DOI:
10.1039/C5RA14723A
(Paper)
RSC Adv., 2015,
5, 80844-80852
Synthesis, antimicrobial evaluation and molecular modeling of some novel phenothiazine derivatives†
Received
24th July 2015
, Accepted 14th September 2015
First published on 14th September 2015
Abstract
A series of novel phenothiazine derivatives with biologically active moieties were synthesized. Treatment of 3-oxo-3-(10H-phenothiazin-10-yl)propanenitrile (2) with carbon disulfide gave 3,3-dimercapto-2-(10H-phenothiazine-10-carbonyl)acrylonitrile (4) which reacted with different reagents to afford novel fused heterocyclic compounds. The newly synthesized compounds were examined for their antimicrobial activity. Among the tested compounds, compounds 2, 4, 5a,b and 13 showed the highest antimicrobial activity. Molecular modeling was evaluated and was in agreement with experimental biological activity results.
1. Introduction
The chemistry of phenothiazine provides a significant amount of related compounds that have a variety of biological processes. Synthetic phenothiazine and its related compounds are basically effective in the treatment of a number of medical conditions.1,2 They possess a wide spectrum of pharmacological/biological activities3–13 and several of their derivatives are in clinical use. Phenothiazine and its related compounds have been reported to possess various biological activities such as antimalarial,14 antipsychotropic,15 antimicrobial,16 antitubercular,17,18 antitumor19,20 and analgesic.21 Phenothiazine itself is found to be a worming agent for livestock. The pesticidal action of phenothiazine results from the fact that they affect the nervous system of insects by inhibiting the breakdown of acetylcholine.2 The carbonyl and the cyano functions of the cyanoacetamides are readily well positioned for reactions with common reagents to form a variety of heterocyclic derivatives. Cyanoacetamides are highly reactive compounds having an active methylene which can take part in a variety of condensation and substitution reactions. In recent years, a number of different quantum mechanical theories arose, for example the Density Functional Theory (DFT). It was considered an effective computational method for studying organic compounds.
The DFT method explains the chemical reactivity and orbitals, energy band gap, electronegativity, and dipole moment.22 Therefore, in the present work we aimed to synthesize new phenothiazine derivatives and perform evaluations for their anticipated antimicrobial activities.
2. Results and discussion
2.1. Chemistry
In the continuation of our research interests, we reported here the behavior of 3-oxo-3-(10H-phenothiazin-10-yl)propanenitrile (2) toward some bifunctional reagents as a facile and appropriate route to synthesize some heterocyclic compounds containing a phenothiazine moiety.
The cyanoacetamide 2 was synthesized from the reaction of phenothiazine (1) with a mixture of cyanoacetic acid and acetic anhydride (Scheme 1). Compound 2 was confirmed using both spectral and analytical data. The IR spectrum showed absorption bands at 2259 and 1679 cm−1 corresponding to CN and C
O, respectively. The 1H-NMR spectrum showed one singlet signal at δ 3.86 ppm attributable to CH2 protons of the cyanoacetyl group and a multiplet signal at δ 7.46–7.55 ppm due to the aromatic system. Also its mass spectrum showed a molecular ion peak at m/z = 266 (M)+ attributable to its correct molecular formula C15H10N2OS. Reaction of 2 with carbon disulfide afforded the non-isolable intermediate 3 which reacted in situ with dilute hydrochloric acid to afford 3,3-dimercapto-2-(10H-phenothiazine-10-carbonyl)acrylonitrile (4). The 1H-NMR spectrum of 4 revealed a singlet signal at δ 1.5 ppm attributable to two SH protons. Moreover, the mass spectrum proved the structure through the appearance of a molecular ion peak at m/z = 342 (M)+ corresponding to the correct molecular formula C16H10N2OS3 (Scheme 1).
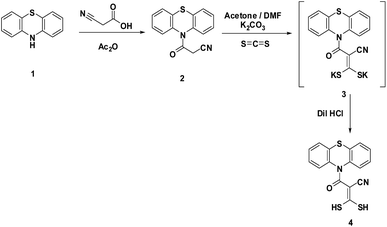 |
| Scheme 1 Reaction of compound 2 with carbon disulfide. | |
The intermediate 3 reacted with different halo-alkanes namely, 1-chlorododecane, 1-chlorohexadecane, 1,3-dibromopropane and 1,5-dibromopentane in a stirring mixture of acetone and N,N-dimethylformamide (1
:
2) containing anhydrous potassium carbonate as a base to afford dithia derivatives 5a,b and 6a,b, respectively (Scheme 2). Compound 5a was confirmed using both elemental analysis and spectral data. The 1H-NMR spectrum showed two triplet signals at δ 2.10 and 3.50 ppm corresponding to two identical CH3 groups and two identical S–CH2 groups, respectively. In addition, a broad signal at δ 3.30–3.89 ppm due to two (CH2)10 protons and a multiplet signal at δ 7.13–7.43 ppm corresponding to the aromatic system were seen. Moreover, the mass spectrum showed a molecular ion peak at m/z = 679, (M + 1)+ and 680 (M + 2)+ equivalent to the correct molecular formula C40H58N2OS3. The 1H-NMR spectrum of 5b revealed two triplet signals at δ 0.88 and 3.50 ppm corresponding to two CH3 and two SCH2 groups, respectively, in addition to a multiplet signal at δ 1.26–1.42 ppm due to two (CH2)14 protons and a multiplet signal at δ 7.30–7.67 ppm equivalent to the aromatic system. Moreover, the mass spectrum exhibited a molecular ion peak at m/z = 790 (M)+ confirming the correct molecular formula C48H74N2OS3. The 1H-NMR spectrum of 6a displayed a triplet signal at δ 2.89 ppm attributable to SCH2 protons and a broad signal centered at δ 3.50 ppm corresponding to CH2 protons. The mass spectrum indicated a molecular ion peak at m/z = 382 (M)+ confirming the molecular formula C19H14N2OS3 (Scheme 2). Compound 6b was established using both spectral and analytical data. The IR spectrum exhibited absorption frequencies at 2220 and 1698 cm−1 corresponding to CN and C
O groups, respectively. The 1H-NMR spectrum showed a triplet signal at δ 2.85 ppm corresponding to SCH2 protons, and a multiplet at δ 1.32–1.95 ppm corresponding to three CH2 protons. The mass spectrum showed a molecular ion peak at m/z = 410 (M)+ attributable to the correct molecular formula C21H18N2OS3. Furthermore, the reaction of intermediate 3 with an equimolar amount of dihaloalkanes under the same reaction conditions afforded compounds 7 and 8. The 1H-NMR spectrum of 7 revealed a singlet signal at δ 4.13 ppm corresponding to two SCH2 protons. Also, the mass spectrum showed a molecular ion peak at m/z = 443 (M − 1)+ corresponding to the molecular formula C24H16N2OS3. Structure 8 revealed absorption frequencies at 2239–2218 and 1720–1698 cm−1 attributable to three CN functions and three C
O functions, respectively. Also, the mass spectrum showed a molecular ion peak at m/z = 495 (M)+ corresponding to the molecular formula C24H8N4O3S3 (Scheme 2).
