DOI:
10.1039/C5RA05007C
(Paper)
RSC Adv., 2015,
5, 33786-33791
Green synthesis of a palladium–polyaniline nanocomposite for green Suzuki–Miyaura coupling reactions†
Received
21st March 2015
, Accepted 2nd April 2015
First published on 7th April 2015
Abstract
A palladium–polyaniline (Pd–PANI) nanocomposite was successfully synthesized using a one-pot green synthetic procedure in water by the reaction between aniline hydrochloride and potassium hexachloropalladate. Strong interaction between PANI and Pd was clearly evident in the UV-visible and Fourier transform infrared (FTIR) spectrum of the nanocomposite. Powder X-ray diffraction (XRD) patterns revealed the presence of Pd(0) in the nanocomposite with a fcc crystal structure. Field emission scanning electron microscopy (FE-SEM) and transmission electron microscopy (TEM) imaging showed that the Pd–PANI nanocomposite has spherical morphology with an average particle size of 175 ± 42 nm. High resolution TEM imaging and energy dispersive X-ray (EDX) spectroscopy studies revealed that very small nanoparticles of Pd (3.1 ± 0.9 nm) were found dispersed throughout the PANI matrix. X-ray photoelectron spectroscopy (XPS) revealed the presence of Pd(0) in the nanocomposite. The catalytic behavior of the Pd–PANI nanocomposite was studied for Suzuki–Miyaura coupling reactions in the presence of different bases in both organic and aqueous media. The results revealed that the Suzuki–Miyaura reaction proceeds much faster in water than in toluene. The excellent catalytic activity of the nanocomposite resulted in 86 and 91% yield in water and toluene respectively, when potassium carbonate was used as the base.
1. Introduction
A large number of scientific fields have benefitted immensely due to the advent of nanoscience and nanotechnology.1 One of the many fields where nanotechnology was immediately applied was heterogeneous catalysis. Catalysis is chiefly a surface phenomenon and reduction in particle size leads to highly enhanced surface area and this in turn results in enhanced catalytic activity. It is expected that nanocatalysts will provide a breakthrough in cutting edge technologies. The development of new catalysts, especially for different organic reactions is always demanding. Cost effectiveness, enhancement in the product yield and reduction in reaction time are the major drivers for the development of novel catalysts. Need for developing ‘green’ synthetic processes has recently emerged as an additional driver, mainly due to the increased awareness about environmental concerns and legislations.2 In this context, nanotechnology and green chemistry can come together for the development of such catalysts and this is the central theme of the present paper.
Carbon–carbon coupling reactions such as Suzuki–Miyaura, Heck, Sonogashira, Stille are very important to synthesize large number of pharmaceutical drugs, natural products, cosmetics, polymers and liquid crystals.3 Pd is the catalyst of choice and is being widely used as catalyst for Suzuki–Miyaura coupling reactions.3 A plethora of literature is available on the development of Pd catalysts for Suzuki–Miyaura coupling reactions. Heterogeneous Pd-catalysts are attractive than the traditional homogeneous Pd-catalysts. This is due to the possibility of catalyst recyclability and for avoiding toxicity imparted from residual catalysts, especially in the synthesis of drug molecules.4–6 Nano sized Pd-particles supported on a suitable support are the most effective catalysts due to increase in surface area to volume ratio, which consequently leads to better interaction of the catalyst with the reactants.7
Another open challenge in the development of catalysts for Suzuki–Miyaura coupling reactions is the need to develop catalysts that are active in water as the reaction medium.8,9 Most often Suzuki–Miyaura coupling reactions are performed in organic solvents. Recently, there is more focus on performing the Suzuki–Miyaura coupling reactions in water as a green solvent. A number of concerns such as cost, safety and health hazards that are associated with the organic solvents can be overcome by the use of water as a solvent. However, most organic compounds are water insoluble and hence performing reactions in water becomes difficult. A plethora of research is being done on developing methods for performing organic reactions in water. The published literature until 2010 on Suzuki–Miyaura coupling reactions in water have been reviewed by Polshettiwar et al.10 Evidently, most often the Suzuki–Miyaura coupling reactions in water was performed by using homogeneous catalysts. Only a limited number of heterogeneous catalysts such as Pd supported on various silica supports have been studied for Suzuki–Miyaura reactions in water.11–13 One notable common aspect of these catalysts is the presence of an organic surfactant such as cetyl trimethylammonium bromide (CTAB), polyethylene glycol (PEG) to facilitate the mixing of the catalysts with water and to prevent aggregation. Additionally, majority of these catalysts were made by tethering Pd-containing organic compounds to the silica backbone. Progressive deactivation of some of these catalysts was observed due to the decomposition of the catalyst.14 Thus, only limited attention was paid to develop Pd nanoparticles supported on a suitable support as catalysts for performing Suzuki–Miyaura reactions in water. Here we report the effective use of Pd–PANI nanocomposite as a reusable catalyst for performing Suzuki–Miyaura coupling reaction in water. Interestingly, it was also found that the same catalyst can be used in organic solvents also.
