DOI:
10.1039/C5RA05643H
(Review Article)
RSC Adv., 2015,
5, 38892-38905
Coumarins as privileged scaffold for anti-inflammatory drug development
Received
30th March 2015
, Accepted 20th April 2015
First published on 20th April 2015
Abstract
Several literature reports had highlighted the significance of coumarin nucleus as a source of potential candidates for anti-inflammatory drug development. Various natural coumarins such as umbelliferone, scopoletin, visnadin, marmin and esculetin demonstrated potent anti-inflammatory activity through various mechanisms. Keeping in view the importance of naturally occurring coumarins, researchers have extensively explored these by synthesizing various derivatives as anti-inflammatory agents. The present review describes different synthetic modifications carried out on coumarin nucleus, assessment of their anti-inflammatory potential and structure–activity relationship (SAR) study, reported during 1992–2014. SAR may help medicinal chemists in rational design of future anti-inflammatory molecules based on coumarin scaffold.
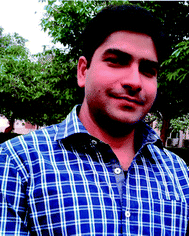 Jagdeep GroverJagdeep | Jagdeep Grover was born in 1988 in Kotkapura city, Punjab, India. He received his B. Pharm. from Guru Nanak Dev University (GNDU), Amritsar in June 2009, and his M. S. (Pharm.) from National Institute of Pharmaceutical Education and Research (NIPER), Mohali, India in June 2011. In July 2011, he joined Ph.D. program under the supervision of Professor Sanjay M. Jachak at NIPER Mohali. Currently he is a fourth year Ph.D. student working on design and synthesis of natural product analogues and their biological evaluation for anti-inflammatory activity. |
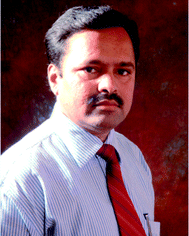 Sanjay M. JachakSanjay | Sanjay M. Jachak is Professor in the Department of Natural Products, National Institute of Pharmaceutical Education and Research (NIPER), Mohali, Punjab, India. He received Ph.D. in Pharmacy (specialization: phytochemistry) from Karl Franzens University, Graz, Austria in 1997. He was lecturer at the College of Pharmacy, Nashik, India from 1998–1999. He joined NIPER, Mohali as Assistant Professor of Natural Products in 1999 and became Professor in 2011. His research interests include characterization of anti-inflammatory and antimycobacterial (anti-TB) natural products, design and synthesis of bioactive natural products and standardization of herbal drugs and their formulations. |
Introduction
Inflammation is caused by a variety of stimuli including physical damage, microbial invasion, ultra violet irradiation, and immune reactions. Redness, heat, swelling, and pain are classical symptoms of inflammation. Redness and heat are caused by the increased blood flow whereas swelling occurs due to the increased movement of fluid and white blood cells into the area of inflammation. Release of chemical mediators and compression of nerves in vicinity of the inflammatory process causes pain.1 If not controlled; inflammation cascades can lead to the development of diseases such as rheumatoid arthritis, chronic asthma, multiple sclerosis, inflammatory bowel disease, and psoriasis. Many of these diseases are debilitating and are becoming increasingly common in our aging society. Rheumatoid arthritis, an inflammatory condition of multiple joints, affects 0.3–1.0% of the general population and is more prevalent among women in developed countries. Osteoarthritis affects 9.6% of men and 18% of women aged more than 60 years. Increases in life expectancy and aging populations are expected to make osteoarthritis the fourth leading cause of disability by the year 2020.2,3 Chronic inflammation leads to cancer, which is witnessed by recent experimental and clinical studies. It has been estimated that 15–20% of all cancer deaths resulted from underlying infections and inflammatory reactions.4 The inflammatory cascade involves a series of events and various inflammatory mediators. The major anti-inflammatory targets include enzymes, cyclooxygenase (COX-1 and COX-2), 5-lipooxygenase (5-LOX), inducible nitric oxide synthase (iNOS), inosine monophosphate dehydrogenase; cytokines and cytokine receptors, tumor necrosis factor (TNF)-α and TNF-RII, interleukin (IL)-1β and IL-1RA, IL-2 and IL-2R, interferon (IFN)-α2, IFN-β1, and IFN-γ.5,6
Natural products being structurally most diverse compounds have engrossed substantial attention from medicinal chemists for the development of anti-inflammatory agents.7–9 Coumarins (also known as 1,2-benzopyrone or, less commonly, as o-hydroxycinnamic acid-8-lactone), constitute an important class of natural products, mainly found in Rutaceae and Umbelliferae family. These compounds are broadly classified as, simple coumarins, furanocoumarins, pyranocoumarins, biscoumarins, triscoumarins, and coumarinolignans. Coumarins have been reported to possess a wide range of biological activities including, antimicrobial, antiviral, anticancer, anti-inflammatory, antioxidant, and anticoagulant. To date, more than 1300 such compounds have been identified.10 Coumarins like esculetin, fraxetin, daphnetin and other related coumarin derivatives recognized as inhibitors of not only LOX and COX enzymes, but also of the neutrophil-dependent superoxide anion generation. Many research groups had reported that various coumarin derivatives block inflammation by inhibiting various targets. However, no such molecule has made its way to the clinics so far.11 Fylaktakidou et al. (2004) reviewed the potential of various natural and synthetic coumarin derivatives as anti-inflammatory and antioxidant molecules.12 Curini et al. (2006) described an array of biological activities of prenyloxycoumarins and prenyloxyfuranocoumarins.13 Recently, Bansal et al. (2013) discussed coumarin as a potential nucleus for development of anti-inflammatory molecules.14 In addition to anti-inflammatory properties, coumarins have also been reviewed as potential anticancer15,16 and monoamine oxidase inhibitors.17 All these reviews presents an comprehensive discussion on the biological aspects of coumarins, but none of them have attempted to present chemical classification of these derivatives, which could assist medicinal chemist to design future anti-inflammatory agents, based on coumarin scaffold. Further their effect on various anti-inflammatory targets such as IL-6, COX-2, TNF-α, LOX, iNOS, etc. has been summarized. In this context, we performed critical analysis of literature reports published on coumarin derivatives as anti-inflammatory agents till December 2014 and presented a classification of these derivatives on the basis of chemical modifications. Further, anti-inflammatory activity of these coumarins is being discussed with structural–activity relationships (SAR), as per the following scheme:
