DOI:
10.1039/C6QO00133E
(Research Article)
Org. Chem. Front., 2016,
3, 870-874
Copper-catalyzed tandem arylation–cyclization of 2-alkynylaryl isothiocyanates with diaryliodonium salts: an efficient synthesis of thiochromeno[2,3-b]indoles†
Received
31st March 2016
, Accepted 12th May 2016
First published on 18th May 2016
Abstract
A catalytic tandem arylation–cyclization approach using 2-alkynylphenyl isothiocyanates with diaryliodonium salts is described. The reaction is performed under mild conditions and thiochromeno[2,3-b]indoles are obtained in moderate to good yields. This tandem protocol involves chemoselective S-arylation, regioselective 5-endo-trig cyclization and Friedel–Crafts-type cyclization processes. Two C–C bonds, one C–S bond, and two heterocyclic rings are formed in a single step. Preliminary mechanistic studies indicate that a carbocation mechanism is involved.
Introduction
It is found that 2,3-fused polycyclic indole derivatives widely exist in bioactive natural products,1 as exemplified by aspidospermine,2 yohimbine,3 and strychnine.4 Over the past few decades, indoles containing heteroacenes have also received much attention because of their potential application in organic field-effect transistors (OFETs) and organic light emitting diodes (OLEDs).5 In addition, fused-thiopyran scaffolds have been found to exhibit a broad spectrum of bioactivities, such as anti-cancer,6 anti-bacterial,7 and anti-hyperplasia activities.8 Certain thiopyrano[2,3-b]indoles have also shown to possess analgesic activity.9 Despite the development of various methodologies for the preparation of 2,3-fused indoles,10 including thiopyrano[2,3-b]indoles,11 sometimes, they suffer from tedious multistep routes and high cost transition metals. Therefore, it would be desirable to develop a more concise method.
Due to its high reactivity and low toxicity, the diaryliodonium salt has emerged as an efficient arylating reagent in organic synthesis.12 In the last three years, significant progress has been made in the tandem arylation–cyclization reactions to construct various heterocyclic compounds initiated by diaryliodonium salts.13 Nitriles,14 alkenes,15 and alkynes16 were frequently employed as substrates in these transformations. However, cyclization based on isothiocyanates is still underdeveloped.
Recently, the construction of fused heterocycles through bicyclization17 has attracted significant attention due to their high efficiency, especially when using 2-alkynylaryl isothiocyanates18 as starting materials. In 2003, Nanni and coworkers19 reported a radical cyclization of 2-alkynylphenyl isothiocyanates with aryl radicals, generated from diazonium tetrafluoroborates, to synthesize thiochromeno[2,3-b]indoles via imidoyl radicals (Scheme 1a). Only 7 examples were obtained as mixtures of isomers through competitive [4 + 2] and [4 + 1] radical cyclizations. We envisioned that 2-alkynylphenyl isothiocyanates could be combined with an aryl carbocation generated from diaryliodonium salts, in which a single isomer of thiochromeno[2,3-b]indoles might be generated through [4 + 2] cyclization exclusively. However, control of the chemo- and regioselectivity is challenging, because alkynes are known to react with diaryliodonium salts.20 In continuation of our previous study,21 we report herein a Cu-catalyzed chemo- and regioselective domino arylation–cyclization approach for the synthesis of thiochromeno[2,3-b]indoles as a single isomer from 2-alkynylaryl isothiocyanates and diaryliodonium salts (Scheme 1b).
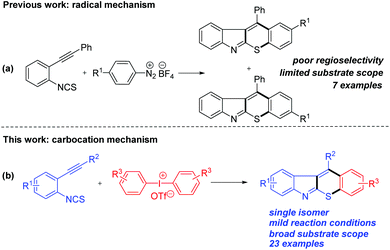 |
| Scheme 1 Synthesis of thiochromeno[2,3-b]indoles. | |
Results and discussion
Initially, we examined several different copper catalysts using 2-(2-phenylethynyl)phenyl isothiocyanate 1a with diphenyliodonium triflate 2a (Table 1, entries 1–4), and found that Cu(OTf)2 gave the best result. The 11-phenylthiochromeno[2,3-b]indole 3a was obtained in 45% yield. In the absence of the Cu catalyst, no desired product was observed (entry 5). Moreover, lower yields were obtained when the anions of the diphenyliodonium salts were changed to hexafluorophosphate (PF6−) or tetrafluoroborate (BF4−) (entries 6 and 7). Diminished yields were obtained at decreased and elevated temperatures (entries 8 and 9). In order to further improve the yield, a base was added to quench trifluoromethanesulfonic acid formed during the reaction (entries 10–13). Pleasingly, the yield of 3a was up to 64% when 1 equiv. of K2CO3 was added (entry 10). The yield of 3a was not improved when the catalyst loading was increased to 20 mol% or reduced to 5 mol% (entries 14 and 15). After optimization, the best conditions were established as follows: Cu(OTf)2 (0.1 equiv.) as the catalyst and K2CO3 (1.0 equiv.) as the base in DCE (1.0 mL) at 50 °C under N2 for 6 h. The structure of 3a was confirmed by X-ray diffraction analysis (Fig. S1 in the ESI†).