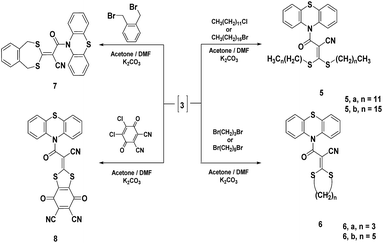 |
| Scheme 2 Reaction of intermediate 3 with dihalo-reagents. | |
In addition to that mentioned above, the reaction of intermediate 3 with two moles of 6-chloropyrimidine-2,4-diamine and/or 6-chloro-1,3,5-triazine-2,4-diamine and/or phenacyl bromide in a stirring mixture of acetone and N,N-dimethylformamide (1
:
2) containing anhydrous potassium carbonate as a base afforded the new phenothiazine derivatives 9–11 (Scheme 3). Compounds 9–11 were confirmed on the basis of spectral and analytical data. The IR spectra generally displayed absorption frequencies at 3398–3210, 2220–2192 and 1644–1721 cm−1 corresponding to NH2, CN and C
O functions, respectively. The 1H-NMR spectrum of compound 9 revealed three singlet signals at δ 4.48, 6.33 and 6.82 ppm corresponding to two C5–H pyrimidine protons and four NH2 protons, respectively. Also, the mass spectrum showed a molecular ion peak at m/z = 556 (M − 2)+ corresponding to the molecular formula C24H18N10OS3. In addition, the 1H-NMR spectrum of compound 10 revealed two singlet signals at δ 6.94 and 7.12 ppm corresponding to four NH2 functions. Also, the mass spectrum showed a molecular ion peak at m/z = 560 (M)+ equivalent to the molecular formula C22H16N12OS3. The 1H-NMR spectrum of compound 11 showed two singlet signals at δ 4.48 and 5.93 ppm consistent with SCH2 and NH2 protons, respectively. The mass spectrum revealed a molecular ion peak at m/z = 578 (M)+ due to the molecular formula C32H22N2O3S3. On the other hand, the reaction of the intermediate 3 with hydrazine hydrate in a stirring mixture of acetone and N,N-dimethylformamide (1
:
2) containing potassium carbonate as a base at room temperature, afforded compound 12. The IR spectrum revealed absorption frequencies at 3410, 3398, 3111, 3096 and 1698 cm−1 corresponding to two NH2, two NH and C
O functions, respectively. Moreover, the 1H-NMR spectrum revealed four singlet signals at δ 5.98, 6.27, 8.46 and 12.33 ppm corresponding to two NH2 and two NH protons. The mass spectrum displayed a molecular ion peak at m/z = 338 (M)+ corresponding to the molecular formula C16H14N6OS (Scheme 3).
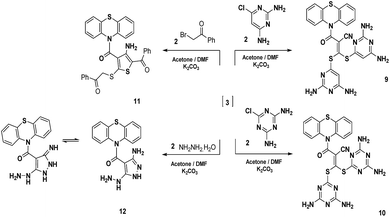 |
| Scheme 3 Reaction of intermediate 3 with haloreagents and hydrazine hydrate. | |
Furthermore, the reaction of 3 with chloroacetyl chloride in a stirring mixture of acetone and N,N-dimethylformamide (1
:
2) containing anhydrous potassium carbonate as a base afforded compound 13. Treatment of compound 13 with methyl iodide in the presence of sodium ethoxide gave the acyclic compound 14 which underwent cyclization to afford compound 15 (Scheme 4). Compounds 13–15 were established on the bases of spectral and analytical data. Compound 13 displayed absorption frequencies at 2218, 1710 and 1694 cm−1 corresponding to CN and two C
O functions. The 1H-NMR spectrum of 13 revealed a singlet signal at δ 3.85 ppm corresponding to CH2 of the dithiolanone ring. Also, the mass spectrum showed the molecular ion peak at m/z = 381 (M)+ attributable to the correct molecular formula C18H10N2O2S3. The 1H-NMR spectrum of compound 14 showed two singlet signals at δ 2.80 and 3.98 ppm attributable to SCH3 and SCH2 protons, a triplet signal at δ 1.25 and a quartet at δ 4.13 ppm corresponding to CH3 and OCH2 protons, respectively. The mass spectrum showed a molecular ion peak at m/z = 442 (M)+ equivalent to the molecular formula C21H18N2O3S3. In addition, the 1H-NMR spectrum of 15 revealed two singlet signals at δ 2.51 and 5.82 ppm corresponding to SCH3 and NH2 protons. Also, the mass spectrum showed a molecular ion peak at m/z = 442 (M)+ due to the molecular formula C21H18N2O3S3 (Scheme 4). Also, the intermediate 3 reacted with dimethyl sulfoxide in a stirring mixture of acetone and N,N-dimethylformamide (1
:
2) containing potassium carbonate as a base at room temperature, to afford the S-alkyl derivative 16. Compound 16 was established by both spectral and analytical data. The 1H-NMR spectrum of compound 16 revealed a singlet signal at δ 2.37 ppm corresponding to two SCH3 protons. The mass spectrum showed a molecular ion peak at m/z = 371 (M + 1)+ confirming the molecular formula C18H14N2OS3. When compound 16 was heated with 6-aminothiouracil in refluxing DMF, this afforded the corresponding pyridopyrimidine derivative 17. Structure 17 was suggested for the reaction product on the basis of elemental analysis and spectral evidence. The IR spectrum revealed absorption bands at 3410, 3120, 3098, 1698 and 1680 cm−1 corresponding to NH2, two NH and two C
O functions. Moreover, the 1H-NMR spectrum revealed four singlet signals at δ 2.54, 6.35, 11,47 and 12.33 ppm corresponding to SCH3, NH2 and two NH protons. In addition to, the mass spectrum showed a molecular ion peak at m/z = 465 (M)+ equivalent to the molecular formula C21H15N5O2S3 (Scheme 4).
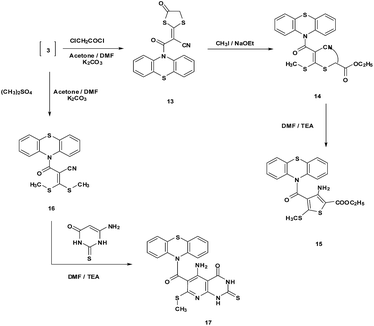 |
| Scheme 4 Reaction of intermediate 3 with chloroacetyl chloride/methyl iodide and dimethyl sulphate/6-aminothiouracil. | |
2.2. Biological activity
The newly synthesized target compounds were evaluated for their in vitro antibacterial activity against S. aureus and B. subtilis as examples of Gram-positive bacteria and E. coli and P. aeruginosa as examples of Gram-negative bacteria. They were also evaluated for their in vitro antifungal potential against Candida albicans and F. oxysporum fungal strains.
