Efficient, versatile and practical palladium-catalyzed highly regioselective ortho-halogenation of azoxybenzenes†
Received
19th October 2015
, Accepted 4th November 2015
First published on 6th November 2015
Abstract
A highly efficient and practical strategy for regio-selective ortho-halogenation (I, Br, Cl) of azoxybenzenes with NXS in the presence of palladium catalysts has been developed in good to excellent yields. The reaction proceeds smoothly and can tolerate a variety of functional groups. Moreover, this chemistry can be applied to substrates in at least a gram scale.
Introduction
Over the past decade, aryl halides have emerged as extremely valuable starting materials for constructing complex skeletons in synthetic elaboration due to their role as precursors for the synthesis of organometallic reagents,1 nucleophilic aromatic substitution2 as well as transition-metal catalyzed cross-coupling reactions.3 Generally, the most common methods for the synthesis of halogenated arenes involve electrophilic aromatic substitution4 and ortho-lithiation followed by a halogen quench,5 which shows many limitations and suffers from several notable disadvantages.6,7b With regard to the development of selective halogenation reactions, the emergence of direct C–H functionalization/halogenation provides an atom-economic strategy,8,9 which produces halogenated aromatic products with high selectivity that would not ordinarily be obtained under traditional conditions. Since the pioneering work of Sanford,7 Shi10 and Yu,11 the groups of Bedford12 and Xu13 illustrated the major importance of these ortho-selective transformations with the assistance of diverse directing groups. However, although significant progress in C–H bond functionalization/halogenation has been achieved, application of this strategy to create carbon–halogen bonds for more diverse structures is still surprisingly underdeveloped (Scheme 1).
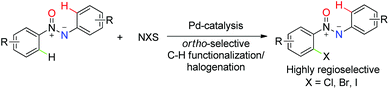 |
| Scheme 1 Pd-catalyzed ortho-halogenation of azoxybenzenes with NXS. | |
Owing to their unique structure and liquid crystalline properties,14 azoxy compounds are able to serve as polymer inhibitors, stabilizers, dyes and key materials in electronic devices.15 Furthermore, it is well known that regioselective control of C–H bond activation in azoxy compounds is an extreme challenge, due to the two different coordinating sites provided by the directing groups16 (N or O). In spite of the development of numerous methods for the synthesis of azoxy compounds,9l,17 exploration in the sterically hindered ortho-functionalization of azoxybenzenes is very limited.18 Recently, Wang18a and ourselves18c,d reported the ortho-acylation of azoxybenzenes, and subsequently a method for the alkenylation of azoxy compounds was established.18b As far as we know, there are no reports in the curet literature on the ortho-halogenation of azoxybenzenes, which represent a profoundly more desirable target as they are synthetically highly important yet this goal is still unmet. Encouraged by our previous research19 and as part of our ongoing interest in C–H activation, herein we describe a novel and efficient approach to the synthesis of a variety of azoxy halides by Pd-catalyzed ortho-C–H bond halogenation employing commercially available electrophilic halogenating reagents—particularly N-bromo-, N-chloro-, and N-iodosuccinimide.
Results and discussion
Our initial investigation was carried out by examining azoxybenzene (1a, 1.0 equiv.) and NBS (1.1 equiv.) in the presence of 10 mol% Pd(OAc)2 in AcOH at 100 °C for 12 h (Table 1, entry 1). To our delight, the ortho-bromo-product was isolated in 87% yield, and further screening of solvents showed no difference in this reaction (Table 1, entries 2–5). Inspired by the previous discovery of the large beneficial effects of Brønsted acids in promoting the electrophilicity of palladium(II) catalysts20 and rendering NBS a more effective source of Br+,21 we attempted to improve the desired product yield. Much to our surprise, this reaction was dramatically affected by the addition of Brønsted acids, and the corresponding yield increased to 85% in DCE with p-toluenesulfonic acid (PTSA) as an additive in contrast to 18% without the acid (Table 1, entry 9). Other Brønsted acids, including acetic acid and trifluoroacetic acid, also proved to be effective. It was found that the reaction temperature was crucial to this transformation, and the best result (97%) was observed at 40 °C (Table 1, entry 13), while increasing or lowering the reaction temperature lowered the efficiency. Subsequently, different Pd catalysts were also investigated. Only Pd(OAc)2 facilitated this procedure with any appreciable conversion. Finally, the optimized reaction conditions for the ortho-C–H halogenation of azoxy compounds were determined to be NBS (1.1 equiv.) with 10 mol% Pd(OAc)2 as the catalyst, 0.5 equiv. of PTSA as additive, and DCE as solvent, at 40 °C under air for 12 h.
Table 1 Optimization of the reaction conditiona,b
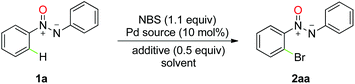
|
Entry |
Catalyst |
Additive |
Solvent |
Yieldb [%] |
All the reactions were carried out in the presence of 0.2 mmol of 1a, 0.22 mmol of NBS and 0.1 mmol of acid (if any) in 1.0 mL of solvents at 100 °C under air condition.
Isolated yields.
At 80 °C.
At 60 °C.
At 40 °C.
At room temperature.
|
1 |
Pd(OAc)2 |
|
AcOH |
87% |
2 |
Pd(OAc)2 |
|
DCE |
18% |
3 |
Pd(OAc)2 |
|
DME |
n.r. |
4 |
Pd(OAc)2 |
|
Toluene |
Trace |
5 |
Pd(OAc)2 |
|
MeCN |
55% |
6 |
Pd(OAc)2 |
AcOH |
MeCN |
56% |
7 |
Pd(OAc)2 |
TsOH·H2O |
MeCN |
58% |
8 |
Pd(OAc)2 |
AcOH |
DCE |
30% |
9 |
Pd(OAc)2 |
TsOH·H2O |
DCE |
85% |
10 |
Pd(OAc)2 |
TFA |
DCE |
69% |
11 |
Pd(OAc)2 |
TsOH·H2O |
DCE |
88%c |
12 |
Pd(OAc)2 |
TsOH·H2O |
DCE |
92%d |
13 |
Pd(OAc)2 |
TsOH·H2O |
DCE |
97%e |
14 |
Pd(OAc)2 |
TsOH·H2O |
DCE |
80%f |
15 |
PdCl2 |
TsOH·H2O |
DCE |
60% |
16 |
Pd(TFA)2 |
TsOH·H2O |
DCE |
62% |
17 |
Pd(PPh3)4 |
TsOH·H2O |
DCE |
57% |
With a reliable protocol in hand, the scope of the ortho-bromination of azoxy compounds was investigated. The results, summarized in Table 2, revealed that the reaction had good compatibility with several functional groups, and good to excellent yields were obtained for most cases. For example, nearly quantitative yields were obtained with methyl group substituted substrates, and an interesting regioselectivity was found with meta-substituted aromatic rings due to the steric hindrance (Table 2, 2ba–2da). It is noteworthy that the reaction can tolerate various halogen groups such as fluoro, chloro and bromo, which afford the desired products in good yields under a slightly higher temperature and could be used for further transformations into other important skeletons (Table 2, 2fa–2ha). However, in the presence of methoxyl, a strongly electron-donating group, the corresponding product was obtained in a slightly lower efficiency along with unidentified by-products (Table 2, 2ea). Moreover, when a trifluoromethyl-substituted azoxy compound was exposed to the reaction conditions, the desired bromination product was observed in moderate yield even at high temperature (Table 2, 2ia). Much to our pleasure, this transformation could also be successfully extended to unsymmetrical azoxybenzenes with excellent yields (Table 2, 2ka–2la), and the desired regioselectivity was obtained thanks to the assistance of the N-atom in the azoxy group (vide infra).
