DOI:
10.1039/C6QO00109B
(Research Article)
Org. Chem. Front., 2016,
3, 875-879
Metal-free borylation of electron-rich aryl (pseudo)halides under continuous-flow photolytic conditions†
Received
22nd March 2016
, Accepted 12th May 2016
First published on 13th May 2016
Abstract
A metal-free borylation reaction of electron-rich aryl chlorides, fluorides, mesylates and phosphates under continuous-flow photolytic conditions is reported. The flow setup was designed to facilitate this process efficiently in comparison with the batch mode. Owing to its unique chemical selectivity, mild reaction conditions, good functional group tolerance and substrate scope, this reaction adds a complementary protocol to the current synthetic methods for boronic acid derivatives. The proposed reaction mechanism involves a photolytically generated triplet aryl cation, and DFT calculations suggest that the borylation product is formed in an anion-mediated single step process passing a minimum energy crossing point.
Arylboronic acid derivatives are essential synthetic building blocks and are widely used in the syntheses of pharmaceuticals, materials, and other functional molecules, due to their versatile transformations, broad availability, air and/or moisture stability, and ease of handling.1 Conventionally, arylboronic acid derivatives can be prepared by treatment of arylmagnesium or lithium reagents with trialkyl borates, followed by transesterification or hydrolysis. However, these reactions suffer from limited functional group tolerance. Over the past two decades, transition metal-catalyzed reactions have been developed for the conversion of aryl iodides, bromides, and triflates to the corresponding boronates.2,3 Nevertheless, only a few catalyst systems are effective for the borylation of the less reactive aryl chlorides and mesylates, and no general methods are available for aryl fluorides. Recently, direct aromatic C–H borylation methods have been developed using precious transition metal catalysts.4 These catalytic approaches possess tremendous versatility and functional group compatibility, and thus have been widely used in the syntheses of arylboronic acid derivatives. To lower the costs and reduce heavy metal residues in the products, however, transition metal-free methods should be more desirable. In this regard, borylation reactions from arylamine derivatives have been developed.5 Alternatively, aryl iodides or bromides could be effectively borylated with alkali metal alkoxides as promoters.6,7 Mild borylation of diaryliodonium salts has also been reported.8 Additionally, useful methods for direct transition metal-free C–H borylation of electron-rich arenes and heteroarenes have been developed.9,10
Despite these advancements, new methods for syntheses of functional aryl boronates in a chemoselective and environmentally friendly fashion from broadly available starting materials under mild reaction conditions are still attractive. Moreover, new reactions that are complementary to the current methods in terms of substrate scope and reaction conditions are highly desirable. Very recently, we discovered an efficient borylation reaction of aryl iodides and bromides under photochemical conditions (Scheme 1a).11
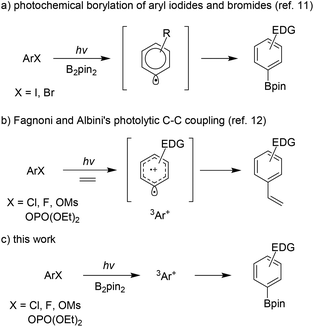 |
| Scheme 1 Previous photochemical borylation and the outline of this work. | |
Preliminary mechanistic studies indicated an aryl radical intermediate that reacted with activated diboron species to generate the borylation product and a boron-centered radical.11b,c Under the same conditions, however, aryl chlorides and fluorides were less reactive. In a set of related photochemical C–C coupling reactions, Fagnoni, Albini and their co-workers have demonstrated that electron-rich aryl chlorides and fluorides were particularly more effective than the corresponding bromides and iodides, and the key intermediate was a photochemically generated triplet aryl cation (Scheme 1b).12 Herein, we describe our recently obtained results in the development and computational mechanistic studies of a photochemical C–B bond-forming reaction of electron-rich aryl chlorides, fluorides and phenol derivatives (Scheme 1c).
4-Chlorophenol (1a) was used as a model substrate for initial investigations (Table 1). A solution of 1a and bis(pinacolato)diboron (B2pin2, 2) (1
:
1 molar ratio) was placed in a quartz test tube and irradiated with UV light. Encouragingly, the desired borylation product could be observed when the reactions were conducted in polar solvents such as acetonitrile, methanol and trifluoroethanol for 10 hours under irradiation with a 300 W high pressure mercury lamp (Table 1, entries 1–3). Using water and acetone as co-solvents slightly increased the yields (entries 4 and 5). Addition of bases led to remarkable effects (entries 6–8) and among them, tetramethylethylenediamine (TMEDA) gave a somewhat higher yield (28%, entry 6). Using two equivalents of B2pin2 could further improve the yield to 37% (entry 9) and reducing the amount of TMEDA to 0.5 equivalents resulted in comparable yield (entry 10).