The disk diffusion technique was used for the determination of the antibacterial and antifungal activity. Ampicillin and clotrimazole were used as reference drugs. The MIC (μg mL−1) values were recorded in Table 1. The results depicted in Table 1 revealed that most of the tested compounds displayed variable inhibitory effects on the growth of the tested Gram-positive and Gram-negative bacterial strains, and also against the antifungal strains. Generally most of the tested compounds revealed better activity against the Gram-positive rather than the Gram-negative bacteria.
Table 1 Minimal inhibitory concentration (MIC, μg mL−1) of some newly synthesized compoundsa
Compound no. |
MIC in (μg mL−1) |
Bacteria |
Fungi |
Gram-negative bacteria |
Gram-positive bacteria |
E. coli |
P. aeruginosa |
S. aureus |
B. subtilis |
C. albicans |
F. oxysporum |
MIC: minimal inhibitory concentration values with SEM = 0.02, NA: no activity. |
2 |
125 |
125 |
125 |
125 |
250 |
125 |
4 |
125 |
125 |
125 |
125 |
125 |
62.5 |
5a |
125 |
62.5 |
62.5 |
62.5 |
NA |
NA |
5b |
125 |
62.5 |
57.5 |
62.5 |
NA |
NA |
6a |
NA |
NA |
125 |
125 |
NA |
NA |
6b |
NA |
NA |
NA |
NA |
NA |
NA |
7 |
125 |
62.5 |
125 |
125 |
250 |
125 |
8 |
125 |
125 |
125 |
62.5 |
62.5 |
31.5 |
9 |
125 |
125 |
125 |
125 |
125 |
125 |
10 |
125 |
125 |
187.5 |
125 |
125 |
125 |
11 |
125 |
62.5 |
125 |
125 |
NA |
NA |
12 |
250 |
125 |
250 |
125 |
250 |
125 |
13 |
31.5 |
62.5 |
NA |
NA |
NA |
NA |
15 |
125 |
62.5 |
187.5 |
125 |
3.9 |
3.2 |
17 |
250 |
250 |
250 |
250 |
250 |
125 |
Ampicillin |
125 |
125 |
187.5 |
125 |
NA |
NA |
Clotrimazole |
NA |
NA |
NA |
NA |
5.8 |
4.2 |
Regarding structure–activity relationships, it was revealed that compounds 2 and 4 which contain the cyanoacetamide moiety were equipotent to ampicillin in inhibiting the growth of E. coli and P. aeruginosa (MIC = 125 μg mL−1), while their activities were 33% more than that of ampicillin against S. aureus (MIC = 125 μg mL−1). Compound 13 recorded an increase in its activity by 75% more than ampicillin against E. coli (MIC = 31.5 μg mL−1) and 50% more than P. aeruginosa (MIC = 62.5 μg mL−1). Compounds 5a and b showed an increase in their activity by more than 66% (MIC = 62.5 μg mL−1) and 69%, (MIC = 57.5 μg mL−1), respectively, over ampicillin against S. aureus while they showed 50% (MIC = 62.5 μg mL−1) more activity than B. subtilis. On the other hand, compound 17 showed very low activity toward most of the tested organisms.
Moreover, the experimental results for the newly synthesized compounds were in agreement with theoretical data as shown by determining the energy gap (EHOMO–ELUMO) which is an indication of the biological activity. Since molecules with a small energy gap are more polarized and reactive than hard ones, they easily offer electrons to an acceptor. Also, the low values for the energy gap may be due to groups entering into conjugation with those soft molecules.23–25 Therefore, the energy gap for the compounds with the three highest antimicrobial activities (4, 5b and 13; Table 1) were determined (Table 2). The energy gap of compounds 5b and 13 (Fig. 2 and 3, respectively) were smaller than that of compound 4 (Fig. 1) indicating the ease of charge transfer in compounds 5b and 13 which enhanced their biological activity compared to compound 4 and are in agreement with the experimental data in Table 1. Table 2 shows the HOMO–LUMO energy gap, dipole moment and binding energy of compounds 5b and 13 computed using DFT, BLYP energy functional and the basis set DNP. Compound 13 has the most potent activity against the Gram-negative bacteria, E. coli and P. aeruginosa . This could be related to the lowest value for the dipole moment for compound 13 compared to compounds 4 and 5b. The inverse correlation between the dipole moment and the activity of compound 13 could be clarified in the concept that decreasing the dipole moment will decrease the polarity and increase the lipophilic nature of the compound which favours its permeation more efficiently through the lipid layer of a microorganism.26 The negative results can be attributed either to the inability of these compounds to diffuse through the cell wall of the bacterium and hence are unable to interfere with its biological activity or they can diffuse and are inactivated by an unknown cellular mechanism i.e. bacterial enzymes.27
Table 2 Calculated total energy and binding energy (BLYP/DNP) for compounds (4, 5b and 13) using DFT
Compound |
HOMO–LUMO energy gap (eV) |
Dipole moment Debye |
Binding energy, Hartree |
4 |
−1.877 |
2.1151 |
−6.3781553 |
5b |
−2.167 |
5.6224 |
−6.7501331 |
13 |
−2.488 |
1.9024 |
−20.8448839 |
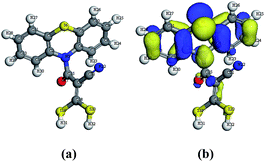 |
| Fig. 1 (a) Molecular geometry of compound 4 and (b) calculated HOMO superimposed on the isosurface of the molecular geometry of compound 4. | |
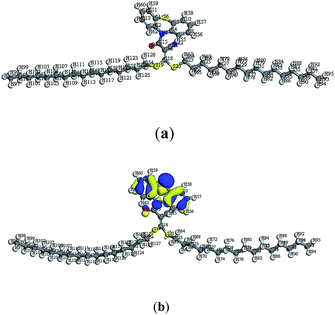 |
| Fig. 2 (a) Molecular geometry of conformer 5b and (b) calculated HOMO superimposed on the isosurface of the molecular geometry of conformer 5b. | |
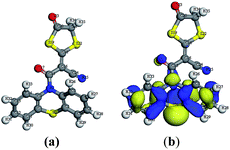 |
| Fig. 3 (a) Molecular geometry of conformer 13 and (b) calculated HOMO superimposed on the isosurface of the molecular geometry of conformer 13. | |
Also, the isolated compound 13 showed large binding energy values compared to compounds 4 and 5b, which improved the stability of this compound.28
Regarding the activity of the compounds against antifungal strains, the results revealed that compound 15 was 32.7% (MIC = 3.9 μg mL−1) and 24% (MIC = 3.2 μg mL−1) higher than clotrimazole in inhibiting the growth of C. albicans and F. oxysporum, respectively. Compounds 2, 4, 7, 8, 9, 10, 12 and 17 exhibited moderate growth inhibitory activity against C. albicans and F. oxysporum as revealed from their MIC values (MIC = 31.5–250 μg mL−1).
