A biomimetic approach to hormone resistant prostate cancer cell isolation using inactivated Sendai virus (HVJ-E)†
Received
10th September 2015
, Accepted 27th October 2015
First published on 10th November 2015
Abstract
Our study reports a versatile immobilization method of Hemagglutinating Virus of Japan Envelope (HVJ-E) for the generation of viral-mimetic surfaces for hormone resistant prostate cancer cell isolation. HVJ-E has recently attracted much attention as a new type of therapeutic material because hormone resistant prostate cancer cells such as PC-3 cells possess the HVJ-E receptors, GD1a. The HVJ-E was successfully immobilized on precursor films composed of poly-L-lysine and alginic acid via layer-by-layer assembly without changing the biological activity. The monolayer adsorption of HVJ-E particles was confirmed by quartz crystal microbalance, fluorescent and atomic force microscopy analyses. By developing the HVJ-E coating with an affinity based cell trap within a glass capillary tube, we are able to gently isolate PC-3 from LN-Cap cells that represent adenocarcinoma without compromising cell viability. We achieved approximately 100% cell separation efficiency only by 60 seconds of flowing. We believe that the proposed technique offers significant promise for the creation of a hormone resistant cancer cell trap on a broad range of materials.
Introduction
Prostate carcinoma (PC) is the most common malignancy among elderly men in the world and is becoming a public health concern because the number of men over 65 years is expected to rise dramatically in the coming years. Although PC is often very slow growing, it can be aggressive, especially in young men. Around 25% of cases occur in men younger than 65. Although the incidence of deaths from PC has decreased over the last decade as a result of widespread prostate-specific antigen (PSA) screening among men younger than 65 years, PC has still been considered as a life-threatening disease because the five-year survival rate drops to 30 percent if the cancer has spread to distant parts of the body.1–3 In general, more than 90% of the PCs are a type called acinar adenocarcinoma which is characterized by the expression of androgen receptor (AR) and PSA.4 So, nearly everyone diagnosed with prostate cancer has this type. The remaining 10%, on the other hand, are variant forms including ductal adenocarcinoma and small cell neuroendocrine carcinoma (SCNC).5 These types of cells tend to grow and spread more quickly than acinar adenocarcinoma. In particular, SCNCs are highly aggressive, usually presenting with locally advanced disease or distant metastasis, and the patients usually die within months of diagnosis. In addition, SCNCs are often seen as recurrent tumors in patients who have received hormonal therapy that stops androgen production and inhibits AR function.6 This explains that SCNCs are considered as hormone-resistant PCs due to the lack of AR and PSA expression in contrast to the majority of prostatic adenocarcinomas. Therefore, the PSA test cannot be used to diagnose such type of carcinomas. From these perspectives, there is an urgent need to develop a new screening tool for malignant SCNCs.
Here we demonstrate for the first time a versatile and facile method for isolation of prostatic SCNCs from adenocarcinoma by the use of a biomimetic approach (Fig. 1). We have focused on hemagglutinating virus of Japan envelope (HVJ-E) which is derived from inactivation of original HVJ or Sendai virus by UV irradiation to destroy the internal RNA.7 The HVJ-E has recently been attracting considerable attention as a new type of therapeutic material due to its unique features. For example, HVJ-E itself has neither infective nor proliferative potential in humans, but it still possesses the cell attachment and fusion proteins on the envelope surface. In addition, HVJ-E has known to bind preferentially to hormone-resistant PCs because of the high expression of GD1a.8 More recently, Kaneda and co-workers have found that HVJ-E itself mediates a powerful anti-tumor effect by enhancing cytokine production in dendritic cells, generating tumor-specific cytotoxic T cells, and inhibiting regulatory T-cell activity.9,10
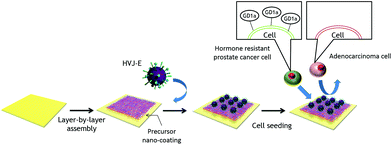 |
| Fig. 1 Schematic illustration for immobilization of HVJ-E particles onto the LbL precursor surface for hormone resistant cancer cell separation. | |
In this study, we first attempted to generate a homogeneous monolayer of HVJ-E on a substrate without changing the biological activity. We choose a layer-by-layer (LbL) assembly11,12 process for constructing the precursor film for HVJ-E immobilization to avoid the deactivation of surface proteins on the fragile envelope. The monolayer adsorption of HVJ-E particles was confirmed by quartz crystal microbalance (QCM), and fluorescent and atomic force microscopy (AFM) analysis. Then, we cultured PC-3 cells and LN-Cap cells on the HVJ-E immobilized surface, which represent SCNCs and adenocarcinomas, respectively. By developing the HVJ-E coating within a glass capillary tube, we are able to gently isolate PC-3 from LN-Cap cells.
