DOI:
10.1039/C5RA06927K
(Communication)
RSC Adv., 2015,
5, 38906-38909
[n]Pseudorotaxanes constructed by a bis(p-phenylene)-34-crown-10-based cryptand: different binding behaviors induced by minor structural changes of guests†
Received
24th February 2015
, Accepted 21st April 2015
First published on 22nd April 2015
Abstract
Three pseudorotaxanes consisting of a bis(p-phenylene)-34-crown-10-based cryptand host and 1,2-bis(4-pyridinium)ethane/ethylene derivative guests were obtained. It was found that small structural changes on guests could have a big effect on the binding behaviors of host–guest complexes.
In host–guest chemistry, threaded structures1 have attracted a lot of attention not only because of their contributions in topology, but also due to their potential applications in artificial molecular machines, metal–organic frameworks, supramolecular polymers, nanotechnology, etc.2 As the fundamental building blocks for fabricating advanced mechanically interlocked species with intriguing properties, pseudorotaxanes,3 self-assembly complexes in which macrocyclic hosts encircle linear guests, play important roles in threaded structures and supramolecular chemistry. In general, minor structural changes on hosts or guests can cause large effects on binding behaviors and geometries of the resultant threaded structures, thereby influencing the formation of advanced supramolecular assemblies.4 In view of [n]pseudorotaxanes, the same phenomena happen sometimes in many aspects, such as the stoichiometry, association constants, and so on.5 Gibson et al. reported that association constants of pseudocryptand-type [2]pseudorotaxanes based on the self-assembly of bis(m-phenylene)-32-crown-10 pyridyl, quinolyl, and naphthyridyl derivatives with paraquat improve remarkably.6a Schalley and coworkers reported that the deslipping reaction of rotaxanes can be obviously caused by the simple exchange of a CH group by an isoelectronic N atom.6b Therefore, revealing the effect of structural changes on host–guest binding behaviors is very important and will promote the efficient construction of functional supramolecular materials.7
Vinylogous viologens, which were synthesized to modify and expand properties and functions of paraquats (due to their electrochemical and photochemical activities), were attractive building units for the preparation of pseudorotaxanes.8 1,2-Bis(4-pyridinium)ethane derivatives, as analogues of vinylogous viologens, have rarely been used to construct mechanically interlocked molecules, such as pseudorotaxanes and rotaxanes, possibly because of the lack of powerful hosts which can recognize them efficiently.9 Cryptands have been proved to be much more powerful hosts for organic salts than their crown ether precursors because of the introduction of additional binding sites and the preorganization of the host structures during the association process. Therefore, it is worthwhile to study the novel recognition motifs based on cryptands, vinylogous viologens, and their analogues. Herein, we reported three [n]pseudorotaxanes formed between bis(p-phenylene)-34-crown-10 (BPP34C10)-based cryptand 1 and 1,2-bis(4-pyridinium)ethane/ethylene derivatives (2, 3, and 4, Scheme 1). Though these three guests showed subtle differences in structures, the corresponding [n]pseudorotaxanes between cryptand 1 and them exhibited different host–guest binding behaviors and geometries in the solid state. By self-assembly of cryptand 1 and guest 2, a [2]pseudorotaxane driven by hydrogen bonding and π–π stacking interactions was formed in the solid state. Nevertheless, simply changing the benzyl groups in guest 2 to methyl groups afforded a [3]pseudorotaxane as shown by X-ray analysis. What is more, if the bridged ethyl chain in guest 3 was further replaced by an ethylene chain (guest 4), the association constant between 1 and 4 increased about 465 and 128 times in acetone than those of 1 ⊃ 2 and 1 ⊃ 3, respectively.
