DOI:
10.1039/C5FO00817D
(Paper)
Food Funct., 2016,
7, 308-314
The effect of recombinant human lactoferrin from the milk of transgenic cows on Salmonella enterica serovar typhimurium infection in mice
Received
6th July 2015
, Accepted 21st September 2015
First published on 29th September 2015
Abstract
Lactoferrin (LF) is a multifunctional protein with antibacterial and immunomodulatory activities. Given this beneficial effect, transgenic approaches have been used to produce lactoferrin. The aim of the current study was to investigate the in vivo effect of recombinant human lactoferrin (rhLF) from the milk of transgenic cows on Salmonella enterica serovar typhimurium (ST) infection in mice. Two hours before the infection with 0.3 ml at 2 × 105 CFU ml−1 of ST, each animal in the ST + rhLF group received 0.3 ml of rhLF with 20 mg ml−1 concentration while the ST group received PBS as placebos with the same volume through oral gavage. The mice were infected with ST once only on the first day. After the infection, the mice received 0.3 ml of rhLF with 20 mg ml−1 (6 mg d−1) concentration or PBS, respectively, for 7 days. Mortality and weight were monitored daily. Bacterial enumeration in the blood, liver, and spleen and histopathological analysis of the liver, spleen, kidney and intestine were conducted. The results showed that rhLF decreased the bacterial load in the liver and spleen of mice, reduced the degree of mice hepatomegaly and splenomegaly, and attenuated infectious inflammation with less histopathological abnormalities in the liver, spleen and kidney of mice in the ST infection. This study showed that rhLF with 6 mg per day had antibacterial activity of alleviating the infection caused by ST bacteria, which indicated that rhLF could be used as a supplement in special products.
Introduction
Salmonella infection is one of the most common and widely distributed foodborne diseases, which constitutes a major public health burden and represents a significant cost in many countries.1 It is estimated that tens of millions of human cases occur worldwide every year and the disease results in more than one hundred thousand deaths. An estimated 1.0 million non-typhoidal Salmonella infections result in 350
000 hospitalizations and 378 deaths annually in the USA.2
Human lactoferrin (hLf) is an 80 kDa glycoprotein which is mainly found in milk and also in other secretions, like tears and saliva, and in the granules of neutrophils. It is a bioactive milk protein with multiple biological functions, such as antimicrobial activities, improving iron absorption, modulating immune system, anticarcinogenic and anti-inflammatory properties,3,4 among which the antimicrobial properties are the most widely studied one.5–8 It was reported that lactoferrin has shown an inhibitory effect on Gram-positive bacteria and Gram-negative bacteria, including various enteropathogens such as Helicobacter pylori, Staphylococcus aureus, Shigella flexneri, enteropathogenic Escherichia coli (EPEC) and Salmonella enterica serovar typhimurium.9–14
The usefulness of lactoferrin (LF) has led scientists to investigate the use of health-enhancing nutraceutical protein in food and pharmaceutical applications. However, due to the high cost of lactoferrin isolated from breast milk, it is difficult and unfeasible to achieve large-scale production. With advances in genetic engineering, it is possible to produce recombinant human lactoferrin in various expression systems, such as in microbial, animal and plant systems.4 Among these expression systems, large quantities of proteins from the milk of transgenic cows could be a cost-effective alternative for the production of recombinant proteins through cell culture or fermentation.15 It was reported that the main physico-chemical and biochemical characteristics of rhLF from transgenic cows have been shown to be practically the same as those of the milk protein. However, the antibacterial effect of rhLF has scarcely been explored. Therefore, the aim of the present study was to explore the antibacterial effect of rhLF from the milk of transgenic cows on oral infection of ST in BALB/c mice in vivo.
Materials and methods
Bacteria strain and culture conditions
The parent strain, Salmonella enterica serovar typhimurium strain 50333 was kindly provided by The Foodborne Disease Surveillance Department, China National Center for Food Safety Risk Assessment. Bacteria were routinely cultured in BHI broth (Oxoid) and on solid BHA agar plates (Oxoid) at 37 °C. Prior to animal infections, bacteria were cultured overnight in BHI broth, washed in a buffer containing 0.1 M phosphate-buffered saline (PBS, pH 7.2–7.4), and resuspended in the same buffer to obtain 108 colony forming units (CFU) per ml.
