DOI:
10.1039/C5NJ01536G
(Paper)
New J. Chem., 2016,
40, 764-769
Antibacterial activity of vinyl imidazole(VI) functionalized silica polymer nanocomposites (SBA/VI) against Gram negative and Gram positive bacteria
Received
(in Montpellier, France)
17th June 2015
, Accepted 10th November 2015
First published on 16th November 2015
Abstract
Vinyl imidazole functionalized ordered mesoporous silica (SBA/VI) were synthesized by in situ radical polymerization of vinyl imidazole monomers and characterized for antibacterial activity against Gram positive and Gram negative bacteria. The results showed an increasing trend with the concentration of vinyl imidazole(VI) and maximum antibacterial activity could be achieved with SBA/VI55 nanocomposites. The possible explanation for higher antibacterial activity is also explained.
Introduction
In the current scenario, nanocomposites derived from porous templates are advantageous in the field of materials and biosciences because of their improved antifungal, antimicrobial, anti-inflammatory and anticarcinogenic activities. Ordered mesoporous silica materials exhibit remarkable advantages because of their high surface area, large pore volume, tuneable pore diameters and flexibility to incorporate different organic–inorganic moieties for various applications.1–5 Moreover, they can be modified in such a way that their effective concentration may focus only on targeted cells thereby reducing the chances of side effects and drug dose by improving the pharmacokinetics.6–9 These structural characteristics enhance their thermal and chemical stability making them biocompatible for drug delivery, antibiotics, sensing and catalysis etc.10–13 Nowadays nanobiotechnology is blooming worldwide due to the specific size and the shape of its functional activities that largely depend upon the particle size, morphology and concentration.7 Mixed metal oxide nanoparticles, metal nanoparticles and silver incorporated SBA-15 materials have been reported for their antibacterial activity.6,13–15 One of the important organic ligands, namely, vinyl imidazole(VI) is known to exhibit antibacterial, antifungal and other important biological activities. Different materials such as poly(N-imidazole) grafted chitosan, imidazole-imidazolium containing polymers, poly(3-hydroxyoctanoate) grafted vinyl imidazole etc., have been studied for antibacterial activity.16–18 The cleavage of RNA has also been reported using the imidazole polymer.19 Still, in spite of the chemical and thermal stability of the imidazole ring, it undergoes various aromatic transformations to achieve stable configuration. Therefore, the polymers or heterogeneous systems containing the vinyl imidazole ligand are strongly desired for improved and cost effective biological applications. Many researchers have reported the polymerization of polyanilines, methyl methacrylate and semiconducting polymers into different mesoporous channels. In all of these studies, the polymers filled the entire volume of the silica mesopores, resulting into nonporous materials in most of the cases.20–22 In our continuing efforts, we have reported various functionalized ordered silica polymer nanocomposites for catalytic and adsorption applications.23–25 However, according to the best of our knowledge, no report is available in the literature for the synthesis and characterization of vinyl imidazole functionalized ordered mesoporous silica polymer nanocomposites (SBA/VI) by in situ radical polymerization of vinyl monomers for antibacterial applications. SBA-15 has been chosen as a matrix because of its high surface area, tunable pore size and pore volume and it can be modified and thus applicable for biological studies.26,27 The endeavour of selecting vinyl imidazole along with styrene and divinyl benzene is to generate hydrophobicity on a highly hydrophilic mesoporous silica framework that may provide significant interactions for antimicrobial, anti inflammatory and anticancer activities. Moreover, vinyl imidazole, being polar, ionisable and biodegradable, does not have adverse affects on human health.18,28 Therefore, in the present investigation, we report antibacterial activity of SBA/VI nanocomposites against Gram positive – Bacillus subtilis (B. subtilis) (S. mutans), Staphylococcus aureus (S. aureus) and Gram negative – Escherichia coli (E. coli), Salmonella typhi (S. typhi), Pseudomonas aeruginosa (P. aeruginosa) bacteria. The selection of these bacteria was made on the basis of their pathogenic nature. Bacillus subtilis (B. subtilis) and Escherichia coli (E. coli) can cause food poisoning.29,30Streptococcus mutans (S. mutans) found in oral cavity and can cause tooth decay.31Staphylococcus aureus (S. aureus) leads to skin infection, respiratory disease and food poisoning.32Salmonella typhi (S. typhi) is responsible for typhoid fever33 and Pseudomonas aeruginosa (P. aeruginosa) generally creates nosocomial infection, pneumonia and urinary tract infections.34
Materials and methods
Synthesis of SBA-15
All the chemicals were procured from Sigma Aldrich and used without further purification. The synthesis of SBA-15 was done using Pluronic (P123) (EO20PO70EO20, MW = 5800, Aldrich) and TEOS as the surfactant and silica source respectively.35 The synthesis of SBA-15 takes place maintaining the molar ratio of P123
:
H2O
:
HCl
:
TEOS as 1
:
5562.9
:
86.29
:
42.51. Thus 6 g of P123 was dissolved in 100 g of distilled water and 11.6 g of conc. HCl (35%) under continuous stirring. After 6 h, 14.6 g of TEOS (ACROS, 98%) was added at 35 °C under continuous stirring for 24 h. Subsequently the mixture was kept for hydrothermal treatment for 24 h at 100 °C in a closed polypropylene bottle. After filtration, the solid product was dried at 80 °C, further calcined at 550 °C for 6 h to remove the template.