Table 2 Scope of the ortho-bromination of azoxybenzene with NBSa,b
All the reactions were carried out in the presence of 0.2 mmol of 1, 0.22 mmol of NBS and 0.1 mmol of TsOH·H2O in 1.0 mL DCE at 40 °C under air condition.
Isolated yields.
At 80 °C.
At 100 °C.
|
|
To further establish the power of this strategy, we next examined the chlorination and iodination of azoxy compounds under the same reaction conditions. Although moderate yields were obtained with simple replacement of NBS by NCS or NIS under otherwise identical conditions,22 we were pleased to observe that a subtle change in the solvent exerts significant effect on the corresponding ortho-chlorination and iodination reactions. Much to our pleasure, azoxybenzene was found to generate the ortho-chlorinated and ortho-iodinated products in 96% and 95% yields in AcOH, respectively (see ESI† for more details). More importantly, for most of the substrates we employed, moderate to good yields were observed in this reaction and good tolerance to the chemically active functional groups was also revealed (Tables 3 and 4). Interestingly, high selectivity was obtained for the chlorination and iodination reactions when meta-substituted azoxybenzene was involved, which led to the formation of the single regioselective products in excellent yields. It is important to note that this chemistry was successfully extended to polyhalogenated aromatic azoxy compounds in spite of relatively low efficiencies, which are hard to prepare by traditional methods.
Table 3 Scope of the ortho-chlorination of azoxybenzene with NCSa,b
All the reactions were carried out in the presence of 0.2 mmol of 1 and 0.22 mmol of NCS in 1.0 mL AcOH at 100 °C under air condition.
Isolated yields.
At 120 °C.
|
|
Table 4 Scope of the ortho-iodination of azoxybenzene with NISa,b
All the reactions were carried out in the presence of 0.2 mmol of 1 and 0.22 mmol of NIS in 1.0 mL AcOH at 60 °C under air condition.
Isolated yields.
At 80 °C.
At 120 °C.
|
|
A distinctive feature of transition-metal catalyzed C–H functionalization is its limited reacting scale, which is a key restraining factor in the use of many strategies in organic synthesis. For this purpose, the reaction was attempted on a gram scale under the established conditions. We were delighted to observe that 2.48 g (yield 90%) of 2-bromo-azoxybenzene, 1.97 g (yield 85%) of 2-chloro-azoxybenzene and 2.66 g (yield 82%) of 2-iodo-azoxybenzene were obtained with 10 mmol (1.98 g) of azoxybenzene as the reactant and 5% Pd(OAc)2 as the catalyst, which demonstrates the possibility to use this transformation in general organic synthesis.
Based on our experimental results and previous literature,7,9f a possible mechanism was proposed to account for the ortho-halogenation of azoxy compounds, which is depicted in Scheme 2. Initially, a five-membered palladacycle intermediate I is generated with the assistance of the N atom by chelation-directed C–H activation, which is generally considered to be a better coordinating atom than O. Oxidative addition of NXS to the palladacycle leads to the formation of Pd(IV) complex II,7,23 followed by reductive elimination to afford ortho-halogenated azoxy compounds and regenerate active Pd(II) to continue the catalytic cycle.
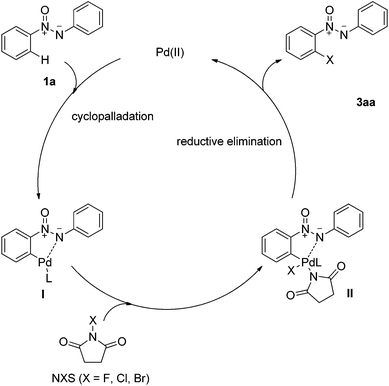 |
| Scheme 2 Proposed reaction mechanism. | |
Conclusions
In summary, we have developed an efficient, versatile and practical catalytic system for the synthesis of ortho-halogenated azoxybenzenes via a regio-selective directing-group-assisted strategy. The potential for gram scale functionalization has been demonstrated and different NXS can be employed as effective halogenating reagents in this transformation. Further investigations on the application of this chemistry and other functionalizations of azoxy compounds are ongoing in our laboratory.
Experimental
General experimental information
All reactions involving air- and moisture-sensitive reagents were carried out under a nitrogen atmosphere. Toluene, DMF, 1,2-dichloroethane, DMSO, 1,4-dioxane and CH3CN were distilled from appropriate drying agents prior to use. All chemicals were purchased from Aldrich and used without further purification. Thin-layer chromatography (TLC) was performed using 60 mesh silica gel plates visualized with short-wavelength UV light (254 nm). Silica gel 60 (230–400 mesh) was used for column chromatography. 1H NMR and 13C NMR spectra were recorded on a Bruker INOVA-400. NMR spectra were recorded on a 400 instrument (400 MHz for 1H and 100 MHz for 13C). Chemical shifts (δ) were measured in ppm relative to TMS δ = 0 for 1H, or to chloroform δ = 77.0 for 13C as internal standard. Data are reported as follows: chemical shift, multiplicity (s = singlet, d = doublet, t = triplet, q = quartet, m = multiplet), coupling constants, J, reported in hertz. Mass data were measured with Thermo Scientific DSQ II mass spectrometer. Azoxybenzenes were prepared from arylamines, according to the literature.1
General catalytic procedure for ortho-bromination of azoxybenzenes with NBS
A mixture of azoxybenzene (39.6 mg, 0.2 mmol, 1.0 equiv.), TsOH·H2O (19.0 mg, 0.1 mmol, 0.5 equiv.), NBS (39.2 mg, 0.22 mmol, 1.1 equiv.) and Pd(OAc)2 (4.5 mg, 0.02 mmol, 10 mol%) in DCE (1 mL) was stirred under air at 40 °C for 12 h followed by cooling. The volatiles were removed under reduced pressure. The contents were subjected to flash chromatography to give the corresponding product (97%) as a pale yellow oil. The purified material was dried under an oil-pump vacuum.