Source of rhLF
The rhLF was separated from the milk of transgenic cows bred by Jipulin Biotechnology Limited Company of Beijing and was purified by Engineering Research Institute of Chinese Academy of Sciences. The purity of the rhLF was 95% with an iron saturation of 13.68%. This rhLF had been evaluated for its genetic toxicity,16 effect on the growth of rats17 and on the iron status of rats suffering from iron deficiency.18 Its antibacterial activity on certain Gram-negative and Gram-positive bacteria in vitro was also investigated (data not shown). Here, we used the same rhLF as those in other studies. Before daily usage, rhLF was dissolved immediately in PBS with pH 7.2–7.4 and then given to the mice. The concentration of rhLF in the experiments was 20 mg ml−1.
Experimental animals
Female BALB/c strain mice of 6–8 weeks and 17–20 g body weight were used for the experiment. All the mice were healthy prior to inoculation. The animals were subjected to 12 hours light–dark cycles in a temperature and humidity-controlled room during the study process. They had free access to feed and water and were observed once daily before the experiment.
Identification of LD50
After 5 days of accommodation period, four groups with ten mice in each group were administered by oral gavage with four serial doses of ST strain 50333, which was 108, 107, 106, and 105 CFU ml−1 respectively. Each mouse was given 0.3 ml bacterial solution diluted with PBS buffer. Before ingestion, the mice were allowed to have water freely but were restricted to have feed overnight. After inoculation, the mortality of mice was observed for 10 days. Then the LD50 of Salmonella enterica serovar typhimurium was estimated by the Bliss method.
Throughout the determination, the LD50 of Salmonella enterica serovar typhimurium strain 50333 was 2 × 106 CFU ml−1.
Animal assignment in antibacterial process
1/10 of LD50 bacterial solution was used for ST infection treatment, which was 2 × 105 CFU ml−1. The mice were randomly divided into two groups with twelve in each group according to their body weight, the ST group as the control and rhLF as the treatment group (ST + rhLF).
Animal infections in the treatment process
The mice were infected with ST once only on the first day during the period of experiment. Two hours before the infection with 0.3 ml of 2 × 105 CFU ml−1 of ST, each animal in the ST + rhLF group received 0.3 ml of rhLF at 20 mg ml−1 while the ST group received PBS as placebos with the same volume through oral gavage. For infection treatment inoculations, a gavage needle was used. After the infection, the mice were given 0.3 ml of rhLF with 20 mg ml−1 (6 mg d−1) or PBS, respectively, for 7 days. Animals had access to drinking water and food ad libitum.
During the period of the experiment, general performance, mortality and body weight of the mice had been monitored daily for seven days. Any animal that exhibited severe clinical abnormalities became moribund, or lost 20% of initial body weight was sacrificed by ether narcosis followed by exsanguinations. Seven days later, the animals were anaesthetized with ether and then the abdominal cavity was opened aseptically to perform cardiac puncture for blood. Soon after that, their organs (intestine, liver, spleen and kidney) were aseptically removed. The protocol was approved by the Experimental Animal Welfare and Ethics Committee of National Institute for Nutrition and Health, Chinese Center for Diseases Control and Prevention (China CDC, Permit Number: 2013-020). All efforts were made to minimize their suffering.
Bacterial load in mice tissue
Mice liver and spleen were weighed, and half of them were put into cold sterile PBS with the volume proportion of one to ten, and then ground in a sterilized mortar. Serial dilutions (10−2, 10−3, 10−4 and 10−5) of blood and the homogenized liver and spleen were spread on XLT4 agar plates (BD) and incubated at 37 °C in a constant temperature box for 24 to 48 hours to quantify ST as CFU g−1 of tissue. Each dilution had parallel samples for quality control.
Pathology scoring
Tissues from the intestine, kidney, liver and spleen were used for histopathological analysis. The tissue samples were fixed in 3% formalin, embedded in paraffin and stained with hematoxylin and eosin. To avoid bias, the degree of inflammation in organs was analyzed by a pathologist blind to the group assignment. Pathological scores were determined by five average fields/samples and the degree of inflammation was scored on a 0 to 3+ scale (0: no obvious pathological changes, essentially normal; 1+ mild lesions: lesions are sporadic and focus and lesion range was less than 1/3 of the total tissue area; 2+ moderate lesions: focal lesions show flakes or intensive and scope of lesions reached 1/3–2/3 of the total tissue area; 3+ severe lesions: the scale of lesions was more than 2/3 of the total tissue area).