Since the tested compounds were more active against Gram-positive than Gram-negative bacteria, it may be concluded that the antimicrobial activity of the compounds is related to the cell wall structure of the bacteria. It is possible because the cell wall is essential to the survival of bacteria and some antibiotics are able to kill bacteria by inhibiting a step in the synthesis of peptidoglycan. Gram-positive bacteria possess a thick cell wall containing many layers, consisting of a few layers of peptidoglycan surrounded by a second lipid membrane containing peptidoglycan and teichoic acids, but in contrast, Gram-negative bacteria have a relatively thin cell wall of lipopolysaccharides and lipoproteins. These differences in cell wall structure can produce differences in antibacterial susceptibility and some antibiotics can kill only Gram-positive bacteria and are inactive against Gram-negative pathogens.29
In conclusion, the present study describes the synthesis and investigates the antimicrobial activities of some newly functionalized phenothiazine derivatives. Also, the HOMO, LUMO, dipole moment and charges on the atoms were used to confirm the geometry of the isolated compounds. Moreover, the compounds were screened for antimicrobial activity. Regarding the structure–activity relationship, compounds 5a and b exhibited the highest biological activity toward Gram positive bacteria, while compound 13 was the highest toward Gram negative bacteria. On the other hand, compound 15 showed the highest activity against pathogenic fungi by C. albicans and F. oxysporum using the MIC method.
2.3. Molecular modeling
All molecular geometries were fully optimized using the DMOL3 program30 implemented in Materials Studio 7.0 (Accelrys, CA).31 DMOL3, and BLYP energy functional with the basis set DNP were performed. DFT semi-core pseudopods calculations (dspp) were performed with the double numerical basis sets plus polarization functional (DNP). This basis set was comparable in quality to Gaussian 6-31G sets.32,33 Delley et al. showed that the DNP basis sets were more accurate than Gaussian basis sets of the same size.34 The RPBE functional was so far the best exchange–correlation functional,35,36 based on the generalized gradient approximation (GGA), and was employed to take account of the exchange and correlation effects of electrons.
3. Experimental
3.1. Instruments
All melting points are recorded on a Gallenkamp electric melting point apparatus and are uncorrected. The IR spectra ν/cm−1 (KBr) were recorded on a Perkin Elmer Infrared Spectrophotometer Model 157, Grating. The 1H-NMR spectra were run on a Varian Spectrophotometer at 300 and 75 MHz, respectively, using tetramethylsilane (TMS) as an internal reference and DMSO-d6 as a solvent. The mass spectra (EI) were recorded at 70 eV with Kratos MS equipment and/or a Varian MAT 311 A Spectrometer. Elemental analyses (C, H and N) were carried out at the micro analytical center of Cairo University, Giza, Egypt, and the results were found to be in good agreement (±0.3%) with the calculated values.
3.1.1. Synthesis of 3-oxo-3-(10H-phenothiazin-10-yl)propanenitrile (2). Phenothiazine (1) (10 mmol) was fused with an equimolar amount of cyanoacetic acid (10 mmol) in acetic anhydride at 80–85 °C in a boiling water-bath for 40 min. The reaction mixture was left for 1 h and then filtered off. The solid product was washed with ethanol (20 mL) to produce 2 as white crystals. Yield: 90%, mp: 215–217 °C. IR (KBr): ν/cm−1 = 2259 (C
N), 1679 (C
O); 1H-NMR (DMSO-d6) δ (ppm) = 3.86 (s, 2H, CH2), 7.49–7.55 (m, 8H, Ar-H); MS (EI, 70 eV): m/z (%) = 266 (M+; 100), 198 (98), 167 (78), 96 (88); chemical formula: C15H10N2OS: (266.05): calcd: C, 67.65; H, 3.78; N, 10.52%; found: C, 67.82; H, 3.54; N, 10.91%.
3.1.2. Synthesis of 3,3-dimercapto-2-(10H-phenothiazine-10-carbonyl)acrylonitrile (4). To a stirred suspension of potassium carbonate in a mixture of acetone and DMF (2
:
1), N-cyanoacetyl phenothiazine (2) (10 mmol) was added. To the resulting solution, carbon disulfide (10 mmol) was added and the reaction mixture was stirred for 24 h at room temperature to give the nonisolable intermediate 3. The reaction mixture was poured onto crushed ice and neutralized by dilute HCl. The obtained solid product was collected by filtration, washed, dried and crystallized from ethanol to give compound 4.White crystals; yield 82%, mp 218 °C; IR (KBr): ν/cm−1 = 2218 (CN), 1692 (C
O), 1310 (C
S); 1H-NMR (DMSO-d6) δ (ppm) = 1.5 (s, 1H, SH), 7.40–7.70 (m, 8H, Ar-H); MS (EI, 70 eV): m/z (%) = 342 (M+; 65), 241 (34), 187 (45), 172 (26), 93 (100); chemical formula: C16H10N2OS3: (342.00); calcd: C, 56.12; H, 2.94; N, 8.18%; found: C, 56.17; H, 2.89; N, 8.24%.
3.2. General procedure for the synthesis of compounds 5a and b
To a stirred mixture of 3 (10 mmol), 1-chlorododecane and/or 1-chlorohexadecane (10 mmol) was added and stirred for 4 h. The reaction mixture was poured onto crushed ice and neutralized by dil. HCl. The obtained solid product was collected by filtration, washed, dried and crystallized from ethanol to give compounds 5a and b.
3.2.1. Synthesis of 3,3-bis(dodecylthio)-2-(10H-phenothiazine-10-carbonyl)acrylonitrile (5a). Beige crystals; yield 76%, mp 227 °C; IR (KBr): ν/cm−1 = 2216 (CN), 1720 (C
O), 1317 (C
S); 1H-NMR (DMSO-d6) δ (ppm) = 2.10 (t, 6H, 2CH3), 3.49 (t, 4H, 2SCH2), 3.50 (br, 40H, 2(CH2)10), 7.13–7.43 (m, 8H, Ar-H); MS (EI, 70 eV): m/z (%) = 680 (M+ + 2) (18), 391 (34), 327 (21), 276 (47), 77 (100); chemical formula: C40H58N2OS3: (678.37): calcd: C, 70.75; H, 8.61; N, 4.13%; found: C, 70.82; H, 8.58; N, 4.19%.