Synthesis of SBA/VI nanocomposites
The synthesis methodology of surface modified SBA/VI involves the impregnation of vinyl monomers (styrene & VI), the cross linker (divinyl benzene) and the radical initiator, a,a′-azoisobutyronitrile (AIBN), into the SBA-15 mesopore walls and equilibrated under reduced pressure to achieve a uniform distribution similar to our previous report.26,27,36Table 1 shows the amount of different vinyl monomers, cross- linker and radical initiator used to synthesize SBA/VI nanocomposites. After impregnating the solution, the samples were heated to 40 °C to remove dichloromethane and subjected to a freeze–vacuum–thaw cycle to remove the residual solvent and air. The samples were sealed in a pyrex tube and subjected to control temperature programming for polymerization. The temperature scheme follows 45 °C for 24 h, 60 °C for 4 h, 100 °C, 120 °C and 150 °C for 1 h. The samples were named as SBA/VIxy, where x, y represent the % of styrene and vinyl imidazole. The synthesized samples were characterized by using standard characterization techniques. Powder X-Ray Diffraction (PXRD) patterns were recorded on a Hecus X-Ray Systems S3 Model using Cu Kα radiation (k = 1.5404 A) from 0–50. Nitrogen adsorption–desorption isotherms were measured by using a Micromeritics ASAP 2020 analyzer at −196 °C. Scanning electron microscopy (SEM) was recorded on a Hitachi Model S-520/JEOL JSM at at 10 kV accelerating voltage with different magnification. Elemental analysis was done using CHN analysis.
Table 1 Concentration of different monomers for the synthesis of SBA/VI nanocomposites (SBA-15 = 1 g with 30 wt% of polymers)
Sample |
Sty : VI (%) |
Sty (g) |
VI (g) |
DVB (g) |
AIBN (g) |
SBA/VI91 |
90 : 10 |
0.20 |
0.02 |
0.07 |
0.02 |
SBA/VI82 |
80 : 20 |
0.18 |
0.04 |
0.07 |
0.02 |
SBA/VI73 |
70 : 30 |
0.16 |
0.06 |
0.07 |
0.02 |
SBA/VI64 |
60 : 40 |
0.14 |
0.08 |
0.07 |
0.02 |
SBA/VI55 |
50 : 50 |
0.12 |
0.10 |
0.07 |
0.02 |
Antimicrobial activity studies via a disk diffusion method
The antimicrobial activity of SBA/VI nanocomposites against Gram positive and Gram negative was studied using a disk diffusion method. The pure culture of organisms B. subtilis (ATCC 2063), S. mutans (MTCC 497), S. aureus (ATCC 6538), E. coli (ATCC 2065), S. typhi (ATCC 6539), P. aeruginosa (MTCC 741) was subcultured in nutrient broth at 35 °C ± 2 °C in an incubator for 24 h. For antimicrobial activity studies, 100 μL bacterial inoculums of each fresh bacteria culture (having 106 colony-forming units (CFU) mL−1) were spread on a nutrient agar plate with the help of a sterile glass-rod spreader. The impregnated disk (a sterile filter disk of 6 mm diameter) with SBA-15 and different concentrations of SBA/VI nanocomposites was placed on a nutrient agar plate containing different bacterial cultures. Control experiments were also carried out in the presence of known standard antibiotic Ofloxacin. After overnight incubation at 35° ± 2 °C, the zone of inhibition was measured in millimetre (mm).