Statistical analysis
The mortality was determined by the chi-square test. For continuous variables (body weight, bacterial load in organs, organ's weight), Student's t test was used. The Kruskal–Wallis test was used and then followed by a Dunnett-type method when appropriate. Log transformation was done for bacterial load before comparison between groups. For categorical variables (the number of mice with detectable bacteria in their organs and histopathological analysis), the chi-square test was performed. The difference was considered to be statistically significant when P < 0.05. SPSS 16.0 was used for the analysis.
Results
Effect of rhLF on mortality of mice
A total of three deaths occurred in the two groups, three in the ST group on the fifth day, and none in the group interfered with rhLF. The chi-square test showed that there was no significant difference between the ST group and ST + rhLF group (χ2 = 3.43, P > 0.05).
Effect of rhLF on the body weight of mice
Changes in the body weight of the mice were monitored at the same time of the day for one week (Table 1). The results showed that the weight of mice in the ST group was similar to that of the ST + rhLF group each day after Salmonella enterica serovar typhimurium infection (P > 0.05).
Table 1 Mice body weight before and after infection of Salmonella enterica serovar typhimurium (g, mean ± SD)
Group |
Before infection |
After infection |
Day 1 |
Day 2 |
Day 3 |
Day 4 |
Day 5 |
Day 6 |
Day 7 |
ST |
18.38 ± 0.69 |
19.26 ± 1.02 |
19.29 ± 0.67 |
18.78 ± 0.84 |
18.29 ± 1.07 |
18.09 ± 1.42 |
17.77 ± 1.77 |
18.22 ± 2.34 |
ST + rhLF |
18.48 ± 0.88 |
19.16 ± 1.07 |
19.28 ± 1.08 |
19.23 ± 1.13 |
19.23 ± 1.18 |
19.14 ± 1.17 |
19.10 ± 0.88 |
18.93 ± 1.34 |
Effect of rhLF on the organ ratio of mice
As shown in Table 2, there was no obvious difference in the wet weight of the liver and the ratio of liver to body weight between the ST group and ST + rhLF group (P > 0.05). The wet weight of spleen and the ratio of spleen to body weight in the ST group were obviously higher than that of the ST + rhLF group (P < 0.05).
Table 2 The organ weight of mice in each group
Group |
Wet weight of liver (g) |
Ratio of liver to body weight (%) |
Wet weight of spleen (g) |
Ratio of spleen to body weight (%) |
*Compared with the control group, P < 0.05. |
ST |
0.89 ± 0.45 |
5.37 ± 2.49 |
0.13 ± 0.07 |
0.79 ± 0.43 |
ST + rhLF |
1.04 ± 0.28 |
6.28 ± 1.77 |
0.08 ± 0.04* |
0.48 ± 0.19* |
Effect of rhLF on the bacterial load in mice tissues
The number of mice with detectable Salmonella enterica serovar typhimurium in the blood and liver was similar between the ST group and ST + rhLF group. While the number of mice with bacteria in the spleen of the ST + rhLF group was apparently less than that of the ST group (Table 3).
Table 3 The number of mice with detectable Salmonella enterica serovar typhimurium in blood, liver and spleen
Group |
Blood |
Liver |
Spleen |
Yes |
% |
Yes |
% |
Yes |
% |
*Compared with the control group, P < 0.05. |
ST |
11 |
91.67 |
12 |
100 |
12 |
100 |
ST + rhLF |
7 |
58.33 |
10 |
83.33 |
7* |
58.33* |
Compared to the ST group, the bacterial load in the liver and spleen in the ST + rhLF group was obviously reduced (Table 4). The bacteria level in blood was similar between these two groups (P < 0.05).
