DOI:
10.1039/C5RA13961A
(Paper)
RSC Adv., 2015,
5, 95882-95893
The pharmacokinetics, bioavailability and excretion of columbianetin acetate and its metabolite columbianetin were analysed in rat plasma by LC-MS/MS after administration of columbianetin acetate and Angelicae pubescentis radix extract
Received
15th July 2015
, Accepted 22nd October 2015
First published on 22nd October 2015
Abstract
Angelicae pubescentis radix (APR) has been widely used in the clinic for the treatment of rheumatoid arthritis. Columbianetin acetate and its metabolite columbianetin have various biological activities. A sensitive, accurate and precise HPLC-MS/MS method was established for simultaneous determination of columbianetin acetate and columbianetin in rat plasma. It was found that columbianetin acetate was rapidly and widely distributed in rats, and eliminated rapidly from plasma. Columbianetin acetate could be metabolized into columbianetin in vivo. The absolute bioavailability of pure columbianetin acetate is 7.0 ± 4.3%. Other co-existing ingredients in the APR extract could increase the concentration of its metabolite columbianetin in plasma and this was caused by columbianetin-β-D-glucopyranoside. Cumulative excretion of columbianetin acetate in urine accounted for 0.0109 ± 0.0067% of the total dosage. The cumulative amounts of columbianetin acetate in the feces account for 9.32 ± 6.63% of the total dose. Columbianetin acetate was mainly excreted in the feces.
1. Introduction
Traditional Chinese medicines (TCMs) have been used clinically for preventing and treating diseases for thousands of years. By virtue of their clinical application over such a long period of time, a large amount of TCMs have been proven to be effective. Unlike chemical medicine, TCM is a complex system with various active ingredients in which one active ingredient can interact with the others. According to the results of several previous studies, there are significant differences between the pharmacokinetic properties of the active components in their pure forms and those in herbal medicines1,2 or even in formulas.3
Angelicae pubescentis radix (APR), the root of Angelica pubescens (Apiaceae) Maxim. f. biserrata Shan et Yuan is officially listed in the Chinese Pharmacopoeia (National Commission of Chinese Pharmacopoeia, 2010). APR has been widely used in the clinic for centuries as a known TCM for the treatment of rheumatoid arthritis both in Chinese herbal medicine4,5 and Chinese patent medicine.4 Research has shown that APR extracts exhibit anti-inflammatory and analgesic effects.6 The coumarins from APR could prolong the hypnotic duration of pentobarbital sodium.7 Additionally, there are reports that APR has been used clinically in the treatment of influenza A,8 migraines,9 osteoporosis,10 and strokes.11 Coumarins are the major bioactive components in APR.12,13 Columbianetin acetate is one of the major coumarins in APR,14 and columbianetin (Fig. 1) is a major bioactive coumarin. These two coumarins were both first isolated from APR in 1987.12
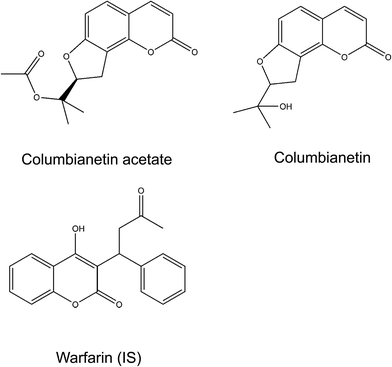 |
| Fig. 1 Chemical structures of columbianetin, columbianetin acetate and warfarin (IS). | |
According to reports in the literature, columbianetin acetate has been shown to have multiple biological activities including anti-tumor,15 anti-platelet formation,13 anti-inflammatory and analgesic properties.16 Columbianetin has also been found to inhibit platelet aggregation and lipid peroxidation,17 inhibit histamine release,18,19 inhibit activation of the viral-genome and the viability of Raji cells15 and possess anti-inflammatory activity19 and anti-pathogen effects.20 It was usually reported that some compounds may be metabolized to analogues in vivo after administration.21 Columbianetin acetate and columbianetin are analogues with the same mother nucleus. It was necessary to investigate the relationship between columbianetin acetate and columbianetin in vivo. At present, pharmacokinetic studies on columbianetin acetate after oral administration of APR extract in rats have been reported in our previous research.22 However, there is no report on the study of the in vivo pharmacokinetics, excretion and bioavailability of pure columbianetin acetate. To our knowledge, the influence of other co-existing ingredients in the APR extract on columbianetin acetate and columbianetin has also not been reported.
In the present study, a simple and sensitive high performance liquid chromatography-tandem mass spectroscopy (LC-MS/MS) method has been developed for the quantitative determination of columbianetin acetate and its metabolite columbianetin in rat plasma. The newly established LC-MS/MS method was successfully applied to the pharmacokinetics, oral bioavailability and excretion study after administration of columbianetin acetate and APR extract to rats.
2. Experimental
2.1. Chemicals and reagents
Methanol of HPLC grade, ethyl acetate and petroleum ether of analytical grade were purchased from Tianjin Concord Science Co. Ltd, (Tianjin, China). Acetonitrile of Chromatographic grade for liquid chromatography was purchased from Merck (Darmstadt, Germany). Ammonium acetate (purity > 99.99%) was purchased from Tianjin Guangfu Institute of Fine Chemicals (Tianjin, China). Warfarin (purity > 98%) was purchased from National Institute for the Control of Pharmaceutical and Biological Products (Beijing, China). APR was obtained from Anguo city (Hebei, China). The species were authenticated by Professor Lin Ma (Tianjin University of Traditional Chinese Medicine) and the voucher specimens were deposited at Tianjin University of Traditional Chinese Medicine, Tianjin, China. Columbianetin acetate (purity > 98%) was isolated from APR in our laboratory. Deionized water was purified with a Milli-Q Academic ultra-pure water system (Millipore, Milford, MA, USA).
2.2. Apparatus and conditions
Chromatographic separation was carried out on an Agilent 1200 series liquid chromatographic system (Agilent Technologies, Santa Clara, CA, USA) equipped with a G1322A degasser, G1312A Bin Pump, G1367B autosampler, G1316A thermostatic column compartment and an Agilent Eclipse Plus C18 (4.6 × 100 mm, 1.8 μm) column with a security guard C18 (2.1 mm × 12.5 mm, 5 μm) column. The column oven temperature was set at 20 °C. According to our previous study, acetonitrile (solvent system A) and water containing 1 mmol L−1 ammonium acetate (solvent system B) were selected as mobile phase components with a gradient elution of 40% A at 0–5 min, 40–70% A at 5–10 min, 70–75% A at 10–11 min, 75–90% A at 11–19 min, and 90% A at 19–23 min.22 The re-equilibration time of gradient elution was 5 min. The flow rate was set at 0.3 mL min−1 and the injection volume was 5 μL.
