DOI:
10.1039/C6QO00091F
(Research Article)
Org. Chem. Front., 2016,
3, 697-700
A palladium-catalyzed tandem reaction of 2-alkynylbenzenesulfonamides with 2-(2-bromoarylidene)cyclobutanones†
Received
4th March 2016
, Accepted 25th March 2016
First published on 28th March 2016
Abstract
The core of benzo[f][1,2]thiazonin-5(4H)-one 3,3-dioxide is efficiently assembled through a palladium-catalyzed tandem reaction of 2-alkynylbenzenesulfonamides with 2-(2-bromobenzylidene)cyclobutanones. A range of polycycles with a nine-membered sultam ring are generated in moderate to good yields. During the transformation, three new bonds are formed via a tandem process with the construction of a nine-membered sultam ring. Double carbometallation is believed to be involved with the ring-opening of cyclobutanone.
Introduction
Currently, continuous efforts have been made for the synthesis of complex heterocycles with potential biological activities. So far, many strategies have been developed for accessing these molecules.1 Among the approaches utilized, tandem reactions have attracted much more attention due to their efficiency, experimental ease, and atom economy.2 As part of a program in our laboratory for the generation of natural product-like compounds, we are interested in the development of tandem reactions for the assembly of N-heterocycles.3,4 For example, 2-alkynylbenzenebromide has been utilized successfully as a versatile building block for the generation of polycycles with complexity and diversity catalyzed by palladium salt.5 The tandem process was designed based on the multi-active sites of the substrates, which involved the alkynyl moiety and aryl bromide. During the transformation, double carbometallation of triple bonds was demonstrated as the key step for the outcome of excellent regioselectivity and chemoselectivity.4 Recently, the structure of 2-(2-bromoarylidene)cyclobutanone attracted our attention, which was reported by Yu and co-workers in the (PhSe)2-catalyzed Baeyer–Villiger oxidation.6 From the structure of 2-(2-bromoarylidene)cyclobutanone, we conceived that this compound might be used as a substitute for 2-alkynylbenzenebromide for the synthesis of diverse polycycles via double carbometallation as well. We reasoned that the active (arylidene)cyclobutanone could be considered as an equivalent to the alkynyl moiety during the reaction. Indeed, we found that 2-(2-bromobenzylidene)cyclobutanone could be efficiently employed in the palladium-catalyzed tandem reaction of 2-alkynylphenol for the generation of benzo[b]naphtho[2,3-d]oxocin-6-ones.7 As expected, good functional group tolerance as well as excellent selectivity was observed. The result was interesting, since several competitive pathways were minimized. Therefore, the excellent selectivity and reactivity prompted us to explore its further applications.
It is well known that cyclic sulfonamides can be found in many bioactive natural products and pharmaceuticals.8 For example, Tianeptine (trade-names: Stablon, Coaxil, Tatinol) is a drug for the treatment of major depressive episodes.9 Considering the synthetic efficiency as well as easy availability, 2-alkynylbenzenesulfonamide would be the choice for the preparation of cyclic sulfonamides.10 As shown in Scheme 1, in the presence of a palladium catalyst, the reaction of 2-alkynylbenzenesulfonamide 1 with aryl bromide would proceed through 6-endo cyclization or 5-exo cyclization, providing the corresponding benzo[e][1,2]thiazine 1,1-dioxide I or 3-methylene-benzo[d]isothiazole 1,1-dioxide II, respectively.10 During the reaction, an oxidative addition of Pd(0) to aryl bromide would occur firstly. The in situ generated Pd(II) would then activate the triple bond of 2-alkynylbenzenesulfonamide 1, thus facilitating the intramolecular nucleophilic addition of sulfonamide to alkyne to form cyclic sulfonamide. Encouraged by the advancement of double carbometallation,4 we postulated that another pathway might be feasible if 2-(2-bromobenzylidene)cyclobutanone 2 would be used as the source of aryl bromide. We reasoned that after the oxidative addition of Pd(0) to 2-(2-bromobenzylidene)cyclobutanone 2, a palladium(II) intermediate A would be formed. It might undergo direct coordination of Pd(II) with the triple bond of 2-alkynylbenzenesulfonamide 1, followed by insertion to provide intermediate B. Further carbometallation would occur to afford intermediate Cvia intramolecular insertion of Pd(II) to the double bond of 2-(2-bromobenzylidene)cyclobutanone 2. The subsequent aromatization as a driving force would lead to β-elimination with the cleavage of the C–C bond to produce intermediate D, which would then undergo intramolecular nucleophilic attack to generate the polycyclic nine-membered sultam 3. Since the excellent regioselectivity and chemoselectivity of double carbometallation has been demonstrated,4 we believed that this hypothesized synthetic route was feasible and the competitive pathways could be avoided (cyclization10 and direct C–N bond formation11). Therefore, we started to explore the practicability of this proposed palladium-catalyzed tandem reaction of 2-alkynylbenzenesulfonamide 1 with 2-(2-bromobenzylidene)cyclobutanone 2.