Determination of minimum inhibitory concentration (MIC)
The MIC of SBA/VI nanocomposites was determined by the broth dilution method.37 10 ml of nutrient broth with different concentration of the samples (50–1000 μg ml−1) was prepared. 100 μl of Gram negative and Gram positive bacterial suspension of 106 CFU ml−1 was inoculated in each set and incubated at 37 °C. The optical density was measured periodically at 600 nm.
Results and discussion
Characterization of SBA/VI nanocomposites
The PXRD pattern of the samples (Fig. 1) showed characteristic peaks at (100), (110) and (200) planes similar to the SBA-15 material.35 The peaks can be indexed to reflections comprising of two dimensional hexagonal p6mm symmetry indicating that the ordered mesoporous structures were retained in all the samples. The intensity of the peaks decreased with an increase in the concentration of vinyl imidazole indicating that vinyl imidazole has been incorporated uniformly inside the mesopores. However, the long range ordering of the structure was slightly disturbed for SBA/VI55 nanocomposites because of the excess strain generated with increased concentrations of the monomers.
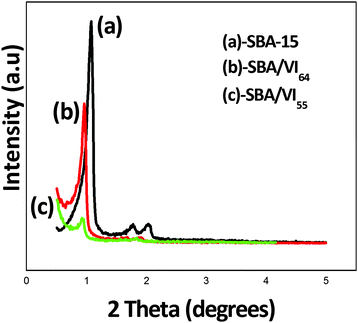 |
| Fig. 1 PXRD pattern of (a) SBA-15, (b) SBA/VI64 and (c) SBA/VI55 nanocomposites. | |
N2-adsorption-desorption isotherms of all the samples (Fig. 2) showed a Type IV adsorption isotherm similar to SBA-15 according to IUPAC classification with a H1 hysteresis loop relatively at higher pressure (p/p0) in the range of 0.4–0.5 indicating the retention of mesoporosity even after the functionalization of vinyl monomers within the mesoporous framework. Total surface area, pore size and pore volume decreased with an increase in the concentration of the monomers further confirming the incorporation of monomers inside the mesopores of the silica surface.
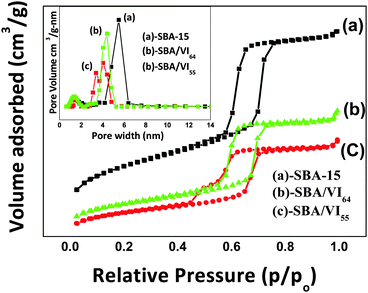 |
| Fig. 2 Nitrogen adsorption–desorption isotherms and pore size distribution (the inset) of (a) SBA-15, (b) SBA/VI64 and (c) SBA/VI55 nanocomposites. | |
The percentages of C, H and N contents are in agreement with the theoretically calculated values. All the structural parameters are shown in Table 2. SEM images (Fig. 3) showed rod type geometry of all the samples. The surface morphology showed slight agglomeration and distortion with an increase in the concentration of VI.