Mass spectroscopy data were acquired from an API 3200 tandem mass spectrometer (Concord, Ontario, Canada) with Analyst 1.4.2 software (AB MDS Sciex). The components were detected and quantified by electrospray ionization (ESI) in positive ion mode and multiple-reaction monitoring (MRM) mode, respectively. The spray voltage was at +5500 V. The turbo spray temperature was set at 400 °C. The collision gas, nebulizer gas (gas 1), auxiliary gas (gas 2) and curtain gas were optimized at 5, 6, 40 psi and 25 psi respectively. The precursor-to-product ion pairs, dwell time, declustering potential (DP), entrance potential (EP), collision energy (CE) and collision cell exit potential (CXP) for columbianetin, columbianetin acetate and warfarin (IS) were set according to previous studies.22,23
2.3. Preparation of the APR extract and isolation of columbianetin acetate
APR was air-dried, cut into pieces and soaked in 75% ethanol for 8 h in a separation funnel. The solvent level was maintained above the medicinal material even after swelling. The ratio of material to solvent was 1
:
8. After percolation, the ethanol extract was concentrated using a rotary evaporator under reduced pressure and dried out in a vacuum oven to get the APR extract. The APR extract was dissolved in water and extracted with ethyl acetate three times. HPLC showed that all the columbianetin acetate was in the ethyl acetate phase after the triple extraction. Six fractions were obtained from the ethyl acetate phase of the APR extract by normal-phase silica column chromatography (petroleum ether–ethyl acetate (4
:
1, v/v)). Fraction 5 was columbianetin acetate. Its purity was more than 98%.
2.4. Quantitative determination of columbianetin acetate in the APR extract
The concentration of columbianetin acetate in the APR extract was determined by HPLC-UV according to our previous studies.14 The powdered APR extract (102 mg) was accurately weighed in triplicate. The sample was extracted with 10 mL of methanol using an ultrasonic bath for 40 min and then cooled at room temperature. Methanol was added to compensate for the lost weight. The solution was centrifuged at 14
000 rpm for 10 min at 4 °C. The supernatant was transferred into vials for injection. Quantitative determination of columbianetin acetate in the APR extract was conducted using HPLC-UV (Agilent 1200 series liquid chromatographic system).
2.5. Preparation of stock and working solutions
Stock solutions of columbianetin and columbianetin acetate were both dissolved in methanol to a concentration of 1 mg mL−1. An internal standard warfarin solution was prepared in methanol at a concentration of 100 ng mL−1. Appropriate aliquots of the stock solutions of columbianetin and columbianetin acetate were mixed to prepare a mixed stock solution. To prepare the standard solutions for calibration and quality control samples, the mixed stock solution was serially diluted with methanol to obtain working stock solutions at final concentrations of 0.5, 1, 2, 5, 10, 50, 100, 500, 1000, 2000, and 5000 ng mL−1. All the solutions were stored at 4 °C and brought to room temperature before use.
2.6. Preparation of samples and quality control samples
100 μL of plasma samples and 10 μL of internal standard (IS) solutions were added to 1.5 mL Eppendorf tubes. Samples were extracted with 1000 μL of ethyl acetate, vortexed for 3 min to mix sufficiently and then centrifuged at 14
000 rpm for 10 min at 4 °C. The supernatants were transferred into another Eppendorf tube and dried out under a nitrogen stream. The residue was redissolved in 100 μL of methanol, oscillated ultrasonically at a working frequency of 40 kHz, and then centrifuged again at 14
000 rpm for 10 min at 4 °C. Subsequently, 5 μL aliquots of the supernatants were transferred into vials for injection.
Quality control (QC) samples at a low, medium and high concentration (2, 100 and 1000 ng mL−1) were prepared by spiking appropriate standard solutions of columbianetin and columbianetin acetate into blank plasma to the required plasma concentrations following the same preparation and operation method described above.
2.7. Method validation
2.7.1. Specificity and sensitivity. The chromatographs of six different batches of blank rat plasma samples and the corresponding spiked plasma were compared in order to test the specificity. For determination of columbianetin and columbianetin acetate, the lower limit of quantification (LLOQ) was defined as the lowest concentration in the calibration curve. The signal to noise ratio (S/N) at this concentration was larger than 5 while accuracy ranged from 80 to 120% and the relative standard deviation (RSD) (n = 6) was within 20% (guidance for industry: bioanalytical method validation, U.S. Department of Health and Human Services, 2001).24
2.7.2. Linearity. Calibration curve samples were prepared by spiking blank rat plasma with working stock solutions prepared as described above. Using a linear regression analysis, calibration curves were plotted by the peak-area ratios (y) of analyte against internal standard versus the nominal concentration (x).
2.7.3. Precision and accuracy. The validation of the intra-assay precision and accuracy of the method were evaluated by determination of the LLOQ samples and QC samples (n = 6) at three concentrations (1, 2, 100, 1000 ng mL−1). For the validation of inter-assay precision and accuracy, LLOQ, low, medium and high QC samples from three assays were evaluated. Precision was expressed by the relative standard deviation (RSD) and accuracy was calculated as a ratio of mean measured concentration to nominal concentration.
2.7.4. Recovery and matrix effect. The recoveries of two analytes in rat plasma were determined in six replicates of three concentrations of the QC samples (2, 100, 1000 ng mL−1). They were calculated as the percentage of the analyte peak area from the extracted QC plasma of the mean peak area from the extracted blank plasma spiked with the neat solutions. The matrix effects for the two analytes were determined using extracted blank rat plasma samples spiked with two analytes at three QC concentrations (2, 100, 1000 ng mL−1). The matrix effect was determined as the percentages of the analyte peak areas from the extracted blank plasma spiked with the two analyte solutions of the mean peak area of the analyte solutions at the same concentration prepared in the HPLC eluent.
2.7.5. Stability experiments. Stability experiments were conducted to confirm stability under the conditions that samples might be exposed to during storage and handling. The stability of the analytes after freeze/thaw cycles, after long-term storage and in an autosampler for 24 h was investigated at three concentrations (low, medium, high) of the QC samples using six replicates. For the freeze/thaw cycle test, QC samples were frozen at −20 °C and thawed at room temperature for a period of 12 h, three times. For the long-term stability test, QC samples were frozen at −20 °C for two weeks and were thawed at room temperature before injection. The stability of processed QC samples kept in an autosampler for 24 h was also investigated.Experiments were also carried out to evaluate the stability of columbianetin acetate and columbianetin alone in rat plasma, respectively. The interconversion between these two compounds, which may exist during the process of analysis, was also tested. Working stock solutions and QC samples for columbianetin and columbianetin acetate alone were prepared as described above.
2.8. Excretion of columbianetin acetate and columbianetin as its metabolite in rat urine and feces
Ten rats were housed in stainless steel metabolic cages provided with urine–feces separators. The rats were fasted overnight but had free access to water. The rats were orally administrated with columbianetin acetate which had been dissolved in 0.5% CMC-Na at a dosage of 5 mg kg−1 and then placed in metabolic cages that allowed for the separate collection of urine and feces. Urine and feces samples for each rat were collected at pre-dose (0 h) and at different time intervals (0–4, 4–8, 8–12, 12–24, 24–36, 36–48, 48–60, and 60–72 h). After the volumes of urine obtained were measured, the urine samples collected were centrifuged at 14
000 rpm for 10 min at 4 °C, and the supernatants of the urine samples and feces samples were stored at −20 °C until analysis. The preparation of the urine samples was the same as the preparation of the plasma samples as described above and the feces samples were dried out in a drying oven at 40 °C. After the weights of the feces samples obtained were measured, they were crushed and homogenized by a mortar. The preparation method of the feces samples was similar to that of the herbal sample preparation according to our previous study.25 The procedure was briefly as follows. 10 mg of feces powder and 1 mL of 70% methanol were added to 1.5 mL polythene tubes, mixed sufficiently for 3 min by vortexing and ultrasonically extracted for 30 min. Then the feces samples were centrifuged at 14
000 rpm for 10 min at 4 °C. 90 μL of the supernatant and 10 μL of IS were mixed together and transferred into vials for analysis by LC-MS/MS.
