Photo-Fries rearrangement of aryl acetamides: regioselectivity induced by the aqueous micellar green environment†
Received
27th September 2015
, Accepted 24th November 2015
First published on 1st December 2015
Abstract
Photochemical reactions tend to give more than one photoproduct. However, such a reaction can be a powerful synthetic tool when it is possible to conduct it in regioselective conditions yielding a single photoproduct. Water–surfactant solutions as reaction media can be considered as an approach in this context because they show products with different features than those from isotropic solutions. Here we describe results obtained from studying the effect on the prototypical photoreaction, known as the photo-Fries reaction of several substituted acetanilides and α-naphthyl acetamide within surfactant micelles (ionic and non-ionic micelles). This reaction involves homolytic cleavage of a C–N bond to yield a singlet radical pair. The surfactant micelles control the rotational and translational mobility of the radical pair, resulting in noticeable photoproduct selectivity.
Introduction
Generally, photochemical reactions tend to give more than one product. For such reactions to be useful in synthesis it is necessary to be able to control the experimental conditions to yield a single product. Therefore, product selectivity in reactions is a great challenge in synthetic organic photochemistry, especially when radical pairs or radical–ion pairs are formed as primary intermediate species. The use of confined assemblies, such as zeolites, micelles, polyolefin films, cavitands, dendrimers, etc., can be considered as strategies devised to control product distributions in photochemical reactions.1 The restricted mobility within the hydrophobic cores of these confined assemblies limits the tendency of radicals, radical cations, or other reactive intermediates to undergo radical-destroying bimolecular reactions and prevents the access to adventitious reagents (i.e. water and oxygen) that would cause their decay in solution. In the present article we describe the results of our studies on the use of cationic, anionic and neutral surfactant micelles, which are capable of solubilizing organic molecules (hydrophobic molecules) in water, as the media in achieving product selectivity in the prototypical photoreaction, the photo-Fries reactions of several substituted aryl acetanilides.
The photo-Fries rearrangement reaction was discovered by Anderson and Reese2 and involves the homolytic cleavage of a carbon–heteroatom bond, i.e., C–O, C–S and C–N, of esters, thioesters and amides, respectively.3 The radical mechanism of the photo-Fries rearrangement is well established and it is known that this rearrangement occurs mainly through the excited singlet state.1i,4 Previous studies on the product distribution of the photo-Fries rearrangement reaction of (hetero)aryl amides in isotropic media afforded ortho- and para-rearranged photoproducts as well as the corresponding aryl amine (Scheme 1).5
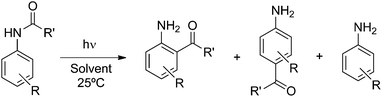 |
| Scheme 1 The photo-Fries rearrangement of acetanilides. | |
Surfactants, which are amphiphilic molecules, aggregate in solution to form micelles when their concentration is above the critical micellar concentration (cmc). Under these conditions, the micelles enhance the solubility of hydrophobic compounds in water. In fact, the most important property of micelles is that they have the ability to concentrate guest molecules into relatively small effective volumes and then to promote the interaction of such molecules.1c,6 The model of a micelle usually presented is such that the interior contains the hydrophobic chain part of the amphiphilic moiety while the polar head group (charged or neutral head) is located at the surface, forming a double layer in contact with the surrounding water. Micelles are not a static species but rather exist in a dynamic equilibrium with the surfactant monomers, they can be employed to organize organic substrates.7 In micelles, large cage effects are observed relative to homogeneous solvents whose magnitude cannot be explained by the microviscosity inside the micelle. The main reason for this observation is that the hydrophobicity of the solutes inhibits diffusion into the aqueous phase, thereby increasing the time spent by the reaction intermediates in the restricted space of the micelle. If the intermediates are geminate radical pairs, which are produced photochemically, the rotational and translational mobility of these species are constrained inside the micelles. Therefore, the product distribution and the relative chemical yield of the products observed in micellar solution can be significantly different relative to homogeneous solution.1m,7
There have been a lot of studies on controlling the reactivity of radicals generated within the micelle supercage where polarity, viscosity, electrostatic, and hydrophobic interactions as well as hydrogen-bonding solvation may play critical roles in determining their reactivities.8 In addition, several studies designed to analyze and quantify micellar cage effects (reactivity, selectivity and efficiency) on photochemical reactions in water have been performed. Because the photo-Fries rearrangement involves in-cage recombination versus diffusion of radicals, aryl esters have been chosen as model substrates to study the non-homogeneous environments.9 In these studies, SDS was also the preferred surfactant among other ionic surfactants for carrying out the photoreactions. However, some examples in the literature show that the photo-Fries rearrangement reaction of benzamides in SDS micellar solution and acetanilides in water–cyclodextrin systems have also been studied.9f,g Since 2-amino arylketones are key synthons in the preparation of 4-quinolones and their derivatives, with demonstrated biological and pharmaceutical properties,10 we decided to study the photo-Fries rearrangement of a series of substituted aryl and polycyclic aryl amides in micellar solution in order to evaluate if this provides high selectivity for 2-amino phenone formation within the micelle assembly. The structures of the aryl acetamides and surfactants employed in this study are shown in Scheme 2.
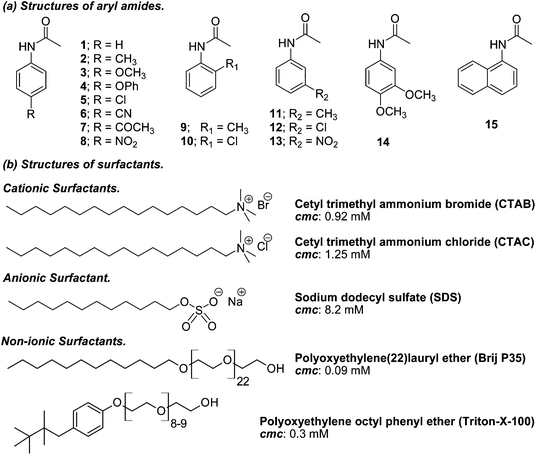 |
| Scheme 2 Structures of the aryl acetamides and the surfactants studied. | |
Experimental
Materials and equipment
Acetanilide, ortho-, meta and para-substituted anilines, 3,4-dimethoxyaniline and α-naphthylacetamide were purchased from Aldrich and were used without further purification. Surfactants (SDS, Brij, CTAC, CTAB, Triton X-100 and Tween 80) were purchased from Fluka and Sigma Aldrich. Spectrograde solvents were obtained from J. T. Baker and were used as received. Pyridine was distilled and stored over KOH pellets. Melting points were determined using a Fisher Jones apparatus and are not corrected. 1H and 13C NMR spectra were registered using a Bruker AC-200 spectrometer in CDCl3; chemical shifts (δ) are reported in parts per million (ppm), relative to internal tetramethylsilane. 2D NOESY spectra were registered using a Bruker AC-500 spectrometer in D2O; chemical shifts (δ) are reported in parts per million (ppm), relative to internal trimethylsilylpropionic acid. Coupling constant (J) values are given in Hz. The measurements were carried out using standard pulse sequences. GC analysis was carried out using a Hewlett Packard 5890 gas chromatograph using an Ultra 2 capillary chromatographic column. For amides 1–14, the chromatograms were recorded with the following program: initial temperature: 100 °C, 2 minutes; rate: 10 °C min−1; final temperature: 250 °C, 10 minutes. For amide 15, the chromatograms were recorded as follows: initial temperature: 150 °C, 2 minutes; rate: 10 °C min−1; final temperature: 250 °C, 15 minutes. Reverse phase HPLC analysis was carried out using a JASCO PU 1580 with a UV detector PU 1575 using a RP–18 reverse chromatographic column (Supelco; eluent: MeCN–H2O mixtures; flow: 1 mL min−1; detector: UV, λ 230 and 260 nm). The UV-visible spectra were measured using a Shimadzu UV-1203 spectrophotometer. All the measurements were made using 1 cm stoppered quartz cells at 298 K.