1. Naturally occurring coumarins
2. Synthetically derived coumarin derivatives
2.1 Alkyl derivatives
2.2 Pyrazole and isoxazole derivatives
2.3 Oxadiazole and thiazole derivatives
2.4 Schiff base, Mannich base and amide derivatives
2.5 Benzofuran derivatives
2.6 Miscellaneous derivatives
1. Naturally occurring coumarins
Coumarins are widely distributed in plant kingdom and are present in notable amounts in Rutaceae and Umbelliferae families. Maximum contents are found in fruits, roots, stems, and leaves of these plant families. The naturally derived coumarins have been found to exert anti-inflammatory action through various mechanisms such as inhibition of COX and LOX, reducing edema formation by stimulating phagocytosis and inhibition of iNOS etc.10,11,13 Umbelliferone (1), a simple coumarin isolated from Justicia pectoralis elicited a significant anti-edema effect in carrageenan-induced rat paw edema assay.18 Silvan et al. reported four hydroxylated and methylated coumarins namely esculetin (2), scopolin (3), herniarin (4) and scopoletin (5) from Santolina oblongifolia. These four coumarins inhibited thromboxane B2 (TXB2) release in platelets with the percentages of inhibition being slightly lower than the positive control, ibuprofen (Table 1).19 Scopoletin (5), a well-known anti-inflammatory coumarin from Erycibe obtusifolia has been found to ameliorate clinical symptoms of rat adjuvant-induced arthritis. It down regulated the overexpression of vascular endothelial growth factor, basic fibroblast growth factor and IL-6 in the synovial tissues of adjuvant-induced arthritic rats.20 Menghini et al. reported isolation of three pyranocoumarins (6–8) and a prenyloxycoumarin (9) from Ligusticum lucidum (Fig. 1) and evaluated for anti-inflammatory potential in croton oil-induced ear dermatitis in mice. Visnadin (8) and (R)-(+)-7-(2′,3′-epoxy-3′-methylbutoxy)-coumarin (9) displayed potent topical anti-inflammatory activity which was modulated by substituents on their aromatic ring. Presence of 7-(2′,3′-epoxy-3′-methylbutoxy) group on coumarin nucleus led to higher anti-inflammatory activity, whereas that of a saturated pyrano-fused ring (selidinin, 6 and (+)-praeruptorin A,7) is associated with a lower anti-inflammatory effect.21 Nazari et al. described an anti-inflammatory activity of sesquiterpene–coumarins from Ferula species including fukanemarin B, fukanefuromarins E–G, umbelliprenin and 8′-acetoxy-5′-hydroxyumbelliprenin.22 Bioassay-guided isolation from Angelica decursiva revealed umbelliferone 6-carboxylic acid (10) as a potential inhibitor of NO production in RAW 264.7 cells with an IC50 value of 72.98 μg mL−1.23 Recently, antioxidant and anti-inflammatory effects of six coumarins; scopoletin (5), scoparone (11), fraxetin (12), 4-methyl-umbeliferone (13), esculin (14) and daphnetin (15) were investigated (Table 1) and esculin, scoparone and daphnetin were found to be most active among these coumarins.24 In addition, esculetin (2), fraxetin (12) and daphnetin (15) are bestowed with potent 5-LOX inhibition with IC50 of 8.3, 75 and 20 μM respectively.11 Columbianetin (16) and libanoridin (17), two coumarins isolated from Corydalis heterocarpa were evaluated for anti-inflammatory effects in HT-29 human colon carcinoma cells. Libanoridine displayed significant inhibition of inflammatory mediators such as iNOS, COX-2, TNF-α, and IL-1β, whereas columbianetin did not show any activity.25
Table 1 Anti-inflammatory activity of naturally occurring coumarinsc
Target/activity |
Dose/concentration |
Compound |
% Inhibition |
Ref. |
IC50 (μg mL−1). Value in parenthesis defines particular hour at which edema inhibition was measured. CPE: carrageenan induced rat paw edema. |
CPE |
20 mg kg−1 |
1 |
36 (3 h)b |
18 |
TXB2 |
100 μM |
2 |
77.32 |
19 |
3 |
73.21 |
4 |
76 |
5 |
71.47 |
Croton oil-induced ear dermatitis |
0.3 μmol cm−2 |
6 |
27 |
21 |
7 |
22 |
8 |
43 |
9 |
68 |
NO production (RAW 264.7 cells) |
|
10 |
72.98a |
23 |
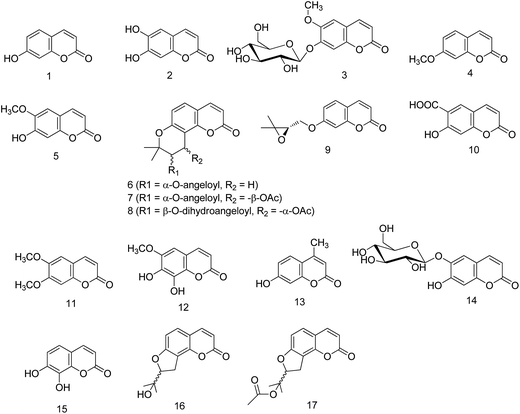 |
| Fig. 1 Chemical structures of naturally occurring coumarins (Class 1). | |
2. Synthetically derived coumarin derivatives
2.1. Alkyl derivatives
This class mainly includes ether, ester, alkyl or aryl substituted derivatives of coumarin and their SAR for anti-inflammatory activity. During the last decade, many derivatives bearing alkyl/aryl substituents mainly at C-4, C-6 and C-7 position of coumarin ring were synthesized (Fig. 2) and evaluated for anti-inflammatory activity (Table 2). Jackson et al. synthesized ether derivatives of coumarin (18–21) as inhibitors of iNOS. Various modifications were carried out at C-4 and C-6 of coumarin to study SAR. Compounds substituted with halogens at C-6 showed better activity as compared to unsubstituted compounds. Within halogens, chlorine substitution (compound 18) was preferred over other halogens. Moreover, single basic nitrogen with the primary substituted aminopropyl functionality was favored. Compound 18 emerged as most active derivative with IC50 of 60 nM for mouse iNOS and 185-fold and 9-fold selectivity for bovine endothelial and, rat neuronal nitric oxide synthase respectively. Further, it also exhibited inhibition of human iNOS by competing with arginine at the substrate binding site with a Ki of 104 nM.26 Lin et al. prepared a series of 6-acyl-4-aryl/alkyl-5,7-dihydroxycoumarins as inhibitors of NO production in LPS-induced RAW 264.7 cells. From this series, one compound (23) emerged as most potent inhibitor of NO production with an IC50 value of 7.6 μM. The study suggested that isovaleryl and tert-butylacetyl substituents (22–26) at C-6 position of coumarin ring are crucial for the activity. In contrast, substituents at C-4 position had less influence on inhibiting NO production in RAW 264.7 cells.27
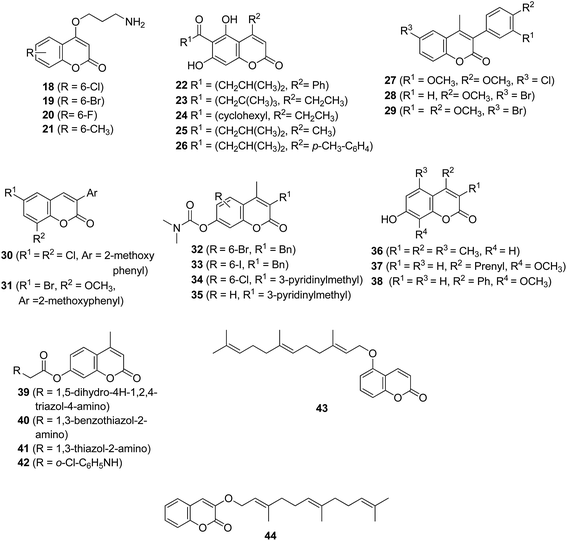 |
| Fig. 2 Chemical structures of alkyl derivatives of coumarin (Class 2.1). | |
Table 2 Anti-inflammatory activity of alkyl derivativesa
Target/activity |
Dose/concentration |
Compound |
IC50 (μM) |
Ref. |
% Inhibition, –: No significant inhibition, CPE: carrageenan induced rat paw edema. |
iNOS |
|
18 |
0.061 |
26 |
19 |
0.143 |
20 |
0.441 |
21 |
0.304 |
NO production (RAW 264.7 cells) |
|
22 |
9.0 |
27 |
23 |
7.6 |
24 |
13.0 |
25 |
9.3 |
26 |
12.1 |
30 |
8.5 |
29 |
31 |
6.9 |
Lipoxygenase |
100 μM |
27 |
12a |
28 |
28 |
—a |
29 |
86a |
TNF-α |
|
32 |
0.06 |
30 |
33 |
0.06 |
34 |
0.45 |
35 |
2.7 |
NO production (J774 macrophage) |
10 μM |
36 |
51.8a |
32 |
37 |
57.0a |
38 |
71.5a |
CPE |
20 mg kg−1 |
39 |
27.6 (3 h)a |
33 |
40 |
40.4 (3 h)a |
41 |
47.8 (3 h)a |
42 |
12.8 (3 h)a |
Lipoxygenase |
|
43 |
0.8 |
34 |
44 |
1.7 |