2.9. Pharmacokinetic study in rats
Male Sprague-Dawley rats (230 ± 20 g weight) were maintained in a rodent feeding room under a light/dark cycle of 12/12 h for 7 days before use. Food and water were given ad libitum except for 12 h before the experiment. Animal welfare and experimental procedures were conducted under the guidelines for the care and use of laboratory animals of the Ethics Review Committee of Tianjin University of Traditional Chinese Medicine. Columbianetin acetate for the intravenous administration study was ground with gum arabic (1
:
15, w/w) and dissolved in normal saline to a concentration of 5 mg mL−1. For the oral administration study, columbianetin acetate and the APR extract were dissolved in 0.5% carboxymethyl cellulose sodium solution (CMC-Na). The rats were randomly divided into five groups. The first group was given columbianetin acetate intravenously at a dose of 5 mg kg−1 (i.v. 5 mg group). Two of the groups were administered columbianetin acetate orally at a dose of 5 mg kg−1 (i.g. 5 mg kg−1 columbianetin acetate) and the APR extract at a corresponding dose (i.g. 5 mg kg−1 columbianetin acetate in extract), respectively. Another two groups were administered columbianetin acetate orally at a dose of 10 mg kg−1 (i.g. 10 mg kg−1 columbianetin acetate) and the APR extract spiked with columbianetin acetate at a corresponding dose of 10 mg kg−1 (i.g. 10 mg kg−1 APR adding columbianetin acetate), respectively. Blood samples (250 μL) were collected from the sub-orbital vein into 1.5 mL polythene tubes, which had been heparinized to prevent blood coagulation, at 0.033, 0.083, 0.167, 0.33, 0.5, 0.75, 1, 2, 3, 4, 5, 6, and 8 h after intravenous administration, and at 0.033, 0.083, 0.167, 0.33, 0.5, 0.75, 1, 2, 4, 5, 6, 8, 12, and 24 h after oral administration, respectively. Blood samples were immediately centrifuged after collection at 4000 rpm for 10 min at 4 °C to obtain plasma. Plasma was transferred into non-heparinized tubes and stored at −20 °C until analysis.
2.10. Chemical hydrolysis analysis
The samples of the APR extract, columbianetin acetate and pure columbianetin were dissolved in 3 mL of methanol. After being completely dissolved by ultrasonication, 2 mL of diluted hydrochloric acid was added into the reaction system, adequately mixed by vortexing and then placed in a water bath at 37 °C, and sufficiently reacted for 2 h. After reaction, the solutions were centrifuged at 14
000 rpm for 10 min at 4 °C, the supernatants were transferred into vials for analysis by an ultra-high performance liquid chromatography system equipped with a PDA detector (UHPLC-PDA). In this study, standard reaction solutions (1×) were prepared by diluting 234 mL of concentrated hydrochloric acid (12 mol L−1) in 1000 mL of distilled water. To investigate chemical hydrolyzation in different acidic conditions, eight different concentrations of hydrolysis solutions (standard reaction solutions (1×) and 2 fold (2×), 4 fold (4×), 6 fold (6×), 8 fold (8×), 12 fold (12×), 16 fold (16×) and 20 fold (20×) of the standard reaction solutions) were selected. The method of quantitative analysis by UHPLC-PDA was the same as in our previous study.26 All the chemical hydrolyzation experiments were carried out in triplicate; results were expressed as means ± standard deviation.
2.11. Data analysis
The “Drug and Statistics 1.0” program (DAS 1.0) (Medical College of Wannan, China) was employed for the purpose of analyzing the pharmacokinetic parameters after intravenous administration of columbianetin acetate and oral administration of columbianetin acetate and APR extract, respectively. AIC (Akaike information criterion) was used to judge the compartment model of the columbianetin acetate.
3. Results and discussion
3.1. Determination of the concentration of columbianetin acetate in the APR extract
Standards of the columbianetin acetate at 5, 10, 25, 50, 125, and 150 μg mL−1 concentrations were detected by the HPLC-UV-detector and a standard curve was drawn. The APR extract was dissolved in methanol, and then detected by HPLC. The standard curve equation was y = 0.0672x − 0.291 (y: peak-area ratios, x: concentration). The results indicate that in order to give the same dose of 5 mg kg−1 columbianetin acetate, the corresponding dose of the APR extract should be 0.72 g kg−1.
3.2 Optimization of the analytical method
It has already been confirmed in our previous studies that liquid–liquid extraction with ethyl acetate is suited to extract coumarins such as columbianetin acetate and columbianetin with excellent specificity and reproducibility.22 Warfarin (structure shown in Fig. 1) was selected as an internal standard (IS) in this study. Belonging to the coumarin class, warfarin, columbianetin acetate and columbianetin have similar chemical structural formulas. Until now, research has shown that there is no warfarin in APR.22 Thus, taking warfarin as an IS will not affect the results especially when the APR extract is administered orally. Furthermore, method validation has proven that warfarin has no interference from the endogenous matrix, and these three coumarins were easily quantified by HPLC-MS/MS without cross interference. Thus, warfarin was an excellent IS for quantifying the concentration of columbianetin acetate and columbianetin in rat plasma. Liquid–liquid extraction with ethyl acetate was selected to extract columbianetin acetate and columbianetin from rat plasma samples.
3.3. Method validation
3.3.1. Specificity and sensitivity. The representative chromatograms of the blank plasma and blank plasma spiked with columbianetin, columbianetin acetate and warfarin (IS), respectively, are shown in Fig. 2. The retention time of the columbianetin was about 8.06 min, the columbianetin acetate was 16.28 min and the IS was 15.43 min. This suggests that no interference from endogenous plasma substances or metabolites was observed and good separation of the analytes was achieved.
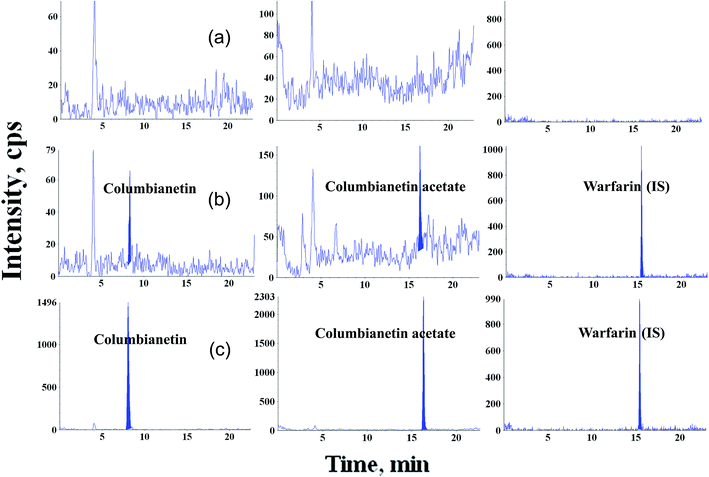 |
| Fig. 2 Representative chromatograms of (A) blank rat plasma, (B) blank rat plasma spiked with the standard compounds at LLOQ and (C) real samples after administration of columbianetin acetate. | |
3.3.2. Calibration curves and lower limits of quantification. The plasma calibration curves for both columbianetin acetate and columbianetin demonstrated a reliable reproducibility over the range of 1–5000 ng mL−1. The regression equations obtained for columbianetin acetate and columbianetin were y = 0.0173x + 0.0523 and y = 0.104x + 0.186, using a weighting factor (1/x2), respectively. The lower limits of quantification (LLOQ) for determination of columbianetin and columbianetin acetate in plasma were both 1 ng mL−1. The calibration curves from the stability experiments of columbianetin acetate and columbianetin alone in plasma were y = 0.00563x + 0.00778 (r = 0.9978) and y = 0.00327x + 0.00286 (r = 0.9988), using a weighting factor (1/x2), respectively. For the quantitative analysis by UHPLC, the calibration curves of columbianetin acetate and columbianetin were y = 15
792.776 − 440.9404 (r = 0.9996) and y = 17
162.017 − 393.88545 (r = 0.9999), using a weighting factor (1/x2), respectively.