2-(2-Bromophenyl)-2-oxo-1-phenylhydrazin-2-ium-1-ide (2aa).
Pale yellow oil. 1H NMR (400 MHz, CDCl3) δ: 8.17 (d, J = 12.0 Hz, 2 H), 7.71–7.63 (m, 2 H), 7.52–7.42 (m, 4 H), 7.34 (t, J = 8.0 Hz, 1 H). 13C NMR (100 MHz, CDCl3) δ: 149.33, 143.66, 134.03, 130.86, 130.26, 128.77, 128.22, 125.40, 124.81, 115.11. HRMS (ESI) ([M + Na]+) Calcd for C12H9BrN2ONa+: 298.9796, Found [M + Na]+: 298.9744.
2-(2-Bromo-4-methylphenyl)-2-oxo-1-(p-tolyl)hydrazin-2-ium-1-ide (2ba).
Pale yellow oil. 1H NMR (400 MHz, CDCl3) δ: 8.10 (d, J = 8.0 Hz, 2 H), 7.55–7.43 (m, 2 H), 7.29 (d, J = 4 Hz, 2 H), 7.22 (d, J = 8.0 Hz, 1 H), 2.41 (d, J = 8.0 Hz, 6 H). 13C NMR (100 MHz, CDCl3) δ: 147.10, 141.52, 141.45, 140.86, 134.21, 129.32, 128.73, 125.52, 124.55, 114.75, 21.61, 20.93. HRMS (ESI) ([M + Na]+) Calcd for C14H13BrN2ONa+: 327.0103, Found [M + Na]+: 326.9997.
2-(2-Bromo-5-methylphenyl)-2-oxo-1-(m-tolyl)hydrazin-2-ium-1-ide (2ca).
Deep red oil. 1H NMR (400 MHz, CDCl3) δ: 7.95 (d, J = 8.0 Hz,2 H), 7.55 (d, J = 8.0 Hz, 1 H), 7.46 (d, J = 8.0 Hz, 1 H), 7.40–7.36 (m, 1 H), 7.24 (d, J = 8.0 Hz, 1 H), 7.15–7.13 (m, 1 H), 2.42 (s, 3 H), 2.38 (s, 3 H). 13C NMR (100 MHz, CDCl3) δ: 149.11, 143.71, 138.79, 138.57, 133.61, 131.62, 130.98, 128.55, 125.80, 125.29, 122.43, 111.57, 21.43, 20.79. HRMS (ESI) ([M + H]+) Calcd for C14H14BrN2O+: 305.0290, Found [M + H]+: 305.0208.
2-(2-Bromo-6-methylphenyl)-2-oxo-1-(o-tolyl)hydrazin-2-ium-1-ide (2da).
Deep yellow oil. 1H NMR (400 MHz, CDCl3) δ: 8.18–8.15 (m, 1 H), 7.53 (d, J = 4.0 Hz, 1 H), 7.34–7.19 (m, 5 H), 2.44 (s, 3 H), 2.42 (s, 3 H). 13C NMR (100 MHz, CDCl3) δ: 148.80, 141.96, 134.90, 132.67, 130.96, 130.87, 130.15, 129.95, 129.12, 125.90, 121.60, 115.26, 18.48, 17.19. HRMS (ESI) ([M + Na]+) Calcd for C14H13BrN2ONa+: 327.0103, Found [M + Na]+: 327.0035.
2-(2-Bromo-4-methoxyphenyl)-1-(4-methoxyphenyl)-2-oxohydrazin-2-ium-1-ide (2ea).
Pale yellow oil. 1H NMR (400 MHz, CDCl3) δ: 8.28 (d, J = 8.0 Hz, 2 H), 7.62 (d, J = 12.0 Hz, 1 H), 7.19 (d, J = 4.0 Hz, 1 H), 6.98 (d, J = 12.0 Hz, 2 H), 6.94–6.92 (m, 1 H), 3.88 (s, 3 H), 3.85 (s, 3 H). 13C NMR (100 MHz, CDCl3) δ: 160.71, 160.30, 142.97, 137.76, 127.82, 125.91, 118.67, 115.97, 113.70, 113.65, 55.92, 55.51.HRMS (ESI) ([M + Na]+) Calcd for C14H13BrN2O3Na+: 359.0002, Found [M + Na]+: 358.9878.
2-(2-Bromo-4-fluorophenyl)-1-(4-fluorophenyl)-2-oxohydrazin-2-ium-1-ide (2fa).
Pale yellow oil. 1H NMR (400 MHz, CDCl3) δ: 8.25 (dd, J = 8.0 Hz, J = 12.0 Hz, 2 H), 7.68 (dd, J = 4.0 Hz, J = 8.0 Hz, 1 H), 7.45 (dd, J = 4.0 Hz, J = 8.0 Hz, 1 H), 7.17 (t, J = 8.0 Hz, 3 H). 13C NMR (100 MHz, CDCl3) δ: 162.92 (d, J = 252.0 Hz), 162.16 (d, J = 253.0 Hz), 145.82, 140.10 (d, J = 3.0 Hz), 128.01(d, J = 10.0 Hz), 126.36(d, J = 9.0 Hz), 121.25(d, J = 26.0 Hz), 116.23 (d, J = 10.0 Hz), 115.76 (d, J = 23.0 Hz), 115.37 (d, J = 23.0 Hz). HRMS (ESI) ([M + Na]+) Calcd for C12H7BrF2N2ONa+: 334.9602, Found [M + Na]+: 334.9737.
2-(2-Bromo-4-chlorophenyl)-1-(4-chlorophenyl)-2-oxohydrazin-2-ium-1-ide (2ga).
Pale yellow oil. 1H NMR (400 MHz, CDCl3) δ: 8.14 (d, J = 8.0 Hz, 2 H), 7.72 (s, 1 H), 7.62 (d, J = 8.0 Hz, 1 H), 7.47–7.42 (m, 3 H). 13C NMR (100 MHz, CDCl3) δ: 147.68, 141.96, 136.40, 135.90, 133.73, 129.02, 128.42, 126.94, 125.79, 115.97. HRMS (ESI) ([M + Na]+) Calcd for C12H7BrCl2N2ONa+: 366.9017, Found [M + Na]+: 366.9335.