Table 1 Optimization of the reaction conditionsa

|
Entry |
Catalyst (mol%) |
X |
Base (eq.) |
T [°C] |
Yieldb (%) |
Reaction conditions: 1a (0.2 mmol), 2a (1.5 equiv.), DCE (1.0 mL), 6 h, N2.
Isolated yield.
|
1 |
CuCl (10) |
OTf |
|
50 |
20 |
2 |
CuBr (10) |
OTf |
|
50 |
32 |
3 |
Cu(OTf)2 (10) |
OTf |
|
50 |
45 |
4 |
CuTc (10) |
OTf |
|
50 |
0 |
5 |
— |
OTf |
|
50 |
0 |
6 |
Cu(OTf)2 (10) |
PF6 |
|
50 |
25 |
7 |
Cu(OTf)2 (10) |
BF4 |
|
50 |
34 |
8 |
Cu(OTf)2 (10) |
OTf |
|
30 |
26 |
9 |
Cu(OTf)2 (10) |
OTf |
|
80 |
31 |
10
|
Cu(OTf)
2
(10)
|
OTf
|
K
2
CO
3
(1.0)
|
50
|
64
|
11 |
Cu(OTf)2 (10) |
OTf |
Na2CO3 (1.0) |
50 |
37 |
12 |
Cu(OTf)2 (10) |
OTf |
Cs2CO3 (1.0) |
50 |
42 |
13 |
Cu(OTf)2 (10) |
OTf |
DABCO (1.0) |
50 |
0 |
14 |
Cu(OTf)2 (20) |
OTf |
K2CO3 (1.0) |
50 |
37 |
15 |
Cu(OTf)2 (5) |
OTf |
K2CO3 (1.0) |
50 |
28 |
With the optimal conditions in hand, a variety of alkynylaryl isothiocyanates were tested (Table 2). The R2 group in substrates 1 exhibited obvious electronic effects. Products 3b, 3c and 3g were afforded in good yields (65–68%) when R2 was an electron-donating aryl group such as 4-MeC6H4, 4-MeOC6H4 and 3-MeC6H4. Whereas, when R2 was an electron-withdrawing aryl group, such as 4-FC6H4, 4-ClC6H4, and 4-BrC6H4, the reaction proceeded to generate compounds 3d–3f in moderate yields (42–57%). The substrate with a thiophene group was also tolerated and product 3h was isolated in 70% yield. Moreover, product 3i was obtained in 51% yield when R2 was a cyclopropyl group (a group can stabilize the adjacent carbocation). Unfortunately, no desired product 3j was formed when R2 was an n-butyl group, which is probably due to the adjacent positive charge could not be stabilized by this group. In addition, products 3k–3n were obtained in good yields (60–66%), regardless of the substitution positions and electronic properties of the R1 group.
Table 2 Synthesis of thiochromeno[2,3-b]indoles 3a–3n from various 2-alkynylaryl isothiocyanates 1a
Reaction conditions: 1a (0.5 mmol), 2a (1.5 equiv.), Cu(OTf)2 (0.1 equiv.), K2CO3 (1.0 equiv.), DCE (2.5 mL), 50 °C, 6 h, N2.
The reaction time is 15 min.
|
|
We next investigated the scope of diaryliodonium salts 2 under the optimal conditions (Table 3). Substrates bearing fluoro, chloro, bromo, ester, and trifluoromethyl groups at the para-position of the aryl ring all worked well to produce the desired thiochromeno[2,3-b]indoles 3o–3s in 46–65% yields. The expected products 3t–v were afforded smoothly regardless of the position of the methyl group on the phenyl ring. While the meta substituted substrate gave two inseparable isomers in 1.8
:
1 ratio and 67% overall yield (3v/3v′). Additionally, diaryliodonium salts with 2,4-dimethyl groups also afforded the desired products 3w, albeit in lower yield.