Determination of the constants of binding (Kb) of acetanilides in micellar media
Solutions of acetanilides 4, 11, 12 and 13 were prepared in deionized water (MilliQ) and their concentration varied between 5.5 × 10−5 M and 1.0 × 10−4 M. An aliquot (2 mL) of the acetanilide solution was placed in a two-faced stoppered quartz cuvette provided with a stir bar and the UV-visible spectrum was registered, then the A0 value at the maximum wavelength was read. Next, aliquots of concentrated surfactant solution (10 μL) were added successively and the UV-visible spectra were registered taking for each solution the A value at the maximum wavelength. After each addition of surfactant solution the resulting solution was stirred for 20 minutes. With the values of A0 and A in hand, plots of (A0/(A − A0)) versus the reciprocal of the concentration of the surfactants were created and the data were fitted with a linear regression program provided by SigmaPlot version 11.0. From the ratio of the slope and the origin it was easy to calculate the Kb values.
Synthesis of acetanilides 2–15
To a solution of the acetanilides (0.010 mol) in pyridine (10 mL) chilled in an ice-bath was added dropwise acetyl chloride (0.012 mol) for 10 minutes under stirring. The reaction mixture stood for an additional 45 minutes under stirring. Once tlc confirmed total consumption of the starting material, the reaction mixture was extracted with dichloromethane (10 mL) and washed with a solution of diluted HCl (10 mL). The organic phase was then washed with water, dried with Na2SO4, filtrated and evaporated under pressure. The acetanilides were purified from the solid residue using column chromatography with hexane and ethyl acetate mixtures giving the corresponding acetanilides in excellent yields (>90%). The acetanilides 2–14 were characterized by comparison with the physical constant (m.p.) and spectroscopical data (1H-NMR and 13C-NMR) reported in the literature. These data and the corresponding references are listed in ESI.†
Photoirradiations
General procedure.
Photoirradiations of acetanilides in homogeneous media.
Stock solutions of amides 1–15 (0.106 mmol) in cyclohexane (100 mL) were prepared. An aliquot (65 mL) was placed in a stoppered Erlenmeyer quartz flask (100 mL) and was degassed with argon for 30 min. The flask was placed in a home made optical bench which provided the possibility to use two or four lamps. The solutions of the amides were stirred during the irradiation process. Irradiations with λexc = 313 nm were carried out with four phosphorous–coated lamps (HelioQuartz, each of 18 Watts, purchased in Italy) that give a nearly parallel beam at 313 nm. Irradiations with λexc = 254 nm were carried with four germicidal lamps (Philips, each of 20 Watts, purchased in Argentina). The progress of the reaction was monitored using TLC [eluent: hexane–ethyl acetate (8
:
2 v/v); spots were visualized with UV light (254 and 366 nm) and with I2] and by GC analysis (Ultra 2 capillary column) following the programs above mentioned depending on the amide analyzed. When the conversion of the starting material was higher than 90%, the photolyzed solution was evaporated carefully to dryness under reduced pressure. The yellowish solid residue obtained was worked up by silica gel column chromatography (eluent: hexane 100% followed by hexane–ethyl acetate mixtures). From the eluted fractions, the photoproducts were isolated and characterized by means of physical and spectroscopic methods.
Photoirradiations of acetanilides in micellar media.
Stock solutions of surfactants in deionized water were freshly prepared and the concentrations of these solutions were: SDS 0.10 M; CTAC 0.02 M; CTAB 0.02 M; Brij-P35 0.05 M; Triton-X-100 0.05 M and Tween 80 0.10% (v/v). The acetanilide (5 mg) was placed in a stoppered quartz cell provided with a stir bar (3 ml) and the surfactant stock solution (2 mL) was added. Then, the solution was vigorously stirred for one hour and degassed with argon for an additional 20 min. The quartz cell was placed in a homemade optical bench equipped with two germicidal lamps (Philips, each of 20 Watts, purchased in Argentina). The progress of the photoreaction was monitored using two different methods, UV-visible spectroscopy and GC analysis (Ultra 2 capillary column). The conversion of the amides was kept below 20% to avoid secondary reactions and the formation of by-products. Prior to injection into the GC apparatus, the micellar solutions were treated as follows. The photolyzed solutions were diluted with 2 mL of an aqueous solution of NaCl and then extracted with ethyl acetate (3 × 2 mL) while the system was carefully shaken. The organic layer was separated, dried over Na2SO4 and evaporated to dryness under vacuum. The yellowish solid residue was dissolved using dicloromethane (2.00 mL) and this new solution was injected into the GC for chromatographic analysis. The products were characterized by comparison with the physical constant (m.p.) and spectroscopical data (1H-NMR and 13C-NMR) reported in the literature. These data and the corresponding references are listed in ESI.† The substituted anilines 1c–15c were identified by comparison of their chromatographic characteristics with those of authentic commercial samples.
Results and discussion
The use of micelles of ionic and neutral surfactants to carry out photoreactions requires knowledge of the location of the reactants in the system. We conducted UV-visible and 1H NMR studies of guest molecules (acetanilides) within micelles to gain an understanding of the reactants’ positioning. Our first approach was to determine the binding constant (Kb) between acetanilides and micelles using UV-visible spectroscopy. Among the guest acetanilides investigated, we selected acetanilides 4, 11, 12 and 13 which were soluble enough in water to record the UV-visible spectrum and then, to determine the Kb. The UV-visible spectra of 4 and 13 in water in the presence and absence of the surfactants Brij-P35 and CTAC, respectively, are presented in Fig. 1. Addition of increasing amounts of surfactant produced both bathochromic and hyperchromic shifts of the lower energy band. This spectroscopic behaviour demonstrates that the 4 and 13 acetanilides bind to the micelle and we propose that this molecular interaction takes place with the hydrophobic core of the micelle. A similar behaviour was observed for acetanilides 11 and 12 and the UV-visible spectra are shown in Fig. 1S (see ESI†).
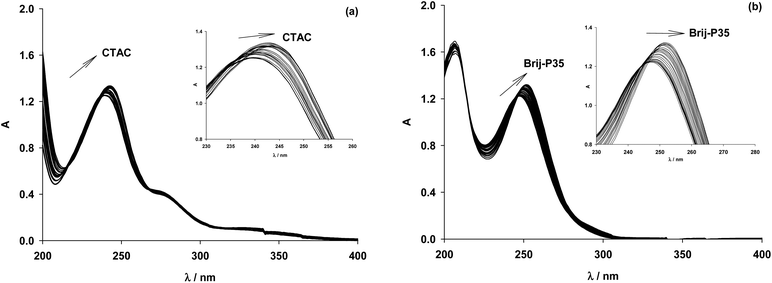 |
| Fig. 1 UV-visible spectra of acetanilides. Effect of addition of surfactants in the acetanilide water solution (room temperature): (a) acetanilide 13 with increasing concentration of CTAC and (b) acetanilide 4 with increasing concentration of Brij-P35. Insets: partial spectrum magnification showing the hyperchromic and bathochromic shifts for acetanilide 13 around 240 nm (plot (a)) and for acetanilide 4 around 247 nm (plot (b)). | |
Furthermore, if the binding of the guest to the micelle can be described as an equilibrium, Kb can be written according to eqn (1) where S is the acetanilide, Surf is the surfactant and [S-Surf] is the acetanilide surfactant complex.
|  | (1) |
The application of the Lambert–Beer law to eqn (1) gives eqn (2) where A and A0 are the absorbance at the maxima wavelength in the presence and absence of surfactant, respectively, and εS and εC are the molar absorptivity of the acetanilides and the complex. Rearranging eqn (2) provides eqn (3) where a linear relationship is observed between (A − A0)−1 and the reciprocal of the concentration of the surfactant.
| 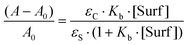 | (2) |
|  | (3) |
Fig. 2 shows the experimental data obtained for the acetanilide 4-Brij-P35 and acetanilide 13-CTAC systems and also shows the best linear regression curves. The plots for the other surfactants are shown in Fig. 2S (see ESI†).