1-(4-Bromophenyl)-2-(2,4-dibromophenyl)-2-oxohydrazin-2-ium-1-ide (2ha).
Pale yellow oil. 1H NMR (400 MHz, CDCl3) δ: 8.06 (d, J = 8.0 Hz, 2 H), 7.88 (d, J = 4.0 Hz, 1 H), 7.63–7.58 (m, 3 H), 7.54 (d, J = 8.0 Hz, 1 H). 13C NMR (100 MHz, CDCl3) δ: 148.09, 142.28, 136.46, 132.02, 131.37, 127.07, 125.98, 124.29, 124.25, 116.13. HRMS (ESI) ([M + Na]+) Calcd for C12H7Br3N2ONa+: 454.8001, Found [M + Na]+: 454.7833.
2-(2-Bromo-4-(trifluoromethyl)phenyl)-2-oxo-1-(4-(trifluoromethyl)phenyl)hydrazin-2-ium-1-ide (2ia).
Pale yellow oil. 1H NMR (400 MHz, CDCl3) δ: 8.22 (d, J = 12.0 Hz, 2 H), 8.01 (s, 1 H), 7.79–7.74 (m, 4 H). 13C NMR (100 MHz, CDCl3) δ: 151.06, 145.59, 133.23 (q, JC–F = 33.0 Hz), 131.62 (q, JC–F = 33.0 Hz), 131.49 (d, JC–F = 4.0 Hz), 126.00 (q, JC–F = 3.0 Hz), 125.57, 125.40, 122.96 (q, JC–F = 118.0 Hz), 122.95 (q, JC–F = 118.0 Hz), 115.86. HRMS (ESI) ([M + Na]+) Calcd for C14H7BrF6N2ONa+: 434.9538, Found [M + Na]+: 434.9381.
2-(2-Bromo-6-methoxyphenyl)-2-oxo-1-phenylhydrazin-2-ium-1-ide (2ka).
Pale yellow oil. 1H NMR (400 MHz, CDCl3) δ: 8.15 (d, J = 8.0 Hz, 2 H), 7.51–7.42 (m, 3 H), 7.26–2.25 (m, 2 H), 7.00 (d, J = 8.0 Hz, 1 H). 13C NMR (100 MHz, CDCl3) δ: 152.88, 143.73, 130.59, 130.14, 128.70, 125.48, 125.38, 124.63, 116.46, 111.49, 56.52. HRMS (ESI) ([M + Na]+) Calcd for C13H11BrN2O2Na+: 328.9902, Found [M + Na]+:328.9902.
2-(2,6-Dibromophenyl)-2-oxo-1-phenylhydrazin-2-ium-1-ide (2la).
White solid. 1H NMR (400 MHz, CDCl3) δ: 8.15 (d, J = 8.0 Hz, 2 H), 7.65 (d, J = 8.0 Hz, 2 H), 7.54–7.46 (m, 3 H), 7.20 (t, J = 8.0 Hz, 1 H). 13C NMR (100 MHz, CDCl3) δ: 143.40, 132.59, 130.92, 130.57, 128.84, 125.42, 116.65. HRMS (ESI) ([M + Na]+) Calcd for C12H8Br2N2ONa+: 376.8901, Found [M + Na]+: 376.8903.
General catalytic procedure for ortho-chlorination/iodination of azoxybenzenes with NCS/NIS
A mixture of azoxybenzene (39.6 mg, 0.2 mmol, 1.0 equiv.), NCS/NIS (0.22 mmol, 1.1 equiv.) and Pd(OAc)2 (4.5 mg, 0.02 mmol, 10 mol%) in AcOH (1 mL) was stirred under air at 60 °C or 100 °C for 12 h as specified in Tables 2 and 3 followed by cooling. The volatiles were removed under reduced pressure. The contents were subjected to flash chromatography to give the corresponding products (96% and 95%) pale yellow oils. The purified materials were dried under an oil-pump vacuum.
2-(2-Chlorophenyl)-2-oxo-1-phenylhydrazin-2-ium-1-ide (3aa).
Deep red oil. 1H NMR (400 MHz, CDCl3) δ: 8.15 (d, J = 8.0 Hz, 2 H), 7.68–7.65 (m, 1 H), 7.53–7.47 (m, 3 H), 7.43–7.38 (m, 3 H). 13C NMR (100 MHz, CDCl3) δ: 147.68, 143.70, 130.89, 130.67, 130.22, 128.74, 127.50, 126.74, 125.38, 124.79. HRMS (ESI) ([M + Na]+) Calcd for C12H9ClN2ONa+: 255.0301, Found [M + Na]+: 255.0212.
2-(2-Chloro-4-methylphenyl)-2-oxo-1-(p-tolyl)hydrazin-2-ium-1-ide (3ba).
Pale yellow oil. 1H NMR (400 MHz, CDCl3) δ: 8.10 (d, J = 8.0 Hz, 2 H), 7.56 (d, J = 8.0 Hz, 1 H), 7.32–7.25 (m, 3 H), 7. 17 (d, J = 8.0 Hz, 1 H), 2.41 (d, J = 4.0 Hz, 6 H). 13C NMR (100 MHz, CDCl3) δ: 145.54, 141.66, 141.31, 140.79, 131.15, 129.31, 128.04, 126.40, 125.53, 124.63, 21.57, 21.00. HRMS (ESI) ([M + Na]+) Calcd for C14H13ClN2ONa+: 283.0614, Found [M + Na]+: 283.0519.
2-(2-Chloro-5-methylphenyl)-2-oxo-1-(m-tolyl)hydrazin-2-ium-1-ide (3ca).
Deep red oil. 1H NMR (400 MHz, CDCl3) δ: 7.97 (d, J = 8.0 Hz, 2 H), 7.48 (d, J = 4.0 Hz, 1 H), 7.40–7.37 (m, 2 H),7.26–7.21 (m, 2 H), 2.43 (s, 3 H), 2.40 (s, 3 H). 13C NMR (100 MHz, CDCl3) δ: 147.34, 143.65, 138.59, 138.07, 131.41, 131.05, 130.51, 128.56, 125.83, 125.15, 123.47, 122.46, 21.45, 20.78. HRMS (ESI) ([M + Na]+) Calcd for C14H13ClN2ONa+: 283.0614, Found [M + Na]+: 283.0512.
2-(2-Chloro-6-methylphenyl)-2-oxo-1-(o-tolyl)hydrazin-2-ium-1-ide (3da).