Materials & methods
Materials
Poly-L-lysine hydrobromide (Mw 30
000–70
000), alginic acid sodium salt from brown algae (low viscosity), Dulbecco's phosphate buffer saline, pKH26 red fluorescent cell linker kit for general cell membrane labeling (pKH-26) and pKH67 green fluorescent cell linker kit for general cell membrane labeling (pKH-67) were purchased from Sigma Aldrich (St. Louis, MO). Quartz Crystal Microbalance substrate (9A-AQM-Au) was purchased from SEIKO EG&G (Tokyo, Japan). Cell counting kit-8 was purchased from Dojindo Molecular Technologies, Inc. (Kumamoto, Japan). The HVJ-E was purchased from Ishihara Sangyo Kaisha, Ltd (Osaka, Japan). Glass coverslips (15 mm in diameter) were purchased from Matsunami glass (Osaka, Japan). The RPMI-1640 with L-glutamine liquid for cell culture experiment was purchased from Nacalai tesque (Kyoto, Japan).
Preparation of HVJ-E immobilized glass substrates
A cationic poly-L-lysine (PLL) and an anionic alginic acid (ALG) were dissolved in Dulbecco's phosphate buffer saline (PBS) at concentrations of 1.0 mg mL−1 and 5.0 mg mL−1, respectively. The pH of PLL or ALG solution was then adjusted to 7.4 by the addition of hydrochloride (HCl; 0.1 M) or sodium hydroxide (NaOH; 0.1 M) solution, respectively. Next, glass coverslips, QCM substrates or glass tubes with 1.0 mm in diameter were washed with detergent followed by washing with Milli Q water three times. Then each substrate was dried with N2 gas. These cleaned substrates were dipped in the PLL solution for 15 minutes to form a cationic surface layer, followed by washing with Milli Q water three times and then dried with N2 gas. The cationic PLL coated substrates were then dipped in the anionic ALG solution for 15 minutes to form a polyionic complex on the substrate followed by washing and drying using the same procedure as before. These alternately cationic/anionic polymer adsorptions and washing processes were repeated for 0–18 layers in order to construct an LbL polymer multilayer on the substrate or inside the glass tube. For HVJ-E immobilization, PBS solutions with four different HVJ-E concentrations of 120, 1200, 3000, and 6000 Hemagglutinin Unit mL−1 (HAU mL−1) were prepared. The prepared LbL assembled substrates with PLL outermost layer were exposed to a different HVJ-E solution for 120 minutes. The samples were then cleaned using Milli-Q water and dried with N2 gas.
Characterization of HVJ-E immobilized glass substrates
A quartz crystal analyzer (QCA917, SEIKO EG&G. Tokyo Japan) was used for the quantification of the amount of the adsorbed LbL film and HVJ-E. The QCM substrate (9A-AQM-Au) has a 0.195 cm2 of the surface area. Then the frequency shift (F) was converted to the mass (ng) of the LbL multilayer and HVJ-E by Sauerbrey's equations.13 The static contact angles on the HVJ-E immobilized surfaces were also measured with DM-701 (Kyowa Interface Science Co., Ltd Saitama, Japan). Tissue culture polystyrene (TCPS) was modified with an LbL multilayer and 6000 HAU mL−1 of HVJ-E solution. The HVJ-E coated TCPS was immersed in a tank of Milli-Q water and then a 1 μL air bubble was deposited on the immersed surface by a nozzle from inside the vessel. Then the contact angle was calculated using the image analyzer software. Surface morphologies of LbL and HVJ-E immobilized substrate were observed by SPM-9500 (Shimadzu Co., Kyoto, Japan) with a Si3N4 cantilever in a non-contact mode (spring contact = 42 N m−1), respectively.
Hemolysis assay
3 mL of chicken red blood was dissolved in 12 mL of PBS and centrifuged at 1000 rpm for 5 min. The precipitate was washed twice with PBS and centrifuged at 1000 rpm for 5 min. 1 mL of the precipitate was dissolved in 49 mL of PBS. A glass coverslip and an LbL coated glass and a HVJ-E coated glass were placed into a 24 well plate. In the case of the DTT treated substrate, a 5 mM of DTT solution was co-incubated for 30 min at 37 °C before RBC injection. After the treatment RBC solutions were put into each well and cultured for 2 hours at 37 °C, respectively. Then each of the solutions was centrifuged and absorbance at 541 nm of the supernatant was measured by UV-vis spectrum.