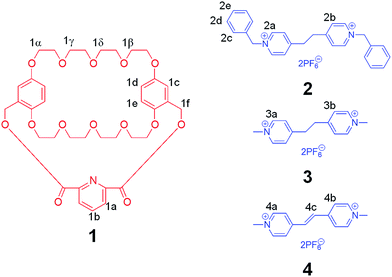 |
| Scheme 1 Chemical structures of BPP34C10-based cryptand 1 and 1,2-bis(4-pyridinium)ethane/ethylene derivatives 2, 3, and 4. | |
Equimolar acetone solutions of cryptand 1 with viologens 2, 3, and 4 were pale orange due to charge transfer interactions between the electron-rich aromatic rings of the host and the electron-poor pyridinium rings of the guests, which was a direct evidence for host–guest complexation. All of these three host–guest complexes were of 1
:
1 stoichiometry in solution as demonstrated by Job plots10 (Fig. S2–S4, ESI†) based on UV-vis spectroscopy absorbance data in acetone. This was further confirmed by the electrospray ionization mass spectrometry (ESI-MS): m/z 546.9 for [1 ⊃ 2 − 2PF6]2+ (Fig. S5, ESI†); m/z 470.9 for [1 ⊃ 3 − 2PF6]2+ (Fig. S6, ESI†); m/z 469.6 for [1 ⊃ 4 − 2PF6]2+ and m/z 1084.0 for [1 ⊃ 4 − PF6]+ (Fig. S7, ESI†). Moreover, ESI-MS showed a single peak at m/z 834.6 corresponding to [12 ⊃ 3 − 2PF6]2+ (Fig. S6, ESI†), indicating the [3]pseudorotaxane formation in gaseous phase.
By probing the charge transfer bands of the complexes by UV-vis spectroscopy and employing a titration method, the association constants (Ka) were determined: 4.65 (±0.22) × 102 M−1 for 1 ⊃ 2 (Fig. S8, ESI†), 1.69 (±0.23) × 103 M−1 for 1 ⊃ 3 (Fig. S9, ESI†), and 2.16 (±0.21) × 105 M−1 for 1 ⊃ 4 (Fig. S10, ESI†), respectively. It was obvious that the Ka value of 1 ⊃ 4 was much higher than those of 1 ⊃ 2 and 1 ⊃ 3, which indicated that vinylogous viologen 4 was a stronger electron-acceptor than the viologen derivatives 2 and 3. Furthermore, the proton NMR spectra of equimolar (2.00 mM) acetone-d6 solutions of cryptand 1 with guests 2 and 3 both displayed only one set of peaks, indicating fast-exchange complexation on the 1H NMR time scale (Fig. 1, spectra a–e). After complexation, protons H1a and H1b on host 1 shifted downfield, while H1c, H1d, and H1e on host 1 and H2b on guest 2 shifted upfield. The proton NMR spectra of an equimolar (2.00 mM) acetone solution of 1 and 3 was also investigated and displayed similar chemical shift changes (Fig. 1, spectra a–c). However, compared with the spectrum of complex 1 ⊃ 4 (Fig. 3a), only slight chemical shift changes were observed for the protons of guests 2 and 3, indicating that the binding affinity between host 1 and guest 4 is stronger than those of complexes 1 ⊃ 2 and 1 ⊃ 3. These results were consistent with the association constants of these [n]pseudorotaxanes.