Deep red oil. 1H NMR (400 MHz, CDCl3) δ: 8.12–8.10 (d, J = 8.0 Hz, 1 H), 7.36–7.27 (m, 5 H), 7.23 (t, J = 8.0 Hz, 1 H), 2.43 (s, 3 H), 2.40 (s, 3 H). 13C NMR (100 MHz, CDCl3) δ: 147.27, 142.10, 134.69, 132.64, 130.86, 129.63, 129.46, 129.03, 127.83, 126.67, 125.94, 121.58, 18.40, 16.96. HRMS (ESI) ([M + Na]+) Calcd for C14H13ClN2ONa+: 283.0614, Found [M + Na]+: 283.0611.
2-(2-Chloro-4-methoxyphenyl)-1-(4-methoxyphenyl)-2-oxohydrazin-2-ium-1-ide (3ea).
Pale yellow oil. 1H NMR (400 MHz, CDCl3) δ: 8.29 (s, 1 H), 8.27 (s, 1 H), 7.64 (d, J = 12.0 Hz, 1 H), 7.00 (d, J = 4.0 Hz, 1 H), 6.99 (d, J = 0.0 Hz, 1 H), 6.97 (t, J = 4.0 Hz, 1 H), 6.89 (dd, J = 4.0 Hz, J = 8.0 Hz, 1 H), 3.88 (s, 3 H), 3.85 (s, 3 H). 13C NMR (100 MHz, CDCl3) δ: 160.70, 160.38, 141.31, 137.81, 127.83, 126.00, 115.63, 113.69, 113.04, 55.90, 55.50. HRMS (ESI) ([M + Na]+) Calcd for C14H13ClN2O3Na+: 315.0512, Found [M + Na]+: 315.0404.
2-(2-Chloro-4-fluorophenyl)-1-(4-fluorophenyl)-2-oxohydrazin-2-ium-1-ide (3fa).
Pale yellow oil. 1H NMR (400 MHz, CDCl3) δ: 8.27–8.24 (m, 2 H), 7.72–7.69 (dd, J = 4.0 Hz, J = 8.0 Hz, 1 H), 7.29–7.26 (m, 1 H), 7.20–7.15 (m, 2 H), 7.15–7.10 (m, 1 H). 13C NMR (100 MHz, CDCl3) δ: 162.90 (d, J = 252.0 Hz), 162.20 (d, J = 253.0 Hz), 144.14, 140.10 (d, J = 3.0 Hz), 128.40 (d, J = 11.0 Hz), 128.03 (d, J = 8.0 Hz), 126.44 (d, J = 10.0 Hz), 118.26 (d, J = 25.0 Hz), 115.75 (d, J = 22.0 Hz), 114.80 (d, J = 22.0 Hz). HRMS (ESI) ([M + Na]+) Calcd for C12H7ClF2N2ONa+: 291.0113, Found [M + Na]+: 291.0110.
1-(4-Chlorophenyl)-2-(2,4-dichlorophenyl)-2-oxohydrazin-2-ium-1-ide (3ga).
Pale yellow oil. 1H NMR (400 MHz, CDCl3) δ: 8.14 (d, J = 12.0 Hz, 2 H), 7.65 (d, J = 8.0 Hz, 1 H), 7.56 (d, J = 4.0 Hz, 1 H), 7.46 (d, J = 8.0 Hz, 2 H), 7.40 (dd, J = 4.0 Hz, J = 8.0 Hz, 1 H). 13C NMR (100 MHz, CDCl3) δ: 146.00, 141.97, 136.36, 135.91, 130.80, 129.01, 127.92, 127.82, 126.96, 125.86. HRMS (ESI) ([M + Na]+) Calcd for C12H7Cl3N2ONa+: 322.9522, Found [M + Na]+: 322.9409.
2-(4-Bromo-2-chlorophenyl)-1-(4-bromophenyl)-2-oxohydrazin-2-ium-1-ide (3ha).
Pale yellow oil. 1H NMR (400 MHz, CDCl3) δ: 8.07 (s, 1 H), 8.05 (s, 1H), 7.71 (s, 1 H), 7.63–7.62 (m, 1 H), 7.61–7.59 (m, 1 H), 7.57–7.55 (m, 2 H). 13C NMR (100 MHz, CDCl3) δ: 13C NMR (101 MHz, CDCl3) δ: 146.46, 142.33, 133.61, 132.02, 130.76, 127.99, 127.10, 126.03, 124.30, 124.14. HRMS (ESI) ([M + Na]+) Calcd for C12H7Br2N2ONa+: 410.8511, Found [M + Na]+: 410.8362.
2-(2-Chloro-4-(trifluoromethyl)phenyl)-2-oxo-1-(4-(trifluoromethyl)phenyl)hydrazin-2-ium-1-ide (3ia).
Pale yellow oil. 1H NMR (400 MHz, CDCl3) δ: 8.22 (d, J = 8.0 Hz, 2 H), 7.84 (d, J = 8.0 Hz, 2 H), 7.77 (d, J = 8.0 Hz, 2 H), 7.72 (dd, J = 4.0 Hz, J = 8.0 Hz, 1 H). 13C NMR (100 MHz, CDCl3) δ: 149.38, 145.61, 133.24 (q, JC–F = 33.0 Hz), 131.67 (q, JC–F = 33.0 Hz), 128.47 (q, JC–F = 4.0 Hz), 127.87, 126.02 (q, JC–F = 4.0 Hz), 125.61, 125.53, 124.84 (q, JC–F = 3.0 Hz), 123.02 (q, JC–F = 105.0 Hz), 123.01 (q, JC–F = 106.0 Hz). HRMS (ESI) ([M + Na]+) Calcd for C14H7ClF6N2ONa+: 391.0049, Found [M + Na]+: 390.9896.
2-(2-Iodophenyl)-2-oxo-1-phenylhydrazin-2-ium-1-ide (4aa).
Pale yellow oil. 1H NMR (400 MHz, CDCl3) δ: 8.17 (t, J = 8.0 Hz, 2 H), 7.94 (d, J = 8.0 Hz, 1 H), 7.65–7.63 (m, 1 H), 7.53–7.42 (m, J = 8.0 Hz, 4 H), 7.21–7.17 (m, 1 H). 13C NMR (100 MHz, CDCl3) δ: 152.60, 143.58, 140.42, 130.99, 130.26, 129.07, 128.77, 125.39, 124.32, 87.97. HRMS (ESI) ([M + Na]+) Calcd for C12H9IN2ONa+: 346.9657, Found [M + Na]+: 346.9650.
2-(2-Iodo-4-methylphenyl)-2-oxo-1-(p-tolyl)hydrazin-2-ium-1-ide (4ba).