3.3.3. Precision and accuracy. The intra-assay and inter-assay precision and accuracy of the method are summarized in Table 1 by analyzing the LLOQ samples and QC samples at three concentrations in six replicates. For the results of the QC samples, the intra-assay precision was less than 11.6% for columbianetin, 10.9% for columbianetin acetate while the inter-assay precision was within 13.2% for columbianetin and within 11.9% for columbianetin acetate. For the results of the LLOQ samples, the intra-assay precision was 8.14% and 19.0% for columbianetin and columbianetin acetate, respectively. The inter-assay precision was 6.25% for columbianetin and 16.7% for columbianetin acetate. The intra-assay accuracy ranged from 90.2% to 99.0% and the inter-assay accuracy ranged from 89.6% to 100% for columbianetin and columbianetin acetate. Following the USFDA guidelines, all the intra- and inter-assay values for both columbianetin and columbianetin acetate were found to be within the acceptable variable limits. According to these results, the method was confirmed to be precise and accurate for determination of columbianetin and columbianetin acetate in rat plasma.
Table 1 Intra-assay, inter-assay accuracy and precision of the columbianetin acetate and columbianetin detection assays (n = 6)
|
Concentration (ng mL−1) |
Intra-assay |
Inter-assay |
Accuracy (%) |
RSD (%) |
Accuracy (%) |
RSD (%) |
Columbianetin |
1 |
106 |
8.14 |
107 |
6.25 |
2 |
99.0 |
11.6 |
92.5 |
13.2 |
100 |
92.1 |
6.13 |
93.4 |
5.79 |
1000 |
97.4 |
8.13 |
100 |
7.71 |
Columbianetin acetate |
1 |
101 |
19.0 |
99.2 |
16.7 |
2 |
90.0 |
8.32 |
90.3 |
11.9 |
100 |
90.2 |
10.9 |
89.6 |
7.43 |
1000 |
93.5 |
5.31 |
93.0 |
6.10 |
3.3.4. Recovery and matrix effects. The extraction recoveries were 114%, 101% and 110% for columbianetin and 103%, 98.5%, and 93.4% for columbianetin acetate QC samples at the concentrations of 2, 100 and 1000 ng mL−1, respectively. The corresponding RSDs were 12.8%, 9.98% and 4.97% for columbianetin and 8.61%, 2.96% and 3.84% for columbianetin acetate, respectively (Table 2). As shown in Table 2, the matrix effects of columbianetin and columbianetin acetate ranged from 101% to 119%, and 104% to 112% for all levels of the QC samples, respectively. Based on these results, the ethyl acetate method of extraction from plasma samples could be proved to be precise and feasible for columbianetin and columbianetin acetate and the conclusion could be drawn that endogenous substances have little influence on the determination of columbianetin and columbianetin acetate in rat plasma. These results were consistent with our previous study.
Table 2 Recovery and matrix effects of the columbianetin acetate and columbianetin extraction methods (n = 6)
|
Concentration (ng mL−1) |
Recovery |
Matrix effects |
Mean (%) |
RSD (%) |
Mean (%) |
RSD (%) |
Columbianetin |
2 |
114 |
12.8 |
119 |
9.94 |
100 |
101 |
9.98 |
101 |
8.41 |
1000 |
110 |
4.97 |
103 |
2.65 |
Columbianetin acetate |
2 |
103 |
8.61 |
112 |
14.5 |
100 |
98.5 |
2.96 |
113 |
10.9 |
1000 |
93.4 |
3.84 |
104 |
3.26 |
3.3.5. Stability. The results of the stability experiments consisting of stability after freeze/thaw cycles, stability after a two week duration and stability in an autosampler for 24 h, for columbianetin and columbianetin acetate, both together and alone in plasma, are shown in Tables 3 and 4. Even though it had been found that (shown in Table 5) 0.49 ± 0.07% at the high concentration (1000 ng mL−1) and 0.05 ± 0.01% at the medium concentration (100 ng mL−1) of the columbianetin acetate were converted into columbianetin in the stability experiment consisting of a measurement of stability after two weeks of columbianetin acetate alone in plasma. This phenomenon of conversion also occurred in the freeze/thaw cycle stability experiments at the high concentration (1000 ng mL−1) where 2.07 ± 0.10% of the columbianetin acetate changed, the results were all within the acceptable variable limits, indicating that columbianetin and columbianetin acetate in rat plasma were stable after three freeze/thaw cycles, after being frozen for two weeks and after being kept in an autosampler for 24 h. The results suggested that samples should be detected after two weeks and the storage and handling of samples have no significant effect on the results of the analysis during the experimental process.
Table 3 Stability of columbianetin acetate and columbianetin together in the plasma (n = 6)
|
Concentration (ng mL−1) |
Freeze–thaw cycles |
Stability for two weeks |
Autosampler for 24 h |
Accuracy (%) |
RSD (%) |
Accuracy (%) |
RSD (%) |
Accuracy (%) |
RSD (%) |
Columbianetin |
2 |
84.8 |
15.0 |
113 |
12.8 |
94.5 |
11.8 |
100 |
92.8 |
9.54 |
97.5 |
9.11 |
96.7 |
7.14 |
1000 |
114 |
6.95 |
113 |
7.37 |
104 |
8.60 |
Columbianetin acetate |
2 |
83.8 |
8.38 |
83.7 |
12.3 |
90.0 |
8.32 |
100 |
106 |
12.8 |
102 |
6.42 |
87.1 |
4.75 |
1000 |
100 |
6.36 |
99.8 |
8.90 |
86.2 |
10.1 |
Table 4 Stability of columbianetin acetate and columbianetin alone in the plasma (n = 6)
|
Concentration (ng mL−1) |
Freeze–thaw cycles |
Stability for two weeks |
Autosampler for 24 h |
Accuracy (%) |
RSD (%) |
Accuracy (%) |
RSD (%) |
Accuracy (%) |
RSD (%) |
Columbianetin |
2 |
115 |
12.6 |
106 |
8.83 |
110 |
9.16 |
100 |
103 |
4.72 |
103 |
3.55 |
110 |
3.96 |
1000 |
104 |
4.29 |
104 |
4.42 |
106 |
2.68 |
Columbianetin acetate |
2 |
111 |
10.2 |
115 |
9.34 |
109 |
13.2 |
100 |
107 |
2.92 |
103 |
6.01 |
105 |
4.41 |
1000 |
109 |
2.11 |
95.5 |
6.08 |
109 |
2.99 |
Table 5 Columbianetin acetate which has been converted to columbianetin during the stability experiments
|
Concentration (ng mL−1) |
Freeze–thaw cycles |
Stability for two weeks |
Columbianetin acetate |
2 |
— |
— |
100 |
— |
0.05 ± 0.01% |
1000 |
2.07 ± 0.10% |
0.49 ± 0.07% |
3.4. Pharmacokinetic studies
The developed LC-MS/MS method was successfully applied to the pharmacokinetic study of columbianetin acetate and columbianetin in rat plasma following intravenous and oral administration of pure columbianetin acetate and oral administration of the APR extract.