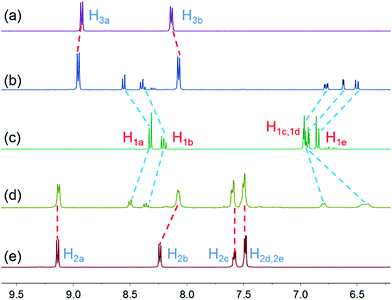 |
| Fig. 1 Partial 1H NMR spectra (acetone-d6, 293 K, 400 MHz): (a) 2.00 mM 3; (b) 2.00 mM 3 + 2.00 mM 1; (c) 2.00 mM 1; (d) 2.00 mM 1 + 2.00 mM 2; (e) 2.00 mM 2. | |
With the purpose of further studying the host–guest complexation and the effects on the formation of [n]pseudorotaxanes caused by minor changes of the guests, a yellow crystal with 1
:
1 complexation stoichiometry between 1 and 2 was obtained via the vapor diffusion of diisopropyl ether into its acetonitrile solution. X-ray analysis of this crystal demonstrated that a [2]pseudorotaxane structure, stabilized by hydrogen bonds between the hydrogen atoms of 2 and ether oxygen atoms of 1, and CH⋯π interactions, indeed formed between host 1 and guest 2 in the solid state (Fig. 2a). While for host–guest system between 1 and 3, X-ray diffraction analysis gave a strong evidence that a [3]pseudorotaxane structure was formed in the solid state (Fig. 2b). The complex is stabilized by twelve hydrogen bonds (h, i, j, k, l, and m), including four water bridge hydrogen bonds (k and j) between host 1 and guest 3, and face-to-face π-stacking between aromatic rings of 1 and 3. It is noteworthy that four hydrogen bonds (m and l) between the ether oxygen atoms of one host and the hydrogen atoms of the other host molecule formed, which favors the formation of a macrocyclic dimer structure. We reasoned that 1 and 2 did not form a [3]pseudorotaxane like 12 ⊃ 3 (Fig. 2b) because the phenyl-substituted viologen derivative 2 appeared as a “zig-zag” shape after complexing with host 1, which prevented it to thread through the second host molecule. Furthermore, the CH⋯π interaction (g) between 1 and 2 also helped to “hold” this [2]pseudorotaxane structure in the solid state.
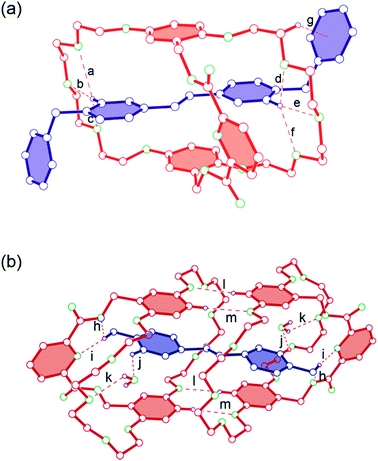 |
| Fig. 2 Ball-stick views of X-ray crystal structures of (a) 1 ⊃ 2 and (b) 12 ⊃ 3. Host 1 is red, guests 2 and 3 are blue, hydrogens are purple, oxygens are green, and nitrogens are sky blue. PF6− counterions and hydrogens except the ones involved in hydrogen bonding between 1 and 2 (or 3) were omitted for clarity. Hydrogen bond parameters are as follows: C⋯O (N) distance (Å), H⋯O (N) distance (Å), C(N)–H⋯O (N) angles (deg): (a) 3.17, 2.84, 101.5; (b) 3.37, 2.45, 162.3; (c) 3.17, 2.51, 126.7; (d) 2.98, 2.44, 115.7; (e) 3.19, 2.26, 164.0; (f) 2.97, 2.67, 98.9; (g) 3.69, 2.89, 138.9; (h) 3.24, 2.64, 120.2; (i) 3.43, 2.45, 174.6; (j) 3.30, 2.58, 133.0; (k) 3.16, 2.53, 132.0; (l) 3.53, 2.59, 175.6; (m) 3.54, 2.59, 175.7. | |
In order to clarify how small changes on guests influence the binding behaviors of the [n]pseudorotaxanes, the complex 1 ⊃ 4 was studied. 1H NMR spectra of an equimolar solution of host 1 and guest 4 showed that no signals corresponding to uncomplexed species were observed, indicating fast exchange on 1H NMR time scale (Fig. 3a). After complexation, peaks corresponding to H1a and H1b of host 1 shifted downfield, while H1c, H1d, and H1e of host 1 and H4a, H4b, and H4c of guest 4 moved upfield due to the shielding effect of the aromatic rings and electron density changes induced by the noncovalent interactions (H-binding and charge-transfer interactions) between cryptand 1 and guest 4, as we observed for 1 ⊃ 2 and 1 ⊃ 3. A yellow crystal of 1 ⊃ 4 was also grown by the same vapor diffusion method. It was interesting that the crystal structure of complex 1 ⊃ 4 showed a [2]pseudorotaxane structure instead of a [3]pseudorotaxane structure in the solid state (Fig. 3b), although guest 4 was very similar to guest 3. The possible reason is that guest 4 is a π-extended viologen derivative and possesses a much more rigid ethylene chain compared with the flexible ethyl chain in guest 3, which facilitates the efficient charge-transfer interaction in a [2]pseudorotaxane-type threaded structure.