Pale yellow oil. 1H NMR (400 MHz, CDCl3) δ: 8.11 (d, J = 8.0 Hz, 2 H), 7.77 (s, 1 H), 7.52 (d, J = 8.0 Hz, 1 H), 7.30 (d, J = 8.0 Hz, 2 H), 7.26 (d, J = 4.0 Hz, 1 H), 2.43 (s, 3 H), 2.38 (s, 3 H). 13C NMR (100 MHz, CDCl3) δ: 150.44, 141.45, 140.79, 140.64, 140.09, 129.56, 129.30, 125.50, 124.02, 87.84, 21.60, 20.67. HRMS (ESI) ([M + Na]+) Calcd for C14H13IN2ONa+: 374.9970, Found [M + Na]+: 374.9964.
2-(2-Iodo-5-methylphenyl)-2-oxo-1-(m-tolyl)hydrazin-2-ium-1-ide (4ca).
Pale yellow oil. 1H NMR (400 MHz, CDCl3) δ: 7.97 (d, J = 4.0 Hz, 2 H), 7.78 (d, J = 8.0 Hz, 1 H), 7.44 (d, J = 0.0 Hz, 1 H), 7.39 (t, J = 8.0 Hz, 1 H), 7.25 (d, J = 4.0 Hz, 1 H), 7.00–6.98 (m, 1H), 2.43 (s, 3 H), 2.38 (s, 3 H). 13C NMR (100 MHz, CDCl3) δ: 152.47, 143.58, 139.97, 139.71, 138.55, 131.91, 130.99, 128.53, 125.77, 124.93, 122.43, 83.74, 21.46, 20.82. HRMS (ESI) ([M + Na]+) Calcd for C14H13IN2ONa+: 374.9970, Found [M + Na]+: 374.9963.
2-(2-Iodo-6-methylphenyl)-2-oxo-1-(o-tolyl)hydrazin-2-ium-1-ide (4da).
Pale yellow oil. 1H NMR (400 MHz, CDCl3) δ: 8.28 (dd, J = 4.0 Hz, J = 8.0 Hz, 1 H), 7.72 (d, J = 8.0 Hz, 1 H), 7.31–7.23 (m, 4 H), 7.06–7.01 (m, 1 H), 2.43 (d, J = 4.0 Hz, 6 H). 13C NMR (100 MHz, CDCl3) δ: 152.23, 141.82, 137.32, 135.18, 132.02, 131.05, 130.84, 130.20, 129.20, 125.81, 121.67, 88.49, 18.60, 17.54. HRMS (ESI) ([M + Na]+) Calcd for C14H13IN2ONa+: 374.9970, Found [M + Na]+: 374.9963.
2-(2-Iodo-4-methoxyphenyl)-1-(4-methoxyphenyl)-2-oxohydrazin-2-ium-1-ide (4ea).
Pale yellow oil. 1H NMR (400 MHz, CDCl3) δ: 8.30 (t, J = 4.0 Hz, 1 H), 8.27 (t, J = 4.0 Hz, 1 H), 7.59 (d, J = 8.0 Hz, 1 H), 7.43 (d, J = 8.0 Hz, 1 H), 7.01–6.99 (m, 1 H), 6.98–6.94 (m, 2 H), 3.86 (s, 3 H), 3.84 (s, 3H). 13C NMR (100 MHz, CDCl3) δ: 160.67, 160.07, 146.25, 137.67, 127.78, 125.27, 125.09, 114.35, 113.69, 88.60, 55.86, 55.49. HRMS (ESI) ([M + Na]+) Calcd for C14H13IN2O3Na+: 406.9869, Found [M + Na]+: 406.9860.
2-(4-Fluoro-2-iodophenyl)-1-(4-fluorophenyl)-2-oxohydrazin-2-ium-1-ide (4fa).
Pale yellow oil. 1H NMR (400 MHz, CDCl3) δ: 8.27–8.23 (m, 2 H), 7.68–7.63 (m, 2 H), 7.22–7.16 (m, 3 H). 13C NMR (100 MHz, CDCl3) δ: 162.82 (d, J = 252.0 Hz), 161.79 (d, J = 257.0 Hz), 149.00, 139.96 (d, J = 3.0 Hz), 127.95 (d, J = 8.0 Hz), 127.32 (d, J = 25.0 Hz), 125.68 (d, J = 10.0 Hz), 116.04 (d, J = 23.0 Hz), 115.72 (d, J = 23.0 Hz), 88.35 (d, J = 9.0 Hz). HRMS (ESI) ([M + Na]+) Calcd for C12H7F2IN2ONa+: 382.9464, Found [M + Na]+: 382.9326.
2-(4-Chloro-2-iodophenyl)-1-(4-chlorophenyl)-2-oxohydrazin-2-ium-1-ide (4ga).
Pale yellow oil. 1H NMR (400 MHz, CDCl3) δ: 8.15 (d, J = 8.0 Hz, 2 H), 7.96 (d, J = 2.0 Hz, 1 H), 7.59 (d, J = 8.0 Hz, 1 H), 7.47 (d, J = 8.0 Hz, 3 H). 13C NMR (100 MHz, CDCl3) δ: 151.01, 141.94, 139.93, 136.33, 135.89, 129.19, 129.03, 126.94, 125.19, 88.46. HRMS (ESI) ([M + Na]+) Calcd for C12H7Cl2IN2ONa+: 414.8878, Found [M + Na]+: 414.8710.
2-(4-Bromo-2-iodophenyl)-1-(4-bromophenyl)-2-oxohydrazin-2-ium-1-ide (4ha).
Pale yellow oil. 1H NMR (400 MHz, CDCl3) δ: 8.11 (s, 1 H), 8.06 (d, J = 8.0 Hz, 2 H), 7.63–7.60 (m, 3 H), 7.52 (d, J = 8.0 Hz, 1 H). 13C NMR (100 MHz, CDCl3) δ: 151.40, 142.56, 142.24, 132.14, 132.02, 127.07, 125.43, 124.38, 124.27, 88.87. HRMS (ESI) ([M + Na]+) Calcd for C12H7Br2IN2ONa+: 502.7867, Found [M + Na]+: 502.7859.
2-(2-Iodo-4-(trifluoromethyl)phenyl)-2-oxo-1-(4-(trifluoromethyl)phenyl)hydrazin-2-ium-1-ide (4ia).
Pale yellow oil. 1H NMR (400 MHz, CDCl3) δ: 8.22 (d, J = 8.0 Hz, 3 H), 7.80–7.75 (m, 4 H). 13C NMR (100 MHz, CDCl3) δ: 154.44, 145.55, 137.78 (d, JC–F = 4.0 Hz), 133.12 (q, JC–F = 34.0 Hz), 131.62 (q, JC–F = 32.0 Hz), 126.42 (q, JC–F = 3.0 Hz), 126.03 (q, JC–F = 4.0 Hz), 125.58, 124.72, 122.84 (q, JC–F = 140.0 Hz), 122.83 (q, JC–F = 142.0 Hz), 88.20. HRMS (ESI) ([M + Na]+) Calcd for C14H7F6IN2ONa+: 482.9405, Found [M + Na]+: 482.9388.