3.4.1. Pharmacokinetics of columbianetin and columbianetin acetate in rats after intravenous administration of pure columbianetin acetate. The mean plasma concentration–time profiles of columbianetin acetate after intravenous administration of columbianetin acetate at a dose of 5 mg kg−1 to rats are shown in Fig. 3. According to AIC comparison and model diagnostics, the model with a lower AIC value should be a priority. The two-compartment model (AIC value = 103.2) should obviously be preferred over the one-compartment model (AIC value = 122.3). The relevant pharmacokinetic parameters are listed in Table 6. Columbianetin acetate was distributed to tissues rapidly based on the distribution half-life (T1/2α) value of 3 min. Taking the average steady-state volume of distribution (V) compared to the value of V1, the former (0.0076 ± 0.0062 L) was 5.42 fold the latter (0.0014 ± 0.0005 L). These values suggested that columbianetin acetate was distributed rapidly and widely in rats. The elimination half-life (T1/2β) was valued at 0.8302 ± 0.7805 h, indicating a rapid elimination for columbianetin acetate from plasma in rats. The total clearance (CL) of columbianetin acetate was assessed to be 0.0069 ± 0.0014 L h−1.
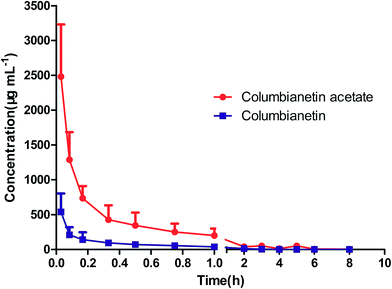 |
| Fig. 3 The mean plasma concentration–time profiles of columbianetin acetate and columbianetin after intravenous administration of pure columbianetin acetate. | |
Table 6 Pharmacokinetics of columbianetin and columbianetin acetate in rats after intravenous administration of columbianetin acetate
Parameters |
Columbianetin acetate |
Columbianetin as the metabolite of columbianetin acetate |
Tmax (h) |
0.03 ± 0.00 |
0.03 ± 0.00 |
Cmax (μg L−1) |
2283 ± 826 |
539 ± 263 |
k10 (h−1) |
5.53 ± 3.38 |
10.90 ± 6.36 |
k12 (h−1) |
10.71 ± 4.60 |
26.77 ± 11.79 |
k21 (h−1) |
4.84 ± 2.94 |
6.14 ± 2.99 |
V1 (L) |
0.0014 ± 0.0005 |
0.0047 ± 0.0034 |
V (L) |
0.0076 ± 0.0062 |
0.0312 ± 0.0184 |
T1/2α (h) |
0.0407 ± 0.0151 |
0.0195 ± 0.0093 |
T1/2β (h) |
0.8302 ± 0.7805 |
0.5824 ± 0.3370 |
CL (L h−1) |
0.0069 ± 0.0014 |
0.0359 ± 0.0095 |
AUC(0–tn) (μg L−1 h−1) |
710 ± 149 |
145 ± 48 |
AUC(0–∞) (μg L−1 h−1) |
757 ± 169 |
148 ± 47 |
When detecting columbianetin acetate, data regarding the detection of columbianetin was also collected by LC-MS after intravenous administration of columbianetin acetate. The mean plasma concentration–time profiles of columbianetin are shown in Fig. 3. It could be concluded that columbianetin acetate was metabolized to columbianetin in vivo. For columbianetin, the two-compartment model AIC value (74.38) was lower than the one-compartment model AIC value (97.06). Thus, the two-compartment model was selected for columbianetin. The pharmacokinetic parameters are also listed in Table 6. After intravenous administration of pure columbianetin acetate, the AUC(0–tn) values of columbianetin acetate and columbianetin were 710 ± 149 μg L−1 and 145 ± 48 μg L−1, respectively. According to the chemical structures of columbianetin acetate and columbianetin shown in Fig. 1, one molecule of the columbianetin metabolite comes from one molecule of columbianetin acetate. Thus, the amount of columbianetin acetate that was metabolized into columbianetin could be calculated. It was found that approximately 23.91% of the columbianetin acetate is metabolized into columbianetin by the liver after intravenous administration of columbianetin acetate. Combined with the preliminary pharmacological experiments, this suggests that columbianetin is one of the main metabolites of columbianetin acetate which plays a role in its efficacy.
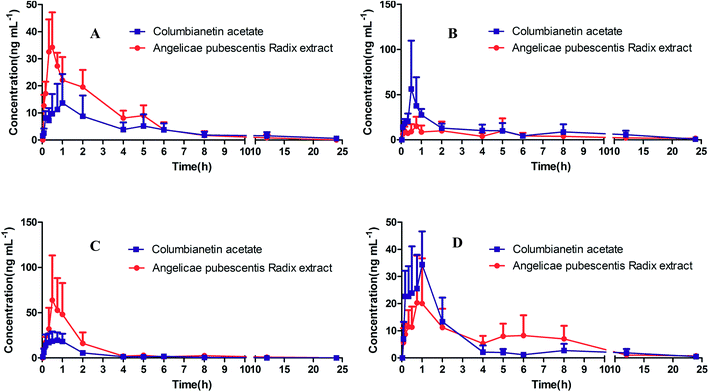 |
| Fig. 4 The mean plasma concentration–time profiles of (A) columbianetin acetate following i.g. administration of 5 mg kg−1 columbianetin acetate and 5 mg kg−1 columbianetin acetate in APR extract; (B) columbianetin acetate following i.g. administration of 10 mg kg−1 columbianetin acetate and 10 mg kg−1 columbianetin acetate in APR extract; (C) columbianetin following i.g. administration of 5 mg kg−1 columbianetin acetate and 5 mg kg−1 columbianetin acetate in APR extract; (D) columbianetin following i.g. administration of 10 mg kg−1 columbianetin acetate and 10 mg kg−1 columbianetin acetate in APR extract. | |
3.4.2. Pharmacokinetics of columbianetin and columbianetin acetate in rats after oral administration of pure columbianetin acetate. In accordance with AIC comparison and model diagnostics, the one-compartment model (AIC value = 57.85) was more suitable than the two-compartment model (AIC value = 58.85). Thus, the one compartment model was selected to describe the pharmacokinetic profile of columbianetin acetate after oral administration of columbianetin acetate. The mean plasma concentration–time profiles of columbianetin acetate and columbianetin are shown in Fig. 4 after oral administration of columbianetin acetate at a dose of 5 mg kg−1 and 10 mg kg−1 to rats. Some relevant pharmacokinetic parameters are listed in Table 7. After oral administration of columbianetin acetate, columbianetin acetate was rapidly absorbed into the blood from the rat gastrointestinal tract. Columbianetin acetate was detected in the plasma from the first blood sampling time (2 min) and rapidly reached Tmax (0.53–0.56 h) for the two oral doses studied. The elimination time of columbianetin acetate is short because the T1/2α value was 3.44 ± 0.71 h. The AUC(0–tn) of columbianetin acetate at doses of 5 and 10 mg kg−1 was 49.89 ± 31.21 and 122.2 ± 34.1 μg L−1 h−1. It was noted that the dose-normalized AUC values of columbianetin acetate (based on 5 mg kg−1) were proportional to the doses studied.