Table 1 Reaction optimization under batch and continuous-flow conditions
Based on the batch conditions and our previous experience in continuous-flow reactions,11,13 we designed and assembled a continuous-flow photochemical reactor and further optimized this borylation reaction.14 Thus, transparent fluorinated ethylene propylene (FEP) tubing was coiled outside of a cooled quartz immersion well in which the mercury lamp was situated (see the ESI†). A stock solution containing all reactants and reagents was introduced into the tubing using a syringe pump. We were delighted to find that when the reaction was conducted in a continuous-flow fashion, the yield was significantly improved (40% to 77%, entry 11 vs. entry 10) with a residence time of only 26 minutes. The yield further increased to 87% when 0.1 equivalents of tetrabutylammonium fluoride (TBAF) were used as an additive (entry 12). A shorter reaction time and a smaller amount of B2pin2 both led to diminished yields (entries 13 and 14).
With the optimized reaction conditions in hand, the substrate scope of this photolytic borylation reaction was examined (Table 2). A series of electron-rich aryl halides or phenol derivatives were subjected to the reaction conditions. All continuous-flow reactions were run with less than 60 minutes of residence time. Aryl chlorides with para- or ortho-electron-donating substituents including both protic and non-protic O-, N-, and S-based groups were all viable substrates in these reactions to produce the corresponding boronates in moderate to good yields. Substituents at the ortho- (for 3b–3d, 3f, 3m and 3n) and/or meta- (for 3g and 3w) positions to the leaving group were also compatible. Aryl boronates containing saturated (3j–3l) and aromatic N-heterocycles (3p and 3q) that are interesting for drug synthesis could be prepared by this reaction. In addition, a few representative aryl fluorides (for 3a, 3e, 3v), phosphates (for 3t–3w) and mesylates (for 3t–3w) could be used as substrates in the borylation reaction. Aryl triflates (3w) were ineffective under the same conditions due to hydrolytic decomposition. Furthermore, the neopentanediolato boronates (3r and 3s) were similarly prepared when bis(neopentanediolato)diboron (B2neop2) was used in place of B2pin2. Remarkably, these electron-rich aryl chlorides, fluorides and mesylates are typically challenging substrates in transition-metal catalyzed borylation reactions. The present work, therefore, offers a complementary method for aryl boronate preparation. To demonstrate the stability and usefulness of this process in an automated system, the borylation of p-chlorophenol (1a) was carried out on a gram scale employing a commercial flow chemistry system (see the ESI†). With no further optimization, the reaction produced the desired boronate 3a in 87% isolated yield. Finally, attempts of this photolytic borylation reaction with electron-neutral aryl chlorides such as p-chlorotoluene and electron-poor substrates such as methyl 2-chlorobenzoate under the same conditions were not successful and only small amounts of hydrodechlorination products were observed.
Table 2 Substrate scope of the continuous-flow photolytic borylation reactiona
Reaction conditions: 1 (c = 0.05 M), 2 or 4 (2.0 equiv.), TMEDA (0.5 equiv.), TBAF (0.1 equiv.), acetone/H2O/CH3CN.
2 (3.0 equiv.), and TBAF (1.0 equiv.). TMEDA: tetramethylethylenediamine, TBAF: tetrabutylammonium fluoride.
|
|
Based on our experimental results, we conducted computational mechanistic studies to understand this new borylation reaction, especially the roles of σ-nucleophiles as previously proposed. The results are summarized in Fig. 1. p-Chloroanisole was used as the model substrate. In analogy to Fagnoni and Albini's work,12c we proposed that this reaction begins with a sensitizer-mediated excitation of the substrate to its triplet state, followed by a heterolytic C–Cl bond cleavage to generate the triplet aryl cation (3Ar+) and a chloride anion. A DFT study pointed out that the 3Ar+ lies 1.4 kcal mol−1 lower than the 1Ar+. To form the singlet borylation product from the triplet intermediate 3Ar+, a state crossing point must be involved. We therefore employed a code developed by Harvey and co-workers to optimize the geometry of minimum energy crossing points (MECPs) between potential energy surfaces of different spin states (Fig. 1).15 As shown in Fig. 1, a three-component interaction of 3Ar+, chloride anions and B2pin2 is taking place until the MECP is achieved. After the MECP, the reaction can be completed directly through TSsinglet (ΔEa = 11.0 kcal mol−1) to form the final product ArBpin.