Table 4 Bacterial load of mice in blood, liver and spleen after infection (CFU g−1 of tissue, log
10)
Group |
Blood |
Liver |
Spleen |
*Compared with the control group, P < 0.05. |
ST |
5.10 ± 2.54 |
7.86 ± 2.40 |
7.85 ± 2.91 |
ST + rhLF |
3.55 ± 3.15 |
5.58 ± 2.81* |
4.19 ± 3.73* |
Effects of rhLF on the histopathology of mice
Histopathological abnormalities in the two groups are displayed in Table 5 and Fig. 1. There was seven animals with inflammation in the liver (7/12, 58.3%) in the ST group, compared with one (1/12, 8.3%) in the ST + rhLF group (Fig. 1A and B; Table 5; P < 0.05). Inflammation degree analysis showed that the ST + rhLF group had much fewer animals with moderate lesions (P < 0.05). Similar abnormal frequencies were observed in the spleen between the ST group and ST + rhLF group (Fig. 1C and D; Table 5; P > 0.05). However, the number of mice with severe lesions in the ST + rhLF group was obviously less than that of the ST group (P < 0.05). The number of mice with moderate lesions in the kidney in the ST + rhLF group was obviously less than that of the control group (Fig. 1E and F; Table 5; P < 0.05). The intestine showed necrotizing inflammation and damage in the intestinal mucosa or intestinal villi in some animals, but there was no significant difference in the frequencies of lesion between the ST group and ST + rhLF group (Fig. 1G and H; Table 5; P > 0.05).
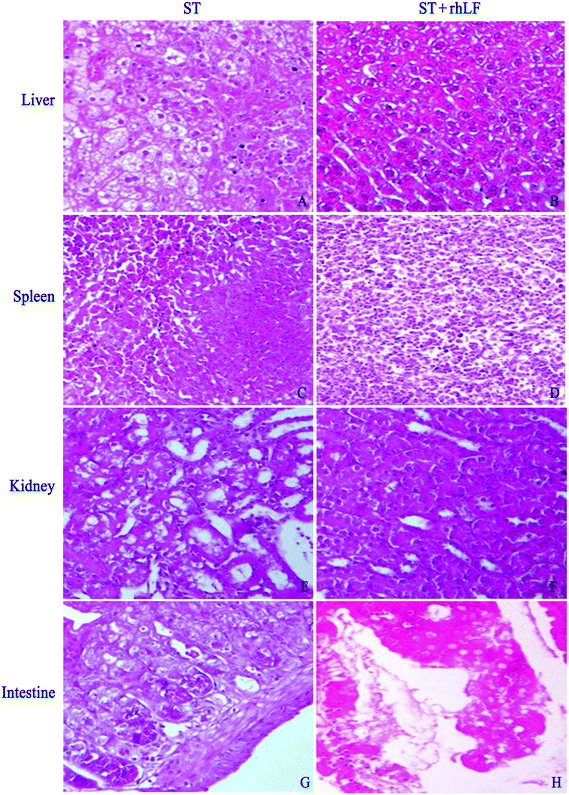 |
| Fig. 1 Histopathological analysis for organs of mice infected with ST. The inflammation degree of mice in the liver (B), spleen (D) and kidney (F) in the ST + rhLF group was less severe than that of the control group (A, C, E, respectively). As to the intestine, there was no obvious difference between the control group (G) and ST + rhLF (H). The scale bar was 1 : 100. | |
Table 5 Histological analysis of the recovered organs
Organs |
ST n/N (%) |
ST + rhLF n/N (%) |
*P < 0.05 for the comparison between rhLF and the control group. |
Liver
|
Inflammation |
7/12(58.3) |
1/12(8.3)* |
3+ |
0/12(0.0) |
0/12(0.0) |
2+ |
5/12(41.7) |
1/12(8.3)* |
1+ |
2/12(16.7) |
0/12(0.0) |
— |
5/12(41.7) |
11/12(91.7) |
Spleen
|
Inflammation |
7/12(58.3) |
5/12(41.7) |
3+ |
5/12(41.7) |
0/12(0.0)* |
2+ |
1/12(8.3) |
0/12(0.0) |
1+ |
1/12(8.3) |
5/12(41.7) |
— |
5/12(41.7) |
7/12(58.3) |
Kidney
|
Inflammation |
9/12(75.0) |
10/12(83.3) |
3+ |
0/12(0.0) |
0/12(0.0) |
2+ |
6/12(50.0) |
1/12(8.3)* |
1+ |
3/12(25.0) |
9/12(75.0) |
— |
3/12(25.0) |
2/12(16.7) |
Intestine
|
Inflammation |
2/12(16.7) |
1/12(8.3) |
3+ |
0/12(0.0) |
0/12(0.0) |
2+ |
1/12(8.3) |
0/12(0.0) |
1+ |
1/12(8.3) |
1/12(8.3) |
— |
10/12(83.3) |
11/12(91.7) |
Discussion
The naturally susceptible mouse lineages (e.g., BALB/c) are widely used to study the pathogenesis of Salmonella enterica serovar typhimurium infections. These animals show signs of illness between 4 and 8 days post oral infection and are characterized by rapid bacterial multiplication in the blood, liver and spleen at a net growth rate of 0.5–1.5 log per day,19–21 which results in bacteraemia, hepatomegaly and splenomegaly.