To gain more in-depth understanding of effect of structural variations on coumarin, a novel series of 3-arylcoumarin derivatives were explored as LOX inhibitors. Within this series (27–29), compound 29 exhibited 86% inhibition of soybean LOX at 100 μM concentration, more than that exhibited by reference compound nordihydroguaiaretic acid (40%). This study has corroborated the significance of halogen substituent at C-6 position of coumarin as an essential structural feature to demonstrate anti-inflammatory activity, as discussed for compounds 18–21.28 Recently Zhang et al. introduced more variations on 3-arylcoumarins and studied nitric oxide production inhibitory activity in lipopolysaccharide-activated mouse macrophage RAW264.7 cells. The study revealed that presence of aromatic ring at C-3 position retains the anti-inflammatory activity of coumarin. Compounds 30 and 31 displayed IC50 of 8.5 and 6.9 μM respectively; comparable with that of curcumin (6.2 μM, positive control).29 Cheng et al. reported coumarin-based carbamates as TNF-α inhibitors (32–35). Various modifications were carried out surrounding the coumarin core structure to optimize in vitro inhibitory activity of TNF-α production by human peripheral blood mononuclear cells (hPBMC). The compounds 32 and 33 exhibited IC50 values of 0.06 μM against TNF-α. SAR revealed hydrophobic group at C-3 favoured TNF-α inhibition and activity increased with the size of alkyl group at this position. Benzyl and 3-pyridinylmethyl group at C-3 position was well tolerated however; removal or extension of methylene link to ethylene abolished the activity. The study further strengthens the importance of 6-halo-substituent for inhibition of TNF-α, as previously described for other targets. Moreover, compound 34 demonstrated 86% inhibition of TNF-α at a dose of 100 mg kg−1 administered subcutaneously in rats.30
It has been reported that 7-hydroxycoumarin is the active metabolite of coumarins in human beings and reported activities of different coumarins may be related to their metabolites.31 So keeping this rationale in background, Timonen et al. reported 7-hydroxycoumarin derivatives as potential anti-inflammatory candidates. Three compounds (36–38) inhibited LPS-induced NO production by more than 50% at the lower concentration tested (10 μM). Compound 36 also displayed 92% inhibition of LPS-induced IL-6 production in J774macrophages at 100 μM concentration. The study revealed the importance of phenyl ring at C-4, as its replacement with prenyl substituent (37) decrease the activity; however methoxy group at C-8 (37 and 38) was not required for activity.32 To investigate the effect of substituents at C-7 of coumarin, derivatives bearing either single substituent or more complex substituent were synthesized. Compounds 39–41 bearing heterocyclic ring in the side chain at C-7, exhibited potent anti-inflammatory activity in carrageenan-induced rat paw edema assay (Table 2). However, replacement of a heterocyclic ring with another substituted phenyl group (compound 42) decreased the activity.33 In order to unravel what could be the effect of longer alkyl chain on 15-LOX inhibition, a series of O-prenylated coumarins were synthesized (Fig. 2). Among the mono isopentenyloxy, -geranyloxy and -farnesyloxy derivatives, farnesyloxy compounds (43, 44) displayed much potent activity against 15-LOX inhibition.34
2.2. Pyrazole and isoxazole derivatives
Pyrazole, a component of many non-steroidal anti-inflammatory drugs (NSAIDs) such as mefobutazone, ramifenazone, famprofazone and celecoxib, has attracted the attention of medicinal chemists for future discovery of anti-inflammatory agents.35 As both coumarins and pyrazoles possess anti-inflammatory activities, researchers across the world have explored these scaffolds in the pursuit of discovering new synthetic derivatives as COX-2 inhibitors and anti-inflammatory agents (Fig. 3, Table 3). Emmanuel-Giota et al. reported a series of 3-(coumarin-4-yl)dihydropyrazoles as soybean LOX inhibitors. In this series, compound 45 showed weak inhibition of enzyme (24% inhibition) at 0.3 mM concentration. Further, this compound also exhibited anti-inflammatory activity (43.6 percent inhibition of edema formation) at a dose of 0.15 mmol per kg body weight.36
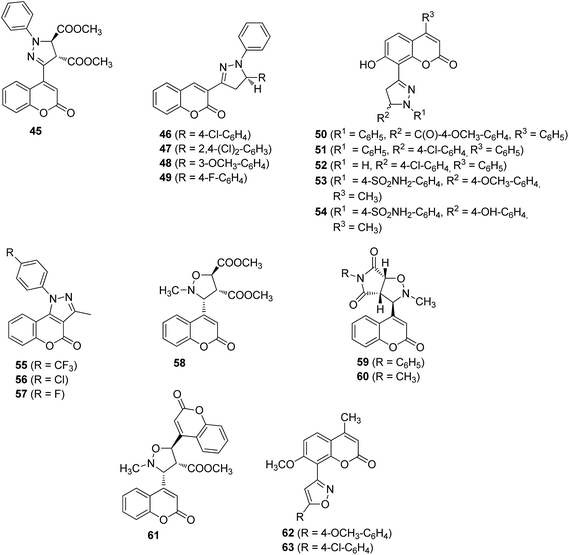 |
| Fig. 3 Chemical structures of pyrazole and isoxazole derivatives of coumarin (Class 2.2). | |
Table 3 Anti-inflammatory activity of pyrazole and isoxazole derivativesb
Target/activity |
Dose/concentration |
Compound |
% Inhibition |
Ref. |
IC50 (μM). CPE: carrageenan induced rat paw edema. |
Lipoxygenase |
0.3 mM |
45 |
24 |
36 |
60 |
17.8 |
CPE |
0.15 mmol kg−1 |
45 |
43.6 |
36 |
200 mg kg−1 |
46 |
64.7 (4 h) |
37 |
47 |
67.5 (4 h) |
48 |
61.5 (4 h) |
49 |
66.7 (4 h) |
37 mg kg−1 |
50 |
18.85 |
38 |
31 mg kg−1 |
51 |
17.40 |
33 mg kg−1 |
52 |
18.85 |
20 mg kg−1 |
53 |
10.6 (5 h) |
39 |
54 |
30.6 (5 h) |
150 μmol kg−1 |
55 |
59.8 (5 h) |
40 |
56 |
49.4 (5 h) |
57 |
55.6 (5 h) |
COX-2 |
|
55 |
1.79a |
56 |
2.43a |
57 |
2.36a |
Considering the importance of diaryheterocylic scaffolds as coxibs and potential anti-inflammatory candidates, a series was prepared by replacing one aryl ring with coumarin. Of these, compound 47 demonstrated highest anti-inflammatory activity in carrageenan-induced paw edema assay. Compound 46, 48 and 49 also displayed significant edema reduction ranging from 61–66 percent at the same dose after 4 h. In addition, compound 47 was found to possess substantial chronic anti-inflammatory activity (45.4 percent inhibition of edema at 200 mg kg−1 on day 19) when evaluated in adjuvant-induced arthritis in rats. These compounds (46–49) caused less gastric ulceration (ulcer index ranges from 1.6 to 2.7) and disruption of gastric epithelial cells at the abovementioned dose as compared to aspirin (ulcer index 4.3).37 In the continuing efforts to develop potent anti-inflammatory molecules, Eissa et al. had synthesized coumarins with novel pyrazole substitution at C-8 position. Compounds 50–52 displayed significant anti-inflammatory activity (Fig. 3 and Table 3). Compound 50 was also found to possess significant analgesic activity (41.23 percent protection against writhing at 1 h) in acetic acid-induced writhing method. Molecular docking study of 50 revealed an important interaction of 7-OH group with Tyr-355 of COX-2, indicating its affinity toward the enzyme.38 A series comprising 1,3,5-trisubstitutedpyrazolines bearing benzene sulfonamide substituent, linked with coumarin at C-8 position was designed and synthesized as anti-inflammatory molecules. However, none of these compounds showed potent anti-inflammatory activity except for compounds 53 and 54 (Table 3) in carrageenan induced rat paw edema assay.39 Inspired from the aforesaid advancement in pyrazole derivatives linked to coumarin, we have synthesized chromenopyrazole derivatives (55–57) as selective COX-2 inhibitors and potential anti-inflammatory agents. The study highlighted the importance of halogen substituents specially p-CF3 group in N-phenyl ring, as a pharmacophoric feature for COX-2 selectivity and anti-inflammatory activity. Further, molecular docking studies predicted the interaction of these ligands with Tyr-355 at the active site of COX-2, for directing COX-2 selectivity.40