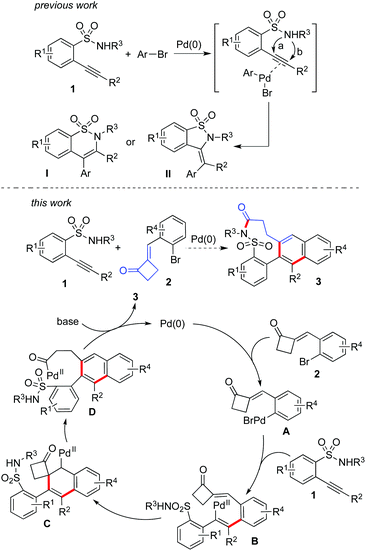 |
| Scheme 1 A proposed palladium-catalyzed reaction of 2-alkynylbenzenesulfonamides 1 with 2-(2-bromobenzylidene)cyclobutanones 2. | |
Results and discussion
At the outset, the reaction of N-methyl-2-(phenylethynyl)benzenesulfonamide 1a and 2-(2-bromobenzylidene)cyclobutanone 2a was selected as the model with an expectation to find the optimal conditions. Initially, the reaction was catalyzed by palladium acetate (5 mol%) in the presence of DPPF (1,1′-bis(diphenylphosphino)ferrocene) and potassium carbonate in 1,4-dioxane under reflux (Table 1, entry 1). The reaction failed, which afforded a trace amount of the product. Other ligands were then screened, and the same result was observed when DPPP (1,3-bis(diphenylphosphino)propane) or X-Phos (2-(dicyclohexylphosphino)-2′,4′,6′-triisopropylbiphenyl) was used as a substitute for the ligand (Table 1, entries 2 and 3). To our delight, the corresponding product 3a was obtained in 46% yield when tricyclohexylphosphine was added in the reaction system (Table 1, entry 4). The structure of compound 3a was verified via X-ray crystallography analysis (Fig. 1, also see the ESI†). As expected, three new bonds were formed via a tandem process with the construction of a nine-membered sultam ring during the transformation. We further examined several bases, and no better results were generated (Table 1, entries 5–8). Subsequently, various palladium catalysts were screened. It was found that palladium acetate was the best choice (Table 1, entries 9–12). We also investigated the reaction in different solvents (Table 1, entries 13–17). Again, the results were inferior when DMF, DMSO, toluene, diglyme (diethylene glycol dimethyl ether), or AmylOH (2-methyl-2-butanol) was utilized as the solvent. The reaction was retarded at 50 °C (Table 1, entry 18). Interestingly, the corresponding product 3a was obtained in 55% yield when the reaction was performed at 80 °C (Table 1, entry 19).
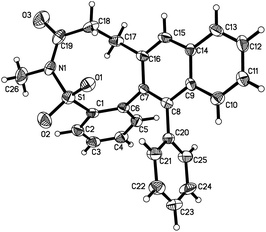 |
| Fig. 1 ORTEP illustration of compound 3a (30% probability ellipsoids). | |
Table 1 Initial studies for the palladium-catalyzed reaction of N-methyl-2-(phenylethynyl)benzenesulfonamide 1a with 2-(2-bromobenzylidene)cyclobutanone 2aa
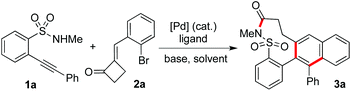
|
Entry |
[Pd] |
Ligand |
Base |
Solvent |
Yieldb (%) |
Reaction conditions: N-methyl-2-(phenylethynyl)benzenesulfonamide 1a (0.2 mmol), 2-(2-bromobenzylidene)cyclobutanone 2a (0.24 mmol), palladium catalyst (5 mol%), phosphine ligand (10 mol%), base (0.4 mmol), solvent (2.0 mL), reflux, N2, overnight.