Previous research based on animal models of experimental infection showed that LF had a protective action against pathogenic bacterial infection caused by E. coli, Staphylococcus aureus and Listeria monocytogenes.22–25 It was reported that the rhLF was effective in a mouse model of Helicobacter infection26 and could protect neonatal rats from gut-related systemic infection.27 The antibacterial effect of rhLF on Salmonella enterica serovar typhimurium infection has rarely been researched. It was reported that bovine LF protected against Salmonella enterica serovar typhimurium infection in BALB/c mice, reducing the severity, mortality and the degree of inflammation of this infection.14 One study showed that bovine lactoferrin reduced the mortality of BALB/c mice lethally infected by Salmonella enterica serovar typhimurium, and reduced bacterial colonization at Peyer's patch and bacterial translocation in the liver and spleen in sublethally infected mice.28 rhLF in the current study decreased the bacterial load in the liver and spleen, and also alleviated infectious inflammation with less histopathological abnormalities in the liver, spleen and kidney of mice in the Salmonella enterica serovar typhimurium infection.
However, Salmonella enterica serovar typhimurium used in the present research caused less infection in the intestine than in the liver, spleen or kidney of mice, which was somehow different from previous studies.14 R. Jiang et al. reported that iron-free and iron-saturated lactoferrin affects human enterocytes in a different way.29,30 In addition, apolactoferrin has been shown as more potent in reducing the growth of numerous bacterial strains.31–34 The iron saturation of the rhLF in this study was lower than that of Susan Mosquito et al., while it was higher than that of Maria Elisa Drago-Serrano et al. Therefore, iron saturation mightn't be a potential factor for the disparity. One possible reason could be considered that different strains of Salmonella enterica serovar typhimurium cause distinctive clinical syndromes.35
rhLF used in the current study also could improve the growth of rats14 and improve the iron status of iron deficiency anemia to some degree by the supplementation of rhLf alone.14,34 Before investigating the antibacterial activity on Salmonella enterica serovar typhimurium infection in mice, the rhLF showed an obvious inhibitory effect against Gram-negative and Gram-positive bacteria, such as Salmonella typhimurium, Pseudomonas aeruginosa, Enterobacter sakazakii, Escherichia coli, Staphylococcus aureus, Listeria monocytogenes in vitro (data not shown). These related data indicated that rhLF has similar functions as natural LF.
Multiple underlying mechanisms have been proposed to explain the antibacterial effect of LF. As an iron chelator, LF can effectively inhibit biofilm formation through iron sequestration;36,37 and directly interact with microbiological membranes,38,39 alter the membrane permeability through dispersion of membrane components such as lipopolysaccharides (LPS) in Gram-negative bacteria and lead to organism's death.40,41 As a multifunctional immunoregulatory protein, lactoferrin could also increase the production of total or specific IgA, IgG and IgM antibodies in mice.42–45 Although the possible mechanisms had not been clarified in this study, we speculated that the antibacterial activity of rhLF in Salmonella enterica serovar typhimurium infection in mice could contribute to the iron chelation, alteration of the membrane permeability and stimulation of the immune system of mice.