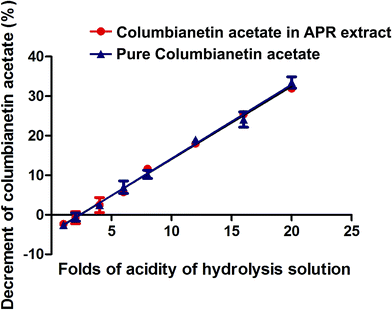 |
| Fig. 5 The relationship between the percentage decrement of columbianetin acetate and different concentrations of hydrochloric acid hydrolysis solution. | |
Table 7 Pharmacokinetics of columbianetin and columbianetin acetate in rats after oral administration of columbianetin acetate and APR extract
Parameters |
Oral administration of columbianetin acetate (5 mg kg−1) |
Oral administration of APR extract (5 mg kg−1) |
Oral administration of columbianetin acetate (10 mg kg−1) |
Oral administration of APR extract and columbianetin acetate (10 mg kg−1) |
Columbianetin acetate |
Columbianetin as metabolite of columbianetin acetate |
Columbianetin acetate |
Columbianetin |
Columbianetin acetate |
Columbianetin as metabolite of columbianetin acetate |
Columbianetin acetate |
Columbianetin |
Significant difference (P < 0.05) from APR extract. Significant difference (P < 0.05) from APR extract and columbianetin acetate (APR + 5 mg). Extremely significant difference (P < 0.01) from APR extract and columbianetin acetate (APR + 5 mg). |
Tmax (h) |
0.56 ± 0.39 |
0.52 ± 0.35 |
0.49 ± 0.15 |
0.66 ± 0.23 |
0.53 ± 0.39 |
0.52 ± 0.31 |
0.38 ± 0.26 |
0.59 ± 0.38 |
Cmax (μg L−1) |
25.34 ± 16.96 |
25.65 ± 9.17 |
34.15 ± 7.99 |
73.35 ± 48.54 |
33.28 ± 4.35 |
31.86 ± 11.93b |
22.68 ± 12.57 |
19.05 ± 14.68 |
ka (h−1) |
23.49 ± 28.62 |
12.05 ± 17.59 |
6.27 ± 2.14 |
14.49 ± 8.85 |
41.31 ± 36.81 |
12.39 ± 3.77c |
32.91 ± 32.11 |
35.92 ± 36.70 |
ke (h−1) |
0.19 ± 0.06a |
0.78 ± 0.58 |
0.39 ± 0.17 |
0.71 ± 0.45 |
0.30 ± 0.40 |
0.46 ± 0.25c |
0.19 ± 0.11 |
0.15 ± 0.08 |
V/F (L) |
0.26 ± 0.14a |
0.25 ± 0.25 |
0.13 ± 0.04 |
0.11 ± 0.15 |
0.39 ± 0.14c |
0.47 ± 0.16 |
1.17 ± 0.55 |
0.79 ± 0.45 |
T1/2ka (h) |
0.038 ± 0.052a |
0.15 ± 0.18 |
0.13 ± 0.06 |
0.10 ± 0.12 |
0.031 ± 0.045 |
0.044 ± 0.042 |
0.033 ± 0.047 |
0.030 ± 0.039 |
T1/2α (h) |
3.44 ± 0.71a |
1.18 ± 0.67 |
2.99 ± 0.78 |
1.30 ± 0.63 |
2.43 ± 1.08 |
1.90 ± 0.88b |
3.63 ± 1.53 |
3.83 ± 0.88 |
Lag time (h) |
0.030 ± 0.039 |
0.036 ± 0.045 |
0.044 ± 0.035 |
0.071 ± 0.031 |
0.046 ± 0.038 |
0.033 ± 0.036 |
0.020 ± 0.030 |
0.067 ± 0.059 |
AUC(0–tn) (μg L−1 h−1) |
49.89 ± 31.21 |
51.58 ± 20.08 |
78.06 ± 15.75 |
98.08 ± 56.26 |
122.2 ± 34.1 |
82.57 ± 24.99 |
89.51 ± 57.89 |
102.4 ± 52.2 |
AUC(0–∞) (μg L−1 h−1) |
56.73 ± 37.35 |
54.29 ± 24.18 |
96.30 ± 33.98 |
102.4 ± 55.1 |
125.1 ± 34.6 |
86.00 ± 28.16 |
95.70 ± 70.17 |
114.3 ± 57.3 |
After oral administration of columbianetin acetate to rats, columbianetin had a high plasma concentration, its Tmax was 0.52 ± 0.35 h, Cmax was 25.65 ± 9.17 μg L−1 and T1/2α was 1.18 ± 0.67 h. Based on these results, it can be seen that a large amount of columbianetin acetate was transformed into columbianetin and this metabolite can be eliminated rapidly from the body. Comparing the columbianetin acetate AUC(0–tn) values after oral administration (49.89 ± 31.21) to the AUC(0–tn) values after intravenous administration (710 ± 149) and comparing the oral and intravenous AUC(0–tn) values of columbianetin (51.58 ± 20.08/145 ± 48) at the same dose of 5 mg kg−1 (Tables 6 and 7), it was worth noting that the oral values are lower than the intravenous values. The mean Tmax for columbianetin acetate and columbianetin was 0.56 h and 0.52 h, respectively. That is to say, the maximum concentration of the metabolite was observed before that of the parent. This was in accord with the phenomenon that columbianetin acetate could be converted into columbianetin in acidic solution. Columbianetin had a high bioavailability in our previous studies and rapidly reached Tmax (11–43 min) for all three oral doses studied.27 It was demonstrated that an amount of columbianetin acetate was hydrolyzed into columbianetin in the stomach under the acidic conditions so that the maximum concentration of the metabolite with a high plasma concentration was observed before that of the parent. The reasons for this result still need further study.
