to pyrrole and thus interferes with the formation of the ternary complex. The addition of nitrobenzene slightly affected the enantioselectivities while significantly slowing down the reaction rate (entries 4–6). With 10 equiv. of additional nitrobenzene, the catalytic process only occurred at around a one-third reaction rate, which indicates a competition between nitrobenzene and the nitroacrylate substrate for the double hydrogen bond binding site at the catalyst (entry 7).
Table 2 Control experiments for probing the hydrogen bond interactions as demonstrated for the enantioselective β-alkylation of pyrrole 1a or 1a′ with nitroacrylate 2a catalyzed by Λ-MTCa
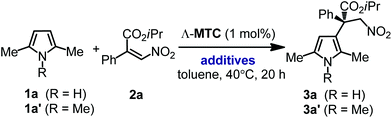
|
Entry |
Pyrroles |
Additives |
Conv.b (%) |
eec (%) |
Reaction conditions: a solution of pyrrole 1a or 1a′ (0.10 mmol), nitroacrylate 2a (47.0 mg, 0.20 mmol), catalyst Λ-MTC (2.2 mg, 0.0010 mmol) and respective additives in anhydrous toluene (0.050 mL) was stirred at 40 °C for 20 h under an argon atmosphere and reduced light.
Conversion determined by 1H NMR spectroscopy.
Enantiomeric excess determined by HPLC on a chiral stationary phase.
Isolated yield after 36 h.
|
1 |
1a
|
None |
78 |
94 |
2 |
1a′
|
None |
71d |
48 |
3 |
1a
|
EtOH (1 eq.) |
15 |
47 |
4 |
1a
|
DMF (1 eq.) |
5 |
15 |
5 |
1a
|
PhNO2 (1 eq.) |
64 |
94 |
6 |
1a
|
PhNO2 (3 eq.) |
53 |
95 |
7 |
1a
|
PhNO2 (10 eq.) |
28 |
96 |
Considering that chiral β-alkylated pyrrole moieties exist in many natural products and biologically active compounds,27 we next evaluated two convenient transformations of this class of Friedel–Crafts alkylation products with retention of the stereogenic configuration as demonstrated for (S)-3a (Fig. 6). For example, reduction of the nitro group with stoichiometric sodium borohydride and nickel chloride followed by N-Boc protection in methanol afforded the pyrrole-based β-amino acid derivative (S)-4a in 76% yield with 94% ee. Alternatively, when the protection step was performed in the presence of excess acetone, a Pictet–Spengler cascade reaction occurred to afford the bicyclic chiral β-amino ester (S)-5a without loss of any enantiopurity (94% ee).
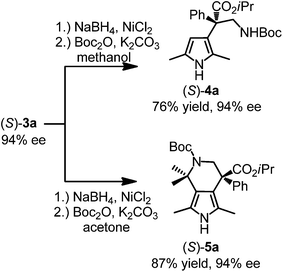 |
| Fig. 6 Follow-up chemistry by reduction of the nitro group. | |
Conclusions
In summary, we herein reported a regio- and highly enantioselective Friedel–Crafts β-alkylation of 2,5-disubstituted pyrroles using nitroacrylates as electrophiles to provide useful chiral building blocks containing all-carbon quaternary stereocenters. The methodology is not limited to nitroacrylates as demonstrated by using a CF3-substituted nitrostyrene derivative. In the developed organometallic catalyst, the iridium center has a purely structural role with substrate activation being mediated by the ligand sphere through hydrogen bond and van-der-Waals interactions.
Acknowledgements
We gratefully acknowledge funding from the National Natural Science Foundation of P. R. China (grant no. 21272192, 21472154 and 21572184), the Fundamental Research Funds for the Central Universities (grant no. 20720160027) and the National Thousand Talents Program of P. R. China, and the 985 Program of the Chemistry and Chemical Engineering disciplines of Xiamen University.
References
-
(a) A. Fürstner, Angew. Chem., Int. Ed., 2003, 42, 3582–3603 CrossRef PubMed;
(b) H. Fan, J. Peng, M. T. Hamann and J.-F. Hu, Chem. Rev., 2008, 108, 264–287 CrossRef CAS PubMed;
(c) S. Thirumalairajan, B. M. Pearce and A. Thompson, Chem. Commun., 2010, 46, 1797–1812 RSC.
- T. Tsuchimoto, Chem. – Eur. J., 2011, 17, 4064–4075 CrossRef CAS PubMed.