One limitation exists in this study was that it couldn't compare the antibacterial effect of rhLF with the natural bovine LF, which needs further study to examine. Another limitation might be that the mechanism by which the rhLF inhibited Salmonella enterica serovar typhimurium to blunt infection remains unclear. In conclusion, the present study demonstrated that recombinant human lactoferrin has considerable protective efficacy at combating Salmonella enterica serovar typhimurium infection in vivo. Therefore, rhLF might also have protective effects on inhibiting enteropathogenic bacteria and preventing foodborne disease. Further work is needed to determine the large potential applications of this valuable protein.
Acknowledgements
This work was supported by the National Key Science & Technology specific projects (no. 81273073). We gratefully acknowledge Jipulin Biotechnology Limited Company of Beijing for supplying rhLF, Cathy Liu and Yiqun Liu for their review of the use of English in this manuscript.
References
- World Health Organization, Salmonella (non-typhoidal).Factsheet no.139., http://www.who.int/mediacentre/factsheets/fs139/en/, (accessed March 2014).
- E. Scallan, R. M. Hoekstra, F. J. Angulo, R. V. Tauxe, M. A. Widdowson, S. L. Roy, J. L. Jones and P. M. Griffin, Foodborne illness acquired in the United States-major pathogens, Emerging Infect. Dis., 2011, 17, 7–15 CrossRef PubMed.
- P. F. Levay and M. Viljoen, Lactoferrin: a general review, Haematologica, 1995, 80, 252–267 CAS.
- I. A. García-Montoya, T. S. Cendón, S. Arévalo-Gallegos and Q. Rascón-Cruz, Lactoferrin a multiple bioactive protein: an overview, Biochim. Biophys. Acta, 2012, 1820, 226–236 CrossRef PubMed.
- S. A. González-Chávez, S. Arévalo-Gallegos and Q. Rascón-Cruz, Lactoferrin: structure, function and applications, Int. J. Antimicrob. Agents, 2009, 33, 301 CrossRef PubMed , e1-8.
- A. H. Albar, H. A. Almehdar, V. N. Uversky and E. M. Redwan, Structural heterogeneity and multifunctionality of lactoferrin, Curr. Protein Pept. Sci., 2014, 15(8), 778–797 CrossRef CAS.
- M. Marchetti, F. Superti, M. G. Ammendolia, P. Rossi, P. Valenti and L. Seganti, Inhibition of poliovirus type 1 infection by iron-, manganese-, and zinc-saturated lactoferrin, Med. Microbiol. Immunol., 1999, 187, 199–204 CrossRef CAS.
- R. Sato, O. Inanami, Y. Tanaka, S. E. Takase and Y. Naito, Oral administration of bovine lactoferrin for treatment of intractable stomatitis in feline immunodeficiency virus (FIV)-positive and (FIV)-negative cats, Am. J. Vet. Res., 1996, 57, 1443–1446 CAS.
- H. F. Gomez, T. J. Ochoa, I. Herrera-Insua, L. G. Carlin and T. G. Cleary, Lactoferrin protects rabbits from Shigella flexneri-induced inflammatory enteritis, Infect. Immun., 2002, 70(12), 7050–7053 CrossRef CAS.
- T. J. Ochoa, E. L. Brown, C. E. Guion, J. Z. Chen, R. J. McMahon and T. G. Cleary, Effect of lactoferrin on Enteroaggregative E. Coli (EAEC), Biochem. Cell Biol., 2006, 84(3), 369–376 CrossRef CAS PubMed.
- T. J. Ochoa, M. Noguera-Obenza, F. Ebel, C. A. Guzman, H. F. Gomez and T. G. Cleary, Lactoferrin impairs type III secretory system function in enteropathogenic Escherichia coli, Infect. Immun., 2003, 71(9), 5149–5155 CrossRef CAS.
- S. Farnaud and R. W. Evans, Lactoferrin—a multifunctional protein with antimicrobial properties, Mol. Immunol., 2003, 40(7), 395–405 CrossRef CAS.
- A. Del Olmo, J. Calzada and M. Nuñez, Antimicrobial effect of lactoferrin and its amidated and pepsin-digested derivatives against Salmonella enteriditids and Pseudomona fluorescence, J. Dairy Sci., 2010, 93, 3965–3969 CrossRef CAS PubMed.