Besides pyrazole, some 3-(coumarin-4-yl)tetrahydroisoxazoles were synthesized and evaluated as soybean LOX inhibitors. Only compound 60 was found to possess LOX inhibitory activity, whereas compounds 58, 59 and 61 did not inhibit LOX. SAR revealed that closure of second ring decreased the anti-inflammatory efficacy.36 Sandeep et al. (2009) reported a series of widely substituted 7-methoxy-4-methyl-8-[5-(substituted aryl)isoxazol-3-yl]-coumarins wherein the compounds 62 and 63 (Fig. 3) demonstrated significant anti-inflammatory activity.41
2.3. Oxadiazole and thiazole derivatives
As aforementioned pyrazole and isoxazole derivatives contributed significantly towards anti-inflammatory lead development, oxadiazole, and thiazoles are other important five membered heterocyclic scaffolds that have been widely studied for their biological activities including anti-inflammatory activity. Herein we present an account of these classes of compounds for anti-inflammatory potential and their SAR (Fig. 4, Table 4). Nicolaides et al. synthesized some 4-(1,2,4-oxadiazolyl) coumarins (64 and 65) and evaluated for in vivo anti-inflammatory and 12-LOX inhibitory activity in vitro. Compound 65 showed significant anti-inflammatory activity (64.6%), higher than that of indomethacin (53.3%). However, none of the compounds were found to be active in 12-LOX assay, which suggest a possible COX inhibitory activity.42 Subsequently, this group further illustrated the versatility at C-4 position of coumarin by synthesizing another series of 3-(coumarin-4-yl)-1,2,4-oxadiazoles (66–70). Within this series, compounds 66, 67 and 70 exhibited significant anti-inflammatory activity in vivo (65–75% inhibition of edema). However, only compound 66 exhibited in vitro LOX inhibitory activity. The study again corroborates different mechanism of action for these compounds other than that of LOX inhibition.43 Recently Akhter et al. reported a series of 3-(5-phenyl/phenylamino-[1,3,4]-oxadiazol-2-yl)-chromen-2-one (71–76) and N-[5-(2-oxo-2H-chromen-3-yl)-[1,3,4]oxadiazol-2-yl]-benzamide derivatives (77). All these compounds exhibited significant anti-inflammatory and COX-1 and COX-2 inhibitory activity. Compound 74 emerged as the most potent as it showed 89% inhibition of rat paw edema and COX-2 selectivity in vitro (COX-1 IC50 = 41.6 μM; COX-2 IC50 = 0.4 μM, SI = 104). It also demonstrated significant activity against 5-LOX and 15-LOX. In addition, it also showed analgesic and antioxidant activity. SAR revealed that chloro substitution at p-phenyl ring at fifth position of oxadiazole (72) was a determinant for anti-inflammatory activity. Replacement of chloro with methyl (73) or methoxy group adversely affected the activity. However, activity indeed increased by adding another chloro group (74) on the phenyl ring that signify the importance of electron withdrawing groups. Compounds (75–77) exhibited comparatively less activity, which may be due to increase in distance between the oxadiazole nucleus and phenyl ring due to presence of linker groups such as –NH–, and –NHCO– between the two rings.44
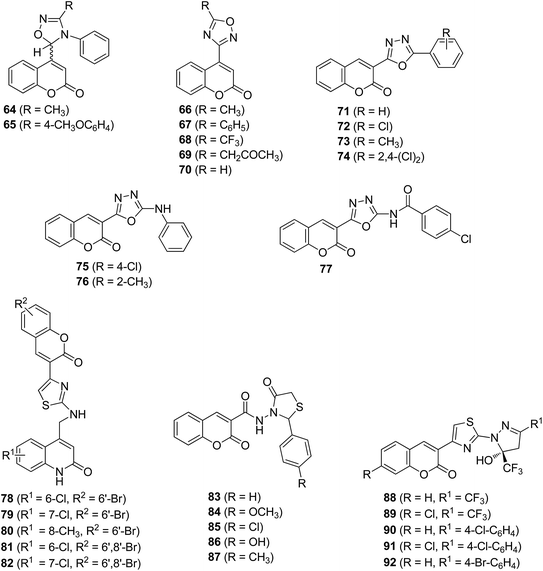 |
| Fig. 4 Chemical structures of oxadiazole and thiazole derivatives of coumarin (Class 2.3). | |
Table 4 Anti-inflammatory activity of oxadiazole and thiazole derivativesb
Target/activity |
Dose/concentration |
Compound |
% Inhibition |
Ref. |
IC50 (μM). CPE: carrageenan induced rat paw edema. |
CPE |
0.15 mmol kg−1 |
64 |
58.5 (3.5 h) |
42 |
65 |
64.6 (3.5 h) |
66 |
68.4 (3.5 h) |
43 |
67 |
75.9 (3.5 h) |
70 |
65.0 (3.5 h) |
20 mg kg−1 |
71 |
80 (3 h) |
44 |
72 |
86 (3 h) |
73 |
75 (3 h) |
74 |
89 (3 h) |
75 |
74 (3 h) |
76 |
72 (3 h) |
77 |
77 (3 h) |
100 mg kg−1 |
78 |
28.57 (8 h) |
45 |
79 |
24.48 (8 h) |
80 |
18.52 (8 h) |
81 |
21.85 (8 h) |
82 |
41.47 (8 h) |
50 mg kg−1 |
88 |
83.10 (1 h) |
47 |
89 |
85.91 (1 h) |
90 |
77.46 (1 h) |
91 |
73.24 (1 h) |
92 |
76.06 (1 h) |
Acetic acid induced writhing |
100 mg kg−1 |
83 |
37.80 |
46 |
84 |
20.48 |
85 |
24.97 |
86 |
16.02 |
87 |
13.72 |
COX-2 |
|
71 |
8.7a |
44 |
72 |
1.2a |
73 |
1.18a |
74 |
0.4a |
75 |
>100a |
76 |
36.6a |
77 |
>100a |
Triheterocyclicthiazoles containing coumarin and carbostyril (1-aza coumarin, 78–82) were reported as anti-inflammatory agents (Fig. 4). All these compounds were found to be slow acting anti-inflammatory agents as they exhibited activity after 4 h till 8 h in carrageenan induced rat paw edema assay. Among these, compound 78 showed 34% and 41% inhibition of edema at 4 and 8 h respectively. In contrast, all compounds displayed significant analgesic activity. Qualitative SAR studies revealed that the chloro substitution at C-7 in carbostyril and 6,8-dibromo substitution in the coumarin ring enhanced anti-inflammatory activity.45 Another series of coumarin based thiazolidin-4-ones was prepared and evaluated for analgesic activity. Among these, compound 83 and 85 displayed potent analgesic activity, whereas remaining compounds (84, 86 and 87) elicited moderate to weak activity.46 Aggarwal et al. evaluated anti-inflammatory potential of various coumarin analogs bearing substituted thiazole ring at C-3 position of coumarin. All the compounds (88–92, Fig. 4) displayed potent anti-inflammatory activity in carrageenan induced rat paw edema assay (Table 4). The study revealed that compounds act as fast acting anti-inflammatory agents. Compounds 88 and 89 emerged as most active compounds.47
2.4. Schiff base, Mannich base and amide derivatives
Maddi et al. reported a series of substituted 3-(benzylideneamino)coumarins as anti-inflammatory agents (93–95). Of this series, compounds 93 and 95 exhibited 60 and 75% inhibition of carrageenan-induced paw edema at 3 h respectively. These also displayed significant analgesic activity in acetic acid-induced writhing in mice model.48 Subsequently, Nicolaides et al. reported novel coumarin-4-carboxamidoximes as potential anti-inflammatory lead molecules (96–98). Compound 96 exhibited more than 80% inhibition of rat paw edema, followed by 97. An increase in biological effect was observed when R = H, was replaced by alkyl or phenyl moieties.43 The importance of azomethine linkage for exhibiting anti-inflammatory activity was further substantiated by Kontogiorgis et al. by preparing coumarin derivatives with a 7-azomethine linkage (99–101, Fig. 5). Compounds 99 and 100 displayed more than 50% inhibition of paw edema (Table 5). Compound 99 with polar substituent at C-7 displayed highest in vivo activity, which signifies the importance of substituent at C-7 of coumarin skeleton.49