Cell separation assay using 2D flat surfaces
Human prostate cancer PC-3 and LN-Cap cells were provided by RIKEN Cell bank, which represent prostatic SCNCs and adenocarcinomas, respectively. These cells were cultured in RPMI-1640 culture medium with 10% fetal bovine serum, respectively. Before cell seeding on the HVJ-E substrate, the PC-3 and LN-Cap cells were stained with pKH-67 and pKH-26 chemical reagents, respectively. After labelling, these cells were mixed and seeded onto the fibronectin (FN) coated, PLL outermost LbL coated and HVJ-E immobilized LbL coated glass coverslip. The cells were cultured for 4, 8, 24 and 72 hours on the substrate and washed twice with PBS. Then the cells were fixed with 4% paraformaldehyde phosphate buffer saline followed by phalloidin staining. The cell viability test was also demonstrated by using cell counting kit-8. The cell separation efficiency was calculated by undernoted formula.
Cell separation assay using 3D capillaries
The mixture of pKH-67 labelled PC-3 cells (10
000 cells per mL) and pKH-26 labelled LN-Cap cells (10
000 cells per mL) was loaded into the HVJ-E coated glass tube (9.5 mL h−1). Then, cells were cultured within the tube without flow for 4 hours at 37 °C. After incubation, cell culture medium was flowed to elute the cells from the tube (9.5 mL h−1). The eluted medium was collected in 96 well plates (50 μL per well). The number of cells was counted by fluorescence microscopy.
Results & discussion
Immobilization of HVJ-E on LbL films
There have been many reported methods to immobilize bioactive molecules or proteins on a substrate. For example, chemical crosslinking such as carbodiimide-crosslinker-chemistry is a versatile method for molecule/protein immobilization.14 However, this method is not suitable for the immobilization of HVJ-E as it is a fragile envelope and the inactivation of the surface protein must be avoided. Therefore, we choose the LbL assembly process for constructing the precursor film for HVJ-E immobilization because this procedure has already been used for many biological applications such as drug delivery,15–19 tissue engineering20,21 and surface modification for biomaterials.22,23 The LbL method also enables to achieve surface coating on a broad range of materials regardless of the geometries, such as capillary channel walls.24 To the best of our knowledge, there are few or no reports that examine the biological activities of envelope-type HVJ-E immobilized on LbL multilayer films. First, the precursor film for HVJ-E immobilization was constructed with an LbL assembly technique using PLL and ALG.
The assembly behavior was monitored by QCM and results are shown in Fig. S1 (in the ESI†). The increasing of frequency shifts showed the successful coating of LbL multilayer films on the QCM substrate. Next, HVJ-E particles were allowed to adsorb on the precursor films with different layer numbers. HVJ-E has anionic zeta potential in physiological pH.25 When HVJ-E adsorption was performed on the LbL film with a cationic layer (PLL) as the outmost layer, the adsorbed amount of HVJ-E increased with increasing layer thickness and reached a plateau at 13 layers and beyond (Fig. 2a). The adsorbed amount of HVJ-E on anionic ALG, on the other hand, decreased and no adsorption was observed on the film with a thickness of more than 12 layers. These results indicate that the surface coverages reached maximum and net charge densities become stable around 12 and 13 layers of LbL assembly for ALG and PLL respectively. Fig. 2b shows the adsorption kinetics for HVJ-E particles on the PLL surface with 11 layers at different HVJ-E concentrations monitored by QCM. The adsorbed amount of HVJ-E on the LbL surface increased with increasing incubation time as well as the HVJ-E concentration. It reached equilibrium at 60 minutes for 6000 HAU mL−1 of HVJ-E solution. This result indicates that the HVJ-E particles were successfully adsorbed on the LbL film as a monolayer. In general, it is difficult to immobilize biomolecules on polyelectrolyte layers as a monolayer because they tend to interact with each other, causing unfavourable aggregation. From this regard, we have previously examined the effects of salt concentration and pH on HVJ-E aggregation because the control of inter-particle electrostatic repulsion can contribute to the close packing of HVJ-E particles.25,26 The monolayer formation was also confirmed by Langmuir adsorption models (Fig. S2 in the ESI†).