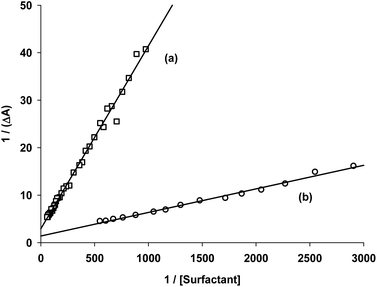 |
| Fig. 2 Plot of reciprocal of ΔA vs. reciprocal of concentration of surfactants in water at room temperature: (a) acetanilide 13 with CTAC and (b) acetanilide 4 with Brij-P35. | |
From the ratio of the slope and the origin it was easy to calculate the Kb values which are collected in Table 1 for acetanilides 4, 11, 12 and 13 with different surfactants. These data show that the acetanilides bind to the micelles and those that have a hydrophobic character, i.e. acetanilide 4, show high values.
Table 1 Binding constant (Kb) in water of acetanilides 4, 11, 12 and 13 in the presence of different surfactants at room temperature
Acetanilides |
K
b/M−1 |
SDS |
CTAC |
CTAB |
Brij-P35 |
4
|
109 |
552 |
459 |
214 |
11
|
7 |
11 |
32 |
63 |
12
|
17 |
135 |
113 |
58 |
13
|
81 |
76 |
70 |
33 |
Savelli and co-workers have estimated a Kb value ≤100 M−1 for substrates that are not very hydrophobic in SDS, CTAC and CTAB such as phenyl chloroformate.11 Furthermore, Quina, Treiner and co-workers pointed out that these values of Kb are typical of organic solutes with aryl moieties.12 This is the case for acetanilides 11, 12 and 13 whose Kb values are lower than 100 M−1. This behaviour can be attributed to the fact that these acetanilides are soluble enough in water at the concentration (≈ 1 × 10−5 M) that the UV-visible experiments were carried out at. Noteworthy is the case of acetanilide 11 where the Kb values in SDS, CATC and CTAB are much lower which is attributed to the high solubility of the acetanilide in water. Acetanilide 4 is an example of a hydrophobic amide. It does not demonstrate much solubility in water at the concentration used in the UV-visible experiments and the Kb values are near or greater than 100 M−1. Cabaleiro Lago and co-workers have estimated the Kb values between a series of substituted benzoyl chlorides and the Brij-P35 surfactant and found that these values are higher than those obtained for CTAC and SDS.13 A minimum value of Kb for the Brij-P35 surfactant was estimated around 190 M−1 which accounts for a more apolar environment using pyrene as a micropolarity probe. Therefore, the data collected in Table 1 for acetanilides 4, 11–13 in this non-ionic surfactant show a similar trend to that which was observed for the ionic surfactants.
With the aim of searching for the location of the acetanilides within the hydrophobic core of the micelles, we conducted 1H NMR studies of guest molecules within surfactant micelles to gain an understanding of the reactants’ positioning. NMR spectroscopy has been employed previously to assess the location of a solute within the micellar assembly.14 Among the fifteen guests investigated, we selected acetanilides 1, 3, 5, 7, 8 and 11 and the surfactants CTAB, SDS and Brij-P35 for the 1D and 2D 1H NMR spectroscopy study. 1H NMR of the surfactants in D2O in the presence and absence of acetanilides showed differential effects on the various protons of the surfactants, causing slightly upfield and downfield shifts (∼0.1–0.01 ppm). The magnitudes of the change in the shifts are much above the value for typical errors in the chemical shifts equal to 0.002 ppm.14cTable 2 shows the differential chemical shifts for CTAB in the presence of several acetanilides. Similar spectroscopic behaviours were also observed for the surfactants SDS and Brij-P35 and the results are shown in Table 2S (see ESI†). The intimate interaction between the acetanilides and the hydrocarbon chains of the surfactants has a significant role in the surfactant proton shift. Thus, the differential chemical shifts shown in Tables 2 and 2S† suggest that the acetanilides could be located in the shell as well as the hydrophobic core of the surfactant micelles. This behaviour can be ascribed to the high concentration of acetanilide solutes used in our NMR experiments that are preferentially solubilized at the core/shell interface but spread further into the shell and the core of the micelle.14a
Table 2 Differential chemical shift (Δδ) of CTAB (70 mM) in the presence of acetanilides (10 mM) in D2O at room temperature
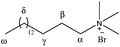
|
|
|
Δδ/ppm |
|
|
|
Acetanilides |
N+(CH3)3 |
α-CH2 |
β-CH2 |
γ-CH2 |
δ-CH2 |
ω-CH3 |
1
|
0.05 |
0.12 |
0.11 |
— |
−0.05 |
0.08 |
3
|
0.04 |
0.08 |
0.06 |
— |
−0.01 |
−0.03 |
5
|
0.03 |
0.08 |
0.08 |
— |
−0.04 |
−0.06 |
8
|
−0.01 |
0.004 |
−0.001 |
0.01 |
0.01 |
−0.02 |
Additional 1H NMR studies were carried out in order to confirm qualitatively the location of the acetanilides within the micelle assembly. 2D NOESY experiments were performed in D2O mixing a surfactant with an acetanilide in a ca. 1
:
1 molar ratio. Fig. 3 shows a 2D NOESY NMR spectrum for a solution of Brij-P35 (7 mM) in the presence of acetanilide 1 (10 mM) in D2O. The inset green frames identify the Nuclear Overhauser Effect (NOE) between the signals of the aromatic protons (H-2, H-3 and H-4) and the methyl protons (H-7) of acetanilide 1 and the signals of the surfactant (H-1, H-2, H-5 and H-6). The numbering of the protons of the surfactant Brij-P35 and acetanilide 1 are shown in Fig. 3. Similar results were obtained for solutions of SDS and CTAB in D2O in the presence of acetanilides 1, 7 and 11 and the corresponding 2D NOESY NMR spectra are shown in Fig. 3S (see ESI†). The intermolecular NOEs connecting the resonances of the acetanilide and surfactant are clearly visible in the figures confirming the results described with 1D NMR spectroscopy. Although a precise positioning cannot be estimated with certainty, we can suggest that the acetanilides are located in the shell as well as the hydrophobic core of the surfactant micelles taking into account that the aromatic protons as well as the methyl protons of the acetanilides correlate nicely with the different protons of the surfactants.