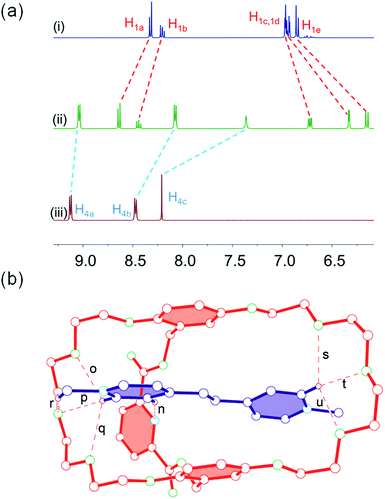 |
| Fig. 3 (a) Partial 1H NMR spectra (acetone-d6, 293 K, 400 MHz): (i) 2.00 mM 1; (ii) 2.00 mM 1 + 2.00 mM 4; (iii) 2.00 mM 4. (b) Ball-stick views of X-ray crystal structure of 1 ⊃ 4. Host 1 is red, guest 4 is blue, hydrogens are purple, oxygens are green, and nitrogens are sky blue. PF6− counterions and hydrogens except the ones involved in hydrogen bonding between 1 and 4 were omitted for clarity. Hydrogen bond parameters are as follows: C⋯O (N) distance (Å), H⋯O (N) distance (Å), C(N)–H⋯O (N) angles (deg): (n) 3.58, 2.68, 159.7; (o) 3.07, 2.55, 114.3; (p) 3.32, 2.41, 159.8; (q) 3.00, 2.65, 102.1; (r) 3.55, 2.62, 157.5; (s) 3.11, 2.60, 113.8; (t) 3.20, 2.26, 154.4; (u) 3.04, 2.63, 106.5. | |
In summary, we studied the recognition of BPP34C10-based cryptand 1 to three 1,2-bis(4-pyridinium)ethane/ethylene derivatives (2–4) with minor structural differences. They revealed different association constants in solution and different binding behaviors and geometries in the solid state. By self-assembly of host 1 with guests 2 and 4, the [2]pseudorotaxane-type threaded structures were formed in the solid state. However, the [3]pseudorotaxane-type host–guest complex 12 ⊃ 3 was obtained in the solid state. The association constant values showed that guest 4 bound host 1 much stronger than guests 2 and 3. These phenomena demonstrated that small structural or geometrical changes on guests could result in large effects on binding behaviors between hosts and guests, thereby leading to big differences in the configurations of the resulting [n]pseudorotaxanes. These different binding behaviors induced by small structure changes can be used to efficiently construct self-sorting system and further to fabricate more complex and highly ordered supramolecular materials with fascinating properties, distinct topologies, and novel function.
Acknowledgements
This work was supported by the Fundamental Research Funds for the Central Universities for financial support.