3.4.3. Pharmacokinetics of columbianetin and columbianetin acetate in rats after oral administration of APR extract. As one of the main components in APR, columbianetin acetate has a high concentration in APR extract. In order to investigate the difference between oral administration of columbianetin acetate and APR extract, APR extract containing corresponding doses of columbianetin acetate (5 mg kg−1) was given to rats orally. Columbianetin acetate and columbianetin plasma samples were collected at the same time, and the mean plasma concentration–time profiles of columbianetin acetate and columbianetin were plotted in Fig. 4. The main pharmacokinetic parameters are exhibited in Table 7. After oral administration of APR extract, the Tmax, Cmax and AUC(0–tn) values for columbianetin acetate were 0.49 ± 0.15 h, 34.15 ± 7.99 μg L−1 and 78.06 ± 15.75 μg L−1 h−1, respectively. There were no significant differences between the plasma concentrations of columbianetin acetate after oral administration of pure columbianetin acetate and that of APR extract. The Cmax and AUC(0–tn) values for columbianetin were 73.35 ± 48.54 μg L−1 and 98.08 ± 56.26 μg L−1 h−1, respectively. Compared with oral administration of columbianetin acetate at the corresponding dose, the Cmax and AUC(0–tn) values for columbianetin after oral administration of APR extract were increased. This phenomenon indicated that other co-existing ingredients in APR extract could increase the concentration of columbianetin in the plasma. There are two possibilities to explain these results. Firstly, it is possible that other derivatives of columbianetin, in particular glycosides, are present in the extract and they are transformed to the molecules of interest in the organism. In this study, a hydrolysis analysis of the extract and pure components was performed to evaluate this problem. In order to make other derivatives of columbianetin fully hydrolyze into columbianetin, different concentrations of hydrochloric acid were used to hydrolyze the APR extract and the corresponding concentrations of pure columbianetin acetate and columbianetin. It was found that columbianetin is stable in the different concentrations of hydrochloric acid. Columbianetin acetate could be hydrolyzed into columbianetin. The APR extract could also be hydrolyzed into columbianetin. As shown in Fig. 5, the hydrolysis percentages of columbianetin acetate and the APR extract were closely coincident. It was summarized that there are no influences of other co-existing ingredients in the APR extract on the transformation of columbianetin acetate into columbianetin. From Fig. 6, the concentrations of columbianetin generated by the hydrolysis of APR extract were higher than those from the hydrolysis of pure columbianetin acetate at all different concentrations of hydrochloric acid. It could be concluded that other derivatives of columbianetin could be hydrolyzed into columbianetin. According to our previous results,26 other derivatives of columbianetin comprised columbianadin and columbianetin-β-D-glucopyranoside. It was also found that the concentration of columbianadin in the APR extract was increased when the concentration of the hydrochloric acid was increased. Another possibility is that co-existing ingredients in the APR extract could change the pharmacokinetic behaviour of columbianetin acetate. In order to confirm an enhancement of absorption or influence on metabolism of an alteration of the pharmacokinetics in the APR extract, additional pharmacokinetic studies were performed adding a known amount of purified columbianetin acetate to the extract (corresponding dose of columbianetin acetate (10 mg kg−1)). It was found that there were no significant differences between plasma concentrations of columbianetin acetate and its metabolite columbianetin between oral administration of pure columbianetin acetate at a dose of 10 mg kg−1 and that of the APR extract with a known amount of purified columbianetin acetate (10 mg kg−1). Based on these results, the pharmacokinetic behaviour of the columbianetin acetate which was added to the extract was enhanced proportionally like that of columbianetin acetate which was added to the purified samples. Therefore, other components in the APR extract did not change the pharmacokinetic behaviour of the columbianetin acetate, which is not the main reason why other co-existing ingredients in the APR extract could increase the concentration of columbianetin in the plasma. Based on the above results, columbianetin-β-D-glucopyranoside, one of the derivatives of columbianetin in the APR extract might be transformed to columbianetin so that administration of the APR extract could increase the concentration of columbianetin in plasma.
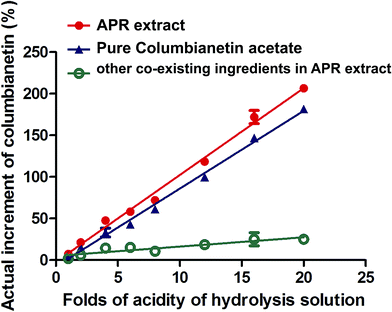 |
| Fig. 6 The relationship between the percentage increment of columbianetin and different concentrations of hydrochloric acid hydrolysis solution. | |
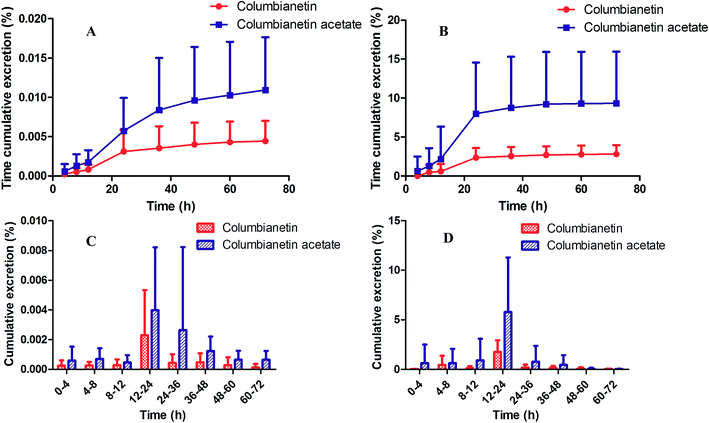 |
| Fig. 7 Time cumulative excretion percentage of columbianetin acetate and its metabolite columbianetin (A) in urine and (B) in feces; cumulative excretion percentage at different time intervals of columbianetin acetate and its metabolite columbianetin (C) in urine and (D) in feces. | |
3.5 Oral bioavailability of columbianetin acetate in rats after administration
As shown in Table 7, the oral bioavailability of pure columbianetin acetate and columbianetin acetate in the APR extract were calculated. In comparison with the AUC(0–tn) values of pure columbianetin acetate delivered orally and by intravenous administration, the absolute bioavailability of columbianetin acetate was 7.0 ± 4.3% and 11 ± 2% in pure form and APR extract, respectively.
3.6. Excretion study of columbianetin acetate and its metabolite columbianetin in rat urine and feces
The characteristics of the cumulative urinary excretion of columbianetin acetate were determined. After oral administration of 5 mg kg−1 columbianetin acetate to rats, both columbianetin acetate and columbianetin were found in the urine and feces. It was demonstrated that columbianetin acetate was metabolized to columbianetin in vivo. Columbianetin acetate and columbianetin could be detected in rat urine until 72 h after dosing. From 12 to 36 h, columbianetin acetate and columbianetin levels in rat urine increased quickly. As shown in Fig. 7, cumulative excretion of columbianetin acetate in urine at 72 h after administration accounted for 0.0109 ± 0.0067% of the total dosage of columbianetin acetate in its original form. Columbianetin as one of metabolites of columbianetin acetate was excreted in the urine and accounted for 0.0044 ± 0.0026% of the total dosage of columbianetin acetate. The above results indicate that the majority of the dosed columbianetin acetate might have been metabolized in the rats. Columbianetin acetate and columbianetin were also determined in feces samples after dosing. The cumulative excretion of columbianetin acetate showed a continuous increase until 36 h. The maximum excretion of columbianetin acetate and columbianetin into feces was observed from 12 to 24 h. The cumulative amounts of columbianetin acetate and columbianetin present 9.32 ± 6.63% and 2.81 ± 1.15% of the total dose, which indicates that the columbianetin acetate was mainly excreted in the feces. In the present study, only columbianetin acetate and its metabolite columbianetin were determined in rat urine and feces. The other metabolites of columbianetin acetate need further study.