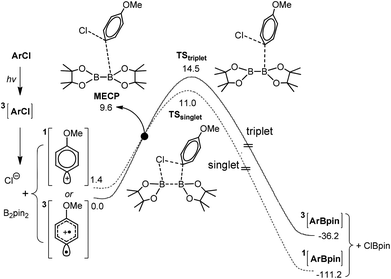 |
| Fig. 1 Proposed mechanism for the photolytic borylation and energy profiles of the product formation step via a singlet and/or triplet aryl cation. All molecular geometries were optimized without constraints via DFT calculations using the UB3LYP functional with the Lanl2DZ basis set for Cl, 6-311G* for B and those C atoms involved in bond breaking/forming processes, and 6-31G for all other atoms (see the ESI† for the computational details). The relative solvation- and entropy-corrected free energies (298 K) are given in kcal mol−1. | |
In conclusion, we have described a metal-free, photochemical C–B bond formation reaction from readily available electron-rich aryl chlorides, fluorides, mesylates and phosphates under continuous-flow conditions. The current method is complementary to the conventional transition metal-based approaches in terms of substrate scope and reactions conditions. Based on both the experimental and computational results, a reaction mechanism was proposed, which involves a triplet aryl cation intermediate and an anion-mediated facile one-step formation of the borylation product.
Acknowledgements
This work was financially supported by the Department of Science and Technology of Shaanxi Province (no. 2015KJXX-02), the National Science Foundation of China (no. 21472146) and the Research Grants Council of Hong Kong (HKUST 603313). We thank Prof. Wenxiu Que's group (XJTU) and Vapourtec Ltd for generously sharing with us the batch and flow photochemistry equipment.
Notes and references
-
(a)
D. G. Hall, in Boronic Acids: Preparation and Applications in Organic Synthesis, Medicine and Materials, ed. D. G. Hall, Wiley-VCH, Weinheim, 2011, pp. 1–134 Search PubMed;
(b) A. J. J. Lennox and G. C. Lloyd-Jones, Chem. Soc. Rev., 2014, 43, 412 RSC;
(c) L. Xu, S. Zhang and P. Li, Chem. Soc. Rev., 2015, 44, 8848 RSC;
(d) J. W. B. Fyfe and A. J. B. Watson, Synlett, 2015, 1139 CAS;
(e) S. Ding, L. Xu and P. Li, ACS Catal., 2016, 6, 1329 CrossRef CAS and references therein.
- For selected examples, see:
(a) T. Ishiyama, M. Murata and N. Miyaura, J. Org. Chem., 1995, 60, 7508 CrossRef CAS;
(b) A. Fürstner and G. Seidel, Org. Lett., 2002, 4, 541 CrossRef;
(c) C. M. So, C. P. Lau and F. Y. Kwong, Angew. Chem., Int. Ed., 2008, 47, 8059 CrossRef CAS PubMed;
(d) G. A. Molander, S. L. J. Trice and S. D. Dreher, J. Am. Chem. Soc., 2010, 132, 17701 CrossRef CAS PubMed;
(e) S. Kawamorita, H. Ohmiya, T. Iwai and M. Sawamura, Angew. Chem., Int. Ed., 2011, 50, 8363 CrossRef CAS PubMed;
(f) K. Huang, D.-G. Yu, S.-F. Zheng, Z.-H. Wu and Z.-J. Shi, Chem. – Eur. J., 2011, 17, 786 CrossRef CAS PubMed;
(g) T. Yamamoto, T. Morita, J. Takagi and T. Yamakawa, Org. Lett., 2011, 13, 5766 CrossRef CAS PubMed;
(h) W. Zhu and D. Ma, Org. Lett., 2006, 8, 261 CrossRef CAS PubMed;
(i) C. Kleeberg, L. Dang, Z. Lin and T. B. Marder, Angew. Chem., Int. Ed., 2009, 48, 5350 CrossRef CAS PubMed;
(j) F. Labre, Y. Gimbert, P. Bannwarth, S. Olivero, E. Duñach and P. Y. Chavant, Org. Lett., 2014, 16, 2366 CrossRef CAS PubMed;
(k) Y. Nagashima, R. Takita, K. Yoshida, K. Hirano and M. Uchiyama, J. Am. Chem. Soc., 2013, 135, 18730 CrossRef CAS PubMed;
(l) L. Xu and P. Li, Chem. Commun., 2015, 51, 5656 RSC;
(m) J. Hu, H. Sun, W. Cai, X. Pu, Y. Zhang and Z. J. Shi, J. Org. Chem., 2016, 81, 14 CrossRef CAS PubMed;
(n) G. A. Molander, S. L. J. Trice, S. M. Kennedy, S. D. Dreher and M. T. Tudge, J. Am. Chem. Soc., 2012, 134, 11667 CrossRef CAS PubMed;
(o) X. W. Liu, J. Echavarren, C. Zarate and R. Martin, J. Am. Chem. Soc., 2015, 137, 12470 CrossRef CAS PubMed.