Isolated yield based on N-methyl-2-(phenylethynyl)benzenesulfonamide 1a.
The reaction was performed at 50 °C.
The reaction occurred at 70 °C.
The reaction was performed at 80 °C.
|
1 |
Pd(OAc)2 |
DPPF |
K2CO3 |
Dioxane |
Trace |
2 |
Pd(OAc)2 |
DPPP |
K2CO3 |
Dioxane |
Trace |
3 |
Pd(OAc)2 |
X-Phos |
K2CO3 |
Dioxane |
Trace |
4 |
Pd(OAc)2 |
PCy3 |
K2CO3 |
Dioxane |
46 |
5 |
Pd(OAc)2 |
PCy3 |
Na2SO3 |
Dioxane |
nd |
6 |
Pd(OAc)2 |
PCy3 |
Cs2CO3 |
Dioxane |
12 |
7 |
Pd(OAc)2 |
PCy3 |
KOAc |
Dioxane |
13 |
8 |
Pd(OAc)2 |
PCy3 |
K3PO4 |
Dioxane |
36 |
9 |
Pd(TFA)2 |
PCy3 |
K2CO3 |
Dioxane |
Trace |
10 |
PdCl2(PhCN)2 |
PCy3 |
K2CO3 |
Dioxane |
15 |
11 |
PdCl2 |
PCy3 |
K2CO3 |
Dioxane |
20 |
12 |
PdCl2(PCy3)2 |
PCy3 |
K2CO3 |
Dioxane |
33 |
13 |
Pd(OAc)2 |
PCy3 |
K2CO3 |
DMF |
nd |
14 |
Pd(OAc)2 |
PCy3 |
K2CO3 |
DMSO |
nd |
15 |
Pd(OAc)2 |
PCy3 |
K2CO3 |
Toluene |
Trace |
16 |
Pd(OAc)2 |
PCy3 |
K2CO3 |
Diglyme |
14 |
17 |
Pd(OAc)2 |
PCy3 |
K2CO3 |
AmylOH |
10 |
18c |
Pd(OAc)2 |
PCy3 |
K2CO3 |
Dioxane |
nd |
19d |
Pd(OAc)2 |
PCy3 |
K2CO3 |
Dioxane |
46 |
20e |
Pd(OAc)2 |
PCy3 |
K2CO3 |
Dioxane |
55 |
The reaction scope was then explored under the optimized conditions. The result is shown in Table 2. The reactions of 2-alkynylbenzenesulfonamides 1 with 2-(2-bromobenzylidene)cyclobutanones 2 proceeded well to provide the corresponding 8,9-dihydrobenzo[h]naphtho[2,3-f][1,2]thiazonin-7(6H)-one 5,5-dioxides 3 in moderate to good yields. The core of the nine-membered sultam ring could be constructed efficiently. A range of 2-alkynylbenzenesulfonamides 1 were examined, and the substrates with electron-donating or electron-withdrawing groups on the aromatic ring were all compatible. Additionally, 2-alkynylbenzenesulfonamides 1 with different substituents attached on the triple bond were good partners as well. Reactions of different 2-(2-bromobenzylidene)cyclobutanones 2 were explored in the meantime. As expected, the transformations proceeded smoothly, leading to the desired products.
Table 2 Scope exploration of the palladium-catalyzed reactions of 2-alkynylbenzenesulfonamides 1 with 2-(2-bromobenzylidene)cyclobutanones 2a
Isolated yield based on 2-alkynylbenzenesulfonamide 1.
|
|
Conclusions
In conclusion, we have discovered that the core of benzo[f][1,2]thiazonin-5(4H)-one 3,3-dioxide can be efficiently assembled through a palladium-catalyzed tandem reaction of 2-alkynylbenzenesulfonamides with 2-(2-bromobenzylidene)cyclobutanones. A range of polycycles with a nine-membered sultam ring are generated in moderate to good yields. During the transformation, three new bonds are formed via a tandem process with the construction of a nine-membered sultam ring. Double carbometallation is believed to be involved with the ring-opening of cyclobutanone, which shows excellent regioselectivity and chemoselectivity during the reaction process.