- S. Mosquito, T. J. Ochoa, J. Cok and T. G. Cleary, Effect of bovine lactoferrin in Salmonellaser. Typhimurium infection in mice, Biometals, 2010, 23, 515–521 CrossRef CAS PubMed.
- J. Zhang, L. Li, Y. Cai, X. Xu, J. Chen, Y. Wu, H. Yu, G. Yu, S. Liu, A. Zhang, J. Chen and G. Cheng, Expression of active recombinant human lactoferrin in the milk of transgenic goats, Protein Expression Purif., 2008, 57, 127–135 CrossRef CAS PubMed.
- S. Liu, X. Feng, C. Li, G. Geng, H. Liu, Y. Zhi and H. Xu, Genetic toxicity of recombinant human laotoferrin, Carcinog., Teratog. Mutagen., 2011, 23(5), 384–387 CAS (Chinese).
- X. Wang, S. Liu, W. Yan and H. Xu, Effect of recombinant human lactoferrin on improving the growth of rats, Chin. J. Food Hyg., 2011, 23(2), 105–108 Search PubMed (Chinese).
- X. Wang, S. Liu, H. Xu and W. Yan, Effects of recombinant human lactoferrin on improving the iron status of IDA rats, J. Hyg. Res., 2012, 41(1), 13–17 CAS (Chinese).
- C. E. Hormaeche, The in vivo division and death rates of Salmonella Typhimuriumin the spleens of naturally resistant and susceptible mice measured by the superinfecting phage technique of Meynell, Immunology, 1980, 41, 973–979 CAS.
- A. Haraga, M. B. Ohlson and S. I. Miller, Salmonellae interplay with host cells, Nat. Rev. Microbiol., 2008, 6(1), 53–66 CrossRef CAS PubMed.
- A. I. Nilsson, E. Kugelberg, O. G. Berg and D. I. Andersson, Experimental adaptation of Salmonella Typhimurium to mice, Genetics, 2004, 168(3), 1119–1130 CrossRef PubMed.
- T. Zagulski, P. Lipí nski, A. Zagulska and Z. Jarz abek, Antibacterial system generated by lactoferrin in mice in vivo is primarily a killing system, Int. J. Exp. Pathol., 1998, 79, 117–123 CAS.
- J. Artym, M. Zimecki and M. L. Kruzel, Enhanced clearance of Escherichia coliand Staphylococcus aureus in mice treated with cyclophosphamide and lactoferrin, Int. Immunopharmacol., 2004, 4, 1149–1157 CrossRef CAS PubMed.
- R. S. Bhimani, Y. Vendrov and P. Furmanski, Influence of lactoferrin feeding and injection against systemic staphylococcal infections in mice, J. Appl. Microbiol., 1999, 86, 135–144 CrossRef CAS.
- H. Y. Lee, J. H. Park, S. H. Seok, M. W. Baek, D. J. Kim, B. H. Lee, P. D. Kang, Y. S. Kim and J. H. Park, Potential antimicrobial effects of human lactoferrin against oral infection with Listeria monocytogenesin mice, J. Med. Microbiol., 2005, 54, 1049–1054 CrossRef CAS PubMed.
- E. J. Dial, J. J. Romero, D. R. Headon and L. M. Lichtenberger, Recombinant human lactoferrin is effective in the treatment of Helicobacter felis-infected mice, J. Pharm. Pharmacol., 2000, 52, 1541–1546 CrossRef CAS.
- L. Edde, R. B. Hipolito, F. F. Hwang, D. R. Headon, R. A. Shalwitz and M. P. Sherman, Lactoferrin protects neonatal rats from gut related systemic infection, Am. J. Physiol.: Gastrointest. Liver Physiol., 2001, 281, G1140–G1150 CAS.
- M. E. Drago-Serrano, V. Rivera-Aguilar, A. A. Reséndiz-Albor and R. Campos- Rodríguez, Lactoferrin increases both resistance to Salmonella Typhimurium infection and the production of antibodies in mice, Immunol. Lett., 2010, 134, 35–46 CrossRef CAS PubMed.
- R. Jiang, V. Lopez, S. L. Kelleher and B. Lönnerdal, Apo- and holo-lactoferrin are both internalized by lactoferrin receptor via clathrin-mediated endocytosis but differentially affect ERKsignaling and cell proliferation in Caco-2 cells, J. Cell Physiol., 2011, 226(11), 3022–3031 CrossRef CAS PubMed.