Acknowledgements
We are grateful to the Northwest University (NF14020), Education Department of Shaanxi Provincial Government (14JK1735), the National Natural Science Foundation of China (21402071) and National Found for Fostering Talents of Basic Science (NFFTBS-J1103311, J1210057) for financial support.
Notes and references
-
(a) N. Sotomayor and E. Lete, Curr. Org. Chem., 2003, 7, 275 CrossRef CAS;
(b)
Handbook of Grignard Reagents, ed. G. S. Silverman and P. E. Rakita, Dekker, New York, 1996 Search PubMed.
-
Organic Reaction Mechanism, ed. M. R. Crampton and A. J. Knipe, Wiley, New York, 2007 Search PubMed.
-
(a)
Metal Catalyzed Cross Coupling Reactions, ed. F. Diederich and P. J. Stang, Wiley-VCH, Weinheim, 1998 Search PubMed;
(b)
A. de Meijere and F. Diederich, Metal-Catalyzed Cross-Coupling Reactions, Wiley-VCH Verlag GmbH & Co. KGaA, Weinheim, Germany, 2004 Search PubMed;
(c) K. C. Nicolaou, P. G. Bulger and D. Sarlah, Angew. Chem., Int. Ed., 2005, 44, 4442 CrossRef CAS PubMed;
(d) J. P. Corbet and G. Mignani, Chem. Rev., 2006, 106, 2651 CrossRef CAS PubMed;
(e) L. C. Campeau and K. Fagnou, Chem. Commun., 2006, 1253 RSC;
(f) L. X. Yin and J. Liebscher, Chem. Rev., 2007, 107, 133 CrossRef CAS PubMed;
(g) G. P. McGlacken and I. J. S. Fairlamb, Eur. J. Org. Chem., 2009, 4011 CrossRef CAS;
(h) I. P. Beletskaya and A. V. Cheprakov, Coord. Chem. Rev., 2004, 248, 2337 CrossRef CAS;
(i) S. V. Ley and A. W. Thomas, Angew. Chem., Int. Ed., 2003, 42, 5400 CrossRef CAS PubMed.
-
(a)
R. Taylor, Electrophilic Aromatic Substitution, John Wiley, New York, 1990 Search PubMed;
(b)
P. B. De la Mare, Electrophilic Halogenation, Cambridge University Press, Cambridge, 1976, ch. 5 Search PubMed;
(c) E. B. Merkushev, Synthesis, 1988, 923 CrossRef CAS.
- V. Snieckus, Chem. Rev., 1990, 90, 879 CrossRef CAS.
-
(a) Y. Zhang, K. Shibatomi and H. Yamamoto, Synlett, 2005, 2837 CAS and references therein;
(b) G. K. S. Prakash, T. Mathew, D. Hoole, P. M. Esteves, Q. Wang, G. Rasul and G. A. Olah, J. Am. Chem. Soc., 2004, 126, 15770 CrossRef CAS PubMed;
(c) K. Tanemura, T. Suzuki, Y. Nishida, K. Satsumabayashi and T. Horaguchi, Chem. Lett., 2003, 32, 932 CrossRef CAS;
(d) H. Firouzabadi, N. Iranpoor and M. Shiri, Tetrahedron Lett., 2003, 44, 8781 CrossRef CAS;
(e) P. V. Vyas, A. K. Bhatt, G. Ramachandraiah and A. V. Bedekar, Tetrahedron Lett., 2003, 44, 4085 CrossRef CAS;
(f) K. Lee, H. K. Cho and C. Song, Bull. Korean Chem. Soc., 2002, 23, 773 CrossRef CAS;
(g) J. Iskra, S. Stavber and M. Zupan, Synthesis, 2004, 1869 CrossRef CAS;
(h) G. K. Dewkar, S. V. Narina and A. Sudalai, Org. Lett., 2003, 5, 4501 CrossRef CAS PubMed;
(i) B. V. Tamhankar, U. V. Desai, R. B. Mane, P. P. Wadgaonkar and A. V. Bedekar, Synth. Commun., 2001, 31, 2021 CrossRef CAS;
(j) D. Roche, K. Prasad, O. Repic and T. J. Blacklock, Tetrahedron Lett., 2000, 41, 2083 CrossRef CAS;
(k) D. C. Braddock, G. Cansell and S. A. Hermitage, Synlett, 2004, 461 CrossRef CAS;
(l) P. A. Evans and T. A. Brandt, J. Org. Chem., 1997, 62, 5321 CrossRef CAS.
-
(a) D. Kalyani, A. R. Dick, W. Q. Anani and M. S. Sanford, Tetrahedron, 2006, 62, 11483 CrossRef CAS;
(b) D. Kalyani, A. R. Dick, W. Q. Anani and M. S. Sanford, Org. Lett., 2006, 8, 2523 CrossRef CAS PubMed;
(c) A. R. Dick, K. L. Hull and M. S. Sanford, J. Am. Chem. Soc., 2004, 126, 2300 CrossRef CAS PubMed;
(d) S. R. Whitfield and M. S. Sanford, J. Am. Chem. Soc., 2007, 129, 15142 CrossRef CAS PubMed;
(e) S. R. Whitfield and M. S. Sanford, Organometallics, 2008, 27, 1683 CrossRef CAS.
- For selected reviews, see:
(a)
G. Dyker, Handbook of C-H Transformations: Applications in Organic Synthesis, Wiley-VCH, Weinheim, 2005 Search PubMed;
(b)
Activation and Functionalization of C-H Bond, ed. K. I. Goldberg and A. S. Goldman, ACS Symposium Series 885, American Chemical Society, Washington, DC, 2004 Search PubMed;
(c) R. Giri, B.-F. Shi, K. M. Engle, N. Maugel and J.-Q. Yu, Chem. Soc. Rev., 2009, 38, 3242 RSC;
(d)
J.-Q. Yu and Z.-J. Shi, C-H Activation, Springer, Berlin, Germany, 2010 Search PubMed.