Notes and references
-
(a) V. Serreli, C. F. Lee, E. R. Kay and D. A. Leigh, Nature, 2007, 445, 523–527 CrossRef CAS PubMed;
(b) C. Zhang, S. Li, J. Zhang, K. Zhu, N. Li and F. Huang, Org. Lett., 2007, 9, 5553–5556 CrossRef CAS PubMed;
(c) F. Wang, C. Han, C. He, Q. Zhou, J. Zhang, C. Wang, N. Li and F. Huang, J. Am. Chem. Soc., 2008, 130, 11254–11255 CrossRef CAS PubMed;
(d) Z. Niu and H. W. Gibson, Chem. Rev., 2009, 109, 6024–6046 CrossRef CAS PubMed;
(e) L. M. Klivansky, G. Koshkakaryan, D. Cao and Y. Liu, Angew. Chem., Int. Ed., 2009, 48, 4185–4189 CrossRef CAS PubMed;
(f) J. A. Foster and J. W. Steed, Angew. Chem., Int. Ed., 2010, 49, 6718–6724 CrossRef CAS PubMed;
(g) J. E. Beves, B. A. Blight, C. J. Campbell, D. A. Leigh and R. T. McBurney, Angew. Chem., Int. Ed., 2011, 50, 9260–9327 CrossRef CAS PubMed;
(h) Z. Zhang, H. Zhang, H. Wang and Y. Liu, Angew. Chem., Int. Ed., 2011, 50, 10834–10838 CrossRef CAS PubMed;
(i) Z. Zhang, Y. Luo, J. Chen, S. Dong, Y. Yu, Z. Ma and F. Huang, Angew. Chem., Int. Ed., 2011, 50, 1397–1401 CrossRef CAS PubMed;
(j) L. Chen, Y. Tian, Y. Ding, Y. Tian and F. Wang, Macromolecules, 2012, 45, 8412–8419 CrossRef CAS;
(k) Y. Liu, Z. Wang and X. Zhang, Chem. Soc. Rev., 2012, 41, 5922–5932 RSC;
(l) B. Zheng, M. Zhang, S. Dong, X. Yan and M. Xue, Org. Lett., 2013, 15, 3538–3541 CrossRef CAS PubMed;
(m) S. Li, J. Huang, F. Zhou, T. R. Cook, X. Yan, Y. Ye, B. Zhu, B. Zheng and P. J. Stang, J. Am. Chem. Soc., 2014, 136, 5908–5911 CrossRef CAS PubMed;
(n) Q. Wang, M. Cheng, Y. Zhao, Z. Yang, J. Jiang, L. Wang and Y. Pan, Chem. Commun., 2014, 50, 15585–15588 RSC;
(o) X. Ma and H. Tian, Acc. Chem. Res., 2014, 47, 1971–1981 CrossRef CAS PubMed;
(p) Z. Huang, L. Yang, Y. Liu, Z. Wang, O. A. Scherman and X. Zhang, Angew. Chem., Int. Ed., 2014, 53, 5351–5355 CrossRef CAS PubMed.
-
(a) G. J. E. Davidson and S. J. Loeb, Angew. Chem., Int. Ed., 2003, 42, 74–77 CrossRef CAS PubMed;
(b) J. V. Hernandez, E. R. Kay and D. A. Leigh, Science, 2004, 306, 1532–1537 CrossRef CAS PubMed;
(c) W. Wang and A. E. Kaifer, Angew. Chem., Int. Ed., 2006, 45, 7042–7046 CrossRef CAS PubMed;
(d) S. Nygaard, S. W. Hansen, J. C. Huffman, F. Jensen, A. H. Flood and J. O. Jeppesen, J. Am. Chem. Soc., 2007, 129, 7354–7363 CrossRef CAS PubMed;
(e) Q. Li, W. Zhang, O. Š. Miljanić, C.-H. Sue, Y.-L. Zhao, L. Liu, C. B. Knobler, J. F. Stoddart and O. M. Yaghi, Science, 2009, 325, 855–859 CrossRef CAS PubMed;
(f) X. Ma and H. Tian, Chem. Soc. Rev., 2010, 39, 70–80 RSC;
(g) W. Yang, Y. Li, J. Zhang, Y. Yu, T. Liu, H. Liu and Y. Li, Org. Biomol. Chem., 2011, 9, 6022–6026 RSC;
(h) C. Li, X. Shu, J. Li, J. Fan, Z. Chen, L. Weng and X. Jia, Org. Lett., 2012, 14, 4126–4129 CrossRef CAS PubMed;
(i) H. Cheng, H. Zhang and Y. Liu, J. Am. Chem. Soc., 2013, 135, 10190–10193 CrossRef CAS PubMed;
(j) K. Wang, C. Wang, Y. Wang, H. Li, C. Bao, J. Liu, S. Zhang and Y. Yang, Chem. Commun., 2013, 49, 10528–10530 RSC;
(k) S. Li, J. Huang, T. R. Cook, J. B. Pollock, H. Kim, K.-W. Chi and P. J. Stang, J. Am. Chem. Soc., 2013, 135, 2084–2087 CrossRef CAS PubMed;
(l) Y.-K. Tian, Y.-G. Shi, Z.-S. Yang and F. Wang, Angew. Chem., Int. Ed., 2014, 53, 6090–6094 CrossRef CAS PubMed;
(m) S. Li, G. Weng, W. Lin, Z. Sun, M. Zhou, B. Zhu, Y. Ye and J. Wu, Polym. Chem., 2014, 5, 3994–4001 RSC;
(n) Y. Yao, K. Jie, Y. Zhou and M. Xue, Chem. Commun., 2014, 50, 5072–5074 RSC;
(o) Q. Zhou, H. Jiang, R. Chen, F. Qiu, G. Dai and D. Han, Chem. Commun., 2014, 50, 10658–10660 RSC;
(p) W. Wang, Y. Zhang, B. Sun, L. Chen, X. Xu, M. Wang, X. Li, W. Jiang and H. Yang, Chem. Sci., 2014, 5, 4554–4560 RSC;
(q) X. Yan, T. R. Cook, J. B. Pollock, P. Wei, Y. Zhang, Y. Yu, F. Huang and P. J. Stang, J. Am. Chem. Soc., 2014, 136, 4460–4463 CrossRef CAS PubMed;
(r) P. Wei, X. Yan and F. Huang, Chem. Soc. Rev., 2015, 44, 815–832 RSC.