PANI is a conducting polymer having very good environment stability. PANI has been used as a catalyst support for a variety of metals, metal ions and metal oxides including Pd. There are a large number of methods reported for the synthesis of Pd or Pd based catalysts.5 However, only very few reports are there on green synthesis of Pd nanoparticles or their composites. Here we report an one-pot green synthesis of Pd–PANI nanocomposite and its application as catalyst for Suzuki–Miyaura coupling reaction. Synthesis of Pd–PANI catalyst was achieved by using aniline hydrochloride as monomer and potassium hexachloropalladate as oxidant in water as solvent. The prepared nanocomposite was used as efficient catalyst in water as well as toluene as reaction medium for Suzuki–Miyaura coupling reactions with good yield.
2. Experimental section
2.1. Materials
Aniline hydrochloride, N-methyl pyrrolidone (NMP), potassium hexachloropalladate (K2PdCl6), silica gel, bromo octane, bromo benzene, iodo benzene, potassium carbonate (K2CO3), sodium hydroxide (NaOH), triethylamine (Et3N), sodium phosphate (NaPO4), phenyl boronic acid and toluene were used as received from Sigma Aldrich. Hexane, ethyl acetate, diethyl ether and thin layer chromatography (TLC) plates were purchased from Merck. Ultra pure water (18.2 MΩ cm) from double stage water purifier (ELGA PURELAB Option-R7) was used to prepare all solutions and in coupling reactions.
2.2. Preparation of the Pd–PANI nanocomposite
Freshly prepared aqueous solution of K2PdCl6 (20 ml, 50 mM) was taken in a 50 ml beaker and was placed in an ice bath. Aqueous solution of aniline hydrochloride (20 ml, 0.1 M) was prepared separately and then added drop wise to the Pd salt solution while maintaining the temperature at 3–5 °C. The reaction mixture was kept under ice cold condition for 12 hours for the completion of reaction. The product was collected by centrifugation at 10
000 rpm for 30 min and repeatedly washed with water. The purified product was dried in oven at 50 °C for 24 h and the dried sample was used for further characterization.
2.3. Characterization techniques
The prepared Pd–PANI nanocomposite was characterized using a number of techniques. UV-visible absorption spectrum of the Pd–PANI nanocomposite in NMP was recorded using Shimadzu UV-4250 double beam spectrophotometer. FTIR spectrum of the Pd–PANI nanocomposite was recorded using Perkin Elmer FT-IR spectrometer (Spectrum Two) in the frequency range from 4000 cm−1 to 600 cm−1 with a resolution of 4 cm−1 and 8 scans. A small amount of the Pd–PANI nanocomposite was mixed with KBr powder and pelletized to record the FTIR spectrum. Blank KBr pellet was used for background correction. XRD of the Pd–PANI nanocomposite was recorded using Smart Lab X-ray diffractometer (Rigaku, Japan) using Cu Kα radiation as X-ray source (λ = 0.1542 nm, 40 mA, 45 kV) at room temperature. The sample was scanned in the 2θ range of 10 to 90° at a scanning rate of 2° min−1 and a step size 0.02°. FESEM imaging and elemental mapping of the Pd–PANI nanocomposite were carried out using FEI Quanta FEG 450. TEM imaging and EDX of the Pd–PANI nanocomposite was done using FEI Tecnai G2 200 S-Twin Model (200 kV) on carbon coated copper grids. XPS data was collected using VG Microtech, model ESCA 3000 equipped with ion gun (EX-05) for cleaning the surface. Atomic absorption spectroscopy (AAS) was carried out using LAB India AA 7000. The 1H spectra of the product of coupling reactions were recorded using JEOL ECS-400 MHz NMR system in CDCl3.