Acknowledgements
Financial support from the National Natural Science Foundation of China (no. 21372046 and 21532001) is gratefully acknowledged.
Notes and references
- For recent reviews, see:
(a) L. Levi and T. J. J. Müller, Chem. Soc. Rev., 2016 10.1039/C5CS00805K;
(b) A. Lledó, A. Pla-Quintana and A. Roglans, Chem. Soc. Rev., 2016 10.1039/C5CS00535C;
(c) Z. Zheng, Z. Wang, Y. Wang and L. Zhang, Chem. Soc. Rev., 2016 10.1039/C5CS00887E;
(d) L. Liu and J. Zhang, Chem. Soc. Rev., 2016, 45, 506 RSC;
(e) X. Guo, D. Gu, Z. Wu and W. Zhang, Chem. Rev., 2015, 115, 1622 CrossRef CAS PubMed;
(f) B. Eftekhari-Sis and M. Zirak, Chem. Rev., 2015, 115, 151 CrossRef CAS PubMed;
(g) R. Chinchilla and C. Nájera, Chem. Rev., 2014, 114, 1783 CrossRef CAS PubMed;
(h) X. Wu, H. Neumann and M. Beller, Chem. Rev., 2013, 113, 1 CrossRef CAS PubMed;
(i) A. Goel, A. Kumar and A. Raghuvanshi, Chem. Rev., 2013, 113, 1614 CrossRef CAS PubMed;
(j) G. S. Singh, K. Mollet, M. D'hooghe and N. D. Kimpe, Chem. Rev., 2013, 113, 1441 CrossRef CAS PubMed;
(k) P. Lu and Y. Wang, Chem. Soc. Rev., 2012, 41, 5687 RSC.
- For selected reviews, see:
(a)
L. F. Tietze, G. Brasche and K. Gericke, Domino Reactions in Organic Synthesis, Wiley-VCH, Weinheim, Germany, 2006 Search PubMed;
(b) J. H. Kim, Y. O. Ko, J. Bouffard and S.-g. Lee, Chem. Soc. Rev., 2015, 44, 2489 RSC;
(c) L. F. Tietze, M. A. Düfert, T. Hungerland, K. Oum and T. Lenzer, Chem. – Eur. J., 2011, 17, 8452 CrossRef CAS PubMed;
(d) J. Panteleev, L. Zhang and M. Lautens, Angew. Chem., Int. Ed., 2011, 50, 9089 CrossRef CAS PubMed.
-
(a) G. Qiu and J. Wu, Chem. Rec., 2016, 16, 19 CrossRef CAS PubMed;
(b) G. Qiu, Y. Kuang and J. Wu, Adv. Synth. Catal., 2014, 356, 3483 CrossRef CAS;
(c) L. He, H. Nie, G. Qiu, Y. Gao and J. Wu, Org. Biomol. Chem., 2014, 12, 9045 RSC;
(d) G. Qiu, Q. Ding and J. Wu, Chem. Soc. Rev., 2013, 42, 5257 RSC;
(e) H. Wang, Y. Kuang and J. Wu, Asian J. Org. Chem., 2012, 1, 302 CrossRef CAS.
-
(a) Y. Luo, X. Pan, X. Yu and J. Wu, Chem. Soc. Rev., 2014, 43, 834 RSC;
(b) G. Qiu and J. Wu, Synlett, 2014, 2703 CAS.
- For selected examples, see:
(a) X. Pan, Y. Luo, Y. Ding, X. Fan and J. Wu, Adv. Synth. Catal., 2014, 356, 1072 CrossRef CAS;
(b) X. Pan, M. Chen, L. Yao and J. Wu, Chem. Commun., 2014, 50, 5891 RSC;
(c) Q. Xiao, Y. Luo, Y. Yan, Q. Ding and J. Wu, RSC Adv., 2013, 3, 5779 RSC;
(d) X. Pan, Y. Luo, G. Liu, S. Pu and J. Wu, Adv. Synth. Catal., 2012, 354, 171 CrossRef CAS;
(e) H. Wang, Y. Luo, B. Zhu and J. Wu, Chem. Commun., 2012, 48, 5581 RSC;
(f) X. Pan, Y. Luo and J. Wu, Chem. Commun., 2011, 47, 8967 RSC;
(g) Y. Luo, X. Pan and J. Wu, Org. Lett., 2011, 13, 1150 CrossRef CAS PubMed;
(h) Y. Luo, L. Hong and J. Wu, Chem. Commun., 2011, 47, 5298 RSC;
(i) Y. Luo and J. Wu, Chem. Commun., 2011, 47, 11137 RSC.