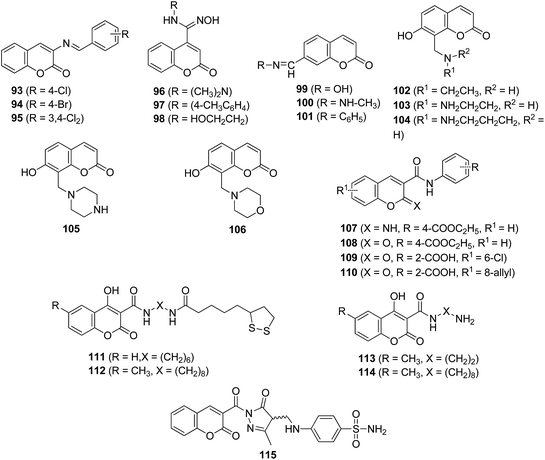 |
| Fig. 5 Chemical structures of Schiff base, Mannich base and amide derivatives of coumarin (Class 2.4). | |
Table 5 Anti-inflammatory activity of Schiff base, Mannich base and amide derivativesa
Target/activity |
Dose/concentration |
Compound |
% Inhibition |
Ref. |
CPE: carrageenan induced rat paw edema. |
CPE |
100 mg kg−1 |
93 |
60.4 (3 h) |
48 |
94 |
55.0 (3 h) |
95 |
74.9 (3 h) |
0.15 mmol kg−1 |
96 |
81.1 (3.5 h) |
43 |
97 |
73.6 (3.5 h) |
98 |
62.3 (3.5 h) |
0.01 mmol kg−1 |
99 |
58.6 (3.5 h) |
49 |
100 |
54.7 (3.5 h) |
101 |
42.5 (3.5 h) |
102 |
53.6 (3.5 h) |
50 |
103 |
56.0 (3.5 h) |
104 |
53.4 (3.5 h) |
105 |
77.7 (3.5 h) |
106 |
75.7 (3.5 h) |
10 mg kg−1 |
107 |
51 (3 h) |
51 |
108 |
48 (3 h) |
109 |
54 (3 h) |
110 |
47 (3 h) |
0.01 mmol kg−1 |
111 |
72 |
52 |
112 |
73 |
113 |
59 |
114 |
59 |
0.03 mmol kg−1 |
115 |
68.86 |
53 |
Keeping 7-OH group intact on coumarin nucleus as an essential feature, same authors have synthesized various Mannich bases at C-8 position (102–106). Compounds 105 and 106 were the most potent as they exhibited 75 and 77% inhibition of paw edema respectively, in comparison to 47% inhibition by indomethacin at the same dose. In addition, these compounds were also devoid of gastrointestinal side effects. SAR suggested that minimum requirement for anti-inflammatory activity of these compounds includes presence of free 7-OH group on coumarin ring and an alicyclic amine (piperazinyl or morpholinyl ring) or a primary aliphatic amine at C-8 position.50 Considering mefenamic and meclofenamic acids as a lead molecule, a series of N-substituted coumarin-3-carboxamide derivatives containing aromatic amino acid residues were synthesized. Of these, four compounds (107–110) were the most active anti-inflammatory agents (Table 5). Compound 109 demonstrated highest rat paw edema inhibition (54%), comparable to that of standard drug, piroxicam (57%).51 Melagraki et al. (2009) reported various coumarin-3-carboxamides and their alpha-lipoic acid conjugates (111–114) to develop a novel hybrid as potent anti-inflammatory lead compounds. The coumarin–lipoic acid conjugates, 111 and 112 were observed as most potent with edema inhibition of 72 and 73% respectively, in vivo. This study indicated an influence of alkyl chain length within coumarin-3-carboxamides, on edema inhibition. In addition, these lipoic acid–coumarin-3-aminoamides hybrids showed higher anti-inflammatory activity than lipoic acid alone.52 Inspired from the recent progress of pyrazole derivatives as anti-inflammatory lead molecules and to alleviate their associated GI side effects, recently a new series of pyrazolone Mannich bases was reported as potent anti-inflammatory agents. Among these, compound 115 showed nearly equipotent anti-inflammatory and analgesic activity as compared to indomethacin, with minimum ulcerogenic index. The study suggested that substituents with low lipophilicity, electron withdrawing power and high polarizability displayed better anti-inflammatory activity.53
2.5. Benzofuran derivatives
Coumarin derivatives substituted with benzofuran ring at C-4 position of coumarin were reported as anti-inflammatory agents (Fig. 6). Within this series, one compound (116) exhibited uniform activity up to 5 h, comparable with standard phenylbutazone (Table 6).54 Taking this compound as a lead, further modifications were carried out on coumarin and benzofuran ring. Compounds 117–119 showed most promising activity, comparable with indomethacin. It was reported that a methoxy (118) and chloro (119) substitution led to increased activity in all cases. Moreover, benzofuranyl ethers were found to possess potent anti-inflammatory activity as compared to simple chromones or 4-hydroxy coumarin, indicating the significance of transformation of aromatic nucleus to heterocyclic moiety.55
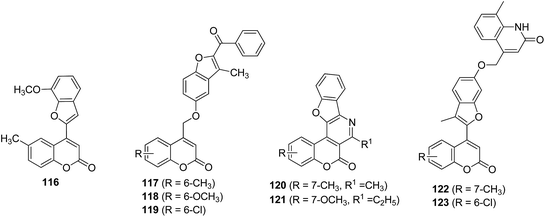 |
| Fig. 6 Chemical structures of benzofuran derivatives of coumarin (Class 2.5). | |
Table 6 Anti-inflammatory activity of benzofuran derivativesa
Target/activity |
Dose/concentration |
Compound |
% Inhibition |
Ref. |
CPE: carrageenan induced rat paw edema. |
CPE |
100 mg kg−1 |
116 |
79 (4 h) |
54 |
116 |
55 (3 h) |
55 |
118 |
59 (3 h) |
119 |
62 (3 h) |
400 mg kg−1 |
120 |
97 (3 h) |
56 |
121 |
67.2 (3 h) |
100 mg kg−1 |
122 |
77 (8 h) |
57 |
123 |
74 (8 h) |
Encouraged by the aforementioned findings, it was envisaged that transformation of tricyclic templates into a pentacyclic system might be associated with interesting biological functions in animal model studies. Based on this rationale, a series of pyridine-fused benzofuranocoumarins was designed and investigated for anti-inflammatory and analgesic properties. Among these, two compounds (120 and 121) emerged as most active compounds showing anti-inflammatory activity in a dose-dependent manner with maximum inhibition up to 97%.56 It was reported that quinolinone derivatives metabolized to the corresponding 8-hydroxycoumarins in biological systems and exhibited significant anti-inflammatory and analgesic activity. So, in view of this observation, Kalkhambkar et al. synthesized coumarin derivatives with quinolinone moiety linked through benzofuran ring (122 and 123), in order to study anti-inflammatory activity of these heterocycles as a single entity. The study revealed that compounds with chlorine substituent (123) displayed highest anti-inflammatory activity.57
2.6. Miscellaneous derivatives