2.4. Catalytic activity
2.4.1. Suzuki–Miyaura reaction in aqueous medium. The catalytic activity of synthesized Pd–PANI nanocomposite was tested for Suzuki–Miyaura coupling reaction using water as the reaction medium (Scheme 1). The procedure for green Suzuki–Miyaura coupling reaction described in the literature was followed.15 Typically, to a 25 ml round bottom flask containing 2 ml water, 1.5 mmol phenylboronic acid, 1 mmol aryl halide, 3 mmol base and 0.01 mol% of Pd–PANI nanocomposite was added. Reaction mixture was saturated with argon gas for 15 min and sealed with Teflon rubber septum. The reaction was carried out in argon atmosphere with stirring for 4 h at 90 °C. The product was extracted in ether and pure product was collected using column chromatography.
 |
| Scheme 1 Suzuki–Miyaura coupling reaction in water. | |
2.4.2. Suzuki–Miyaura reaction in organic medium. The catalytic activity of the prepared Pd–PANI nanocomposite was tested for Suzuki–Miyaura coupling reaction in organic medium also (Scheme 2). Typically, to a 50 ml round bottom flask containing 5 ml toluene, 1 mmol of bromo octane, 1.5 mmol phenyl boronic acid, 3 mmol of a base and 0.036 mol% of the Pd–PANI nanocomposite was added. The reaction mixture was then refluxed in an oil bath under magnetic stirring at 90 °C for 9 h. The product was extracted with ethyl acetate and the pure product was collected using column chromatography. Similar procedure was followed for the Suzuki–Miyaura reaction between bromo benzene and phenylboronic acid (Reaction (2) in Scheme 2).
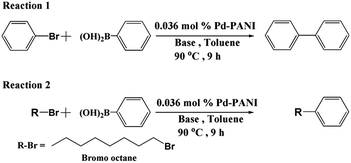 |
| Scheme 2 Suzuki–Miyaura coupling reaction in toluene. | |
2.5. Effect of base
Effect of change in the base that was used for the coupling reaction was studied systematically. Five different bases namely NaOH, K2CO3, Na2CO3, Na2PO4 and (C2H5)3N were used for all the three Suzuki–Miyaura reactions that are presented in Schemes 1 and 2 to study effect of base on the yield of the reaction.
3. Results and discussion
The reaction between K2PdCl6 and aniline hydrochloride was instantaneous, even in ice-cold conditions, as we observed change in color of the solution of the latter immediately on the addition of the former. The colorless solution of aniline hydrochloride changed initially to brown and then a green colored precipitate was formed. The color of the prepared Pd–PANI nanocomposite was green after extraction and purification. This indicates that the PANI in the nanocomposite was in its emeraldine salt form. Oxidation of aniline takes place simultaneously with the reduction of palladium ions on mixing leading to the formation of the Pd–PANI nanocomposite. The Pd salt K2PdCl6 was reported as oxidant for the synthesis of PANI using aniline monomer in organic solvents.16 The present synthesis proceeds in aqueous medium in a single step and hence is a ‘green’ procedure.