- Selected recent examples:
(a) R. Giri, X. Chen, X.-S. Hao, J.-J. Li, J. Liang, Z.-P. Fan and J.-Q. Yu, Tetrahedron: Asymmetry, 2005, 16, 3502 CrossRef CAS;
(b) K. L. Hull, W. Q. Anani and M. S. Sanford, J. Am. Chem. Soc., 2006, 128, 7134 CrossRef CAS PubMed;
(c) J.-J. Li, R. Giri and J.-Q. Yu, Tetrahedron, 2008, 64, 6979 CrossRef CAS;
(d) X. Zhao, E. Dimitrijevic and V. M. Dong, J. Am. Chem. Soc., 2009, 131, 3466 CrossRef CAS PubMed;
(e) F. Kakiuchi, T. Kochi, H. Mutsutani, N. Kobayashi, S. Urano, M. Sato, S. Nishiyama and T. Tanabe, J. Am. Chem. Soc., 2009, 131, 11310 CrossRef CAS PubMed;
(f) E. Dubost, C. Fossey, T. Cailly, S. Rault and F. Fabis, J. Org. Chem., 2011, 76, 6414 CrossRef CAS PubMed;
(g) N. Schröder, J. Wencel-Delord and F. Glorius, J. Am. Chem. Soc., 2012, 134, 8298 CrossRef PubMed;
(h) A. John and K. M. Nicholas, J. Org. Chem., 2012, 77, 5600 CrossRef CAS PubMed;
(i) D. R. Fahey, J. Organomet. Chem., 1971, 27, 283 CrossRef CAS;
(j) Y. Lian, R. G. Bergman, L. D. Lavis and J. A. Ellman, J. Am. Chem. Soc., 2013, 135, 7122 CrossRef CAS PubMed;
(k) H. Li, P. Li and L. Wang, Org. Lett., 2013, 15, 620 CrossRef CAS PubMed;
(l) X.-T. Ma and S.-K. Tian, Adv. Synth. Catal., 2013, 355, 337 CAS.
- X. Wan, Z. Ma, B. Li, K. Zhang, S. Cao, S. Zhang and Z. Shi, J. Am. Chem. Soc., 2006, 128, 7416 CrossRef CAS PubMed.
-
(a) T.-S. Mei, D.-H. Wang and J.-Q. Yu, Org. Lett., 2010, 12, 3140 CrossRef CAS PubMed;
(b) J.-J. Li, T.-S. Mei and J.-Q. Yu, Angew. Chem., Int. Ed., 2008, 47, 6452 CrossRef CAS PubMed;
(c) T.-S. Mei, R. Giri, N. Maugel and J.-Q. Yu, Angew. Chem., Int. Ed., 2008, 47, 5215 CrossRef CAS PubMed;
(d) R. Giri, X. Chen and J.-Q. Yu, Angew. Chem., Int. Ed., 2005, 44, 2112 CrossRef CAS PubMed.
-
(a) R. B. Bedford, J. U. Engelhart, M. F. Haddow, C. J. Mitchell and R. L. Webster, Dalton Trans., 2010, 39, 10464 RSC;
(b) R. B. Bedford, M. F. Haddow, C. J. Mitchell and R. L. Webster, Angew. Chem., Int. Ed., 2011, 50, 5524 CrossRef CAS PubMed.
- B. Song, X. Zheng, J. Mo and B. Xu, Adv. Synth. Catal., 2010, 352, 329 CrossRef CAS.
- T. Ikeda and O. Tsu, Science, 1995, 268, 1873 CAS.
-
(a) J. M. Huang, J. F. Kuo and C. Y. Chen, J. Appl. Polym. Sci., 1995, 55, 1217 CrossRef CAS;
(b) D. Campbell, L. R. Dix and P. Rostron, Dyes Pigm., 1995, 29, 77 CrossRef CAS.
-
(a) S. D. Kahn, W. D. Hehre and J. A. Pople, J. Am. Chem. Soc., 1987, 109, 1871 CrossRef CAS;
(b) H. Basch and T. Hoz, Mol. Phys., 1997, 91, 789 CrossRef CAS.
- For selected examples, see:
(a) Z. Hou, Y. Fujiware and H. Taniguchi, J. Org. Chem., 1988, 53, 3118 CrossRef CAS;
(b) N. Sakai, K. Fuji, S. Nabeshima, R. Ikeda and T. Konakahara, Chem. Commun., 2010, 46, 3173 RSC;
(c) Although regioselective halogenation of azobenzenes has been developed (9l), the azoxy compounds could not be afforded and/or isolated purely by oxidation of corresponding aromatic azo compounds.
-
(a) H. J. Li, P. H. Li, Q. Zhao and L. Wang, Chem. Commun., 2013, 49, 9170 RSC;
(b) H. J. Li, X. Y. Xie and L. Wang, Chem. Commun., 2014, 50, 4218 RSC;
(c) M. Sun, L.-K. Hou, X.-X. Chen, X.-J. Yang, W. Sun and Y.-S. Zang, Adv. Synth. Catal., 2014, 356, 3789 CrossRef CAS;
(d) L. Hou, X. Chen, S. Li, S. Cai, Y. Zhao, M. Sun and X.-J. Yang, Org. Biomol. Chem., 2015, 13, 4160 RSC;
(e) M. Yi, X. Cui, C. Zhu, C. Pi, W. Zhu and Y. Wu, Asian J. Org. Chem., 2015, 4, 38 CrossRef CAS.
-
(a) M. Sun, H. Y. Zhang, Q. Han, K. Yang and S. D. Yang, Chem. – Eur. J., 2011, 17, 9566 CrossRef CAS PubMed;
(b) M. Sun, Y. N. Ma, Y. M. Li, Q. P. Tian and S. D. Yang, Tetrahedron Lett., 2013, 54, 5091 CrossRef CAS;
(c) Y. M. Li, M. Sun, H. L. Wang, Q. P. Tian and S. D. Yang, Angew. Chem., Int. Ed., 2013, 52, 3972 CrossRef CAS PubMed.
- For a review, see: J. Wencel-Delord, T. Dröge, F. Liu and F. Glorius, Chem. Soc. Rev., 2011, 40, 4740 RSC.
- T. Oberhauser, J. Org. Chem., 1997, 62, 4504 CrossRef CAS PubMed.
- The corresponding chlorinated/iodinated products were isolated in 44% and 31% yields, respectively. See ESI† for more details.
- For a review on organopalladium(IV) chemistry, see: L.-M. Xu, B.-J. Li, Z. Yang and Z.-J. Shi, Chem. Soc. Rev., 2010, 39, 712 RSC.
Footnote |
† Electronic supplementary information (ESI) available: Details experimental procedures, complete characterization data, copies of NMR spectra for all crucial compounds. CCDC 1054759. For ESI and crystallographic data in CIF or other electronic format see DOI: 10.1039/c5ob02153g |
|
This journal is © The Royal Society of Chemistry 2016 |
Click here to see how this site uses Cookies. View our privacy policy here.