3.2.2. Synthesis of 3,3-bis(hexadecylthio)-2-(10H-phenothiazine-10-carbonyl)acrylonitrile (5b). White crystals; yield 72%, mp 218 °C; IR (KBr): ν/cm−1 = 2220 (CN), 1680 (C
O), 1310 (C
S).; 1H-NMR (DMSO-d6) δ (ppm) = 0.88 (t, 6H, 2CH3), 1.26–1.42 (m, 56H, 2(CH2)14), 3.50 (t, 4H, 2SCH2), 7.30–7.67 (m, 8H, Ar-H); MS (EI, 70 eV): m/z (%) = 790 (M+) (65), 636 (22), 520 (28), 401 (18), 327 (48), 276 (34), 180 (17), 77 (100); chemical formula: C48H74N2OS3: (790.50): calcd: C, 72.86; H, 9.43; N, 3.54%; found: C, 72.92; H, 9.39; N, 3.58%.
3.3. General procedure for the synthesis of compounds 6a and b
To a stirred mixture of 3 (10 mmol), 1,3-dibromopropane and/or 1,5-dibromopentane (10 mmol) was added and stirred for 4 h. The reaction mixture was poured onto crushed ice and neutralized by dil. HCl. The obtained solid product was collected by filtration, washed, dried and crystallized from ethanol to give compounds 6a and b.
3.3.1. Synthesis of 2-(1,3-dithian-2-ylidene)-3-oxo-3-(10H-phenothiazin-10-yl)propanenitrile (6a). Orange crystals; yield 68%, mp 289 °C; IR (KBr): ν/cm−1 = 2221 (CN), 1712 (C
O), 1314 (C
S); 1H-NMR (DMSO-d6) δ (ppm) = 2.89 (t, 4H, 2SCH2), 3.50 (br, 2H, CH2), 6.74–7.67 (m, 8H, Ar-H); MS (EI, 70 eV): m/z (%) = 382 (M+) (18), 319 (100), 289 (34), 160 (28), 121 (18), 77 (34); chemical formula: C19H14N2OS3: (382.03): calcd: C, 59.66; H, 3.69; N, 7.32%; found: C, 59.72; H, 3.72; N, 7.38%.
3.3.2. Synthesis of 2-(1,3-dithiolan-2-ylidene)-3-oxo-3-(10H-phenothiazin-10-yl)propanenitrile (6b). Yellow crystals; yield 62%, mp 272 °C; IR (KBr): ν/cm−1 = 2220 (CN), 1698 (C
O), 1321 (C
S); 1H-NMR (DMSO-d6) δ (ppm) = 1.32–1.95 (m, 6H, 3CH2), 7.32–7.72 (m, 8H, Ar-H); MS (EI, 70 eV): m/z (%) = 410 (M+) (21), 346 (27), 279 (42), 175 (58), 77 (100), 65 (78); chemical formula: C21H18N2OS3: (410.06): calcd: C, 61.43; H, 4.42; N, 6.82%; found: C, 61.46; H, 4.38; N, 6.86%.
3.4. General procedure for the synthesis of compounds 7 and 8
To a stirred mixture of 3 (10 mmol), 1,2-bis(bromomethyl)benzene (10 mmol) and/or 4,5-dichloro-3,6-dioxocyclohexa-1,4-diene-1,2-dicarbonitrile (10 mmol) was added and stirred for 4 h. The reaction mixture was poured onto crushed ice and neutralized by dil. HCl. The obtained solid product was collected by filtration, washed, dried and crystallized from ethanol to give compounds 7 and 8, respectively.
3.4.1. Synthesis of 2-(1,5-dihydrobenzo[e][1,3]dithiepin-3-ylidene)-3-oxo-3-(10H-phenothiazin-10-yl)propanenitrile (7). Brown crystals; yield 68%, mp 345 °C; IR (KBr): ν/cm−1 = 2222 (CN), 1698 (C
O); 1H-NMR (DMSO-d6) δ (ppm) = 4.13 (s, 4H, 2SCH2), 7.02–7.78 (m, 12H, Ar-H); MS (EI, 70 eV): m/z (%) = 443 (M+ − 1) (18), 304 (58), 246 (60), 155 (42), 123 (100); chemical formula: C24H16N2OS3: (444.04); calcd: C, 64.84; H, 3.63; N, 6.30%; found: C, 64.92; H, 3.68; N, 6.26%.
3.4.2. Synthesis of 2-(1-cyano-2-oxo-2-(10H-phenothiazin-10-yl)ethylidene)-4,7-dioxo-4,7-dihydro benzo[d][1,3]dithiole-5,6-dicarbonitrile (8). Beige crystals; yield 72%, mp 295 °C; IR (KBr): ν/cm−1 = 2239, 2220, 2218 (3CN), 1723, 1720, 1698 (3C
O); 1H-NMR (DMSO-d6) δ (ppm) = 6.89–7.02 (m, 8H, Ar-H); MS (EI, 70 eV): m/z (%) = 495 (M+) (48), 454 (64), 380 (72), 278 (68), 224 (60), 171 (100), 132 (74); chemical formula: C24H8N4O3S3: (495.98); calcd: C, 58.06; H, 1.62; N, 11.28%; found: C, 57.96; H, 1.89; N, 11.32%.
3.5. General procedure for the synthesis of compounds 9, 10, 12 and 16
To a stirred mixture of 3 (10 mmol), 6-chloropyrimidine-2,4-diamine (20 mmol) and/or 6-chloro-1,3,5-triazine-2,4-diamine (20 mmol) and/or hydrazine hydrate (20 mmol) and/or dimethyl sulfoxide (20 mmol) was added and stirred for 4 h. The reaction mixture was poured onto crushed ice and neutralized by dil. HCl. The obtained solid product was collected by filtration, washed, dried and crystallized from ethanol to give compounds 9, 10, 12 and 16, respectively.
3.5.1. Synthesis of 3,3-bis((2,6-diaminopyrimidin-4-yl)thio)-2-(10H-phenothiazine-10-carbonyl)acrylonitrile (9). Beige crystals; yield 60%, mp 289 °C; IR (KBr): ν/cm−1 = 3397, 3315, 3309, 3210 (4NH2), 2192 (CN), 1644 (C
O); 1H-NMR (DMSO-d6) δ (ppm) = 4.48 (s, 2H, C5–H pyrimidine), 6.33 (s, 4H, 2NH2), 6.82 (s, 4H, 2NH2), 7.70–7.78 (m, 8H, Ar-H); MS (EI, 70 eV): m/z (%) = 556 (M+ − 2) (52), 473 (18), 304 (59), 246 (78), 155 (48), 123 (100), 91 (78); chemical formula: C24H18N10OS3: (558.08): calcd: C, 51.60; H, 3.25; N, 25.07%; found: C, 51.59; H, 3.22; N, 24.97%.