3.1. Characterization
The UV-visible absorption spectrum of the prepared Pd–PANI nanocomposite is shown in Fig. 1. The Pd–PANI nanocomposite showed three characteristic absorption peaks at wavelength of 290 nm, 425 nm and 600 nm. The peak at 290 nm is due to plasmon absorption of Pd nanoparticles.17 The two broad absorption bands that are peaking at 425 nm and 600 nm correspond to π–π* transition within benzenoid units and transition from benzenoid to quinoid of PANI respectively.18 A blue shift of the π–π* transition band (benzenoid to quinoid units) was observed in the nanocomposite when compared to pure PANI. This indicates that there is interaction between palladium nanoparticles and nitrogen atom of the PANI.16
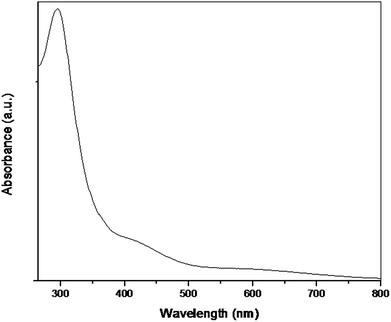 |
| Fig. 1 UV-visible absorption spectrum of Pd–PANI nanocomposite. | |
Recorded FTIR spectrum of the prepared Pd–PANI nanocomposite is shown in Fig. 2. The most important characteristic vibrational bands of Pd–PANI nanocomposite were observed at 3454 cm−1 (N–H stretching), 1571 cm−1 (quinoid ring stretching), 1492 cm−1 (benzenoid ring stretching), 1305 cm−1 (secondary C–N stretching), 1119 cm−1 (vibration band of dopant anion) and 803 cm−1 (para disubstituted benzene rings).19 Also the shifting of N
Q
N stretching (Q = quinoid unit) and N–B–N stretching (B = benzenoid unit) towards lower frequencies are due to the interaction between PANI and Pd.20
 |
| Fig. 2 FTIR spectrum of Pd–PANI nanocomposite. | |
The XRD pattern of the prepared Pd–PANI nanocomposite is shown in Fig. 3. The broad peak centered at 25° having a shoulder at 20° is due to the crystalline domains of PANI.21,22 There were a number of other distinct peaks observed in the XRD pattern corresponding to Pd in the Pd–PANI nanocomposite. Peaks at 2θ 40°, 46°, 68°, 82°and 86° are due to the (111), (200), (220), (113) and (222) reflection planes of metallic Pd with fcc lattice structure.23 These peaks also matched well with the standard values (JCPDS file no. 87-0638) and with the reported data for Pd.24
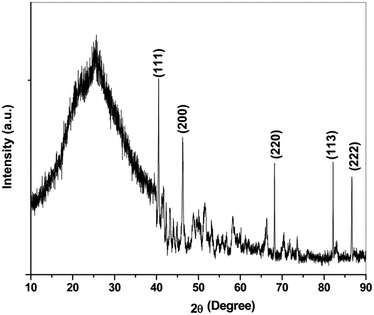 |
| Fig. 3 XRD pattern of Pd–PANI nanocomposite. | |
The morphology of the prepared Pd–PANI nanocomposite was studied using FE-SEM and TEM imaging and the corresponding images are shown in Fig. 4. It is evident from the FE-SEM image that the Pd–PANI nanocomposite has spherical morphology with average particle size of 175 ± 42 nm. The same morphology and particle size was confirmed from TEM images also. Surface of the Pd–PANI nanocomposite appeared rough in the FESEM images. It shows that the Pd nanoparticles are present on the surface of the nanocomposite. A high resolution TEM image is shown in Fig. 5. From Fig. 5, it is clear that Pd is present in the form of small spherical nanoparticles in the PANI matrix. The particle size of the Pd nanoparticles was 3.0 ± 0.9 nm.
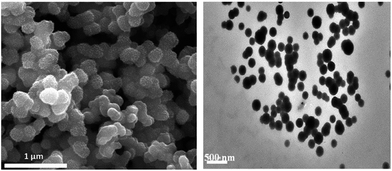 |
| Fig. 4 FESEM and TEM image of Pd–PANI nanocomposite. | |
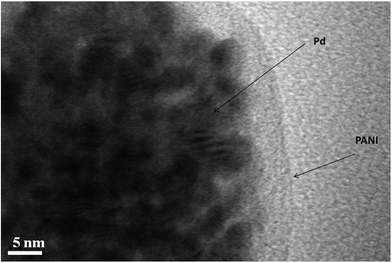 |
| Fig. 5 High resolution TEM image of the Pd–PANI nanocomposite. | |
The elemental mapping of Pd and N was done using energy dispersive X-ray (EDX) spectroscope attached to the FESEM and the corresponding elemental map is presented in Fig. 6. From the elemental map of Pd as shown in Fig. 6b and c it is evident that Pd nanoparticles are present throughout the spherical particles of Pd–PANI nanocomposite. From a comparison of the elemental map of Pd and N, it can be seen that the Pd nanoparticles predominates the surface as the concentration of Pd was higher than N.