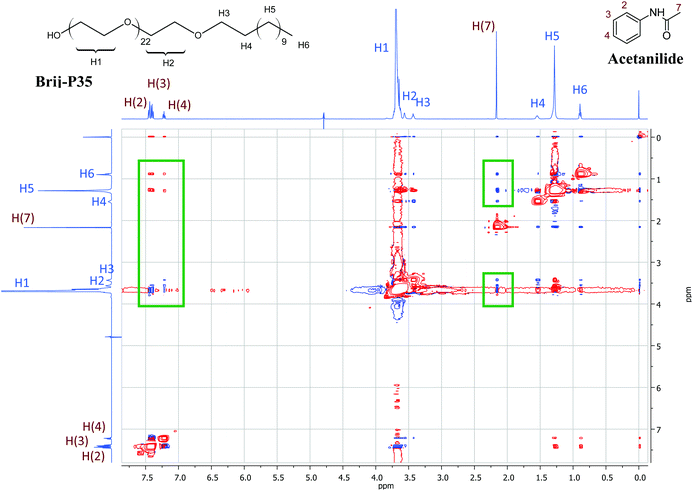 |
| Fig. 3 2D NOESY spectrum of a solution of Brij-P35 (7 mM) and 1 (10 mM) in D2O at room temperature. | |
The NMR spectroscopic results encouraged us to study the photochemical reactions of substituted acetanilides in water–surfactant solutions as reaction media because working with acetanilide concentrations around or higher than 5.0 × 10−3 M means that the substrates are spread within the shell/hydrophobic core of the micelle and the photoreaction is expected to take place within the micelle.
To examine the capability of neutral and ionic micelles as a reaction medium to induce photoproduct regio-selectivity from aryl amides, the unimolecular photochemical reaction, specifically the photo-Fries rearrangement of several substituted aryl acetamides and α-naphthyl acetamide (see Scheme 4) was investigated. In this reaction, upon irradiation, a molecule from its singlet excited state fragments to form a radical pair. Depending upon the mobility and confinement provided by the medium, the primary intermediates can yield various products as discussed below.
Irradiation of acetanilide (1) in cyclohexane, an isotropic medium that represents the behavior of a guest in an unrestricted environment, resulted in the formation of 45% 1a and 38% 1b (Table 3). A small amount of cage escape product (1c) was also observed. Irradiation of acetanilide 1 in an aqueous solution in the presence of neutral and ionic surfactants yields 2-acetyl aniline (1a) with noticeable selectivity and in high yield which is a quite different behavior compared to that in cyclohexane (Table 3). The selectivity obtained with the Brij-P35 surfactant is better (quantitative) than that obtained with micelles from classical surfactants such as SDS, CTAC, CTAB, Tween 80, and Triton-X100 where a significant amount of compound 1b (13–27%) was obtained. Furthermore, no aniline was observed when the irradiations of 1 were carried out in water in the presence of surfactants which evidences the suppression of products arising from cage escape. In all the experiments performed in water in the presence of surfactants, the concentration of the surfactants was 100 times higher than the cmc (see Table 3). Note that all photochemical reactions were kept at about 20% conversion, which is a standard operating procedure in mechanistic photochemistry.15 This avoids complications in analysis due to secondary photoreactions from the products of the reaction under investigation.
Table 3 Irradiation of acetanilide (1) in cyclohexane and micellar media
Solvent |
Surfactant |
Yielda (%) |

|

|

|
1a
|
1b
|
1c
|
λ
exc: 254 nm; atmosphere: Ar; [acetanilide]: 0.010 M; Δt: 360 min; T: 25 °C.
[Tween 80]: 0.10% v/v; Δt: 180 min.
|
Cyclohexane |
— |
45 |
38 |
15 |
H2O |
SDS (0.10 M) |
73 |
17 |
|
H2O |
Brij-P35 (0.05 M) |
>99 |
|
|
H2O |
CTAC (0.02 M) |
66 |
27 |
|
H2O |
CTAB (0.02 M) |
68 |
22 |
|
H2O |
Tween 80 b |
72 |
23 |
|
H2O |
Triton X-100 (0.05 M) |
85 |
13 |
|
The results presented above clearly demonstrate that the singlet radical pair A, i.e., anilinium radical and acetyl radical (see Scheme 3), generated in the shell/core of the micellar assembly is restrained within the cage eliminating diffusion into the aqueous phase, and thereby no aniline (1c) was obtained. Another important aspect, namely the higher ratio of the products 1a
:
1b in the micellar systems than in cyclohexane implies suppression of the rotational freedom of the encapsulated molecules in the micro-heterogeneous medium. Therefore, we can conclude that the lack of cage escape products and the selectivity of the photoreaction are due to a significant rigidity and confinement of the micellar assembly. However, there exists some amount of rotation for the radical pair A within the long chain micelles, resulting in the formation of 1b.
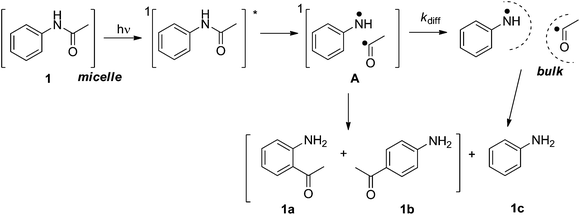 |
| Scheme 3 Photochemical reaction of acetanilide in micellar media. | |
Next, we studied the photo-Fries rearrangement of several mono ortho- and para- and also disubstituted acetanilides bearing electron donor and electron withdrawing groups in homogeneous (cyclohexane) and micellar media. In Scheme 4 are shown the photoreactions of the acetanilides (2–15) together with the corresponding product distribution. The chemical yields of the photoproducts are collected in Table 4.
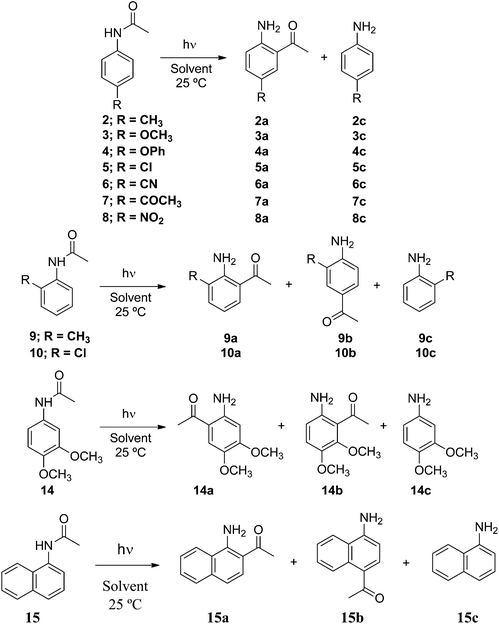 |
| Scheme 4 Photoreaction of acetanilides 2–15. | |
Table 4 Irradiation of acetanilides (2–7 and 9–15) in cyclohexane and water–surfactant media
Acetanilides |
2
|
3
|
4
|
5
|
6
|
7
|
Mediumb |
Yielda (%) |
2a
|
2c
|
3a
|
3c
|
4a
|
4c
|
5a
|
5c
|
6a
|
6c
|
7a
|
7c
|
Cyclohexane |
48 |
28 |
49 |
26 |
62 |
28 |
42 |
25 |
64 |
35 |
48 |
41 |
SDS |
88 |
9 |
95 |
2 |
93 |
6 |
98 |
|
88 |
22 |
>99 |
|
Brij |
95 |
2 |
>99 |
|
>99 |
|
97 |
|
>99 |
|
— |
|
CTAC |
>99 |
|
>99 |
|
>99 |
|
>99 |
|
73 |
27 |
>99 |
|
CTAB |
>99 |
|
>99 |
|
>99 |
|
>99 |
|
80 |
20 |
>99 |
|
Triton X-100 |
90 |
7 |
>99 |
|
90 |
5 |
>99 |
|
63 |
37 |
>99 |
|
Acetanilides |
9
|
10
|
14
|
15
|
Mediumb |
Yielda,c (%) |
9a
|
9b
|
9c
|
10a
|
10b
|
10c
|
14a
|
14b
|
14c
|
15a
|
15b
|
15c
|
λ
exc: 254 nm; atmosphere: Ar; [acetanilides]: 0.010 M; T: 25 °C.