- Recent examples of Friedel–Crafts α-alkylation of pyrroles to create stereocenters, see:
(a) F. Guo, D. Chang, G. Lai, T. Zhu, S. Xiong, S. Wang and Z. Wang, Chem. – Eur. J., 2011, 17, 11127–11130 CrossRef CAS PubMed;
(b) T. Arai, A. Awata, M. Wasai, N. Yokoyama and H. Masu, J. Org. Chem., 2011, 76, 5450–5456 CrossRef CAS PubMed;
(c) L. Liu, H. Ma, Y. Xiao, F. Du, Z. Qin, N. Li and B. Fu, Chem. Commun., 2012, 48, 9281–9283 RSC;
(d) G. Zhang, Org. Biomol. Chem., 2012, 10, 2534–2536 RSC;
(e) Y. Liu, Z. Cao and H. Du, J. Org. Chem., 2012, 77, 4479–4483 CrossRef CAS PubMed;
(f) J. Gao, H. Wu, B. Xiang, W.-B. Yu, L. Han and Y.-X. Jia, J. Am. Chem. Soc., 2013, 135, 2983–2986 CrossRef CAS PubMed;
(g) A. K. Chittoory, G. Kumari, S. Mohapatra, P. P. Kundu, T. K. Maji, C. Narayana and S. Rajaram, Tetrahedron, 2014, 70, 3459–3465 CrossRef CAS;
(h) Y.-Z. Hua, X.-W. Han, X.-C. Yang, X. Song, M.-C. Wang and J.-B. Chang, J. Org. Chem., 2014, 79, 11690–11699 CrossRef CAS PubMed.
- M. Westermaier and H. Mayr, Chem. – Eur. J., 2008, 14, 1638–1647 CrossRef CAS PubMed.
- C. Berini, N. Pelloux-Léon, F. Minassian and J.-N. Denis, Org. Biomol. Chem., 2009, 7, 4512–4616 CAS.
- H. Yoon, D. A. Petrone and M. Lautens, Org. Lett., 2014, 16, 6420–6423 CrossRef CAS PubMed.
- H. Oyama and M. Nakada, Tetrahedron: Asymmetry, 2015, 26, 195–202 CrossRef CAS.
- Y. Zhang, N. Yang, X. Liu, J. Guo, X. Zhang, L. Lin, C. Hu and X. Feng, Chem. Commun., 2015, 51, 8432–8435 RSC.
- J.-R. Gao, H. Wu, B. Xiang, W.-B. Yu, L. Han and Y.-X. Jia, J. Am. Chem. Soc., 2013, 135, 2983–2986 CrossRef CAS PubMed.
- J.-Q. Weng, Q.-M. Deng, L. Wu, K. Xu, H. Wu, R.-R. Liu, J.-R. Gao and Y.-X. Jia, Org. Lett., 2014, 16, 776–779 CrossRef CAS PubMed.
- H. Wu, W.-J. Sheng, B. Chen, R.-R. Liu, J.-R. Gao and Y.-X. Jia, Synlett, 2015, 2817–2820 CAS.
- T. Arai, Y. Yamamoto, A. Awata, K. Kamiya, M. Ishibashi and M. A. Arai, Angew. Chem., Int. Ed., 2013, 52, 2486–2490 CrossRef CAS PubMed.
- K. Mori, M. Wakazawa and T. Akiyama, Chem.
Sci., 2014, 5, 1799–1803 RSC.
- H. Wu, R.-R. Liu, C. Shen, M.-D. Zhang, J. Gao and Y.-X. Jia, Org. Chem. Front., 2015, 2, 124–126 RSC.
- L.-A. Chen, X. Tang, J. Xi, W. Xu, L. Gong and E. Meggers, Angew. Chem., Int. Ed., 2013, 52, 14021–14025 CrossRef CAS PubMed.
- M.-H. Zhuo, G.-F. Liu, S.-L. Song, D. An, J. Gao, L. Zheng and S. Zhang, Adv. Synth. Catal., 2016, 358, 808–815 CrossRef CAS.
- L.-A. Chen, W. Xu, B. Huang, J. Ma, L. Wang, J. Xi, K. Harms, L. Gong and E. Meggers, J. Am. Chem. Soc., 2013, 135, 10598–10601 CrossRef CAS PubMed.
- H. Huo, C. Fu, C. Wang, K. Harms and E. Meggers, Chem. Commun., 2014, 50, 10409–10411 RSC.
- J. Ma, X. Ding, Y. Hu, Y. Huang, L. Gong and E. Meggers, Nat. Commun., 2014, 5, 4531 CAS.
- X. Ding, H. Lin, L. Gong and E. Meggers, Asian J. Org. Chem., 2015, 4, 434–437 CrossRef CAS.
- J. Liu, L. Gong and E. Meggers, Tetrahedron Lett., 2015, 56, 4653–4656 CrossRef CAS.
- Y. Hu, Z. Zhou and L. Gong, Org. Chem. Front., 2015, 2, 968–972 RSC.
- X. Ding, C. Tian, Y. Hu, L. Gong and E. Meggers, Eur. J. Org. Chem., 2016, 887–890 CrossRef CAS.