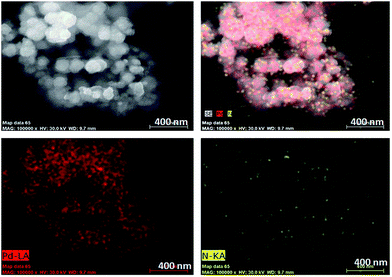 |
| Fig. 6 (a) FESEM image of Pd–PANI nanocomposite. Elemental map of: (b) Pd and N together, (c) Pd alone and (d) N alone. | |
EDX spectroscopic analysis of the sample deposited on TEM grid was also done and a typical spectrum is shown in Fig. 7. The Pd element is clearly seen in EDX spectrum. Presence of Cl was also detected in EDX. This is due to the emeraldine salt form of PANI. AAS studies revealed that the nanocomposite contained 9% (by wt) of Pd.
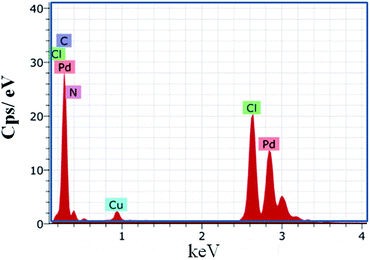 |
| Fig. 7 EDX spectrum of the Pd–PANI nanocomposite. | |
Pd–PANI nanocomposite was further characterized by XPS as shown in Fig. 8 and S1 ESI.† The metallic state of Pd(0) in nanocomposite was confirmed by the XPS peaks at 339.4 eV (Pd(0) 3d5/2) and 344.7 eV (Pd(0) 3d3/2) respectively. Evidently, there was significant shift in the binding energy values and this must be due to interaction of Pd(0) with PANI.25–27 Peak at binding energies of 200.3 eV, 285.2 eV, 401.5 eV, 535.7 eV corresponds to Cl 2p, C 1s, N 1s and O 1s respectively in the core level XPS spectrum (Fig. S1, ESI†). The core level spectrum also matched with the reported data.28
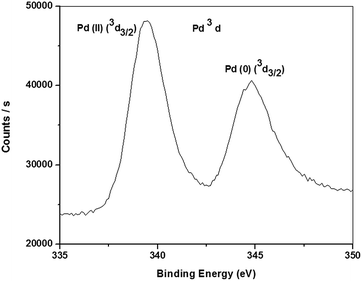 |
| Fig. 8 XPS spectrum of the Pd core level. | |
3.2. Catalytic activity
The catalytic activity of the Pd–PANI nanocomposite was studied in both the aqueous and organic reaction medium and the results are discussed in the following section.
3.2.1. Suzuki–Miyaura coupling reaction. The Pd–PANI nanocomposite showed very good catalytic activity for Suzuki–Miyaura reaction in water and the reaction was almost complete in 4 h. Water and diethyl ether was added to the reaction mixture after completion of the reaction. The oil and aqueous phases were separated. To the oil phase excess amount of sodium sulphate was added and diethyl ether was evaporated on rotary evaporator under reduced pressure. Column chromatography of crude product was done on silica gel column using hexane and ethyl acetate mixture (95
:
5) to collect the pure product and yield was calculated. The formation of the product was confirmed by using NMR spectroscopy and the typical 1H NMR spectrum is reported in Fig. S2 ESI.† The 1H NMR data is summarized below.
Biphenyl. 1H NMR (400 MHz, CDCl3) δ 7.60–7.57 (m, 4H), δ 7.45–7.41 (m, 4H), δ 7.36–7.32 (m, 2H).The Pd–PANI nanocomposite showed good catalytic activity in Suzuki–Miyaura reactions in toluene medium also. The reaction was relatively slow in toluene compared to water. In water, the coupling reaction between bromobenzene and phenyl boronic acid completed in 4 h, whereas in toluene it took 9 h to complete the same reaction. The coupling reaction between octylbromide and phenylbornic acid also proceeded with good yield in toluene. The formation of the products (biphenyl and octyl benzene) was confirmed by using NMR spectroscopy and the typical 1H NMR spectrum is reported in Fig. S2 and S3 ESI† and the results are summarized below.