Concentration of the surfactants in water as indicated in Table 3.
Irradiation of compound 15 (0.010 M) with λexc: 310 nm.
|
Cyclohexane |
42 |
30 |
15 |
39 |
27 |
16 |
51 |
17 |
15 |
40 |
35 |
15 |
SDS |
67 |
33 |
|
66 |
34 |
|
63 |
25 |
|
94 |
|
5 |
Brij |
73 |
27 |
|
69 |
31 |
|
73 |
17 |
|
97 |
|
|
CTAC |
64 |
36 |
|
54 |
46 |
|
70 |
14 |
|
98 |
|
|
CTAB |
70 |
30 |
|
61 |
36 |
|
51 |
17 |
15 |
95 |
1 |
2 |
Triton X-100 |
70 |
30 |
|
>99 |
|
|
63 |
25 |
|
93 |
|
|
The acetanilides 2–14 upon irradiation in cyclohexane afforded the migrated photoproducts and the corresponding substituted anilines (Table 4). No selectivity was observed in the homogeneous media. However, when the photoreactions were performed in water–surfactant media noticeable selectivity was observed. Irradiation of the para-substituted acetanilides bearing electron donor and electron withdrawing substituents (2–5 and 7) afforded the ortho-migrated products (2a–5a and 7a) in high to quantitative yields. However, in some few cases para-substituted anilines were detected in very low yields (≤9%) showing again a significant inhibition of the out-of-cage escape of the radical intermediates formed within the micelle.
Irradiation of compound 6 in all micellar media gives the desired ortho-migrated photoproduct (6a) in good yields together with significant amounts (22–37%) of p-cyanoaniline 6c. When surfactant Brij-P35 was used the photochemical reaction gave 6a in quantitative yield (Table 4). We attributed the formation of 6c to the partial solubility of acetanilide 6 in water where the photoreaction takes place simultaneously within the micellar assembly and in the bulk.
When 4-nitro acetanilide (8) was irradiated in isotropic and anisotropic media for 12 h no photo-Fries rearrangement took place, and the starting material was recovered quantitatively. This behavior can be explained by nitroarenes showing a high intersystem-crossing quantum yield (ϕISC = ca. 0.50) and hence, a change in the multiplicity of the excited state from a singlet to triplet excited state is promoted.16 Therefore, we propose that a population of the triplet excited state of acetanilide 8 is favored because the photo-Fries reaction of acetanilides takes place from the singlet excited state,3 and no reaction occurs from the triplet excited state of acetanilide 8.
The irradiation of the ortho-substituted acetanilides 9 and 10 in micellar solution showed a noticeable selectivity (see Table 4). No substituted anilines (9c and 10c) were formed while the migrated photoproducts were obtained in fairly good yield. The migration of the acetyl group to the ortho position of the phenyl moiety is favored over the para position giving an ortho
:
para ratio of ca. 2
:
1. It is worth noting that the irradiation of compound 10 in water–Triton X-100 media gives product 10a in quantitative yield. A similar behavior was observed for the irradiation of 3,4-dimethoxy acetanilide (14) in micellar media. The photoproducts 14a and 14b were formed exclusively, where 14a was always formed in yields higher than 14b; aniline 14c was not detected in the reaction mixture (see Table 4). This selectivity arises from the fact that because of steric hindrance the acetyl radical migrates preferentially to C-6 rather than to C-2 of the aromatic moiety.
Finally, a polycyclic aryl amide such as α-naphthyl acetamide (15) was also irradiated in cyclohexane at λexc 310 nm rendering the expected photoproducts 15a, 15b and 15c (see Table 4). When the photo-Fries reaction of 15 occurred in micellar solutions, compound 15a was formed in excellent yields independent of the nature of the surfactant employed. In few cases, photoproducts 15b and 15c were detected in the reaction mixture in very low yields.
It is worth noting that the irradiation of acetanilides 1, 9 and 10 does not provide the ortho rearranged photoproducts with high selectivity as was observed for the case of the p-substituted acetanilides (2–5 and 7). This behaviour can be attributed to the lower rigidity and confinement of conventional small molecule surfactant micelles when compared to more rigid and confined environments such as amphiphilic homopolymer nanopockets, capsules formed by a deep cavity cavitand and bile salt micelles.1l,m,n Therefore, the loss of rigidity of the micelles favoured the mobility of the singlet radical pairs inside the micelle rendering the corresponding para rearranged photoproducts (1b, 9b and 10b) in significant amounts (see Table 4). Thus, the flexibility of the micelles allows the acetyl radical to reach the para position of the anilinium radical formed during the irradiation. Similar conclusions have been reached by Ramamurthy and co-workers when studying the photo-Fries rearrangement of naphthyl esters in amphiphilic homopolymers and conventional surfactant micelle–water solutions.
The photochemical reaction of the acetanilides 1–7 and 9–15 within the micellar assembly showed a noticeable selectivity favouring the formation of the ortho regioisomers over the escape photoproducts, viz. the anilines. However, significant amounts of the para regioisomers were obtained for acetanilides 1, 9 and 10. The photoreaction proceeded efficiently with a variety of electron donor and electron withdrawing substituents at the para and ortho positions of the aryl moiety. Also, α-naphthyl acetamide, a polycyclic aryl moiety, rearranged with selectivity and high efficiency. These results showed the applicability of the sustainable water surfactant media in the preparation of ortho-amino phenones as an alternative and efficient methodology in organic synthesis.
Conclusions
The photo-Fries reaction of the included photoactive acetanilides has helped establish surfactant micelle assemblies as being capable of affecting the selectivity of this reaction by restricting the freedom of the reactive intermediates. Inclusion of the substrates in the shell as well as in the hydrophobic core of the micelles has been established and confirmed by 1D and 2D 1H-NMR spectroscopies. A noticeable selectivity in product distribution was observed in anisotropic media affording the ortho-migrated photoproducts with good to excellent yields. In all cases it is clear that inhibition of out-of-cage products, viz. anilines, occurs efficiently which means that the micelle assembly is rigid enough to prevent radical escape and/or to behave as a free radical scavenger. Another interesting feature of the micelle assembly is its effectiveness in dictating the behavior of singlet radical pairs suggesting that the micelles are able to constrict the mobility of short-lived radical pairs to some extent. However, significant amounts of the para regioisomers are obtained from acetanilides 1, 9 and 10. From a synthetic viewpoint, micellar media is a sustainable and alternative media that provides 2-acetyl anilines in excellent yields which are useful synthons in the preparation of quinolones.
Acknowledgements
The authors thank Universidad de Buenos Aires (X 0055BA), CONICET (PIP0072CO and PIP0155) and ANPCyT (PICT 2012-0888) for financial support. R.E.B. and S.M.B. are research members of CONICET. D. Iguchi was the recipient of a scholarship from CONICET (Doctorate Program).