Table 3 Synthesis of thiochromeno[2,3-b]indoles 3o–3w from various diaryliodonium salts 2a
Reaction conditions: 1a (0.5 mmol), 2a (1.5 equiv.), Cu(OTf)2 (0.1 equiv.), K2CO3 (1.0 equiv.), DCE (2.5 mL), 50 °C, 6 h, N2.
|
|
Notably, when diaryliodonium salt with a strong electron-donating group (4-OMe) 2x was used to react with 1, the carbamothioates 4a–c were separated in 62–70% yields rather than the desired cyclization products (Scheme 2). Moreover, when bis(2-methoxyphenyl)iodonium salt was used to react with 1, no separable product was formed. The structures of 4 were confirmed by X-ray diffraction analysis of 4a (Fig. S2 in the ESI†). The reason that 2x cannot form the thiochromeno[2,3-b]indole remains unclear, however, the separation of carbamothioates 4 supports the formation of the proposed intermediate A (Scheme 4).
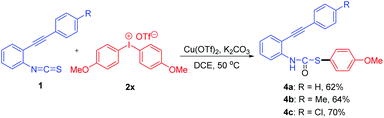 |
| Scheme 2 The reaction of 1 with 2x. | |
Control experiments were carried out to investigate the reaction mechanism. Initially, a radical scavenger 2,6-di-tert-butyl-4-methylphenol (BHT) was added to the reaction mixture under the optimal conditions. The reaction was not inhibited and 3a was obtained in 62% yield (Scheme 3a). In addition, a competition reaction was performed using 2-alkynylphenyl isothiocyanate 1a with unsymmetrical diaryliodonium salt 2b under the standard conditions, product 3t was afforded exclusively in 58% yield and no product 3w was detected (Scheme 3b). These results indicate that a carbocation mechanism might be involved in the reaction.14c,22
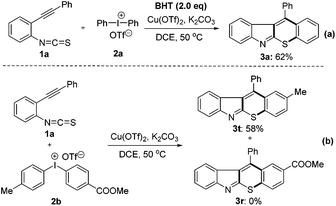 |
| Scheme 3 Control experiments. | |
On the basis of our experiment results, a possible mechanism for the copper-catalyzed domino reaction was proposed (Scheme 4). According to the literature and our previous work,16,21 both the isothiocyanate group and alkyne group in substrate 1a could be arylated by diaryliodonium salts. In this paper, a phenyl carbocation is chemoselectively transferred by a well-established Cu(III) species16 to the isothiocyanate group of 1a to generate a cationic intermediate A. Then, intermediate A is captured by the vicinal alkyne group to give intermediate B through a regioselective 5-endo-trig cyclization (path a). The newly formed carbocation in intermediate B could be stabilized by the adjacent phenyl group. Finally, the thiochromeno[2,3-b]indole is obtained by intramolecular Friedel–Crafts-type cyclization of intermediate B. Another reaction path b through 6-endo-trig cyclization to form quinolin-4(1H)-ones was not observed.
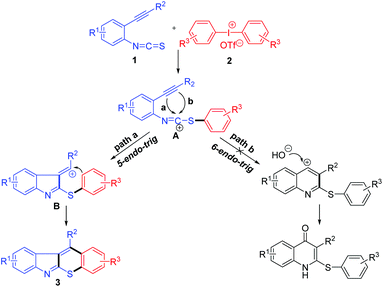 |
| Scheme 4 Proposed reaction mechanism. | |
Conclusions
In conclusion, an efficient copper-catalyzed tandem arylation–cyclization process is developed with readily available starting materials 2-alkynylaryl isothiocyanates and diaryliodonium salts. Tetracyclic thiochromeno[2,3-b]indoles are obtained in good yield through a sequence of chemoselective S-arylation, regioselective 5-endo-trig cyclization, and Friedel–Crafts-type cyclization processes. Moreover, this method features a broad substrate scope, simple operation, and the formation of three chemical bonds and two heterocyclic rings in a single step. Further studies to expand the scope of isothiocyanate-based cyclization with diaryliodonium salts are ongoing in our laboratory.