Table 2 Structural parameters of SBA/VI nanocomposites
Materials |
Surface area (m2 g−1) |
Pore volume (cm3 g−1) |
Pore size (nm) |
Theoretical value |
Experimental value |
C (%) |
H (%) |
N (%) |
C (%) |
H (%) |
N (%) |
SBA-15 |
653 |
0.93 |
5.4 |
— |
— |
— |
— |
— |
— |
SBA/VI64 |
304 |
0.53 |
4.4 |
5.4 |
0.6 |
2.7 |
5.2 |
0.5 |
2.4 |
SBA/VI55 |
269 |
0.41 |
4.0 |
6.7 |
0.7 |
3.4 |
6.5 |
0.6 |
3.0 |
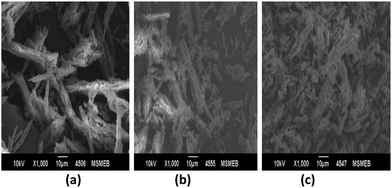 |
| Fig. 3 SEM images of (a) SBA-15 (b) SBA/VI64 and (c) SBA/VI55 nanocomposites. | |
Antimicrobial activity studies
Screening of all the samples for antibacterial activity against Gram positive and Gram negative bacteria showed that the zone of inhibition increased with an increase in the concentration of VI and the maximum inhibition of bacterial growth was obtained with SBA/VI55 nanocomposites under our experimental conditions based on the MIC value (Fig. 5 and Table 3). Therefore, SBA/VI64 and SBA/VI55 were selected for further activity studies. (Fig. 4) showed the zone of inhibition against Gram positive and Gram negative bacteria with different concentrations of SBA/VI64 and SBA/VI55 nanocomposites. No antibacterial activity was observed on pure SBA-15 irrespective of high structural characteristics. This means that the essential activity is due to the vinyl imidazole and the accessibility of vinyl imidazole present on the surface with the bacteria. Therefore, the more the surface area the better will be the dispersion of the ligand with higher concentration. Hence, we believe that the antibacterial activity is controlled by vinyl imidazole along with the surface properties. A closer look at the results (Table 3) indicated that the antibacterial activity against Gram positive is more than the Gram negative. These results are better than the previously reported results on the poly(N-vinylimidazole) grafted macromolecules. The zone of inhibition of SBA/VI55 for Gram negative and Gram positive was upto 20 mm and 27 mm respectively compared to 14 mm and 17 mm on poly(N-vinylimidazole) grafted macromolecules.16 All these results concluded that the antibacterial activity is controlled synergistically by the surface properties of SBA-15 along with the ligand. These results are quite beneficial in terms of low requirements of the sample, biocompatibility and environmental friendly synthesis conditions.
Table 3 Zone of inhibition and MIC values of SBA/VI64 and SBA/VI55 against different Gram negative and Gram positive bacteria
Bacteria |
SBA/VI64 |
SBA/VI55 |
Zone of inhibition (mm) |
MIC value (μg ml−1) |
Zone of inhibition (mm) |
MIC value (μg ml−1) |
B. subtilis
|
11 |
800 ± 2 |
27 |
350 ± 3 |
S. aureus
|
7 |
850 ± 1 |
27 |
400 ± 4 |
S. mutans