- For the design of metal-templated asymmetric catalysts from other groups, see:
(a) Y. N. Belokon, A. G. Bulychev, V. I. Maleev, M. North, I. L. Malfanov and N. S. Ikonnikov, Mendeleev Commun., 2004, 14, 249–250 CrossRef;
(b) Y. N. Belokon, V. I. Maleev, D. A. Kataev, I. L. Mal'fanov, A. G. Bulychev, M. A. Moskalenko, T. F. Saveleva, T. V. Skrupskaya, K. A. Lyssenko, I. A. Godovikov and M. North, Tetrahedron: Asymmetry, 2008, 19, 822–831 CrossRef CAS;
(c) C. Ganzmann and J. A. Gladysz, Chem. – Eur. J., 2008, 14, 5397–5400 CrossRef CAS PubMed;
(d) N. Kurono, K. Arai, M. Uemura and T. Ohkuma, Angew. Chem., Int. Ed., 2008, 47, 6643–6646 CrossRef CAS PubMed;
(e) N. Kurono, N. Nii, Y. Sakaguchi, M. Uemura and T. Ohkuma, Angew. Chem., Int. Ed., 2011, 50, 5541–5544 CrossRef CAS PubMed;
(f) Y. N. Belokon, V. I. Maleev, M. North, V. A. Larionov, T. F. Savel'yeva, A. Nijland and Y. V. Nelyubina, ACS Catal., 2013, 3, 1951–1955 CrossRef CAS;
(g) V. I. Maleev, M. North, V. A. Larionov, I. V. Fedyanin, T. F. Savel'yeva, M. A. Moscalenko, A. F. Smolyakov and Y. N. Belokon, Adv. Synth. Catal., 2014, 356, 1803–1810 CrossRef;
(h) K. G. Lewis, S. K. Ghosh, N. Bhuvanesh and J. A. Gladysz, ACS Cent. Sci., 2015, 1, 50–56 CrossRef CAS PubMed;
(i) V. A. Larionov, E. P. Markelova, A. F. Smol'yakov, T. F. Savel'yeva, V. I. Maleev and Y. N. Belokon, RSC Adv., 2015, 5, 72764–72771 RSC;
(j) A. Serra-Pont, I. Alfonso, C. Jimeno and J. Solà, Chem. Commun., 2015, 51, 17386–17389 RSC;
(k) A. Kumar, S. K. Ghosh and J. A. Gladysz, Org. Lett., 2016, 18, 760–763 CrossRef CAS PubMed;
(l) S. K. Ghosh, C. Ganzmann, N. Bhuvanesh and J. A. Gladysz, Angew. Chem., Int. Ed., 2016, 55, 4356–4360 CrossRef CAS PubMed.
- For reviews covering chiral-at-metal complexes in catalysis, see:
(a) H. Brunner, Angew. Chem., Int. Ed., 1999, 38, 1194–1208 CrossRef CAS;
(b) P. Knight and P. Scott, Coord. Chem. Rev., 2003, 242, 125–143 CrossRef CAS;
(c) M. Fontecave, O. Hamelin and S. Ménage, Top. Organomet. Chem., 2005, 15, 271–288 CrossRef CAS;
(d) E. B. Bauer, Chem. Soc. Rev., 2012, 41, 3153–3167 RSC;
(e) L. Gong, L.-A. Chen and E. Meggers, Angew. Chem., Int. Ed., 2014, 53, 10868–10874 CrossRef CAS PubMed;
(f) Z.-Y. Cao, W. D. G. Brittain, J. S. Fossey and F. Zhou, Catal. Sci. Technol., 2015, 5, 3441–3451 RSC.
- For different aspects of metal-centered chirality, see:
(a) U. Knof and A. von Zelewsky, Angew. Chem., Int. Ed., 1999, 38, 302–322 CrossRef;
(b) H. Brunner, Angew. Chem., Int. Ed., 1999, 38, 1194–1208 CrossRef CAS;
(c) P. D. Knight and P. Scott, Coord. Chem. Rev., 2003, 242, 125–143 CrossRef CAS;
(d) M. Fontecave, O. Hamelin and S. Ménage, Top. Organomet. Chem., 2005, 15, 271–288 CrossRef CAS;
(e)
H. Amouri and M. Gruselle, Chirality in Transition Metal Chemistry, Wiley, Chichester, UK, 2008 Search PubMed;
(f) J. Crassous, Chem. Soc. Rev., 2009, 38, 830–845 RSC;
(g) E. Meggers, Eur. J. Inorg. Chem., 2011, 2911–2926 CrossRef CAS;
(h) J. Crassous, Chem. Commun., 2012, 48, 9684–9692 RSC;
(i) E. C. Constable, Chem. Soc. Rev., 2013, 42, 1637–1651 RSC.
- For recent reviews on natural products containing the pyrrolic ring, see:
(a) I. S. Young, P. D. Thornton and A. Thompson, Nat. Prod. Rep., 2010, 27, 1801–1839 RSC;
(b) X. Wang, Z. Ma, X. Wang, S. De, Y. Ma and C. Chen, Chem. Commun., 2014, 50, 8628–8639 RSC;
(c) V. Bhardwaj, D. Gumber, V. Abbot, S. Dhiman and P. Sharma, RSC Adv., 2015, 5, 15233–15266 RSC.
Footnote |
† Electronic supplementary information (ESI) available: Experimental procedures, characterization data of all new compounds, as well as HPLC traces of partial compounds. CCDC 1407324. For ESI and crystallographic data in CIF or other electronic format see DOI: 10.1039/c6qo00273k |
|
This journal is © the Partner Organisations 2016 |