Mazzei et al. synthesized some of the unsymmetrical methylene derivatives of coumarins (Fig. 7) and evaluated for their capacity to reduce the O2− production by activated human neutrophils. Among these compounds 124 and 125 demonstrated 100% inhibition of neutrophil O2− production, when stimulated with formylmethionine–leucine–phenylalanine (f-MLP). These compounds also showed 63 and 56% inhibition of protein kinase C in neutrophils stimulated with phorbolmyristate acetate (PMA) respectively (Table 7). This study revealed the importance of acetoxy group at C-7 of chromone moiety to counteract the neutrophil activation efficiently.58 Some benzo[l]khellactone derivatives were reported as potential anti-inflammatory agents. Among these, two compounds (126 and 127) displayed 89 and 100% inhibition of soybean LOX. Further, these derivatives also showed potent anti-inflammatory activity in carrageenan-induced rat paw edema assay. The study delineates the significance of esterification of hydroxyl group (127) to enhance anti-inflammatory activity.59 Taking into account the significance of 128 as a 5-LOX inhibitor, modifications were done at 2-cyanoquinoline and dioxabicylcooctanyl moiety to furnish coumarin derivatives substituted with fluorophenyl and hexafluorocarbinol groups. The study disclosed bis(trifluoromethyl)carbinol moiety (129) as an excellent surrogate for the metabolically unstable dioxabicyclooctanyl alcohol to exhibit potent in vitro 5-LOX inhibitory activity.60 In quest for the water soluble coumarin derivatives, Pandey et al. reported 4-methyl and 4,8-dimethylcoumarin derivatives by incorporating polyethylene glycol. PEGylated derivatives (130) were found to be more effective than their non-PEGylated analogues to inhibit intercellular cell adhesion molecule-1 (ICAM-1) expression.61 Upadhyay et al. explored 4-styrylcoumarin derivatives as inhibitors of TNF-α and IL-6. Coumarin possessing unsubstituted styryl group (131) disclosed potent IL-6 inhibitory activity. In contrast, substitution of phenyl ring with methoxy group at m-position (132) confers good TNF-α inhibitory activity.62 Considering the worthy and imperative anti-inflammatory potential of coumarins and chalcones, a series of biscoumarin–chalcone hybrids was prepared. The resultant compounds significantly inhibited edema formation in albino rats and also displayed free radical scavenging activities. Of the various biscoumarin–chalcone derivatives, compound 133 showed significant TNF-α inhibition and moderate anti-inflammatory activity (Table 7).63 A series of linearly and angularly fused coumarins has been reported as anti-inflammatory agents. Of these, the compounds (134–137) were found to be endowed with potent antioxidant and anti-inflammatory properties. Compound 137 emerged as most potent anti-inflammatory agent without any ulcerogenic activity.64 Inspired from the clinically used antiulcer drugs based on benzimidazole nucleus and anti-inflammatory activity of 3-substituted coumarins, a new series comprising of coumarins coupled at C-3 position with benzimidazole at 2-position is reported as safer anti-inflammatory agents. The compounds 138, 139 and 140 were associated with potent anti-inflammatory and antioxidant activity. In consonant with anti-inflammatory potential, compounds 139 and 140 exhibited low ulcer index.65 Recently, a novel piperazine based coumarin derivative IMMLG5521 was reported as potent anti-inflammatory lead molecule. This compound exhibited anti-inflammatory effects, reduced pleural exudate formation, polymorphonuclear leukocytes infiltration, and TNF-α, IL-1β, MIP-2 and IL-8 release.66,67 In addition, it also showed hepatoprotective activity in lung inflammation induced by sephadex in rats. The underlying mechanism is down-regulation of VCAM-1 and ICAM-1 expression and TNF-α level.68 Inspired from the worthy anti-inflammatory activities of IMMLG5521, another 3-piperazinylcoumarin compound IMM-H004 was investigated against various pro-inflammatory mediators. It significantly suppressed the release of TNF-α, IL-1β and NO, and suppressed the expression of pro-inflammatory mediators and cytokines such as iNOS, COX-2, and IL-6 in LPS-stimulated BV2 microglia.69
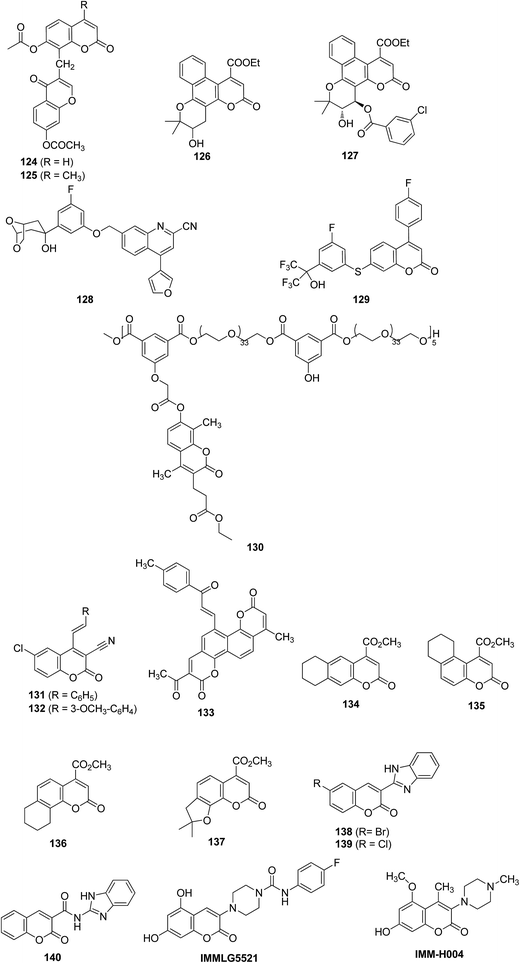 |
| Fig. 7 Chemical structures of miscellaneous coumarin derivatives (Class 2.6). | |
Table 7 Anti-inflammatory activity of miscellaneous coumarin derivativesd
Target/activity |
Dose/concentration |
Compound |
% Inhibition |
Ref. |
IC50 (μM). IC50 (nM). IC50 (μg mL−1). CPE: carrageenan induced rat paw edema. |
Protein kinase C (f-MLF) |
20 μM |
124 |
100 |
58 |
125 |
100 |
Protein kinase C (PKC) PMA |
124 |
63 |
125 |
56 |
Lipoxygenase |
1 mM |
126 |
89.8 |
59 |
127 |
100 |
0.1 mM |
134 |
78 |
64 |
135 |
31 |
136 |
100a |
137 |
4 |
CPE |
0.1 mmol kg−1 |
126 |
48.7 (3.5 h) |
59 |
127 |
53.1 (3.5 h) |
134 |
43.4 (3.5 h) |
64 |
135 |
34 (3.5 h) |
136 |
62 (3.5 h) |
137 |
65 (3.5 h) |
0.056 mmol kg−1 |
138 |
45.4 (6 h) |
65 |
139 |
46.7 (6 h) |
140 |
42.6 (6 h) |
100 mg kg−1 |
133 |
29 (3 h) |
63 |
Human 5-lipoxygenase |
|
128 |
27b |
60 |
129 |
27b |
ICAM-1 |
|
130 |
12.3c |
61 |
IL-6 |
|
131 |
87 |
62 |
132 |
0 |
TNF-α |
10 μM |
131 |
32 |
132 |
73 |
400 μg mL |
133 |
47 |
63 |
Conclusion and future perspectives
The widespread natural distributions and multiple bioactivities of coumarins have intrigued medicinal chemists for decades to engage in research on coumarins. This review article has outlined a variety of modifications carried out on coumarin nucleus with a focus on their anti-inflammatory potential. The aim is to classify the coumarin derivatives on the basis of chemical modifications, which could assist researches in future design of this class of compounds as anti-inflammatory agents. In addition to chemical classification of coumarins, their effect on various anti-inflammatory targets such as IL-6, COX-2, TNF-α, LOX, iNOS, TXB2 etc. has been emphasized. Naturally occurring coumarins (esculetin and fraxetin) as well as synthetic derivatives (compound 27, 28, 29, 43–45, 60 and 134–137) were found to exert anti-inflammatory action generally through inhibition of LOX (5-, 12- and 15-LOX). A farnesyl substituted alkyl derivative (compound 43) showed highest LOX inhibition with IC50 of 0.8 μM. Pyrazole derivatives of coumarin have gained substantial attention in order to develop better anti-inflammatory lead, followed by other heterocyclic systems. Within pyrazole derivatives, compound 47 exhibited highest anti-inflammatory activity in vivo. Two piperazino-coumarin derivatives (compound IMMLG5521and IMM-H004) have also emerged as potent lead molecule for anti-inflammatory drug development. These compounds exhibited anti-inflammatory and hepatoprotective properties by acting on multiple pro-inflammatory mediators such as TNF-α, IL-1β, iNOS, COX-2, and IL-6. The SAR studies revealed suitability of various moieties at different position of coumarin. C-3 and C-7 position of coumarin was found to be extensively studied by substituting it with a variety of heterocyles. Nitrogen containing heterocycles at C-3 position and chlorine at C-6 position augments the anti-inflammatory activity. The position C-5 on coumarin skeleton is found relatively unexplored. Hence it could be targeted to design novel C-5 substituted coumarin-derivatives as future anti-inflammatory lead molecules. However, despite all these efforts, no such coumarin compound has made its way to clinic. Therefore, further studies pertaining to detail mechanism of action and animal studies, of these analogues are required to make them suitable candidate for clinical trials.