|
12 |
900 ± 1 |
25 |
320 ± 0.5 |
P. aeruginosa
|
10 |
900 ± 4 |
20 |
450 ± 2 |
E. coli
|
10 |
950 ± 0.5 |
14 |
450 ± 1 |
S. typhi
|
11 |
1000 ± 1 |
16 |
500 ± 1.5 |
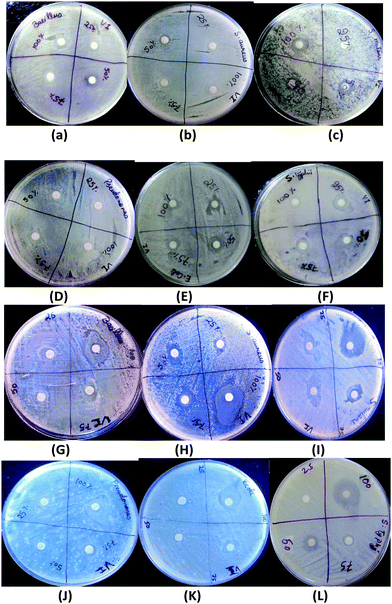 |
| Fig. 4 Zone of inhibition by different concentrations of SBA-15/VI64 against (A) B. subtilis (B) S.aureus (C) S. mutans (D) P. aeruginosa (E) E. coli (F) S. typhi and SBA-15/VI55 against against (G) B. subtilis (H) S. aureus (I) S. mutans (J) P. aeruginosa (K) E. coli (L) S. typhi. | |
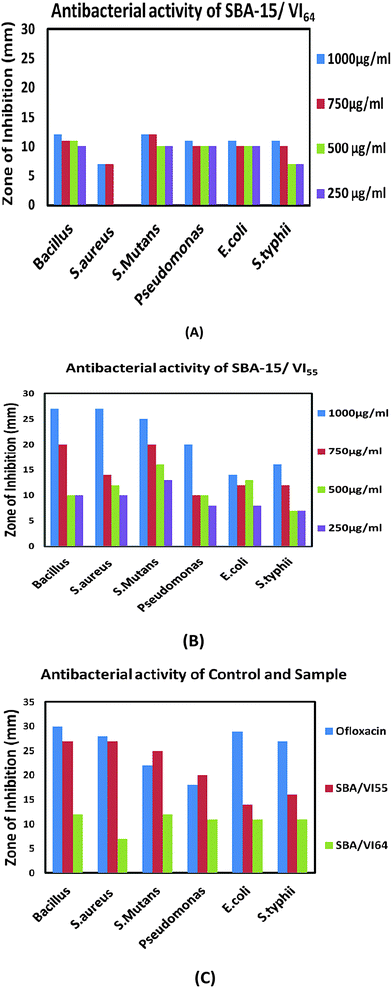 |
| Fig. 5 Antibacterial activity against Gram positive and Gram negative bacteria of (A) SBA/VI64 (B) SBA/VI55 (C) Control and the samples. | |
Mechanistically, the antibacterial activity of SBA/VI (in aqueous media) can be explained by the electrostatic interactions (between positive charge of VI and negative charge of bacterial membranes) and hydrophobic interactions between the alkyl chain (C
C) of vinyl imidazole and the cytoplasmic membrane of bacteria responsible for the agglomeration of SBA/VI nanocomposites on bacterial membranes [Scheme 1] resulting in the disruption of membranes and cell death.38–40 Other mode of action such as the inhibition of mitochondrial ATPase and DNA damage had also been proposed in previous reports.41–43 The vinyl imidazole ions interact with the domain of F1F0 ATPase which is responsible for the pumping of electrons across the inner mitochondrial membrane resulting in the inhibition of mitochondrial ATPase.44 SBA/VI penetrates the cell membrane and binds to DNA through the imidazole groups. This leads to destabilization due to mutation which alters the protein sequences thereby influencing the transcriptional system resulting in the damage of DNA.45
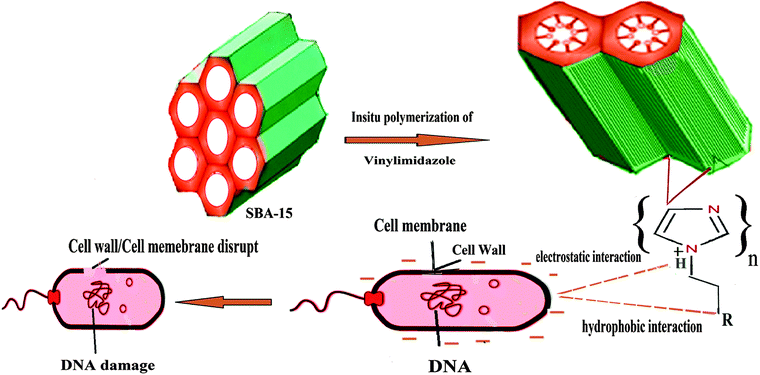 |
| Scheme 1 Mechanistic pathway. | |
Conclusions
SBA/VI nanocomposites were synthesized via in situ radical polymerization of vinyl imidazole inside the mesoporous silica for antibacterial activity. The antibacterial activity against Gram positive and Gram negative bacteria increased with an increase in the concentration of vinyl imidazole and the maximum activity could be achieved with SBA/VI55 nanocomposites. The zone of inhibition for Gram positive and for Gram negative bacteria was upto 27 mm and 20 mm, respectively, for SBA/VI55. The antibacterial activity is beneficial in terms of biodegradability, low requirements of the sample and environmental friendly conditions. Further efforts are currently underway for other bacteria.
Acknowledgements
AD thanks the institute and CSIR, (01 (2696)12/EMR-II), New Delhi for research funding.
References
- A. P. Wight and M. E. Davis, Chem. Rev., 2002, 102, 3589–3614 CrossRef CAS PubMed.