- R. Jiang and B. Lönnerdal, Apo-and holo-lactoferrin stimulate proliferation of mouse crypt cells but through different cellular signaling pathways, Int. J. Biochem. Cell Biol., 2012, 44(1), 91–100 CrossRef CAS PubMed.
- R. R. Arnold, M. Brewer and J. J. Gauthier, Bactericidal activity of human lactoferrin: sensitivity of a variety of microorganisms, Infect. Immun., 1980, 28(3), 893–898 CAS.
- R. R. Arnold, J. E. Russell, W. J. Champion, M. Brewer and J. J. Gauthier, Bactericidal activity of human lactoferrin: differentiation from the stasis of iron deprivation, Infect. Immun., 1982, 35(3), 792–799 CAS.
- R. T. Ellison, T. J. Giehl and F. M. LaForce, Damage of the outer membrane of enteric Gram-negative bacteria by lactoferrin and transferring, Infect. Immun., 1988, 56(11), 2774–2781 CAS.
- J. H. Crosa, Genetics and molecular biology of siderophoremediated iron transport in bacteria, Microbiol. Rev., 1989, 53(4), 517–530 CAS.
- R. M. Tsolis, R. A. Kingsley, S. M. Townsend, T. A. Ficht, L. G. Adams and A. J. Bäumler, Of mice, calves, and men. Comparison of the mouse typhoid model with other Salmonella infections, Adv. Exp. Med. Biol., 1999, 473, 261–274 CrossRef CAS.
- E. C. Leitch and M. D. Willcox, Lactoferrin increases the susceptibility of S. Epidermidis biofilms to lysozyme and vancomycin, Curr. Eye Res., 1999, 19, 12–19 CrossRef CAS.
- E. D. Weinberg, Suppression of bacterial biofilm formation by iron limitation, Med. Hypotheses, 2004, 63, 863–865 CrossRef CAS PubMed.
- N. Leon-Sicairos, F. Lopez-Soto, M. Reyes-Lopez, D. Godinez Vargas, C. Ordaz-Pichardo and M. de la Garza, Amoebicidal activity of milk, apo-lactoferrin, sIgA and lysozyme, Clin. Med. Res., 2006, 4, 106–113 CrossRef CAS.
- N. Orsi, The antimicrobial activity of lactoferrin: current status and perspectives, Biometals, 2004, 17, 189–196 CrossRef CAS.
- R. T. Coughlin, S. Tonsager and E. J. McGroaty, Quantitation of metal cations bound to membranes and extracted lipopolysaccharide of Escherichia coli, Biochemistry, 1983, 22, 2002–2007 CrossRef CAS.
- C. A. Leitch and M. D. Willcox, Elucidation of the antistaphylococcal action of lactoferrin and lysozyme, J. Med. Microbiol., 1999, 48, 867–871 CrossRef PubMed.
- H. Debbabi, M. Dubarry, M. Rautureau and D. Tome, Bovine lactoferrin induces both mucosal and systemic responses in mice, J. Dairy Res., 1998, 65, 283–293 CrossRef CAS.
- R. M. Sfeir, M. Dubarry, P. N. Boyaka, M. Rautureau and D. Tomé, The mode of oral bovine lactoferrin administration influences mucosal and systemic immune responses in mice, J. Nutr., 2004, 134, 403–409 CAS.
- R. Fisher, H. Debbabi, A. Blais, M. Dubarry, M. Rautureau, P. N. Boyaka and D. Tome, Uptake of ingested bovine lactoferrin and its accumulation in adult mouse tissues, Int. Immunopharmacol., 2007, l7, 1387–1393 CrossRef PubMed.
- S. S. Naidu, U. Svensson, A. R. Kishore and A. S. Naidu, Relationship between antibacterial activity and porin binding of lactoferrin in Escherichia coli and Salmonella typhimurium, Antimicrob. Agents Chemother., 1993, 37, 240–245 CrossRef CAS.
|
This journal is © The Royal Society of Chemistry 2016 |
Click here to see how this site uses Cookies. View our privacy policy here.