Acknowledgements
This work was financially supported by the National Natural Science Foundation of China (21372137 and 21572110) and the Natural Science Foundation of Shandong Province (ZR2014BM006).
Notes and references
- For selected reviews, see:
(a) M. Ishikura, T. Abe, T. Choshi and S. Hibino, Nat. Prod. Rep., 2013, 30, 69 RSC;
(b) M. Ishikura and K. Yamada, Nat. Prod. Rep., 2009, 26, 803 RSC;
(c) G. R. Humphrey and J. T. Kuethe, Chem. Rev., 2006, 106, 2875 CrossRef CAS PubMed;
(d) T. Kawasaki and J. A. Salas, Nat. Prod. Rep., 2006, 23, 1007 RSC;
(e) M. Somei and F. Yamada, Nat. Prod. Rep., 2005, 22, 73 RSC;
(f) D. A. Horton, G. T. Bourne and M. L. Smythe, Chem. Rev., 2003, 103, 893 CrossRef CAS PubMed.
-
(a)
J. E. Saxton, Alkaloids, Academic Press, 1998, vol. 51, p. 2 Search PubMed;
(b) J. P. Lajiness, W. Jiang and D. L. Boger, Org. Lett., 2012, 14, 2078 CrossRef CAS PubMed.
-
(a) M. R. Goldberg and D. Robertson, Pharmacol. Rev., 1983, 35, 143 CAS;
(b) D. J. Mergott, S. J. Zuend and E. N. Jacobsen, Org. Lett., 2008, 10, 745 CrossRef CAS PubMed.
- J. Bonjoch and D. Sole, Chem. Rev., 2000, 100, 3455 CrossRef CAS PubMed.
-
(a) N. Kamimoto, D. Schollmeyer, K. Mitsudo, S. Suga and S. R. Waldvogel, Chem. – Eur. J., 2015, 21, 8257 CrossRef CAS PubMed;
(b) L. Qiu, X. Zhuang, N. Zhao, X. Wang, Z. An, Z. Lan and X. Wan, Chem. Commun., 2014, 50, 3324 RSC;
(c) Y. Huang, D. Wu, J. Huang, Q. Guo, J. Li and J. You, Angew. Chem., Int. Ed., 2014, 53, 12158 CrossRef CAS PubMed;
(d) L. Qiu, X. Wang, N. Zhao, S. Xu, Z. An, X. Zhuang, Z. Lan, L. Wen and X. Wan, J. Org. Chem., 2014, 79, 11339 CrossRef CAS PubMed.
-
(a)
K. D. Berlin, D. M. Benbrook and E. C. Nelson, U.S. Patent, 6586460, 2003 Search PubMed;
(b) Y. Sugita, H. Hosoya, K. Terasawa, I. Yokoe, S. Fujisawa and H. Sakagami, Anticancer Res., 2001, 21, 2629 CAS.
- M. J. Brown, P. S. Carter, A. E. Fenwick, A. P. Fosberry, D. W. Hamprecht, M. J. Hibbs, R. L. Jarvest, L. Mensah, P. H. Milner, P. J. O'Hanlon, A. J. Pope, C. M. Richardson, A. West and D. R. Witty, Bioorg. Med. Chem. Lett., 2002, 12, 3171 CrossRef CAS PubMed.
- W. Quaglia, M. Pigini, A. Piergentili, M. Giannella, F. Gentili, G. Marucci, A. Carrieri, A. Carotti, E. Poggesi, A. Leonardi and C. Melchiorre, J. Med. Chem., 2002, 45, 1633 CrossRef CAS PubMed.
-
(a) S. Takada and Y. Makisumi, Chem. Pharm. Bull., 1984, 32, 872 CrossRef CAS;
(b) S. Takada, N. Ishizuka, T. Sasatani, Y. Makisumi, H. Jyoyama, H. Hatakeyama, F. Asanuma and K. Hirose, Chem. Pharm. Bull., 1984, 32, 877 CrossRef CAS PubMed.