4. Conclusion
A sensitive, accurate and precise method has been established for simultaneous determination of the concentration of columbianetin acetate and columbianetin in rat plasma by LC-MS/MS. The pharmacokinetic profiles of columbianetin acetate and columbianetin after intravenous and oral administration of pure columbianetin acetate, and oral administration of the APR extract to rats were obtained. The results showed that columbianetin acetate could be metabolized into columbianetin in vivo. The oral bioavailability of columbianetin acetate in rats after administration of pure columbianetin acetate was 7.0 ± 4.3%. The pharmacokinetic parameters of columbianetin after oral administration of pure columbianetin acetate or APR extract were compared, and the results suggest that other co-existing ingredients in the APR extract could promote the increased concentration of columbianetin in rat plasma. Cumulative excretion of columbianetin acetate in urine accounted for 0.0109 ± 0.0067% of the total dosage. The cumulative amount of columbianetin acetate in the feces presents 9.32 ± 6.63% of the total dose. Columbianetin acetate was mainly excreted in the feces. These results might be helpful for further in vivo study and clinical application of columbianetin acetate and APR extract.
Acknowledgements
This research was supported National Natural Science Foundation of China (81503213 and 81374050), National Science and Technology Support Program Projects (2014BA105B01), Program for Innovative Research Team in Universities of Tianjin (TD12-5033) and PCSIRT (IRT-14R41), Tianjin Science and Technology Plan Project (12ZCDZSY17000) and State the Science & Technology Commission of MOST of China (2014ZX09304307001).
References
- F. Ma, X. Gong, X. Zhou, Y. Zhao and M. Li, J. Ethnopharmacol., 2015, 162, 377–383 CrossRef CAS PubMed.
- J. Shi, Q. Fu, W. Chen, H. P. Yang, J. Liu, X. M. Wang and X. He, J. Ethnopharmacol., 2013, 145, 25–31 CrossRef CAS PubMed.
- T. Lu, J. Song, F. Huang, Y. Deng, L. Xie, G. Wang and X. Liu, J. Ethnopharmacol., 2007, 110, 412–418 CrossRef CAS PubMed.
- Y. W. Ya, X. J. Han, N. N. Shi, L. Y. Wang, X. Liao, L. D. Zhong, Z. X. Bian and A. P. Lu, European Journal of Integrative Medicine, 2014, 6, 176–185 CrossRef.
- C. Zhang, M. Jiang and A. P. Lu, European Journal of Integrative Medicine, 2011, 3, e219–e231 CrossRef.
- X. Li, J. Wang and L. Gao, Afr. J. Tradit., Complementary Altern. Med., 2013, 10 Search PubMed.
- D. C. Wang, T. D. Li and X. Y. Xu, Chin. J. Inf. Tradit. Chin. Med., 2007, 11, 031–032 Search PubMed.
- H. Zhou, L. T. Tao, H. C. Xu, Y. G. Jiang, Y. Q. Deng, Y. Luo and C. J. Lu, World J. Sci. Technol., 2011, 13, 777–782 CrossRef.
- K. G. Cao, L. H. Yu, Y. Gao, Y. P. Fan, J. J. Zhao, X. Z. Zhang, W. Xie, W. M. Yang, M. J. Dong, T. Li and X. Y. Qiao, European Journal of Integrative Medicine, 2014, 6, 259–267 CrossRef.
- Z. Gao, Y. Lu, U. Halmurat, J. Jing and D. Xu, Chin. J. Integr. Med., 2013, 19, 862–868 CrossRef PubMed.
- T. K. Huang, A Handbook of the Composition and Pharmacology of Common Chinese Drugs, China Pharmaceutical Science Press, Beijing, China, 1994, p. 1875 Search PubMed.
- J. X. Pan, Y. K. Lam, B. Arison, J. Smith and G. Q. Han, Acta Pharm. Sin., 1987, 22, 380 CAS.
- R. Z. Li, Y. Q. He, M. Chiao, Y. Xu, Q. B. Zhang, J. R. Meng, Y. Gu and L. P. Ge, Acta Pharm. Sin., 1988, 24, 546–551 Search PubMed.
- Y. X. Chang, Z. W. Zhu, J. Li, Q. H. Zhang and X. W. Qin, Journal of Inner Mongolia University, 2011, 42, 215–223 CAS.
- C. Ito, M. Itoigawa, S. Onoda, A. Hosokawa, N. Ruangrungsi, T. Okuda, H. Tokuda, H. Nishino and H. Furukawa, Phytochemistry, 2005, 66, 567–572 CrossRef CAS PubMed.
- Y.-F. Chen, H.-Y. Tsai and T.-S. Wu, Planta Med., 1995, 61, 2–8 CrossRef CAS PubMed.
- T. B. Ng, F. Liu and Z. T. Wang, Life Sci., 2000, 66, 709–723 CrossRef CAS PubMed.
- K. H. Kang, C. S. Kong, Y. Seo, M. M. Kim and S. K. Kim, Food Chem. Toxicol., 2009, 47, 2129–2134 CrossRef CAS PubMed.
- H. J. Jeong, H. J. Na, S. J. Kim, H. K. Rim, N. Y. Myung, P. D. Moon, N. R. Han, J. U. Seo, T. H. Kang and J. J. Kim, Biol. Pharm. Bull., 2009, 32, 1027–1031 CAS.
- U. Afek, S. Carmeli and N. Aharoni, Phytochemistry, 1995, 39, 1347–1350 CrossRef CAS.
- X. J. Wu, M. L. Zhang, X. Y. Cui, F. Gao, Q. He, X. J. Li, J. W. Zhang, J. P. Fawcett and J. K. Gu, J. Ethnopharmacol., 2012, 139, 201–206 CrossRef CAS PubMed.
- Y. X. Chang, Q. H. Zhang, J. Li, L. Zhang, X. R. Guo, J. He, P. Zhang, L. Ma, Y. R. Deng, B. L. Zhang and X. M. Gao, J. Pharm. Biomed. Anal., 2013, 77, 71–75 CrossRef CAS PubMed.
- Y. X. Chang, Z. W. Zhu, J. Li, Q. H. Zhang, Y. R. Deng, L. Y. Kang, B. L. Zhang and X. M. Gao, Chromatographia, 2011, 74, 639–643 CAS.
- Guidance for industry: bioanalytical method validation, U.S. Department of Health and Human Services, Food and Drug Administration Centre for Drug Evaluation and Research (CDER), Centre for Veterinary Medicine (CVM), 2001.
- J. Li, Q. H. Zhang, J. He, E. W. Liu, X. M. Gao and Y. X. Chang, Sci. World J., 2015, 2015, 10 Search PubMed.
- A. H. Ge, W. F. Ma, C. P. Wang, J. Li, J. He, E. W. Liu, T. A. Adelakum, B. L. Zhang, X. Gao and Y. X. Chang, J. Sep. Sci., 2014, 37, 2523–2534 CrossRef CAS PubMed.
- Q. Luo, C. P. Wang, J. Li, W. F. Ma, Y. Bai, L. Ma, X. M. Gao, B. L. Zhang and Y. X. Chang, J. Ethnopharmacol., 2013, 150, 175–180 CrossRef CAS PubMed.
Footnote |
† The author contributed equal to first author in this study. |
|
This journal is © The Royal Society of Chemistry 2015 |