Notes and references
-
(a)
M. A. Miranda and F. Galindo, in Photochemistry of Organic Molecules in isotropic and Anisotropic Media, ed. V. Ramamurthy and K. S. Schanze, Marcel Dekker, New York, 2003, ch. 2 Search PubMed;
(b)
A. Natarajan, L. S. Kaanumale and V. Ramamurthy, in CRC Handbook of Organic Photochemistry and Photobiology, ed. W. Horspool and F. Lenci, CRC Press, Boca Raton, FL, 2004, vol. 3, p. 107 Search PubMed;
(c)
J. H. Fendler and E. J. Fendler, in Catalysis in Micellar and Macromolecular System, Academic Press, London, 1975 Search PubMed;
(d)
Mixed Surfactant System, ed. P. M. Holland and D. N. Rubingh, American Chemical Society, Washington, DC, 1994 Search PubMed;
(e) N. J. Turro, From Boiling Stones to Smart Crystals: Supramolecular and Magnetic Isotope Control of Radical−Radical Reactions in Zeolites, Acc. Chem. Res., 2000, 33, 637–646 CrossRef CAS PubMed;
(f) K. Bhattachrya, Solvation Dynamics and Proton Transfer in Supramolecular Assemblies, Acc. Chem. Res., 2003, 36, 95–101 CrossRef PubMed;
(g) C. H. Tung, L. Z. Wu, L. P. Zhang and B. Cheng, Supramolecular Systems as Microreactors: Control of Product Selectivity in Organic Phototransformation, Acc. Chem. Res., 2003, 36, 39–47 CrossRef CAS PubMed;
(h) R. S. H. Liu and G. S. Hammond, Reflection on Medium Effects on Photochemical Reactivity, Acc. Chem. Res., 2005, 38, 396–403 CrossRef CAS PubMed;
(i) W. Gu and R. G. Weiss, Extracting fundamental photochemical and photophysical information from photorearrangements of aryl phenylacylates and aryl benzyl ethers in media comprised of polyolefinic films, J. Photochem. Photobiol., C, 2001, 2, 117–137 CrossRef CAS;
(j) V. Ramamurthy, Controlling photochemical reactions via confinement: zeolites, J. Photochem. Photobiol., C, 2000, 1, 145–166 CrossRef CAS;
(k) L. S. Kaanumalle, J. Nithyanandhan, M. Pattabiaman, N. Jayaraman and V. Ramamurthy, Water-Soluble Dendrimers as Photochemical Reaction Media: Chemical Behavior of Singlet and Triplet Radical Pairs Inside Dendritic Reaction Cavities, J. Am. Chem. Soc., 2004, 126, 8999–9006 CrossRef CAS PubMed;
(l) S. Arumugan, D. R. Vutukuri, S. Thayumanavan and V. Ramamurthy, Amphiphilic Homopolymer as a Reaction Medium in Water: Product Selectivity within Polymeric Nanopockets, J. Am. Chem. Soc., 2005, 127, 13200–13206 CrossRef PubMed;
(m) M. Pattabiraman, L. S. Kaanumalle and V. Ramamurthy, Photoproduct Selectivity in Reactions Involving Singlet and Triplet Excited States within Bile Salt Micelles, Langmuir, 2006, 22, 2185–2192 CrossRef CAS PubMed;
(n) L. S. Kaanumalle, C. L. D. Gibb, B. C. Gibb and V. Ramamurthy, Photo-Fries reaction in water made selective with a capsule, Org. Biomol. Chem., 2007, 5, 236–238 RSC;
(o) R. Kulasekharan, R. Choudhurry, R. Prabhakar and V. Ramamurthy, Restricted rotation due to the lack of free space within a capsule translates into product selectivity: photochemistry of cyclohexyl phenyl ketones within a water-soluble organic capsule, Chem. Commun., 2011, 47, 2841–2843 RSC.
- J. C. Anderson and C. B. Reese, Photoinduced Fries rearrangement, Proc. Chem. Soc., London, 1960, 217 CAS.
-
(a) D. Bellus, Photo-Fries rearrangement and related photochemical [1,j] – shift (j: 3, 5, 7) of carbonyl and sulfonyl groups, Adv. Photochem., 1971, 8, 109–159 CrossRef CAS;
(b)
M. A. Miranda, in CRC Handbook of Organic Photochemistry and Photobiology, ed. W. Horspool and P. S. Song, CRC Press, Boca Raton, FL, 1995, p. 570 Search PubMed.
-
(a) J. W. Meyer and G. S. Hammond, Mechanism of photochemical reactions in solution. LXX. Photolysis of aryl esters, J. Am. Chem. Soc., 1972, 94, 2219–2228 CrossRef CAS;
(b) C. E. Kalmus and D. S. Hercules, Thermal .pi.-route rearrangement of 4-cycloheptene-1-methyl acetate, J. Am. Chem. Soc., 1974, 96, 449–460 CrossRef CAS;
(c) N. P. Gristan, Y. P. Tsentalovich, A. V. Yurkovskay and R. Z. Sagdeev, Laser Flash Photolysis and CIDNP Studies of 1-Naphthyl Acetate Photo-Fries Rearrangement, J. Phys. Chem., 1996, 100, 4448–4458 CrossRef;
(d) S. M. Bonesi, L. K. Crevatin and R. Erra Balsells, Photochemistry of 2-acyloxycarbazoles. A potential tool in the synthesis of carbazole alkaloids, Photochem. Photobiol. Sci., 2004, 3, 381 RSC;
(e) L. C. Crevatin, S. M. Bonesi and R. Erra Balsells, Photo-Fries rearrangement of Carbazol-2-yl Sulfonates. Efficient tool for the introduction of sulfonyl groups into polycyclic aromatic compounds, Helv. Chim. Acta, 2006, 89, 1147 CrossRef CAS.
-
(a) H. Shizuka and I. Tanaka, Photochemistry of Acetanilide. I. Quantum Yields of the Rearrangement and Benzene Photosensitized Reaction, Bull. Chem. Soc. Jpn., 1968, 41, 2343–2349 CrossRef CAS;
(b) H. Shizuka, Photochemistry of Acetanilide. II. The Primary Processes in the Photochemical Reaction, Bull. Chem. Soc. Jpn., 1969, 42, 52–57 CrossRef CAS;
(c) H. Shizuka, Photochemistry of Acetanilide. III. The Secondary Processes in the Photochemical Reaction, Bull. Chem. Soc. Jpn., 1969, 42, 57–65 CrossRef CAS;
(d) H. Shizuka, M. Kato, T. Ochaiai, K. Matsui and T. Morita, The Photochemical Rearrangement of Phenyl Acetate, Bull. Chem. Soc. Jpn., 1969, 42, 1831–1836 CrossRef CAS;
(e) H. Shizuka, M. Kato, T. Ochaiai, K. Matsui and I. Tanaka, The Photochemical Rearrangements of N-Acetyl Diphenylamine and N-Acetyl Carbazole, Bull. Chem. Soc. Jpn., 1970, 43, 67–74 CrossRef CAS;
(f) S. M. Bonesi and R. Erra Balsells, Product Study of the photolysis of N-acetyl carbazole in ethanol and dichloromethane solution. Part I, J. Photochem. Photobiol., A, 1991, 56, 55 CrossRef CAS;
(g) S. M. Bonesi and R. Erra Balsells, Photochemical behaviour of N-acetyl and N-benzoyl carbazole: photo-Fries rearrangenent and photoinduced single electron transfer, J. Photochem. Photobiol., A, 1997, 110, 271 CrossRef CAS.