Octyl benzene. 1H NMR (500 MHz, CDCl3) δ 7.60–7.58 (m, 2H), δ 7.45–7.42 (m, 2H), δ 7.36–7.33 (m, 1H), δ 3.40 (t, J = 6.9 Hz, 2H), δ 1.88–1.82 (m, 2H), δ 1.43–1.40 (m, 2H), δ 1.30–1.25 (m, 8H), 0.88 (t, J = 7.0 Hz, 3H).The Suzuki–Miyaura reaction is usually performed in presence of a base and it is known that the nature of the base affects the completion of reaction.29 Hence, the effect of changing the base on the yield of the product was studied using five different bases. The yield of the reaction with each base in different Suzuki–Miyaura reaction in both water and toluene is presented in Table 1.
Table 1 Study of effect of base on the yield of the Suzuki–Miyaura coupling reaction in water and toluene as reaction medium
Scheme |
% Yield of reaction |
NaOH |
K2CO3 |
Na2CO3 |
Na2PO4 |
(C2H5)3N |
1 |
84 |
86 |
80 |
77 |
73 |
2 (R1) |
82 |
91 |
76 |
74 |
58 |
2 (R2) |
85 |
88 |
79 |
63 |
61 |
It can be seen from the table that the yield of the reaction varies significantly with the change in base. Yield for the Suzuki–Miyaura reaction in aqueous medium varied from 86% to 73% with the change in base. In the organic medium the yield changed from 91% to 58% and 88% to 61% respectively in Reaction (1) and Reaction (2). Higher yield was obtained when the base used was K2CO3, irrespective of the reaction medium and reactants. So, it was concluded that K2CO3 is the best base among all for the prepared Pd–PANI catalyst in the Suzuki–Miyaura coupling reactions that were studied. Rafique et al., also reported K2CO3 as the best base for Suzuki–Miyaura reaction with Pd–PANI as catalyst.29 However, NaOH and Na2CO3 also gave good yields. Easy separation and reusability of the catalyst is the major attraction for heterogeneous catalysts such as Pd–PANI. Recyclability of the catalysts was not tested due to the small scale of reaction with very low concentration of catalysts.
4. Conclusions
In summary, a simple single step and green method for the synthesis of Pd–PANI nanocomposite has been developed. The nanocomposite has a spherical morphology and contains very small Pd nanoparticles dispersed in the PANI matrix. Strong interaction between the Pd nanoparticles and the PANI was clearly evident. The prepared Pd–PANI nanocomposite showed very good catalytic activity for Suzuki–Miyaura coupling reactions in water as well as in toluene reaction media. The base that is used for the coupling reaction affects the yield of the reaction. The yield of the coupling reaction using Pd–PANI nanocomposite was better with K2CO3 as the base. Most importantly, the Pd–PANI nanocomposite showed very good catalytic activity for Suzuki–Miyaura coupling reaction in water. Thus, the nanocomposite can be used to perform green Suzuki–Miyaura coupling reactions. The potential of this nanocomposite can be exploited in many other Pd catalyzed reactions also.
Acknowledgements
We are thankful to AMRC, IIT Mandi for laboratory and the characterization facilities. Financial support from DST, New Delhi, India is also acknowledged hereby. We are also thankful to CMSE, NIT Hamirpur (H.P.), India for providing FE-SEM imaging facilities.
Notes and references
- B. Karn and O. Sadik, ACS Sustainable Chem. Eng., 2013, 1, 685 CrossRef CAS.
- J. Clark, Green Chem., 1999, 1, 1–8 RSC.
- X. F. Wu, P. Anbarasan, H. Neumann and M. Beller, Angew. Chem., Int. Ed., 2010, 49, 9047–9050 CrossRef CAS PubMed.
- L. Liu, Y. Dong and N. Tang, Green Chem., 2014, 16, 2185–2189 RSC.
- A. Molnar, Chem. Rev., 2011, 111, 2251–2320 CrossRef CAS PubMed.