Conflict of interest
The authors confirm that this article content has no conflicts of interest.
References
- R. Gautam and S. M. Jachak, Med. Res. Rev., 2009, 29, 767–820 CrossRef CAS PubMed.
- G. R. Burmester, E. Feist and T. Dorrner, Nat. Rev. Rheumatol., 2014, 10, 77–88 CrossRef CAS PubMed.
- R. J. Smith, Drug Discovery Today, 2005, 10, 1598–1606 CrossRef.
- A. Mantovani, P. Allavena, A. Sica and F. Balkwill, Nature, 2008, 454, 436–444 CrossRef CAS PubMed.
- D. L. Simmons, Drug Discovery Today, 2006, 11, 210–219 CrossRef CAS.
- C. A. McCulloch, G. P. Downey and H. El-Gabalawy, Nat. Rev. Drug Discovery, 2006, 5, 864–876 CrossRef CAS PubMed.
- J. Grover, V. Kumar, V. Singh, K. Bairwa, M. E. Sobhia and S. M. Jachak, Eur. J. Med. Chem., 2014, 80, 47–56 CrossRef CAS PubMed.
- K. Bairwa, I. N. Singh, S. K. Roy, J. Grover, A. Srivastava and S. M. Jachak, J. Nat. Prod., 2013, 76, 1393–1398 CrossRef CAS PubMed.
- S. M. Jachak, Curr. Med. Chem., 2006, 13, 659–678 CrossRef CAS.
- F. Borges, F. Roleira, N. Milhazes, L. Santana and E. Uriarte, Curr. Med. Chem., 2005, 12, 887–916 CrossRef CAS.
- L. Wu, X. Wang, W. Xu, F. Farzaneh and R. Xu, Curr. Med. Chem., 2009, 16, 4236–4260 CrossRef CAS.
- K. C. Fylaktakidou, D. J. Hadjipavlou-Litina, K. E. Litinas and D. N. Nicolaides, Curr. Pharm. Des., 2004, 10, 3813–3833 CrossRef CAS.
- M. Curini, G. Cravotto, F. Epifano and G. Giannone, Curr. Med. Chem., 2006, 13, 199–222 CrossRef CAS.
- Y. Bansal, P. Sethi and G. Bansal, Med. Chem. Res., 2013, 22, 3049–3060 CrossRef CAS.
- M. A. Musa, J. S. Cooperwood and M. O. F. Khan, Curr. Med. Chem., 2008, 15, 2664–2679 CrossRef CAS.
- C. Kontogiorgis, A. Detsi and D. Hadjipavlou-Litina, Expert Opin. Ther. Pat., 2012, 22, 437–454 CrossRef CAS PubMed.
- P. O. Patil, S. B. Bari, S. D. Firke, P. K. Deshmukh, S. T. Donda and D. A. Patil, Bioorg. Med. Chem., 2013, 21, 2434–2450 CrossRef CAS PubMed.
- C. S. Lino, M. L. Taveira, G. S. B. Viana and F. J. A. Matos, Phytother. Res., 1997, 11, 211–215 CrossRef CAS.
- A. M. Silvan, M. J. Abad, P. Bermejo and A. Villar, Inflammopharmacology, 1998, 6, 255–263 CrossRef CAS PubMed.
- R. Pan, X. H. Gao, Y. Li, Y. F. Xia and Y. Dai, Fundam. Clin. Pharmacol., 2010, 24, 477–490 CrossRef CAS PubMed.
- L. Menghini, F. Epifano, S. Genovese, M. C. Marcotullio, S. Sosa and A. Tubaro, Phytother. Res., 2010, 24, 1697–1699 CrossRef CAS PubMed.
- Z. E. Nazari and M. Iranshahi, Phytother. Res., 2011, 25, 315–323 CAS.
- D. Zhao, M. N. Islam, B. R. Ahn, H. A. Jung, B. W. Kim and J. S. Choi, Arch. Pharmacal Res., 2012, 35, 179–192 CrossRef CAS PubMed.
- A. Witaicenis, L. N. Seito, A. da Silveira-Chagas, L. D. de Almeida Junior, A. C. Luchini, P. Rodrigues-Orsi, S. H. Cestari and L. C. Di Stasi, Phytomedicine, 2014, 21, 240–246 CrossRef CAS PubMed.
- K. H. Kang, C. S. Kong, Y. Seo, M. M. Kim and S. K. Kim, Food Chem. Toxicol., 2009, 47, 2129–2134 CrossRef CAS PubMed.
- S. A. Jackson, S. Sahni, L. Lee, Y. Luo, T. R. Nieduzak, G. Liang, Y. Chiang, N. Collar, D. Fink and W. He, Bioorg. Med. Chem., 2005, 13, 2723–2739 CrossRef CAS PubMed.
- C. M. Lin, S. T. Huang, F. W. Lee, H. S. Kuo and M. H. Lin, Bioorg. Med. Chem., 2006, 14, 4402–4409 CrossRef CAS PubMed.
- M. Roussaki, C. A. Kontogiorgis, D. Hadjipavlou-Litina, S. Hamilakis and A. Detsi, Bioorg. Med. Chem. Lett., 2010, 20, 3889–3892 CrossRef CAS PubMed.
- W. Pu, Y. Lin, J. Zhang, F. Wang, C. Wang and G. Zhang, Bioorg. Med. Chem. Lett., 2014, 24, 5432–5434 CrossRef CAS PubMed.
- J. F. Cheng, M. Chen, D. Wallace, S. Tith, T. Arrhenius, H. Kashiwagi, Y. Ono, A. Ishikawa, H. Sato and T. Kozono, Bioorg. Med. Chem. Lett., 2004, 14, 2411–2415 CAS.
- B. G. Lake, Food Chem. Toxicol., 1999, 37, 423–453 CrossRef CAS.
- J. M. Timonen, R. M. Nieminen, O. Sareila, A. Goulas, L. J. Moilanen, M. Haukka, P. Vainiotalo, E. Moilanen and P. H. Aulaskari, Eur. J. Med. Chem., 2011, 46, 3845–3850 CrossRef CAS PubMed.
- B. Sandhya, D. Giles, V. Mathew, G. Basavarajaswamy and R. Abraham, Eur. J. Med. Chem., 2011, 46, 4696–4701 CrossRef CAS PubMed.