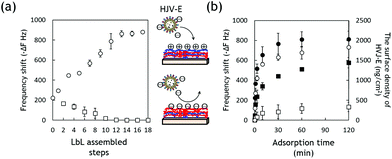 |
| Fig. 2 (a) The adsorption amounts of HVJ-E on the LbL multilayer with PLL (○) or ALG (□) outermost surface monitored by QCM (the concentration of HVJ-E is 6000 HAU mL−1). (b) The adsorption kinetics for HVJ-E particles on the PLL outermost surface with 11 LbL layers at different HVJ-E concentrations monitored by QCM. The concentrations of HVJ-E are 120 HAU mL−1 (■), 1200 HAU mL−1 (□), 3000 HAU mL−1 (●) and 6000 HAU mL−1 (○). | |
To confirm the HVJ-E adsorption visually, HVJ-E was labelled with a fluorescent dye pKH-268 and 6000 HAU mL−1 of HVJ-E was allowed to adsorb on the PLL with 13 layers for 120 minutes. Before HVJ-E adsorption, no red fluorescence was observed on the film without HVJ-E (Fig. S3a in the ESI†), whereas brilliant red emission was observed after the pKH-26 labelled HVJ-E adsorption (Fig. S3b†). AFM observation was also performed to analyze the HVJ-E immobilized surfaces. Fig. 3a–d represents the surface morphologies of the substrates with 0, 120, 1200, and 6000 HAU mL−1 of HVJ-E, respectively. Although the LbL layers were seldom seen, the number of islet-like structures increased with increasing concentration of HVJ-E. The height of the islet is approximately 120 nm as estimated in Fig. 3e, which represents a cross sectional line in Fig. 3d. This value agrees well with the almost half size of HVJ-E observed by DLS.25 The results suggested the successful adsorption of HVJ-E on the LbL surface.
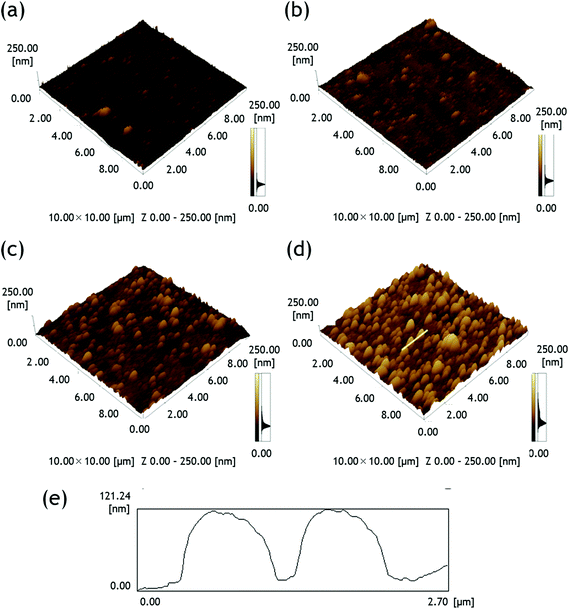 |
| Fig. 3 AFM images for the LbL substrate incubated with HVJ-E particles at the concentration of (a) 0, (b) 120, (c) 1200, and (d) 6000 HAU mL−1. (e) Cross sectional profile of the yellow line indicated in (d). | |
Surface contact angle measurements were also performed for the HVJ-E immobilized films (Fig. S4 in the ESI†). The surface contact angle of a substrate plays an important role in cell culture experiments and can affect the initial cellular attachment.27 Before the HVJ-E immobilizations, the PLL outermost layer had a contact angle of 50°. This value decreased to 30° when 6000 HAU mL−1 of HVJ-E was coated on the PLL surface. Thompson et al. has reported that about a thousand surface proteins of HN and F was located onto the Sendai virus surface envelope.28 The surface proteins of the Sendai virus have three domains, a hydrophilic external domain consisting mainly, hydrophilic structures of the external envelope, hydrophobic parts of the internal envelope and hydrophilic parts of the internal envelope.29 These literature studies indicate that when the external domain of HVJ-E is concentrated onto a substrate, the substrate becomes more hydrophilic.
Next, we have examined whether the surface viral protein activity was retained after adsorption by a haemolysis assay (Fig. S5 in the ESI†). Normally, enveloped viruses such as Sendai virus employ membrane fusion during cell penetration in order to deliver their genetic material across the cell boundary. The virus will also bind to red blood cells (RBCs), causing the formation of a lattice. This property is called hemagglutination, and is the basis of a rapid assay to determine levels of influenza virus present in a sample. The HN protein recognizes sialic acid-containing receptors on RBC surfaces and promotes the fusion activity of the F protein, allowing the virus to penetrate the cell surface. The internal haemoglobin is then released from the cells. Thus, RBC hemolysis assays were performed to evaluate the membrane disruptive activity of the immobilized HVJ-E. The degree of RBC membrane disruption was quantified by measuring the absorbance at 541 nm of the hemoglobin released into the solution by lysed cells.30,31 The results of this assay are detailed in Fig. S5a.† The glass surface shows the minimum haemolytic activity and the cells have an ellipsoidal disk shape as shown in Fig. S5b.† The PLL outermost surface show slightly higher hemolysis than does a glass surface. This increased hemolysis is likely due to the fact that polycationic polymers are used in biology to disrupt cell membranes and enhance the transport of materials into the cell.32 The HVJ-E immobilized surface, on the other hand, shows the maximum hemagglutinating activity among the samples tested in this study (Fig. S5d†). Of particular interest is that anionic charged RBCs attached very well to the anionic charged HVJ-E surface. This result indicates that HN proteins on HVJ-E successfully recognized sialic acid on RBCs. In order to further examine the viral protein activity of the immobilized HVJ-E particles, an inhibition assay was conducted in the presence of dithiothreitol (DTT) that can inactivate viral fusion by reducing the disulfide bond.33 As shown in Fig. S5a and e,† the DTT treated HVJ-E surface has a similar hemagglutinating activity to the glass surface, indicating that HVJ-E lost its haemolytic activity. These results clearly suggested that the immobilized HVJ-E still retains its viral protein activity on the LbL surface.