- F. Hoffmann, M. Cornelius, J. Morell and M. Fröba, Angew. Chem., Int. Ed., 2006, 45, 3216–3251 CrossRef CAS PubMed.
- N. Al-Haq, R. Ramnauth, S. Kleinebiekel, D. Ou, A. Sullivan and J. Wilson, Green Chem., 2002, 4, 239–244 RSC.
- S. Cheng, X. Wang and S. Chen, Top. Catal., 2009, 52, 681–687 CrossRef CAS.
- B. Choudary, M. Kantam, P. Sreekanth, T. Bandopadhyay, F. Figueras and A. Tuel, J. Mol. Catal. A: Chem., 1999, 142, 361–365 CrossRef CAS.
- A. Azam, A. Ahmed, M. Oves, M. Khan, S. Habib and A. Memic, Int. J. Nanomed., 2012, 7, 6003–6009 CrossRef CAS PubMed.
- S. Saravanamurugan, D. Han, J. Koo and S. Park, Catal. Commun., 2008, 9, 158–162 CrossRef CAS.
- M. Foglia, G. Alvarez, P. Catalano, A. Mebert, L. Diaz, T. Coradin and M. Desimone, Recent Pat. Biotechnol., 2011, 5, 54–61 CrossRef CAS PubMed.
- A. M. Mebert, D. E. Camporotondi, M. L. Foglia, G. S. Alvarez, P. L. S. Orihuela, L. E. Diaz and M. Desimone, J. Biomater. Tissue Eng., 2013, 3, 108–121 CrossRef CAS.
- V. S. Y. Lin, C.-Y. Lai, J. Huang, S.-A. Song and S. Xu, J. Am. Chem. Soc., 2001, 123, 11510–11511 CrossRef CAS PubMed.
- D. R. Radu, C.-Y. Lai, J. W. Wiench, M. Pruski and V. S. Y. Lin, J. Am. Chem. Soc., 2004, 126, 1640–1641 CrossRef CAS PubMed.
- R. Casasus, M. D. Marcos, R. Martinez-Manez, J. V. Ros-Lis, J. Soto, L. A. Villaescusa, P. Amoros, D. Beltran, C. Guillem and J. Latorre, J. Am. Chem. Soc., 2004, 126, 8612–8613 CrossRef CAS PubMed.
- K. Govindaraju, S. Tamilselvan, V. Kiruthiga and G. Singaravelu, J. Biopestic., 2010, 3, 394–399 CAS.
- I. Sondi and B. Salopek-Sondi, J. Colloid Interface Sci., 2004, 275, 177–182 CrossRef CAS PubMed.
- L. Wang, H. He, C. Zhang, L. Sun, S. Liu and R. Yue, J. Appl. Microbiol., 2014, 116, 1106–1118 CrossRef CAS PubMed.
- H. Caner, E. Yilmaz and O. Yilmaz, Carbohydr. Polym., 2007, 69, 318–325 CrossRef CAS.
- E. B. Anderson and E. L. Timothy, Polymer, 2010, 51, 2447–2454 CrossRef CAS.
- M. G. Chung, H. W. Kim, B. R. Kim, Y. B. Kim and Y. H. Rhee, Int. J. Biol. Macromol., 2012, 50, 310–316 CrossRef CAS PubMed.
- L. Cheng, K. G. Abhilash and R. Breslow, Proc. Natl. Acad. Sci. U. S. A., 2012, 109, 12884–12887 CrossRef CAS PubMed.
- C.-G. Wu and T. Bein, Science, 1994, 264, 1757–1759 CrossRef CAS PubMed.
- K. Moller, T. Bein and R. X. Fischer, Chem. Mater., 1998, 10, 1841–1852 CrossRef CAS.
- T. Q. Nguyen, J. J. Wu, V. Doan, B. J. Schwartz and S. H. Tolbert, Science, 2000, 288, 652–656 CrossRef CAS PubMed.
- A. Dubey, M. Choi and R. Ryoo, Green Chem., 2006, 8, 144–146 RSC.
- D. Sachdev and A. Dubey, Catal. Lett., 2011, 141, 1548–1556 CrossRef CAS.
- D. Sachdev and A. Dubey, Catal. Commun., 2013, 39, 39–43 CrossRef CAS.