- For selected reviews, see:
(a) M. Shiri, Chem. Rev., 2012, 112, 3508 CrossRef CAS PubMed;
(b) M. Platon, R. Amardeil, L. Djakovitchb and J. C. Hierso, Chem. Soc. Rev., 2012, 41, 3929 RSC;
(c) A. Gimeno, A. Rodríguez-Gimeno, A. B. Cuenca, C. R. de Arellano, M. Medio-Simón and G. Asensio, Chem. Commun., 2015, 51, 12384 RSC;
(d) T. M. Ha, B. Yao, Q. Wang and J. Zhu, Org. Lett., 2015, 17, 1750 CrossRef CAS PubMed;
(e) X. F. Xia, N. Wang, L. L. Zhang, X. R. Song, X. Y. Liu and Y. M. Liang, J. Org. Chem., 2012, 77, 9163 CrossRef CAS PubMed;
(f) S. Ali, Y. X. Li, S. Anwar, F. Yang, Z. S. Che and Y. M. Liang, J. Org. Chem., 2012, 77, 424 CrossRef CAS PubMed;
(g) T. Guo, Q. Jiang, F. Huang, J. Chen and Z. Yu, Org. Chem. Front., 2014, 1, 707 RSC;
(h) Y. S. Zhang, X. Y. Tang and M. Shi, Org. Chem. Front., 2015, 2, 1516 RSC.
-
(a) M. Jha, G. M. Shelke, T. S. Cameron and A. Kumar, J. Org. Chem., 2015, 80, 5272 CrossRef CAS PubMed;
(b) X. Chen, Z. H. Qi, S. Y. Zhang, L. P. Kong, Y. Wang and X. W. Wang, Org. Lett., 2015, 17, 42 CrossRef CAS PubMed;
(c) F. M. Moghaddama, M. R. Khodabakhshi, M. Kiamehr and Z. Ghahremannejad, Tetrahedron Lett., 2013, 54, 2685 CrossRef; S. Majumder and P. J. Bhuyan, Tetrahedron Lett., 2012, 53, 137 Search PubMed.
- For selected reviews, see:
(a) K. Aradi, B. L. Tóth, G. L. Tolnai and Z. Novák, Synlett, 2016, 27, DOI:10.1055/s-0035-1561369;
(b) E. A. Merritt and B. Olofsson, Angew. Chem., Int. Ed., 2009, 48, 9052 CrossRef CAS PubMed;
(c) V. V. Zhdankin and P. J. Stang, Chem. Rev., 2008, 108, 5299 CrossRef CAS PubMed;
(d) V. V. Zhdankin and P. J. Stang, Chem. Rev., 2002, 102, 2523 CrossRef CAS PubMed. For selected arylation reactions, see:
(e) S. G. Modha and M. F. Greaney, J. Am. Chem. Soc., 2015, 137, 1416 CrossRef CAS PubMed;
(f) M. G. Suero, E. D. Bayle, B. S. L. Collins and M. J. Gaunt, J. Am. Chem. Soc., 2013, 135, 5332 CrossRef CAS PubMed;
(g) B. S. L. Collins, M. G. Suero and M. J. Gaunt, Angew. Chem., Int. Ed., 2013, 52, 5799 CrossRef CAS PubMed;
(h) Q. Y. Toh, A. McNally, S. Vera, N. Erdmann and M. J. Gaunt, J. Am. Chem. Soc., 2013, 135, 3772 CrossRef CAS PubMed.
-
(a) S. Zhu and D. W. C. MacMillan, J. Am. Chem. Soc., 2012, 134, 10815 CrossRef CAS PubMed;
(b) H. Jiang, Y. Cheng, R. Wang, Y. Zhang and S. Yu, Chem. Commun., 2014, 50, 6164 RSC;
(c) Y. Wang, C. Chen, S. Zhang, Z. Lou, X. Su, L. Wen and M. Li, Org. Lett., 2013, 15, 4794 CrossRef CAS PubMed.
-
(a) K. Aradi and Z. Novák, Adv. Synth. Catal., 2015, 357, 371 CrossRef CAS;
(b) X. Pang, C. Chen, X. Su, M. Li and L. Wen, Org. Lett., 2014, 16, 6228 CrossRef CAS PubMed;
(c) Y. Wang, C. Chen, J. Peng and M. Li, Angew. Chem., Int. Ed., 2013, 52, 5323 CrossRef CAS PubMed;
(d) X. Su, C. Chen, Y. Wang, J. Chen, Z. Lou and M. Li, Chem. Commun., 2013, 49, 6752 RSC.