Selective adhesion of hormone resistant PC-3 cells on the HVJ-E immobilized surfaces
Since HVJ-E has GD1a receptors on the membrane, it is expected to interact with GD1a expressing cells preferentially. Kawaguchi et al. reported that the total amount of GD1a was much higher in hormone resistant PC-3 cells than LN-Cap cells.8 We have examined the selective adhesion of PC-3 on the HVJ-E immobilized surfaces. The PC-3 cells or LN-Cap cells were seeded and cultured onto FN-coated, PLL outermost LbL coated, and HVJ-E immobilized LbL coated glass coverslip for 72 hours, respectively. Fig. 4a shows phase contrast microscopy images of adhered cells on each substrate. LN-Cap cells adhered and spread well on the FN-coated glass substrate. Cells also spread to PLL outermost LbL coated substrate, although the number of cells is lower than that on the FN-glass. PC-3 cells show a similar trend to that of LN-Cap cells, although cell morphologies are different due to the nature of the PC-3 cells. Of particular interest is that PC-3 cells adhered well to the HVJ-E immobilized surfaces while LN-Cap cells aggregated and formed spheroids. This is because cells usually tend to form spheroidal structures on hydrophilic surfaces to minimize their surface energy as reported in many literature studies.34 Yet, LN-Cap cells possessed proliferative ability on the HVJ-E substrate even though the initial cell attachment was low (Fig. S6 in the ESI†). To investigate the cell–substrate interactions, adhered cells were subjected to washing after 72 hours of incubation. Fig. 4b shows fluorescent microscopy images of adhered cells on the corresponding substrates after washing. The results are quite obvious between LN-Cap and PC-3 cells on the HVJ-E immobilized surfaces. Almost all the spheroids of LN-Cap cells were washed out while many PC-3 cells still adhered to the surface (Fig. 4c). These results clearly indicate that the HVJ-E immobilized substrate has an ability to capture GD1a expressing cells more specifically.
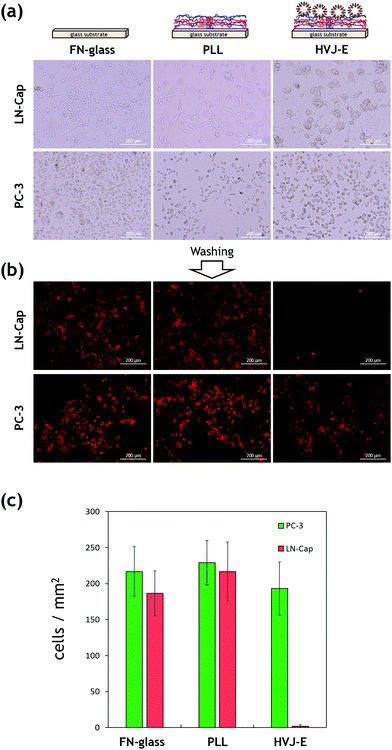 |
| Fig. 4 (a) Phase contrast microscopy images for LN-Cap and PC-3 cells on FN, PLL and HVJ-E coated glass substrates after 72 hours of incubation. (b) After washing with PBS, remaining cells were stained with phalloidin. (c) The number of remaining cells on the substrates were counted. | |
Next, we have examined the effects of immobilized HVJ-E densities and incubation time on the selective adhesion of PC-3 cells. For this experiment, a cell mixture of pKH-67 (green) labelled PC-3 cells and pKH-26 (red) labelled LN-Cap cells was seeded at the same time and cultured on the HVJ-E substrates with different immobilized amounts of HVJ-E for the times indicated. After the incubation, each substrate was washed twice with PBS. Fig. 5a shows fluorescent microscopy images of the mixture of PC-3 and LN-Cap cells adhered on each substrate after washing. For this experiment, we cultured cells only for 24 hours because both PC-3 and LN-Cap cells started to interact and aggregate together when they were co-cultured for 72 hours. The percentages of the adhered cell numbers were plotted against immobilized HVJ-E densities as in Fig. 5b. The results showed that the adsorption ratio of PC-3 to LN-Cap cells was approximately 50% in the case of the FN-coated glass and the PLL outermost LbL film, while approximately 60, 75, and 90% of adhered cells were PC-3 cells on the HVJ-E immobilized film of 1200, 3000, 6000 HAU mL−1, respectively. This result is plausible because the binding affinity between cells and HVJ-E would increase with the HVJ-E density. Fig. 5c shows the effect of incubation time on the selective adhesion of PC-3 cells on the HVJ-E surface of 6000 HAU mL−1. Although a significant difference was not observed between 4 and 24 hours, there was a slight decrease of the efficiency with increasing cell culture time. This is mainly because unexpected LN-Cap cells adhesion increased with increasing time. Although PC-3 cells express more GD1a, LN-Cap cells also express a small amount of GD1a.8 Another reason is that viruses can infect cells through nonspecific mechanisms.35