-
(a) A. Harada, Acc. Chem. Res., 2001, 34, 456–464 CrossRef CAS PubMed;
(b) F. Huang, L. Zhou, J. W. Jones, H. W. Gibson and M. Ashraf-Khorassani, Chem. Commun., 2004, 2670–2671 RSC;
(c) X.-Z. Zhu and C.-F. Chen, J. Am. Chem. Soc., 2005, 127, 13158–13159 CrossRef CAS PubMed;
(d) F. Huang, L. N. Zakharov, A. L. Rheingold, M. Ashraf-Khorassani and H. W. Gibson, J. Org. Chem., 2005, 70, 809–813 CrossRef CAS PubMed;
(e) H.-B. Yang, K. Ghosh, B. H. Northrop, Y.-R. Zheng, M. M. Lyndon, D. C. Muddiman and P. J. Stang, J. Am. Chem. Soc., 2007, 129, 14187–14189 CrossRef CAS PubMed;
(f) F. Wang, B. Zheng, K. Zhu, Q. Zhou, C. Zhai, S. Li, N. Li and F. Huang, Chem. Commun., 2009, 4375–4377 RSC;
(g) W. Jiang, A. Schäfer, P. C. Mohr and C. A. Schalley, J. Am. Chem. Soc., 2010, 132, 2309–2320 CrossRef CAS PubMed;
(h) L. Zhu, M. Lu, Q. Zhang, D. Qu and H. Tian, Macromolecules, 2011, 44, 4092–4097 CrossRef CAS;
(i) X. Ji, M. Zhang, X. Yan, J. Li and F. Huang, Chem. Commun., 2013, 49, 1178–1180 RSC;
(j) M. Arunachalam and H. W. Gibson, Prog. Polym. Sci., 2014, 39, 1043–1073 CrossRef CAS PubMed;
(k) P. Wei, J. Li, X. Yan and Q. Zhou, Org. Lett., 2014, 16, 126–129 CrossRef CAS PubMed;
(l) Z. Li, Y. Zhang, C. Zhang, L. Chen, C. Wang, H. Tan, Y. Yu, X. Li and H. Yang, J. Am. Chem. Soc., 2014, 136, 8577–8589 CrossRef CAS PubMed;
(m) H. Gong, F. Tang, B. M. Rambo, R. Cao, J. Xiang and J. L. Sessler, Chem. Commun., 2015, 51, 1795–1798 RSC;
(n) Q. Wang, M. Cheng, S. Xiong, X.-Y. Hu, J. Jiang, L. Wang and Y. Pan, Chem. Commun., 2015, 51, 2667–2670 RSC.