-
(a) K. L. Billingsley, T. E. Barder and S. L. Buchwald, Angew. Chem., Int. Ed., 2007, 46, 5359 CrossRef CAS PubMed;
(b) D. A. Wilson, C. J. Wilson, C. Moldoveanu, A.-M. Resmerita, P. L. Corcoran, M. Hoang, B. M. Rosen and V. Percec, J. Am. Chem. Soc., 2010, 132, 1800 CrossRef CAS PubMed;
(c) G. A. Molander, L. N. Cavalcanti and C. García-García, J. Org. Chem., 2013, 78, 6427 CrossRef CAS PubMed.
- For selected references of catalytic sp2 C–H borylation, see:
(a) I. A. I. Mkhalid, J. H. Barnard, T. B. Marder, J. M. Murphy and J. F. Hart-wig, Chem. Rev., 2010, 110, 890 CrossRef CAS PubMed;
(b) J. F. Hartwig, Acc. Chem. Res., 2012, 45, 864 CrossRef CAS PubMed;
(c) A. Ros, R. Fernandez and J. M. Lassaletta, Chem. Soc. Rev., 2014, 43, 3229 RSC;
(d) C. N. Iverson and M. R. Smith, J. Am. Chem. Soc., 1999, 121, 7696 CrossRef CAS;
(e) H. Chen, S. Schlecht, T. C. Semple and J. F. Hartwig, Science, 2000, 287, 1995 CrossRef CAS PubMed;
(f) T. Ishiyama, J. Takagi, K. Ishida, N. Miyaura, N. R. Anastasi and J. F. Hartwig, J. Am. Chem. Soc., 2002, 124, 390 CrossRef CAS PubMed;
(g) S. Kawamorita, H. Ohmiya, K. Hara, A. Fukuoka and M. Sawamura, J. Am. Chem. Soc., 2009, 131, 5058 CrossRef CAS PubMed;
(h) I. A. I. Mkhalid, D. N. Coventry, D. A. Jove, A. S. Batsanov, J. A. K. Howard, R. N. Perutz and T. B. Marder, Angew. Chem., Int. Ed., 2006, 45, 489 CrossRef CAS PubMed;
(i) H. X. Dai and J. Q. Yu, J. Am. Chem. Soc., 2012, 134, 134 CrossRef CAS PubMed;
(j) L. Xu, S. Ding and P. Li, Angew. Chem., Int. Ed., 2014, 53, 1822 CrossRef CAS PubMed;
(k) A. J. Roering, V. A. L. Hale, P. A. Squier, M. A. Ringgold, E. R. Wiederspan and T. B. Clark, Org. Lett., 2012, 14, 3558 CrossRef CAS PubMed;
(l) T. J. Mazzacano and N. P. Mankad, J. Am. Chem. Soc., 2013, 135, 17258 CrossRef CAS PubMed;
(m) J. V. Obligacion, S. P. Semproni and P. J. Chirik, J. Am. Chem. Soc., 2014, 136, 4133 CrossRef CAS PubMed;
(n) L.-S. Zhang, G. Chen, X. Wang, Q.-Y. Guo, X.-S. Zhang, F. Pan, K. Chen and Z.-J. Shi, Angew. Chem., Int. Ed., 2014, 53, 3899 CrossRef CAS PubMed;
(o) G. Wang, L. Xu and P. Li, J. Am. Chem. Soc., 2015, 137, 8058 CrossRef CAS PubMed;
(p) T. Dombray, C. G. Werncke, S. Jiang, M. Grellier, L. Vendier, S. Bontemps, J.-B. Sortais, S. Sabo-Etienne and C. Darcel, J. Am. Chem. Soc., 2015, 137, 4062 CrossRef CAS PubMed.