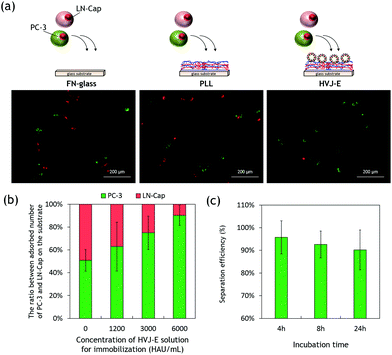 |
| Fig. 5 (a) The mixture of pKH-67 (green) labelled PC-3 and pKH-26 (red) labelled LN-Cap cells were seeded on FN, PLL and HVJ-E coated substrates and cultured for 24 hours. The substrates were then washed twice with PBS and observed by fluorescence microscopy. The effects of immobilized HVJ-E density (b) and incubation time (c) were also studied. | |
Isolation of hormone resistant PC-3 cells using HVJ-E immobilized glass capillaries
Since the proposed LbL deposition method is versatile and can be applied to a broad range of materials, we next performed the isolation of hormone resistant PC-3 cells using 3D capillaries (Fig. 6a). The successful HVJ-E immobilization was monitored by fluorescence microscopy using pKH-26 labelled HVJ-E immobilization (data not shown). 50 μL of cell suspensions (10
000 cells per mL) containing pKH-67 labelled PC-3 cells and pKH-26 labelled LN-Cap cells were injected into a glass capillary tube (1 mm in diameter) and incubated for 4 hours at 37 °C. Approximately 250 cells for each types of PC-3 and LN-Cap cells were loaded into the tube. After incubation, the tube was flushed with cell culture medium at a flow rate of 9.5 mL h−1. Fig. 6b summarizes the remaining cell numbers in the tube. After 20 seconds of continuous flowing, the remaining PC-3 and LN-Cap cells were approximately 160 and 120 cells, respectively. That is, approximately 64 and 48% of PC-3 and LN-Cap cells still remained in the tube. After 60 seconds, however, almost all the LN-Cap cells were flushed out from the tube, while 25% of PC-3 cells still remained. PC-3 cells were continuously eluted from the tube up to 150 seconds. This result indicates that LN-Cap cells were eluted in early fractions, while the interaction between PC-3 cells and the immobilized HVJ-E resulted in the delay of cell elution. Particularly, we achieved approximately 100% cell separation efficiency by 60 seconds of continuous flowing. Note that viability of the eluted cells was preserved to close to 90%.
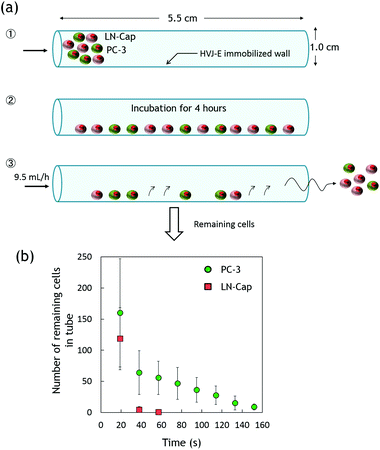 |
| Fig. 6 (a) Schematic procedures for the cell separation system with HVJ-E coated glass tube. First, the mixture of PC-3 and LN-Cap cells was loaded into the tube and incubated for 4 hours at 37 °C. Then, the tube was washed with a cell culture medium at a flow rate of 9.5 mL h−1. (b) The remaining cell numbers were plotted against flow time. | |
Conclusions
In summary, this study demonstrated a novel approach for isolating hormone resistant cancer cells using a glass capillary tube modified with HVJ-E particles. The precursor film inside the tube wall was created by LbL assembly. The monolayer adsorption of HVJ-E particles was successfully performed on the precursor LbL films without changing the biological activity of HVJ-E. To the best of our knowledge, there are no reports that examine the biological activities of immobilized HVJ-E particles on LbL multilayer films because it has been extremely difficult to manipulate or functionalize fragile envelope-type HVJ-E particles. Only by 60 seconds of flowing, we achieved approximately 100% cell separation efficiency between PC-3 and LC-Cap cells which represent hormone resistant SCNCs and adenocarcinomas, respectively. The viability of the eluted cells was preserved to close to 90%. We believe that the proposed technique can be applied to a broad range of materials because the LbL assembly method can be regarded as a simple, versatile, and inexpensive bottom-up nanofabrication technique. This technique can be also applied in the field of regenerative medicine because human bone mesenchymal stem cells (hBMSCs) are known to express high amounts of GD1a after osteoblast differentiation.36 Moreover, HVJ-E has been known to induce apoptosis in cancer cells by activation of caspase 8. Therefore, our HVJ-E immobilized system could pave the way for a variety of applications including cancer therapy and regenerative medicine.