-
(a) G. J. E. Davidson, S. Sharma and S. J. Loeb, Angew. Chem., Int. Ed., 2010, 49, 4938–4942 CrossRef CAS PubMed;
(b) Z. Zhang, Y. Luo, B. Xia, C. Han, Y. Yu, X. Chen and F. Huang, Chem. Commun., 2011, 47, 2417–2419 RSC;
(c) X. Yan, Z. Li, P. Wei and F. Huang, Org. Lett., 2013, 15, 534–537 CrossRef CAS PubMed;
(d) B. Zheng, F. Klautzsch, M. Xue, F. Huang and C. A. Schalley, Org. Chem. Front., 2014, 1, 532–540 RSC.
-
(a) F. Huang, K. A. Switek, L. N. Zakharov, F. R. Fronczek, C. Slebodnick, M. Lam, J. A. Golen, W. S. Bryant, P. E. Mason, A. L. Rheingold, M. Ashraf-Khorassani and H. W. Gibson, J. Org. Chem., 2005, 70, 3231–3241 CrossRef CAS PubMed;
(b) J. Zhang, F. Huang, N. Li, H. Wang, H. W. Gibson, P. Gantzel and A. L. Rheingold, J. Org. Chem., 2007, 72, 8935–8938 CrossRef CAS PubMed;
(c) M. Liu, S. Li, M. Hu, F. Wang and F. Huang, Org. Lett., 2010, 12, 760–763 CrossRef CAS PubMed;
(d) X. Yan, X. Chi, P. Wei, M. Zhang and F. Huang, Eur. J. Org. Chem., 2012, 6351–6356 CrossRef CAS PubMed;
(e) P. Wei, Z. Li and B. Xia, Tetrahedron Lett., 2014, 55, 5825–5828 CrossRef CAS PubMed.
-
(a) Z. Niu, C. Slebodnick, D. Schoonover, H. Azurmendi, K. Harich and H. W. Gibson, Org. Lett., 2011, 13, 3992–3995 CrossRef CAS PubMed;
(b) T. Felder and C. A. Schalley, Angew. Chem., Int. Ed., 2003, 42, 2258–2260 CrossRef CAS PubMed.
-
(a) F. Huang and H. W. Gibson, Chem. Commun., 2005, 1696–1698 RSC;
(b) J. Xu, Y. Chen, L. Wu, C. Tung and Q. Yang, Org. Lett., 2014, 16, 684–687 CrossRef CAS PubMed.
-
(a) P. R. Ashton, R. Ballardini, V. Balzani, A. Credi, M. T. Gandolfi, S. Memzer, L. Pérez-García, L. Prodi, J. F. Stoddart, M. A. Venturi, J. P. White and D. J. Williams, J. Am. Chem. Soc., 1995, 117, 11171–11197 CrossRef;
(b) K. Kim, D. Kim, J. W. Lee, Y. H. Ko and K. Kim, Chem. Commun., 2004, 848–849 RSC;
(c) X. Yan, P. Wei, M. Zhang, X. Chi, J. Liu and F. Huang, Org. Lett., 2011, 13, 6370–6373 CrossRef CAS PubMed;
(d) Y. Han, Y. Gu, J. Xiang and C. Chen, Chem. Commun., 2012, 48, 11076–11078 RSC;
(e) X. Chi and M. Xue, RSC Adv., 2014, 4, 365–368 RSC.
-
(a) C.-F. Lin, Y.-H. Liu, C.-C. Lai, S.-M. Peng and S.-H. Chiu, Angew. Chem., Int. Ed., 2006, 45, 3176–3181 CrossRef CAS PubMed;
(b) C.-F. Lin, C.-C. Lai, Y.-H. Liu, S.-M. Peng and S.-H. Chiu, Chem.–Eur. J., 2007, 13, 4350–4355 CrossRef CAS PubMed.
- P. Job, Ann. Chim., 1928, 9, 113–203 CAS.
Footnote |
† Electronic supplementary information (ESI) available: Determination of association constants, ESI-MS, X-ray crystallographic files (CIF) for 1 ⊃ 2, 12 ⊃ 3, and 1 ⊃ 4, and other materials. CCDC 1046153–1046155. For ESI and crystallographic data in CIF or other electronic format see DOI: 10.1039/c5ra06927k |
|
This journal is © The Royal Society of Chemistry 2015 |