3.5.2. Synthesis of 3,3-bis((4,6-diamino-1,3,5-triazin-2-yl)thio)-2-(10H-phenothiazine-10-carbonyl)acrylonitrile (10). White crystals; yield 58%, mp 335 °C; IR (KBr): ν/cm−1 = 3421, 3402, 3398, 3318 (4NH2), 2198 (CN), 1689 (C
O); 1H-NMR (DMSO-d6) δ (ppm) = 6.94 (s, 4H, 2NH2), 7.12 (s, 4H, 2NH2), 7.30–7.69 (m, 8H, Ar-H); MS (EI, 70 eV): m/z (%) = 560 (M+) (38), 453 (28), 332 (29), 266 (36), 198 (100), 156 (32), 80 (67); chemical formula: C22H16N12OS3: (560.07): calcd: C, 47.13; H, 2.88; N, 29.98%; found: C, 47.29; H, 2.94; N, 30.02%.
3.5.3. Synthesis of (5-hydrazinyl-3-imino-2,3-dihydro-1H-pyrazol-4-yl)(10H-phenothiazin-10-yl)methanone (12). Beige crystals; yield 63% mp 316 °C; IR (KBr): ν/cm−1 = 3410, 3398 (2 NH2), 3096 (NH), 1698 (C
O).1H-NMR (DMSO-d6) δ (ppm) = 5.98 (s, 2H, NH2), 6.27 (s, 2H, NH2), 7.34–7.75 (m, 8H, Ar-H), 8.46 (s, 1H, NH), 12.33 (s, 1H, NH); MS (EI, 70 eV): m/z (%) = 338 (M+) (72), 289 (87), 250 (100), 182 (62), 75 (78); chemical formula: C16H14N6OS: (338.03): calcd: C, 58.35; H, 3.81; N, 7.56%; found: C, 58.42; H, 3.79; N, 7.58%.
3.5.4. Synthesis of 3,3-bis(methylthio)-2-(10H-pheno thiazine-10-carbonyl)acrylonitrile (16). Red crystals; yield 63%, mp 316 °C; IR (KBr): ν/cm−1 = 2228 (CN), 1680 (C
O); 1H-NMR (DMSO-d6) δ (ppm) = 2.37 (s, 6H, 2SCH3), 7.34–7.75 (m, 8H, Ar-H); MS (EI, 70 eV): m/z (%) = 371 (M+) (12), 346 (58), 318 (49), 262 (12), 174 (69), 69 (100); chemical formula: C18H14N2OS3: (370.03): calcd: C, 58.35; H, 3.81; N, 7.56%; found: C, 58.42; H, 3.79; N, 7.58%.
3.5.5. Synthesis of 2-(3-amino-5-((2-oxo-2-phenyl ethyl)thio)-4-(10H-phenothiazine-10-carbonyl)thiophen-2-yl)-1-phenylethan-1-one (11). To a stirred mixture of 3 (10 mmol), phenacyl bromide (20 mmol) was added and stirred for 4 h. The reaction mixture was refluxed in DMF which contained a catalytic amount of TEA for 6 h. The reaction mixture was allowed to cool to room temperature then poured onto cold ice water and neutralized with conc. HCl. The solid obtained was filtered off and recrystallized from ethanol to yield compound 11.Brown crystals; yield 68%, mp 345 °C; IR (KBr): ν/cm−1 = 3398 (NH2), 1721, 1712, 1689 (3C
O); 1H-NMR (DMSO-d6) δ (ppm) = 4.48 (s, 2H, SCH2), 5.93 (s, 2H, NH2), 7.41–7.75 (m, 18H, Ar-H); MS (EI, 70 eV): m/z (%) = 578 (M+) (24), 169 (37), 135 (23), 112 (48), 92 (100); chemical formula: C32H22N2O3S3: (578.08); calcd: C, 66.41; H, 3.83; N, 4.84%; found: C, 66.46; H, 3.79; N, 4.88%.
3.5.6. Synthesis of 3-oxo-2-(4-oxo-1,3-dithiolan-2-ylidene)-3-(10H-phenothiazin-10-yl)propanenitrile (13). To a stirred mixture of 3 (10 mmol), phenacyl bromide (20 mmol) was added and stirred for 4 h. The reaction mixture was poured onto crushed ice and neutralized by dil. HCl. The obtained solid product was collected by filtration, washed, dried and crystallized from ethanol to give compound 13.Orange crystals; yield 70%, mp 226 °C; IR (KBr): ν/cm−1 = 2218 (CN), 1710 (C
O); 1H-NMR (DMSO-d6) δ (ppm) = 3.85 (s, 2H, CH2), 7.49–7.67 (m, 18H, Ar-H); MS (EI, 70 eV): m/z (%) = 318 (M+) (18), 281 (58), 217 (18), 179 (28), 154 (78), 70 (100); chemical formula: C18H10N2O2S3: (381.99): calcd: C, 56.53; H, 2.64; N, 7.32%; found: C, 56.62; H, 2.81; N, 7.28%.
3.5.7. Synthesis of ethyl-2-((2-cyano-1-(methylthio)-3-oxo-3-(10H-phenothiazin-10-yl)prop-1-en-1-yl)thio)acetate (14). To a solution of sodium ethoxide (prepared from 10 mmol sodium metal in 10 mL ethanol), methyl iodide (10 mmol) was added to compound 13 (10 mmol). The reaction mixture was poured onto crushed ice and neutralized by dil. HCl. The obtained solid product was collected by filtration, washed, dried and crystallized from ethanol to give compound 14.Orange crystals; yield 62%, mp 265 °C; IR (KBr): ν/cm−1 = 2220 (CN), 1710, 1698 (2C
O); 1H-NMR (DMSO-d6) δ (ppm) = 1.25 (t, 3H, CH3), 2.80 (s, 3H, SCH3), 3.98 (s, 2H, SCH2), 4.13 (q, 2H, OCH2), 7.32–7.81 (m, 8H, Ar-H); MS (EI, 70 eV): m/z (%) = 442 (M+) (18), 364 (37), 286 (18), 213 (29), 154 (78), 77 (100); chemical formula: C21H18N2O3S3: (442.05): calcd: C, 56.99; H, 4.10; N, 6.33%; found: C, 57.01; H, 4.14; N, 6.28%.
3.5.8. Synthesis of ethyl 3-amino-5-(methylthio)-4-(10H-phenothiazine-10-carbonyl)thiophene-2-carboxylate (15). Compound 14 (10 mmol) was refluxed in DMF which contained a catalytic amount of TEA for 6 h. The reaction mixture was allowed to cool to room temperature and was then poured onto cold ice water and neutralized with conc. HCl. The solid obtained was filtered off and recrystallized from ethanol to yield compound 15.Brown crystals; yield 60%, mp 290 °C; IR (KBr): ν/cm−1 = 3401 (NH2), 1720, 1698 (2C
O); 1H-NMR (DMSO-d6) δ (ppm) = 1.34 (t, 3H, CH3), 2.51 (s, 3H, SCH3), 4.40 (q, 2H, OCH2), 5.82 (s, 2H, NH2), 7.30–7.67 (m, 8H, Ar-H); MS (EI, 70 eV): m/z (%) = 442 (M+) (21), 364 (37), 316 (18), 288 (48), 211 (59), 169 (76), 92 (100); chemical formula: C21H18N2O3S3: (442.05): calcd: C, 56.99; H, 4.10; N, 6.33%; found: C, 57.01; H, 4.14; N, 6.28%.