Acknowledgements
The authors would like to express their gratitude for a Grant-in-Aid for Young Scientists (A) (25702029) from the Ministry of Education, Culture, Sports, Science and Technology (MEXT), Japan. The authors would also like to acknowledge financial support by JSPS KAKENHI (Grant Number 264086). We also acknowledge RIKEN-cell bank for providing the two prostate cancer cell lines.
References
- F. H. Schröder, J. Hugosson, M. J. Roobol, T. L. J. Tammela, S. Ciatto, V. Nelen, M. Kwiatkowski, M. Lujan, H. Lilja, M. Zappa, L. J. Denis, F. Recker, A. Berenguer, L. Määttänen, C. H. Bangma, G. Aus, A. Villers, X. Rebillard, T. van der Kwast, B. G. Blijenberg, S. M. Moss, H. J. de Koning and A. Auvinen, N. Engl. J. Med., 2009, 360, 1320–1328 CrossRef PubMed.
- F. H. Schröder, J. Hugosson, M. J. Roobol, T. L. J. Tammela, S. Ciatto, V. Nelen, M. Kwiatkowski, M. Lujan, H. Lilja, M. Zappa, L. J. Denis, F. Recker, A. Páez, L. Määttänen, C. H. Bangma, G. Aus, S. Carlsson, A. Villers, X. Rebillard, T. van der Kwast, P. M. Kujala, B. G. Blijenberg, U.-H. Stenman, A. Huber, K. Taari, M. Hakama, S. M. Moss, H. J. de Koning and A. Auvinen, N. Engl. J. Med., 2012, 366, 981–990 CrossRef PubMed.
- A. J. Chang, K. a. Autio, M. Roach and H. I. Scher, Nat. Rev. Clin. Oncol., 2014, 11, 308–323 CrossRef PubMed.
- S. Tai, S. Yin, J. M. Squires, H. Zhang, W. K. Oh, C.-Z. Liang and J. Huang, Prostate, 2011, 71, 1668–1679 CrossRef CAS PubMed.
- D. J. Grignon, Mod. Pathol., 2004, 17, 316–327 CrossRef PubMed.
- M. Tanaka, Y. Suzuki, K. Takaoka, N. Suzuki, S. Murakami, O. Matsuzaki and J. Shimazaki, Int. J. Urol., 2001, 8, 431–436 CrossRef CAS PubMed.
- Y. Kaneda, T. Nakajima, T. Nishikawa, S. Yamamoto, H. Ikegami, N. Suzuki, H. Nakamura, R. Morishita and H. Kotani, Mol. Ther., 2002, 6, 219–226 CrossRef CAS PubMed.
- Y. Kawaguchi, Y. Miyamoto, T. Inoue and Y. Kaneda, Int. J. Cancer, 2009, 124, 2478–2487 CrossRef CAS PubMed.
- M. Kurooka and Y. Kaneda, Cancer Res., 2007, 67, 227–236 CrossRef CAS PubMed.
- T. Matsushima-Miyagi, K. Hatano, M. Nomura, L. Li-Wen, T. Nishikawa, K. Saga, T. Shimbo and Y. Kaneda, Clin. Cancer Res., 2012, 18, 6271–6283 CrossRef CAS PubMed.
- G. Decher, Science, 1997, 277, 1232–1237 CrossRef CAS.
- Y. Lvov, H. Haas, G. Decher, H. Moehwald, A. Mikhailov, B. Mtchedlishvily, E. Morgunova and B. Vainshtein, Langmuir, 1994, 10, 4232–4236 CrossRef CAS.
- G. Sauerbrey, Z. Phys., 1959, 155, 206–222 CrossRef CAS.
- Y. Mi, D. Lee, J. Kim, H. Park and W. Jong, J. Controlled Release, 2015, 205, 172–180 CrossRef PubMed.