-
(a) E. Cahard, H. P. J. Male, M. Tissot and M. J. Gaunt, J. Am. Chem. Soc., 2015, 137, 7986 CrossRef CAS PubMed;
(b) D. Holt and M. J. Gaunt, Angew. Chem., Int. Ed., 2015, 54, 7857 CrossRef CAS PubMed;
(c) B. Zhou, W. Hou, Y. Yang, H. Feng and Y. Li, Org. Lett., 2014, 16, 1322 CrossRef CAS PubMed;
(d) E. Cahard, N. Bremeyer and M. J. Gaunt, Angew. Chem., Int. Ed., 2013, 52, 9284 CrossRef CAS PubMed.
-
(a) Á. Sinai, D. Vangel, T. Gáti, P. Bombicz and Z. Novák, Org. Lett., 2015, 17, 4136 CrossRef PubMed;
(b) J. Chen, C. Chen, J. Chen, G. Wang and H. Qu, Chem. Commun., 2015, 51, 1356 RSC;
(c) F. Zhang, S. Das, A. J. Walkinshaw, A. Casitas, M. Taylor, M. G. Suero and M. J. Gaunt, J. Am. Chem. Soc., 2014, 136, 8851 CrossRef CAS PubMed;
(d) D. Zhu, Y. Wu, B. Wu, B. Luo, A. Ganesan, F. Wu, R. Pi, P. Huang and S. Wen, Org. Lett., 2014, 16, 2350 CrossRef CAS PubMed;
(e) J. Peng, C. Chen, J. Chen, X. Su, C. Xi and H. Chen, Org. Lett., 2014, 16, 3776 CrossRef CAS PubMed;
(f) Á. Sinai, Á. Mészáros, T. Gáti, V. Kudar, A. Palló and Z. Novák, Org. Lett., 2013, 15, 5654 CrossRef PubMed.
-
(a) J. K. Qiu, B. Jiang, Y. L. Zhu, W. J. Hao, D. C. Wang, J. Sun, P. Wei, S. J. Tu and G. Li, J. Am. Chem. Soc., 2015, 137, 8928 CrossRef CAS PubMed;
(b) Z. Z. Chen, S. Liu, W. J. Hao, G. Xu, S. Wu, J. N. Miao, B. Jiang, S. L. Wang, S. J. Tu and G. Li, Chem. Sci., 2015, 6, 6654 RSC.
-
(a) W. Hao, J. Zeng and M. Cai, Chem. Commun., 2014, 50, 11686 RSC;
(b) T. Saito, Y. Sonoki, T. Otani and N. Kutsumura, Org. Biomol. Chem., 2014, 12, 8398 RSC;
(c) M. Kaname and H. Sashida, Tetrahedron Lett., 2012, 53, 748 CrossRef CAS;
(d) T. Otani, S. Kunimatsu, T. Takahashi, H. Nihei and T. Saito, Tetrahedron Lett., 2009, 50, 3853 CrossRef CAS;
(e) T. Saito, H. Nihei, T. Otani, T. Suyama, N. Furukawa and M. Saito, Chem. Commun., 2008, 172 RSC;
(f) T. Otani, S. Kunimatsu, H. Nihei, Y. Abe and T. Saito, Org. Lett., 2007, 9, 5513 CrossRef CAS PubMed.
- L. Benati, G. Calestani, R. Leardini, M. Minozzi, D. Nanni, P. Spagnolo, S. Strazzari and G. Zanardi, J. Org. Chem., 2003, 68, 3454 CrossRef CAS PubMed.
-
(a) A. J. Walkinshaw, W. Xu, M. G. Suero and M. J. Gaunt, J. Am. Chem. Soc., 2013, 135, 12532 CrossRef CAS PubMed;
(b) R. J. Phipps, L. McMurray, S. H. Ritter, A. Duong and M. J. Gaunt, J. Am. Chem. Soc., 2012, 134, 10773 CrossRef CAS PubMed.
- W. Guo, S. Li, L. Tang, M. Li, L. Wen and C. Chen, Org. Lett., 2015, 17, 1232 CrossRef CAS PubMed.
-
(a) J. Xu, P. Zhang, Y. Gao, Y. Chen, G. Tang and Y. Zhao, J. Org. Chem., 2013, 78, 8176 CrossRef CAS PubMed;
(b) D. D. Tanner, D. W. Reed and B. P. Setiloane, J. Am. Chem. Soc., 1982, 104, 3917 CrossRef CAS.
Footnote |
† Electronic supplementary information (ESI) available. CCDC 1063949 and 1438783. For ESI and crystallographic data in CIF or other electronic format see DOI: 10.1039/c6qo00133e |
|
This journal is © the Partner Organisations 2016 |