- L. Yu, Y. Wu, H. Cao, X. Zhang, X. Shi, J. Luan, T. Chen, Y. Pan and Q. Xu, Green Chem., 2014, 16, 287 RSC.
- X. Pan, Y. Luo, H. Xia and J. Wu, Chem. Commun., 2015, 51, 16483 RSC.
- For selected examples:
(a) A. K. Ganguly, S. S. Alluri, D. Caroccia, D. Biswas, C.-H. Wang, E. Kang, Y. Zhang, A. T. McPhail, S. S. Carroll, C. Burlein, V. Munshi, P. Orth and C. Strickland, J. Med. Chem., 2011, 54, 7176 CrossRef CAS PubMed;
(b) L. Kiefer, T. Gorojankina, P. Dauban, H. Faure, M. Ruat and R. H. Dodd, Bioorg. Med. Chem. Lett., 2010, 20, 7483 CrossRef CAS PubMed;
(c) P. Gilleron, N. Wlodarczyk, R. Houssin, A. Farce, G. Laconde, J.-F. Goossens, A. Lemoine, N. Pommery, J.-P. Henichart and R. Millet, Bioorg. Med. Chem. Lett., 2007, 17, 5465 CrossRef CAS PubMed;
(d) G. Laconde, P. Depreux, P. Berthelot, N. Pommery and J.-P. Hénichart, Eur. J. Med. Chem., 2005, 40, 167 CrossRef CAS PubMed;
(e) A. K. Ganguly, S. S. Alluri, D. Caroccia, D. Biswas, C.-H. Wang, E. Kang, Y. Zhang, A. T. McPhail, S. S. Carroll, C. Burlein, V. Munshi, P. Orth and C. Strickland, J. Med. Chem., 2011, 54, 7176 CrossRef CAS PubMed.
- S. Kasper and B. S. McEwen, CNS Drugs, 2008, 22, 15 CrossRef CAS PubMed.
- For selected examples, see:
(a) T. M. Ha, B. Yao, Q. Wang and J. Zhu, Org. Lett., 2015, 17, 5256 CrossRef CAS PubMed;
(b) M. Hellal and G. D. Cuny, Tetrahedron Lett., 2011, 52, 5508 CrossRef CAS;
(c) D. K. Barange, V. R. Batchu, D. Gorja, V. R. Pattabiraman, L. K. Tatini, J. M. Babu and M. Pal, Tetrahedron, 2007, 63, 1775 CrossRef CAS.
- For selected examples, see:
(a) P. Bhattacharya, K. Senapati, K. Chattopadhyay, S. M. Mandalb and A. Basak, RSC Adv., 2015, 5, 61562 RSC;
(b) M. K. Ghorai, M. Sayyad, Y. Nanaji and S. Jana, Chem. – Asian J., 2015, 10, 1480 CrossRef CAS PubMed;
(c) S. Shin, Y. Park, C. Kim, J. Son and P. Lee, J. Org. Chem., 2015, 80, 5859 CrossRef CAS PubMed;
(d) J. Zhang, D. Cao, H. Wang, G. Zhao and Y. Shang, Tetrahedron, 2015, 71, 1785 CrossRef CAS;
(e) S. Fang, X. Niu, Z. Zhang, Y. Sun, X. Si, C. Shan, L. Wei, A. Xu, L. Feng and C. Ma, Org. Biomol. Chem., 2014, 12, 6895 RSC.
Footnote |
† Electronic supplementary information (ESI) available: Experimental procedure and related data. CCDC 1453052. For ESI and crystallographic data in CIF or other electronic format see DOI: 10.1039/c6qo00091f |
|
This journal is © the Partner Organisations 2016 |