- I. Makarovsky, Y. Boguslavsky, M. Alesker, J. Lellouche, E. Banin and J. P. Lellouche, Adv. Funct. Mater., 2011, 21, 4295–4304 CrossRef CAS.
- A. Dong, J. Huang, S. Lan, T. Wang, L. Xiao, W. Wang, T. Zhao, X. Zheng, F. Liu, G. Gao and Y. Chen, Nanotechnology, 2011, 22, 295602 CrossRef PubMed.
- K. Shalini, P. K. Sharma and N. Kumar, Chem. Sin., 2010, 1, 36–47 CAS.
- S. Tsubura, H. Mizunuma, S. Ishikawa, I. Oyake, M. Okabayashi, K. Katoh, M. Shibata, T. Iizuka, T. Toda and T. Iizuka, Eur. J. Clin. Microbiol., 2009, 28, 1353–1356 CrossRef CAS PubMed.
- A. M. Hammerum and O. E. Heuer, Clin. Infect Dis., 2009, 48, 916–921 CrossRef CAS PubMed.
- M. S. Amin, R. L. Harrison, T. S. Benton, M. Roberts and P. Weinstein, Pediatr. Dent. J., 2004, 26, 5–10 Search PubMed.
- B. M. Marshall and S. B. Levy, Clin. Microbiol. Rev., 2011, 24, 718–733 CrossRef CAS PubMed.
- U. Dutta, P. K. Garg, R. Kumar and R. K. Tandon, Am. J. Gastroenterol., 2000, 95, 784–787 CrossRef CAS PubMed.
- S. N. Venezia, R. Ben-Ami and Y. Carmeli, Curr. Opin. Infect Dis., 2005, 18, 306–313 CrossRef.
- M. Kruk, M. Jaroniec, C. H. Ko and R. Ryoo, Chem. Mater., 2000, 12, 1961–1968 CrossRef CAS.
- D. Sachdev, A. Dubey, G. R. Wilson and A. Sharma, New J. Chem., 2015, 39, 2633–2641 RSC.
-
M. A. Wikler, National Committee for Clinical Laboratory Standards (NCCLS), 2000, vol. 32, pp. M7-M5.
- M. G. Chung, H. W. Kim, B. R. Kim, Y. B. Kim and Y. H. Rhee, Int. J. Biol. Macromol., 2012, 50, 310–316 CrossRef CAS PubMed.
- A. Kanazawa, T. Ikeda and T. Endo, Antimicrob. Agents Chemother., 1994, 38, 945–952 CrossRef CAS PubMed.
- S. Jiang, L. Wang, H. Yu and Y. Chen, React. Funct. Polym., 2005, 62, 209–213 CrossRef CAS.
- C. Borelli, M. Schaller, M. Niewerth, K. Nocker, B. Baasner, D. Berg, R. Tiemann, K. Tietjen, B. Fugmann and S. Lang-Fugmann, Chemotherapy, 2008, 54, 245–259 CrossRef CAS PubMed.
- K. S. Atwal, P. Wang, W. L. Rogers, P. Sleph, H. Monshizadegan, F. N. Ferrara, S. Traeger, D. W. Green and G. J. Grover, J. Med. Chem., 2004, 47, 1081–1084 CrossRef CAS PubMed.
- K. Hori, A. Sakaguchi, M. Kudoh, K. Ishida, Y. Aoyama and Y. Yoshida, Chem. Pharm. Bull., 2000, 48, 60–64 CrossRef CAS PubMed.
- K. S. Atwal, P. Wang, W. L. Rogers, P. Sleph, H. Monshizadegan, F. N. Ferrara, S. Traeger, D. W. Green and G. J. Grover, J. Med. Chem., 2004, 47, 1081–1084 CrossRef CAS PubMed.
- H. El-Hamsharya, M. M. G. Foudaa, M. Moydeen, M. H. El-Newehy, S. S. Al-Deyab and A. Abdel-Megeed, Int. J. Biol. Macromol., 2015, 72, 1466–1472 CrossRef PubMed.
|
This journal is © The Royal Society of Chemistry and the Centre National de la Recherche Scientifique 2016 |