-
(a) F. Mo, Y. Jiang, D. Qiu, Y. Zhang and J. Wang, Angew. Chem., Int. Ed., 2010, 49, 1846 CrossRef CAS PubMed;
(b) D. Qiu, L. Jin, Z. Zheng, H. Meng, F. Mo, X. Wang, Y. Zhang and J. Wang, J. Org. Chem., 2013, 78, 1923 CrossRef CAS PubMed;
(c) W. Erb, A. Hellal, M. Albini, J. Rouden and J. Blanchet, Chem. – Eur. J., 2014, 20, 6608 CrossRef CAS PubMed;
(d) J. Yu, L. Zhang and G. Yan, Adv. Synth. Catal., 2012, 354, 2625 CrossRef CAS;
(e) R. D. Dewhurst, E. C. Neeve, H. Braunschweig and T. B. Marder, Chem. Commun., 2015, 51, 9594 RSC;
(f) S. Pietch, E. C. Neeve, D. C. Apperley, R. Bertermann, F. Mo, D. Qiu, M. S. Cheung, L. Dang, J. Wang, U. Radius, Z. Lin, C. Kleeberg and T. B. Marder, Chem. – Eur. J., 2015, 21, 7082 CrossRef PubMed;
(g) C. Zhu and M. Yamane, Org. Lett., 2012, 14, 4560 CrossRef CAS PubMed.
-
(a) E. Yamamoto, K. Izumi, Y. Horita and H. Ito, J. Am. Chem. Soc., 2012, 134, 19997 CrossRef CAS PubMed;
(b) R. Uematsu, E. Yamamoto, S. Maeda, H. Ito and T. Taketsugu, J. Am. Chem. Soc., 2015, 137, 4090 CrossRef CAS PubMed;
(c) E. Yama-moto, S. Ukigai and H. Ito, Chem. Sci., 2015, 6, 2943 RSC.
- J. Zhang, H.-H. Wu and J. Zhang, Eur. J. Org. Chem., 2013, 6263 CrossRef CAS.
- N. Miralles, R. M. Romero, E. Fernándes and K. Muñiz, Chem. Commun., 2015, 51, 14068 RSC.
-
(a) A. Prokofjevs, J. W. Kamf and E. Vedejs, Angew. Chem., Int. Ed., 2011, 50, 2098 CrossRef CAS PubMed;
(b) L. Niu, H. Yang, R. Wang and H. Fu, Org. Lett., 2012, 14, 2618 CrossRef CAS PubMed;
(c) V. Bagutski, A. D. Grosso, J. A. Carrillo, I. A. Cade, M. D. Helm, J. R. Lawson, P. J. Singleton, S. A. Solomon, T. Marcelli and M. J. Ingleson, J. Am. Chem. Soc., 2013, 135, 474 CrossRef CAS PubMed.
-
(a) M. A. Légaré, M. A. Courtemanche, Ė. Rochette and F.-G. Fontaine, Science, 2015, 349, 513 CrossRef PubMed;
(b) S. K. Bose and T. B. Marder, Science, 2015, 349, 473 CrossRef CAS PubMed.
-
(a) K. Chen, S. Zhang, P. He and P. Li, Chem. Sci., 2016 10.1039/C5SC04521E;
(b) D. Lu, C. Wu and P. Li, Org. Lett., 2014, 16, 1486 CrossRef CAS PubMed;
(c) D. Lu, C. Wu and P. Li, Chem. – Eur. J., 2014, 20, 1630 CrossRef CAS PubMed;
(d) K. Chen, P. He, S. Zhang and P. Li, Chem. Commun., 2016 10.1039/C6CC01135G;
(e) During the preparation of this manuscript, Larionov and coworkers have independently published photolytic borylation of aryl halides, A. M. Mfuh, J. D. Doyle, B. Chhetri, H. D. Arman and O. V. Larionov, J. Am. Chem. Soc., 2016, 138, 2985 CrossRef CAS PubMed.