- F. M. Menger, The structure of micelles, Acc. Chem. Res., 1979, 12, 111–117 CrossRef CAS.
-
(a) N. J. Turro and J. Mattay, Photochemistry of some deoxybenzoins in micellar solutions. Cage effects, isotope effects, and magnetic field effects, J. Am. Chem. Soc., 1981, 103, 4200–4204 CrossRef CAS;
(b)
N. J. Turro, G. Sidney Cox and M. A. Paczkowski, Photochemistry in Micelles, in Topics in Current Chemistry, Photochemistry and Organic Synthesis, ed. F. L. Boschke, Springer-Verlag, New York, 1985, vol. 129, p. 57 Search PubMed.
-
(a) N. J. Turro and B. Kraeutler, Magnetic field and magnetic isotope effects in organic photochemical reactions. A novel probe of reaction mechanisms and a method for enrichment of magnetic isotopes, Acc. Chem. Res., 1980, 13, 369–377 CrossRef CAS;
(b) N. J. Turro, A. L. Buchachenko and V. F. Tarasov, How Spin Stereochemistry Severely Complicates the Formation of a Carbon–Carbon Bond between Two Reactive Radicals in a Supercage, Acc. Chem. Res., 1995, 28, 69–80 CrossRef CAS;
(c)
K. Lalyanasundaran, in Photochemistry in Microheterogeneous Systems, Academic Press, Inc., Orlando, FL, 1987 Search PubMed.
-
(a) A. K. Singh and T. S. Raghuraman, Photorearrangement of phenyl cinnamates under micellar environment, Tetrahedron Lett., 1985, 26, 4125–4128 CrossRef CAS;
(b) R. Nakagaki, M. Hiramatsu, T. Watanabe, Y. Tanimoto and S. Nagakura, Magnetic isotope and external magnetic field effects upon the photo-Fries rearrangement of 1-naphthyl acetate, J. Phys. Chem., 1985, 89, 3222–3226 CrossRef CAS;
(c) R. Q. Yie, Y. C. Liu and X. G. Lei, The photo-fries rearrangement of α-naphthyl acetate in cyclodextrin and micelle, Res. Chem. Intermed., 1992, 18, 61–69 CrossRef;
(d) B. Suau, G. Torres and M. Valpuesta, The photo-fries rearrangement of 2,5-disubstituted phenyl acetates, Tetrahedron Lett., 1995, 36, 1311–1314 CrossRef;
(e) M. Nowakowska, J. Storsberg, S. Zapotoczny and J. E. Guillet, Studies of the antenna effect in polymer molecules. 28. Photo-Fries rearrangement of 1-naphthyl acetate in aqueous solutions of poly(sodium styrenesulfonate-co-2-vinylfluorene), New J. Chem., 1999, 23, 617–623 RSC;
(f) A. K. Singh and T. S. Raghuraman, Photobehaviour of N-Aryl Amides in Micelle, Synth. Commun., 1986, 16, 485–490 CrossRef CAS;
(g) M. S. Syamala, B. Nageswer Rao and V. Ramamurthy, Modification of Photochemical Reactivity by Cyclodextrin complexation: Product Selectivity in Photo-Fries Rearrangement, Tetrahedron, 1988, 44, 7234–7242 CrossRef CAS;
(h) M. Nasetta, R. H. De Rossi and J. J. Cosa, Influence of Cyclodextrins on the Photo-Fries rearrangement of acetanilide, Can. J. Chem., 1988, 66, 2794–2798 CrossRef.
-
(a) Ch. Felnon and H. Cynamon, Synthesis and Antibacterial Activities of Optically Active Ofloxacin, Antimicrob. Agents Chemother., 1986, 29, 163–164 CrossRef;
(b) K. Drlica and X. Zhao, DNA gyrase, topoisomerase IV, and the 4-quinolones, Microbiol. Mol. Biol. Rev., 1997, 61, 377–392 CAS;
(c)
A. F. Pazkarshii, A. Soldatinkov and A. R. Katritzky, in Heterocyles in life and society, John Wiley and Sons, Chichester, 1997, pp. 147–148 Search PubMed;
(d) D. Edmont, R. Rocher, C. Plisson and J. Chenault, Synthesis and evaluation of quinoline carboxyguanidines as antidiabetic agents, Bioorg. Med. Chem. Lett., 2000, 10, 1831–1834 CrossRef CAS PubMed;
(e) Y. Xia, Z.-Y. Yang, P. Xia, K. F. Bastow, Y. Nakanishi, P. Nampoothiri, E. Hamel, A. Brossi and K.-H. Lee, Antitumor Agents. Part 226:y Synthesis and Cytotoxicity of 2-Phenyl-4-quinolone Acetic Acids and Their Esters, Bioorg. Med. Chem. Lett., 2003, 13, 2891–2893 CrossRef CAS PubMed;
(f) L. A. Mitscher, Bacterial Topoisomerase Inhibitors: Quinolone and Pyridone Antibacterial Agents, Chem. Rev., 2005, 105, 559–592 CrossRef CAS PubMed;
(g) S. Nakamura, M. Kozuka, K. F. Bastow, H. Tokuda, H. Nishino, M. Suzuki, J. Tatsuzaki, S. L. M. Natschke, S.-C. Kuo and K.-H. Lee, Cancer preventive agents, Part 2: Synthesis and evaluation of 2-phenyl-4-quinolone and 9-oxo-9,10-dihydroacridine derivatives as novel antitumor promoters, Bioorg. Med. Chem., 2005, 13, 4396–4401 CrossRef CAS PubMed;
(h) B. d'A. Lucero, C. R. B. Gomes, I. C. Frugulhetti, L. V. Faro, L. Alvarenza, M. C. B. V. Souza, T. M. L. de Souza and V. F. Ferreira, Synthesis and anti-HSV-1 activity of quinolonic acyclovir analogues, Bioorg. Med. Chem. Lett., 2006, 16, 1010–1013 CrossRef CAS PubMed;
(i) C. P. Jones, K. W. Anderson and S. L. Buchwald, Sequential Cu-Catalyzed Amidation-Base-Mediated Camps Cyclization: A Two-Step Synthesis of 2-Aryl-4-quinolones from o-Halophenones, J. Org. Chem., 2007, 72, 7968–7973 CrossRef CAS PubMed;
(j) J. Huang, Y. Chen, A. O. King, M. Dilmeghani, R. D. Larsen, M. M. Faul and A. Mild, One-Pot Synthesis of 4-Quinolones via Sequential Pd-Catalyzed Amidation and Base-Promoted Cyclization, Org. Lett., 2008, 10, 2609–2611 CrossRef CAS PubMed;
(k) T. Zhao and B. Xu, Palladium-Catalyzed Tandem Amination Reaction for the Synthesis of 4-Quinolones, Org. Lett., 2010, 12, 121–215 Search PubMed.
- L. Brinchi, P. di Profio, F. Micheli, R. Germani, G. Savelli and C. A. Bunton, Structure of Micellar head-Groups and the Hydrolysis of Phenyl Chlorofomate. The Role of Perchlorate Ion, Eur. J. Org. Chem., 2001, 1115 CrossRef CAS.