3.5.9. Synthesis of 5-amino-7-(methylthio)-6-(10H-phenothiazine-10-carbonyl)-2-thioxo-2,3-dihydro pyrido[2,3-d]pyrimidin-4(1H)-one (17). A mixture of compounds 16 (10 mmol) and 6-aminothiouracil (10 mmol) were refluxed in DMF which contained a catalytic amount of TEA for 6 h. The reaction mixture was allowed to cool to room temperature and was then poured onto cold ice water and neutralized with conc. HCl. The solid obtained was filtered off and recrystallized from ethanol to yield compound 17.Brown crystals; yield 78% mp, over 350 °C; IR (KBr): ν/cm−1 = 3410 (NH2), 3120, 3098 (2NH), 1698, 1680 (2C
O); 1H-NMR (DMSO-d6) δ (ppm) = 2.54 (s, 3H, SCH3), 6.35 (s, 2H, NH2), 7.29–7.68 (m, 8H, Ar-H), 11.47 (s, 1H, NH), 12.33 (s, 1H, NH); MS (EI, 70 eV): m/z (%) = 465 (M+) (48), 262 (12), 217 (18), 174 (58), 108 (39), 69 (100); chemical formula: C21H15N5O2S3: (465.04); calcd: C, 54.18; H, 3.25; N, 15.04%; found: C, 54.21; H, 3.29; N, 14.98%.
3.6. Biological activity
3.6.1. Antibacterial assay. Antibacterial studies of the newly synthesized compounds were carried out against the representative panel of Gram-positive S. aureus and B. subtilis and Gram-negative E. coli and P. aeruginosa. The activity of the compounds was determined as per the National Committee for Clinical Laboratory Standards (NCCLS) protocol using Müller-Hinton Broth. Primary screening was done first for antibacterial activity in six sets against E. coli, and S. aureus at different concentrations of 1000, 500, and 250 μg mL−1. The compounds found to be active in the primary screening were similarly diluted to obtain concentrations of 200, 125, 100, 62.5, 50, 25, and 12.5 μg mL−1 for a secondary screening to test in a second set of dilutions against all microorganisms. The inoculum size for the test strain was adjusted to 106 CFU mL−1 (Colony Forming Unit per milliliter) by comparing the turbidity (turbidimetric method). The Müller-Hinton Broth was used as a nutrient medium to grow and dilute the compound suspension for test organisms. 2% DMSO was used as a diluent/vehicle to obtain the desired concentration of synthesized compounds and standard drugs to test on the standard microbial strains. Synthesized compounds were diluted to 1000 μg mL−1 concentration as a stock solution. The control tube containing no antibiotic was immediately sub cultured (before inoculation) by spreading a lapful evenly over quarter of plate of medium suitable for the growth of test organisms. The culture tubes were then incubated for 24 h at 36 °C and the growth was monitored visually and spectrophotometrically. 10 μg mL−1 suspensions were further inoculated on an appropriate media and growth was noted after 24 h and 48 h. The lowest concentration (highest dilution) required to arrest the growth of bacteria was regarded as the minimal inhibitory concentration (MIC) i.e. the amount of growth from the control tube before incubation (which represents the original inoculum) was compared. The solvent had no influence on strain growth. The result of this was greatly affected by the size of the inoculum. The test mixture should contain 106 CFU mL−1 of organisms. DMSO and sterilized distilled water were used as negative controls while Ampicillin antibiotic (IU strength) was used as a positive control. The standard drug used in the present study was “ampicillin” for evaluating antibacterial activity.
3.6.2. Antifungal assay. The newly prepared compounds were screened for their antifungal activity as a primary screening in six sets against Candida albicans and F. oxysporum at various concentrations of 1000, 500, and 250 μg mL−1. The primary active compounds were similarly diluted to obtain 200, 125, 100, 62.5, 50, 25, and 12.5 μg mL−1 concentrations for a secondary screening to test a second set of dilutions against fungi. The fungal activity of each compound was compared with clotrimazole as a standard drug, which showed a MIC of 5.8 μg mL−1 against C. albicans. For fungal growth, in the present protocol, we have used Sabouraud’s Dextrose Broth at 28 °C in aerobic condition for 48 h. DMSO and sterilized distilled water were used as negative controls while clotrimazole (IU strength) was used as a positive control.
References
- O. William Foye, Foye’s principles of medicinal chemistry, Lippincott William & Wilkins Publishers, 1960 Search PubMed.
- A. D. Mosnaim, V. V. Ranade, M. E. Wel, J. Puente and A. M. Valenzuela, Phenothiazine molecule provides the basic chemical structure for various classes of pharmacotherapeutic agents, Am. J. Therapeut., 2006, 13, 261–273 CrossRef PubMed.
- F. Galbraith and S. Smiles, The Rearrangement of o-Hydroxysdphones, J. Chem. Soc., 1935, 1234 RSC.
- E. D. Clercq, Targets for the antiviral and antitumor activities of nucleoside, nucleotide and oligonucleotide analogues, Nucleosides Nucleotides, 1985, 4, 3 Search PubMed.
- Phenothiazines and 1,4-Benzothiazines – Chemicals and Biomedical Aspects, ed. R. R. Gupta, Elsevier, Amsterdom, 1988 Search PubMed.
- A. Gupta, V. Saraswat, S. K. Mukhrji and R. R. Gupta, Synthesis of 5,8-dichloro-3-methyl-4H-194-benzothiazines and their conversion into sulfones phosphorus, Phosphorus, Sulfur Silicon Relat. Elem., 1993, 85, 101 CrossRef CAS PubMed.
- S. Shukla and L. Prakash, Nucleosides-ribosylation of some pyrido[2,3-d]pyrimidines and 10H-phenothiazines and their biocidal activity, Indian J. Heterocycl. Chem., 1995, 5, 41 CAS.
- N. Gautam, R. Gupta, D. C. Gautam and R. R. Gupta, Synthesis of 3-bromo-1-methylpheno thiazines by Smiles rearrangement, Heterocycl. Commun., 2000, 6, 369 CrossRef CAS.
- G. Kumar, V. Gupta, D. C. Gautam and R. R. Gupta, A convenient synthesis of 5/7-chloro-4H-1,4-benzothiazines, Heterocycl. Commun., 2002, 8, 381 CAS.
- N. Gautam and D. C. Gautam, Synthesis of 3-bromo-1-methyl phenothiazine sulfones, Int. J. Chem. Sci., 2004, 2(1), 84–87 CAS.
- N. Gautam, D. Hans and D. C. Gautam, Antifungal activity of some 4H-1,4-benzothiazine compounds, Orient. J. Chem., 2005, 21(2), 299–302 CAS.