- M. Iranshahi, A. Jabbari, A. Orafaie, R. Mehri, S. Zeraatkar, T. Ahmadi, M. Alimardani and H. Sadeghian, Eur. J. Med. Chem., 2012, 57, 134–142 CrossRef CAS PubMed.
- M. G. Perrone, P. Vitale, P. Malerba, A. Altomare, R. Rizzi, A. Lavecchia, C. Di Giovanni, E. Novellino and A. Scilimati, ChemMedChem, 2012, 7, 629–641 CrossRef CAS PubMed.
- A. A. Emmanuel-Giota, K. C. Fylaktakidou, D. J. Hadjipavlou-Litina, K. E. Litinas and D. N. Nicolaides, J. Heterocycl. Chem., 2001, 38, 717–722 CrossRef CAS PubMed.
- S. Khode, V. Maddi, P. Aragade, M. Palkar, P. K. Ronad, S. Mamledesai, A. H. M. Thippeswamy and D. Satyanarayana, Eur. J. Med. Chem., 2009, 44, 1682–1688 CrossRef CAS PubMed.
- A. A. M. Eissa, N. A. H. Farag and G. A. H. Soliman, Bioorg. Med. Chem., 2009, 17, 5059–5070 CrossRef CAS PubMed.
- I. G. Rathish, K. Javed, S. Ahmad, S. Bano, M. S. Alam, K. K. Pillai, S. Singh and V. Bagchi, Bioorg. Med. Chem. Lett., 2009, 19, 255–258 CrossRef CAS PubMed.
- J. Grover, V. Kumar, M. E. Sobhia and S. M. Jachak, Bioorg. Med. Chem. Lett., 2014, 24, 4638–4642 CrossRef CAS PubMed.
- G. Sandeep, Y. S. Ranganath, S. Bhasker and N. Rajkumar, Asian J. Res. Chem., 2009, 2, 155–160 Search PubMed.
- D. N. Nicolaides, K. C. Fylaktakidou and K. E. Litinas, J. Heterocycl. Chem., 1996, 33, 967–971 CrossRef CAS PubMed.
- D. N. Nicolaides, K. C. Fylaktakidou, K. E. Litinas and D. Hadjipavlou-Litina, Eur. J. Med. Chem., 1998, 33, 715–724 CrossRef CAS.
- M. Akhter, N. Akhter, M. M. Alam, M. S. Zaman, R. Saha and A. Kumar, J. Enzyme Inhib. Med. Chem., 2011, 26, 767–776 CrossRef CAS PubMed.
- R. G. Kalkhambkar, G. M. Kulkarni, H. Shivkumar and R. N. Rao, Eur. J. Med. Chem., 2007, 42, 1272–1276 CrossRef CAS PubMed.
- C. K. Ramaganesh, Y. D. Bodke and K. B. Venkatesh, Indian J. Chem., Sect. B: Org. Chem. Incl. Med. Chem., 2010, 49B, 1151–1154 CAS.
- R. Aggarwal, S. Kumar, P. Kaushik, D. Kaushik and G. K. Gupta, Eur. J. Med. Chem., 2013, 62, 508–514 CrossRef CAS PubMed.
- V. Maddi, K. S. Raghu and M. N. A. Rao, J. Pharm. Sci., 1992, 81, 964–966 CrossRef CAS PubMed.
- C. A. Kontogiorgis and D. J. Hadjipavlou-Litina, Bioorg. Med. Chem. Lett., 2004, 14, 611–614 CrossRef CAS PubMed.
- C. A. Kontogiorgis and D. J. Hadjipavlou-Litina, J. Med. Chem., 2005, 48, 6400–6408 CrossRef CAS PubMed.
- I. E. Bylov, M. V. Vasylyev and Y. V. Bilokin, Eur. J. Med. Chem., 1999, 34, 997–1001 CrossRef CAS.
- G. Melagraki, A. Afantitis, O. Igglessi-Markopoulou, A. Detsi, M. Koufaki, C. Kontogiorgis and D. J. Hadjipavlou-Litina, Eur. J. Med. Chem., 2009, 44, 3020–3026 CrossRef CAS PubMed.
- K. K. Sivakumar, A. Rajasekaran, P. Senthilkumar and P. P. Wattamwar, Bioorg. Med. Chem. Lett., 2014, 24, 2940–2944 CrossRef CAS PubMed.
- M. Ghate, D. Manohar, V. Kulkarni, R. Shobha and S. Y. Kattimani, Eur. J. Med. Chem., 2003, 38, 297–302 CrossRef CAS.
- M. Ghate, R. A. Kusanur and M. V. Kulkarni, Eur. J. Med. Chem., 2005, 40, 882–887 CrossRef CAS PubMed.
- I. A. Khan, M. V. Kulkarni, M. Gopal, M. S. Shahabuddin and C. M. Sun, Bioorg. Med. Chem. Lett., 2005, 15, 3584–3587 CrossRef CAS PubMed.
- R. G. Kalkhambkar, G. Aridoss, G. M. Kulkarni, R. M. Bapset, T. Y. Mudaraddi, N. Premkumar and Y. T. Jeong, Monatsh. Chem., 2011, 142, 305–315 CrossRef CAS.
- M. Mazzei, R. Dondero, E. Sottofattori, E. Melloni and R. Minafra, Eur. J. Med. Chem., 2001, 36, 851–861 CrossRef CAS.
- D. N. Nicolaides, D. R. Gautam, K. E. Litinas, D. J. Hadjipavlou-Litina and K. C. Fylaktakidou, Eur. J. Med. Chem., 2004, 39, 323–332 CrossRef CAS PubMed.
- E. L. Grimm, C. Brideau, N. Chauret, C. C. Chan, D. Delorme, Y. Ducharme, D. Ethier, J. P. Falgueyret, R. W. Friesen and J. Guay, Bioorg. Med. Chem. Lett., 2006, 16, 2528–2531 CrossRef CAS PubMed.
- M. K. Pandey, S. Balwani, P. K. Sharma, V. S. Parmar, B. Ghosh and A. C. Watterson, Eur. J. Pharm. Sci., 2010, 39, 134–140 CrossRef CAS PubMed.
- K. Upadhyay, A. Bavishi, S. Thakrar, A. Radadiya, H. Vala, S. Parekh, D. Bhavsar, M. Savant, M. Parmar and P. Adlakha, Bioorg. Med. Chem. Lett., 2011, 21, 2547–2549 CrossRef CAS PubMed.
- K. V. Sashidhara, M. Kumar, R. K. Modukuri, R. Sonkar, G. Bhatia, A. K. Khanna, S. Rai and R. Shukla, Bioorg. Med. Chem. Lett., 2011, 21, 4480–4484 CrossRef CAS PubMed.
- T. Symeonidis, K. C. Fylaktakidou, D. J. H. Litina and K. E. Litinas, Eur. J. Med. Chem., 2009, 44, 5012–5017 CrossRef CAS PubMed.
- R. K. Arora, N. Kaur, Y. Bansal and G. Bansal, Acta Pharm. Sin. B, 2014, 4, 368–375 CrossRef PubMed.
- Z. Li, J. Hu, M. Sun, H. Ji, M. Zhao, D. Wu, G. Li, G. Liu and N. Chen, Eur. J. Pharmacol., 2011, 661, 118–123 CrossRef CAS PubMed.
- Z. Li, J. Hu, M. Sun, X. Song, G. Li, Y. Liu, G. Li, H. Ji, G. Liu and N. Chen, Int. Immunopharmacol., 2013, 17, 400–403 CrossRef CAS PubMed.
- Z. Li, J. Hu, M. Sun, H. Ji, S. Chu, G. Liu and N. Chen, Int. Immunopharmacol., 2012, 14, 145–149 CrossRef CAS PubMed.
- X. Song, J. Hu, M. Sun, Z. Li, Z. Zhu, L. Song, Y. Yuan, G. Liu and N. Chen, Brain Res. Bull., 2014, 106, 30–38 CrossRef CAS PubMed.
|
This journal is © The Royal Society of Chemistry 2015 |