-
(a) M. Fagnoni and A. Albini, Acc. Chem. Res., 2005, 38, 713 CrossRef CAS PubMed;
(b) M. Freccero, M. Fagnoni and A. Albini, J. Am. Chem. Soc., 2003, 125, 13182 CrossRef CAS PubMed;
(c) M. D. Carolis, S. Protti, M. Fagnoni and A. Albini, Angew. Chem., Int. Ed., 2005, 44, 1232 CrossRef PubMed;
(d) S. Protti, M. Fagnoni and A. Albini, Angew. Chem., Int. Ed., 2005, 44, 5675 CrossRef CAS PubMed;
(e) V. Dichiarante, M. Fagnoni, M. Mella and A. Albini, Chem. Eur. J., 2006, 12, 3905 CrossRef CAS PubMed;
(f) V. Dichiarante, M. Fagnoni and A. Albini, Angew. Chem., Int. Ed., 2007, 46, 6495 CrossRef CAS PubMed;
(g) V. Dichiarante, D. Dondi, S. Protti, M. Fagnoni and A. Albini, J. Am. Chem. Soc., 2007, 129, 5605 CrossRef CAS PubMed;
(h) S. Lazzaroni, S. Protti, M. Fagnoni and A. Albini, Org. Lett., 2009, 11, 349 CrossRef CAS PubMed;
(i) V. Dichiarante, M. Fagnoni and A. Albini, Green. Chem., 2009, 11, 924 RSC;
(j) S. Protti, M. Fagnoni and A. Albini, J. Org. Chem., 2012, 77, 6473 CrossRef CAS PubMed;
(k) H. Qrareya, C. Raviola, S. Protti, M. Fagnoni and A. Albini, J. Org. Chem., 2013, 78, 6016 CrossRef CAS PubMed.
-
(a) P. Li, J. S. Moore and K. F. Jensen, ChemCatChem, 2013, 5, 1729 CrossRef CAS;
(b) P. Li and S. L. Buchwald, Angew. Chem., Int. Ed., 2011, 50, 6396 CrossRef CAS PubMed.
- For early pioneering work on flow photochemistry, see:
(a) H. Lu, M. A. Schmidt and K. F. Jensen, Lab Chip, 2001, 1, 22 RSC;
(b) K. Ueno, F. Kitagawa and N. Kitamura, Lab Chip, 2002, 2, 231 RSC. For recent reviews on flow photochemistry, see:
(c) J. P. Knowles, L. D. Elliott, K. I. Booker-Milburn and J. Beilstein, Org. Chem., 2012, 8, 2025 CAS;
(d) D. Cambié, C. Bottecchia, N. J. W. Straathof, V. Hessel and T. Noël, Chem. Rev., 2016, 116 DOI:10.1021/acs.chemrev.5b00707. For selected references of continuous-flow photochemical reaction, see:
(e) B. D. A. Hook, W. Dohle, P. R. Hirst, M. Pickworth, M. B. Berry and K. I. Booker-Milburn, J. Org. Chem., 2005, 70, 7558 CrossRef CAS PubMed;
(f) Y. S. M. Vaske, M. E. Mahoney, J. P. Konopelski, D. L. Rogow and W. J. McDonald, J. Am. Chem. Soc., 2010, 132, 11379 CrossRef CAS PubMed;
(g) F. Lévesque and P. H. Seeberger, Angew. Chem., Int. Ed., 2012, 51, 1706 CrossRef PubMed;
(h) D. C. Harrowven, M. Mohamed, T. P. Gonçalves, R. J. Whitby, D. Bolien and H. F. Sneddon, Angew. Chem., Int. Ed., 2012, 51, 4405 CrossRef CAS PubMed;
(i) R. S. Andrews, J. J. Becker and M. R. Gagne, Angew. Chem., Int. Ed., 2012, 51, 4140 CrossRef CAS PubMed;
(j) J. W. Tucker, Y. Zhang, T. F. Jamison and C. R. J. Stephenson, Angew. Chem., Int. Ed., 2012, 51, 4144 CrossRef CAS PubMed;
(k) K. G. Maskill, J. P. Knowles, L. D. Elliott, R. W. Alder and K. I. B. Milburn, Angew. Chem., Int. Ed., 2013, 52, 1499 CrossRef CAS PubMed;
(l) Y. Zhang, M. L. Blackman, A. B. Leduc and T. F. Jamison, Angew. Chem., Int. Ed., 2013, 52, 4251 CrossRef CAS PubMed;
(m) X. Wang, G. D. Cuny and T. Noël, Angew. Chem., Int. Ed., 2013, 52, 7860 CrossRef CAS PubMed.
-
(a) J. N. Harvey, M. Aschi, H. Schwarz and W. Koch, Theor. Chem. Acc., 1998, 99, 95 CrossRef CAS;
(b) J. N. Harvey and M. Aschi, Phys. Chem. Chem. Phys., 1999, 1, 5555 RSC.
Footnote |
† Electronic supplementary information (ESI) available: Materials including experimental procedures, and copies of 1H and 13C NMR spectra of all new products. See DOI: 10.1039/c6qo00109b |
|
This journal is © the Partner Organisations 2016 |