- N. Gautam and D. C. Gautam, Synthesis of 7-Bromo-3,5-Dimethyl-4H-1,4-Benzothiazine Sulfones, Orient. J. Chem., 2006, 22(2), 457–460 CAS.
- V. Gautam, M. Sharma, A. Samarth, A. Kumar, I. K. Sharma, N. Gautam and D. C. Gautam, Synthesis of Some Substituted 10H-Phenothiazines, Ribofuranosides, and their Antioxidant Activity, Phosphorus, Sulfur Silicon Relat. Elem., 2007, 182(6), 1381 CrossRef CAS PubMed.
- J. N. Dominguez, S. Lopez, J. Charris, L. Iarruso, G. Lobo, A. Semenow, J. E. Olson and P. J. Rosenthal, Synthesis and Antimalarial Effects of Phenothiazine Inhibitors of a Plasmodium falciparum Cysteine Protease, J. Med. Chem., 1997, 40, 2726–2732 CrossRef CAS PubMed.
- G. Lin, K. K. Midha and E. M. Hawes, Synthesis of the piperidinone metabolites of piperidine type phenothiazine antipsychotic drugs via ruthenium tetroxide oxidation, J. Heterocycl. Chem., 1991, 28, 215 CrossRef CAS PubMed.
- J. Raval and K. K. Desai, 7. Synthesis and antimicrobial activity of new triazolopyridinyl phenothiazines, ARKIVOC, 2005, xiii, 21 Search PubMed.
- M. Viveros and L. Amaral, Design, Synthesis, characterization and antitubercular activity of some 2-heterocycle substituted phenothiazine, Int. J. Antimicrob. Agents, 2001, 17, 225 CrossRef.
- L. Amarl and J. E. Kristiansen, Phenothiazines: an alternative to conventional therapy for the initial management of suspected multidrug resistant tuberculosis, Int. J. Antimicrob. Agents, 2000, 173 CrossRef.
- N. Motohasho, M. Kawase, S. Saito and H. Sakagami, Phenothiazines: an alternative to conventional therapy for the initial management of suspected multidrug resistant tuberculosis, Curr. Drug Targets, 2000, 1, 237–245 CrossRef.
- N. Motohashi, M. Kawase, S. Saito, T. Kurihara, K. Satoh, H. Nakashima, M. Premanathan, R. Arakaki and H. Sakagami, Synthesis and biological activity of N-acylphenothiazines, Int. J. Antimicrob. Agents, 2000, 14(3), 203–207 CrossRef CAS.
- L. Amaral and J. E. Kristiansen, Phenothiazine: an alternative to conventional therapy for the initial management of suspected multidrug resistant tuberculosis. A call for studies, Int. J. Antimicrob. Agents, 2000, 14, 173–176 CrossRef CAS.
- H. Sahebalzamani, N. Khaligh, S. Ghammamy, F. Salimi and K. Mehrani, Crystal Structure and Density Functional Theory Study on Structural Properties and Energies of a Isonicotinohydrazide Compound, Molecules, 2011, 16, 7715 CrossRef CAS PubMed.
- A. A. Fadda, A. M. El Defrawy and S. A. El-Hadidiy, Synthesis Cytotoxicity Evaluation, DFT Molecular Modeling Studies and Quantitative structure Activity Relationship of Novel 1,8-Naphthyridines, Am. J. Org. Chem., 2012, 2(4), 87–96 CrossRef CAS PubMed.
- A. El-Shafei, A. A. Fadda, S. Bondock, A. M. Khalil and E. H. Tawfic, Facile synthesis, Pure DFT calculations and PM3 Semiempirical MO method validation of Regiospecificity of Novel 1,4-Dihydropyrido [2,3-d]pyrimidine derivatives, Synth. Commun., 2010, 40, 2788–2805 CrossRef CAS PubMed.
- A. El-Shafei, A. A. Fadda, I. I. Abdel-Gawad and E. H. E. Youssif, Stereospecificity of Diels-Alder Reaction Validated Using Ab Initio Calculations: Synthesis of Novel Coumarin and Phenanthridine Derivatives, Synth. Commun., 2009, 39, 2954–2972 CrossRef CAS PubMed.
- R. N. Jones, A. L. Barry, T. L. Gavan and I. I. J. A. Washington, in Manual of Clinical Microbiology, ed. E. H. Lennette, A. Ballows, W. J. Hausler Jr and H. J. Shadomy, Am. Soc. Microbiol., Washington DC, 4th edn, 1985 Search PubMed.
- A. S. El-Tabl, F. A. El-Saied and A. N. Al-Hakimi, Spectroscopic characterization and biological activity of metal complexes with an ONO trifunctionalized hydrazone ligand, J. Coord. Chem., 2008, 61, 2380–2401 CrossRef CAS PubMed.
- M. Aljahdali and A. A. EL-Sherif, Synthesis, characterization, molecular modeling and biological activity of mixed ligand complexes of Cu(II), Ni(II) and Co(II) based on 1,10-phenanthroline and novel thiosemicarbazone, Inorg. Chim. Acta, 2013, 407, 58–68 CrossRef CAS PubMed.
- H. Abou-Melha and A. A. Fadda, Synthesis, spectral characterization and in vitro antimicrobial activity of some new azopyridine derivatives, Spectrochim. Acta, Part A, 2012, 89, 123–128 CrossRef PubMed.
- B. Delley, Determination of effective permittivity and permeability of metamaterials from reflection and transmission coefficients, Phys. Rev. B: Condens. Matter Mater. Phys., 2002, 65, 85403–88509 CrossRef.
- http://accelrys.com/products/collaborative-science/biovia-materials-studio.
- Materials Studio (Version 5.0), Accelrys software Inc., San Diego, USA, 2009 Search PubMed.
- B. S. Jursic, The evaluation of nitrogen containing bond dissociation energies using the ab initio and density functional methods, J. Mol. Struct., 1996, 366, 103–108 CrossRef CAS.
- A. Kessi and B. Delley, Density functional crystal vs. cluster models as applied to zeolites, Int. J. Quantum Chem., 1998, 135–144 CrossRef CAS.
- B. Hammer, L. B. Hansen and J. K. Nørskov, Metal–insulator transition in a disordered two-dimensional electron gas including temperature effects, Phys. Rev. B: Condens. Matter Mater. Phys., 1999, 59, 7413–7421 CrossRef.
- A. Matveev, M. Staufer, M. Mayer and N. Rösch, Density functional study of small molecules and transition-metal carbonyls using revised PBE functionals, Int. J. Quantum Chem., 1999, 75, 863–873 CrossRef CAS.
Footnote |
† Electronic supplementary information (ESI) available. See DOI: 10.1039/c5ra14723a |
|
This journal is © The Royal Society of Chemistry 2015 |
Click here to see how this site uses Cookies. View our privacy policy here.