- K. Ariga, Y. M. Lvov, K. Kawakami, Q. Ji and J. P. Hill, Adv. Drug Delivery Rev., 2011, 63, 762–771 CrossRef CAS PubMed.
- Z. Poon, J. B. Lee, S.
W. Morton and P. T. Hammond, Nano Lett., 2011, 11, 2096–2103 CrossRef CAS PubMed.
- J. Zhang, C. Li, Y. Wang, R.-X. Zhuo and X.-Z. Zhang, Chem. Commun., 2011, 47, 4457–4459 RSC.
- D. G. Shchukin and H. Möhwald, Chem. Commun., 2011, 47, 8730–8739 RSC.
- B. Thierry, P. Kujawa, C. Tkaczyk, F. M. Winnik, L. Bilodeau and M. Tabrizian, J. Am. Chem. Soc., 2005, 127, 1626–1627 CrossRef CAS PubMed.
- B. Thierry, F. M. Winnik, Y. Merhi and M. Tabrizian, J. Am. Chem. Soc., 2003, 125, 7494–7495 CrossRef CAS PubMed.
- M. Matsusaki, K. Kadowaki, Y. Nakahara and M. Akashi, Angew. Chem., Int. Ed., 2007, 46, 4689–4692 CrossRef CAS PubMed.
- T. Crouzier, L. Fourel, T. Boudou, C. Albigès-Rizo and C. Picart, Adv. Mater., 2011, 23, H111–H118 CrossRef CAS PubMed.
- R. Guillot, F. Gilde, P. Becquart, F. Sailhan, A. Lapeyrere, D. Logeart-Avramoglou and C. Picart, Biomaterials, 2013, 34, 5737–5746 CrossRef CAS PubMed.
- W. Li, E. Reátegui, M.-H. Park, S. Castleberry, J. Z. Deng, B. Hsu, S. Mayner, A. E. Jensen, L. V. Sequist, S. Maheswaran, D. a. Haber, M. Toner, S. L. Stott and P. T. Hammond, Biomaterials, 2015, 65, 93–102 CrossRef CAS PubMed.
- T. Okada, K. Uto, M. Sasai, C. M. Lee, M. Ebara and T. Aoyagi, Langmuir, 2013, 7384–7392 CrossRef CAS PubMed.
- K. Uto, K. Yamamoto, N. Kishimoto, M. Muraoka, T. Aoyagi and I. Yamashita, J. Mater. Chem., 2008, 18, 3876 RSC.
- M. Ebara, M. Yamato, T. Aoyagi, A. Kikuchi, K. Sakai and T. Okano, Biomacromolecules, 2004, 5, 505–510 CrossRef CAS PubMed.
- S. D. Thompson, W. G. Laver, K. G. Murti and a. Portner, J. Virol., 1988, 62, 4653–4660 CAS.
- G. Communie, T. Crépin, D. Maurin, M. R. Jensen, M. Blackledge and R. W. H. Ruigrok, J. Virol., 2013, 87, 7166–7169 CrossRef CAS PubMed.
- C. A. Lackey, N. Murthy, O. W. Press, D. A. Tirrell, A. S. Hoffman and P. S. Stayton, Bioconjugate Chem., 1999, 401–405 CrossRef CAS PubMed.
- M. Ui, Y. Tanaka, Y. Araki, T. Wada, T. Takei, K. Tsumoto, S. Endo and K. Kinbara, Chem. Commun., 2012, 48, 4737 RSC.
- D. Luo and W. M. Saltzman, Nat. Biotechnol., 2000, 18, 33–37 CrossRef CAS PubMed.
- F. Dallocchio, M. Tomasi and T. Bellini, Biochem. Biophys. Res. Commun., 1995, 36–41 CrossRef CAS PubMed.
- Y. Iwasaki, U. Takami, Y. Shinohara, K. Kurita and K. Akiyoshi, Biomacromolecules, 2007, 8, 2788–2794 CrossRef CAS PubMed.
- M. Pizzato, E. D. Blair, M. Fling, J. Kopf, a. Tomassetti, R. a. Weiss and Y. Takeuchi, Gene Ther., 2001, 8, 1088–1096 CrossRef CAS PubMed.
- S. Bergante, E. Torretta, P. Creo, N. Sessarego, N. Papini, M. Piccoli, C. Fania, F. Cirillo, E. Conforti, A. Ghiroldi, C. Tringali, B. Venerando, A. Ibatici, C. Gelfi, G. Tettamanti and L. Anastasia, J. Lipid Res., 2014, 55, 549–560 CrossRef CAS PubMed.
Footnote |
† Electronic supplementary information (ESI) available. See DOI: 10.1039/c5bm00375j |
|
This journal is © The Royal Society of Chemistry 2016 |
Click here to see how this site uses Cookies. View our privacy policy here.