-
(a) L. Sepulveda, E. Lissi and F. H. Quina, Interactions of Neutral Molecules with Ionic Micelles, Adv. Colloid Interface Sci., 1986, 25, 1–57 CrossRef CAS PubMed;
(b) F. H. Quina and E. O. Alonso, Incorporation of Nonionic Solutes into Aqueous Micelles: A Linear Solvation Free Energy Relationship Analysis, J. Phys. Chem., 1995, 99, 11708–11714 CrossRef CAS;
(c) H. Abraham, H. S. Chadha, J. P. Dixon, C. Rafols and C. Treiner, Hydrogen bonding Part 40. Factors that influence the distribution of solutes between water and sodium dodecylsulfate micelles, J. Chem. Soc., Perkin Trans. 2, 1995, 887–894 RSC;
(d) H. Abraham, H. S. Chadha, J. P. Dixon, C. Rafols and C. Treiner, Hydrogen bonding. Part 41.1 Factors that influence the distribution of solutes between water and hexadecylpyridinium chloride micelles, J. Chem. Soc., Perkin Trans. 2, 1997, 19–24 RSC.
- P. Campos Rey, C. Cabaleiro Lago and P. Hervés, Solvolysis of Substituted benzoyl Chlorides in Nonionic and Mixed Micllar Solutions, J. Phys. Chem. B, 2010, 114, 14004–14011 CrossRef CAS PubMed.
-
(a) D. J. Luning Prak, W. I. Jahraus, J. M. Sims and A. H. Roy McArthur, An 1H NMR investigation into the loci of solubilization of 4-nitrotoluene, 2,6-dinitrotoluene, and 2,4,6-trinitrotoluene in nonionic surfactant micelles, Colloids Surf., A, 2011, 375, 12–22 CrossRef CAS;
(b) P. Sabatino, A. Szczygielb, D. Sinnaeveb, M. Hakimhashemia, H. Saveyna, J. C. Martinsb and P. Van der Meerena, NMR study of the influence of pH on phenol sorption in cationic CTAB micellar solutions, Colloids Surf., A, 2010, 370, 42–48 CrossRef CAS;
(c) L. A. Bernardez, Investigation on the locus of solubilization of polycyclic aromatic hydrocarbons in non-ionic surfactant micelles with 1H NMR spectroscopy, Colloids Surf., A, 2008, 324, 71–78 CrossRef CAS;
(d) M. E. N. P. Ribeiro, C. L. de Moura, M. G. S. Vieira, N. V. Gramosa, Ch. Chaubundit, M. C. de Mattos, D. Attwood, S. G. Yeats, S. K. Nixon and N. M. P. A. Ricardo, Solubilisation capacity of Brij surfactants, Int. J. Pharm., 2012, 436, 631–635 CrossRef CAS PubMed;
(e) N. Dharaiyaa, S. Chavdaa, K. Singhb, D. G. Marangonib and P. Bahadura, Spectral and hydrodynamic studies on p-toluidine induced growth in cationic micelle, Spectrochim. Acta, Part A, 2012, 93, 306–312 CrossRef PubMed;
(f) J. C. Eriksson, NMR-Experiments on Solubilization in Soap Micelles, Acta Chem. Scand., 1963, 17, 1478–1481 CrossRef CAS;
(g) J. C. Eriksson and G. Gillberg, NMR-Studies of the Solubilisation of Aromatic Compounds in Cetyltrimethylammonium Bromide Solution. II., Acta Chem. Scand., 1966, 20, 2019–2027 CrossRef CAS;
(h) J. E. Gordon, J. C. Robertson and R. L. Thorne, Medium effects on hydrogen-1 chemical shift of benzene in micellar and nonmicellar aqueous solutions of organic salts, J. Phys. Chem., 1970, 74, 957–961 CrossRef CAS;
(i) F. Podo, A. Ray and G. Nemethy, Structure and hydration of nonionic detergent micelles. High resolution nuclear magnetic resonance study, J. Am. Chem. Soc., 1973, 95, 6164–6171 CrossRef CAS;
(j) X. Gao and T. C. Wong, Studies of the binding and structure of adrenocorticotropin peptides in membrane mimics by NMR spectroscopy and pulsed-field gradient diffusion, Biophys. J., 1998, 74, 1871–1888 CrossRef CAS PubMed;
(k) X. Gao and T. C. Wong, NMR studies of adrenocorticotropin hormone peptides in sodium dodecylsulfate and dodecylphosphocholine micelles: Proline isomerism and interactions of the peptides with micelles, Biopolymers, 2001, 58, 20–32 CrossRef CAS PubMed;
(l) C. Chatterjee and C. Mukhopadhyay, Conformational alteration of bradykinin in presence of GM1 micelle, Biochem. Biophys. Res. Commun., 2004, 315, 866–871 CrossRef CAS PubMed;
(m) C. Chatterjee and C. Mukhopadhyay, Interaction and structural study of kinin peptide bradykinin and ganglioside monosialylated 1 micelle, Biopolymers, 2005, 78, 197–205 CrossRef CAS PubMed;
(n) N. Matsumori, T. Houdai and M. Murata, Conformation and Position of Membrane-Bound Amphotericin B Deduced from NMR in SDS Micelles, J. Org. Chem., 2007, 72, 700–707 CrossRef CAS PubMed;
(o) L. A. Bernardez and S. Ghoshal, Selective Solubilization of Polycyclic Aromatic Hydrocarbons from Multicomponent Nonaqueous-Phase Liquids into Nonionic Surfactant Micelles, Environ. Sci. Technol., 2004, 38, 5878–5887 CrossRef CAS PubMed;
(p) M. S. Goldenberg, L. A. Bruno and E. L. Rennwantz, Determination of Solubilization Sites and Efficiency of Water-Insoluble Agents in Ethylene Oxide-Containing Nonionic Micelles, J. Colloid Interface Sci., 1993, 158, 351–363 CrossRef CAS.
- For examples, see:
(a) T. Mori, Y. Inoue and R. G. Weiss, Enhanced Photodecarboxylation of an Aryl Ester in Polyethylene Films, Org. Lett., 2003, 5, 4661–4664 CrossRef CAS PubMed;
(b) T. Hirano, W. Li, L. Abrams, P. J. Krusic, M. F. Ottaviani and N. J. Turro, Supramolecular Steric Effects as the Means of Making Reactive Carbon Radicals Persistent. Quantitative Characterization of the External Surface of MFI Zeolites through a Persistent Radical Probe and a Langmuir Adsorption Isotherm, J. Org. Chem., 2000, 65, 1319–1330 CrossRef CAS PubMed;
(c) C. Cui and R. G. Weiss, Photo-Fries rearrangements of 2-naphthyl acylates as probes of the size and shape of guest sites afforded by unstretched and stretched low-density polyethylene films. A case of remarkable selectivity, J. Am. Chem. Soc., 1993, 115, 9820–9821 CrossRef CAS.
-
(a) S. M. Bonesi, M. Mesaros, F. M. Cabrerizo, M. A. Ponce, G. Bilmes and R. Erra-Balsells, The photophysiscs of nitrocarbazoles used as UV-MALDI matrices. Comparative spectroscopic and optoacoustic studies of mono- and dinitrocarbazoles, Chem. Phys. Lett., 2007, 446, 49 CrossRef CAS;
(b) A. Cors, S. M. Bonesi and R. Erra-Balsells, Photoreduction of nitroarenes with formic acid in acetonitrile at room temperature, Tetrahedron Lett., 2008, 49, 1555 CrossRef CAS.
Footnote |
† Electronic supplementary information (ESI) available. See DOI: 10.1039/c5pp00349k |
|
This journal is © The Royal Society of Chemistry and Owner Societies 2016 |
Click here to see how this site uses Cookies. View our privacy policy here.