- D. Astruc, Inorg. Chem., 2007, 46, 1884–1894 CrossRef CAS PubMed.
- Y. Li, X. M. Hong, D. M. Collard and M. A. El-Sayed, Org. Lett., 2000, 2, 2385–2388 CrossRef CAS PubMed.
- M.-O. Simon and C.-J. Li, Chem. Soc. Rev., 2012, 41, 1415–1427 RSC.
- P. Anastas and N. Eghbali, Chem. Soc. Rev., 2010, 39, 301–312 RSC.
- V. Polshettiwar, A. Decottignies, C. Len and A. Fihri, ChemSusChem, 2010, 3, 502–522 CrossRef CAS PubMed.
- H. Kosslick, I. Mönnich, E. Paetzold, H. Fuhrmann, R. Fricke, D. Muller and G. Oehme, Microporous Mesoporous Mater., 2001, 44, 537–545 CrossRef.
- C. Baleizão, A. Corma, H. García and A. Leyva, Chem. Commun., 2003, 606–607 RSC.
- C. M. Crudden, M. Sateesh and R. Lewis, J. Am. Chem. Soc., 2005, 127, 10045–10050 CrossRef CAS PubMed.
- A. Corma, D. Das, H. García and A. Leyva, J. Catal., 2005, 229, 322–331 CrossRef CAS PubMed.
- J. Yang, D. Wang, W. Liu, X. Zhang, F. Bian and W. Yu, Green Chem., 2013, 15, 3429–3437 RSC.
- R. Gangopadhyay and A. De, Chem. Mater., 2000, 12, 608–622 CrossRef CAS.
- K. Mallick, M. Witcomb and M. Scurrell, Platinum Met. Rev., 2007, 51, 3–15 CrossRef CAS.
- X. Zhang, W. J. Goux and S. K. Manohar, J. Am. Chem. Soc., 2004, 126, 4502–4503 CrossRef CAS PubMed.
- A. A. Athawale, M. V. Kulkarni and V. V. Chabukswar, Mater. Chem. Phys., 2002, 73, 106–110 CrossRef CAS.
- A. Drelinkiewicz, M. Hasik and M. Choczyński, Mater. Res. Bull., 1998, 33, 739–762 CrossRef CAS.
- S. Dutt and P. F. Siril, Mater. Lett., 2014, 124, 50–53 CrossRef CAS PubMed.
- S. Dutt and P. F. Siril, J. Appl. Polym. Sci., 2014, 131, 40800–40808 CrossRef PubMed.
- S. Navaladian, B. Viswanathan, T. Varadarajan and R. Viswanath, Nanoscale Res. Lett., 2009, 4, 181–186 CrossRef CAS PubMed.
- A. Zhang, M. Liu, M. Liu, Y. Xiao, Z. Li, J. Chen, Y. Sun, J. Zhao, S. Fang and D. Jia, J. Mater. Chem. A, 2014, 2, 1369–1374 CAS.
- L. Gao, W. Yue, S. Tao and L. Fan, Langmuir, 2013, 29, 957–964 CrossRef CAS PubMed.
- R. K. Pandey and V. Lakshminarayanan, J. Phys. Chem. C, 2009, 113, 21596–21603 CAS.
- W. J. Stec, W. E. Morgan, R. G. Albridge and J. R. V. Wazer, Inorg. Chem., 1972, 11(2), 219–225 CrossRef CAS.
- S. Dutt, P. F. Siril, V. Sharma and S. Periasamy, New J. Chem., 2015, 39, 902–908 RSC.
- R. U. Islam, M. J. Witcomb, E. van der Lingen, M. S. Scurrell, W. Van Otterlo and K. Mallick, J. Organomet. Chem., 2011, 696, 2206–2210 CrossRef PubMed.
Footnote |
† Electronic supplementary information (ESI) available: Fig. S1, overall XPS spectrum of the Pd–PANI nanocomposite, Fig. S2, NMR spectrum of biphenyl and Fig. S3, NMR spectrum of octyl benzene. See DOI: 10.1039/c5ra05007c |
|
This journal is © The Royal Society of Chemistry 2015 |