DOI:
10.1039/C5RA04696C
(Paper)
RSC Adv., 2015,
5, 51413-51420
Heavier group 2 metal complexes with a flexible scorpionate ligand based on 2-mercaptopyridine†
Received
17th March 2015
, Accepted 3rd June 2015
First published on 3rd June 2015
Abstract
We report the synthesis of novel alkaline earth metal complexes [κ2-SS-(Bmp)2M(THF)n] [M = Ca (2), Sr (3) n = 2; M = Ba (4), n = 3] of a flexible dihydrobis(2-thiopyridone)borate (Bmp) ligand based on 2-mercaptopyridine. Complexes 2–4 were isolated in good yield by the reaction between sodium dihydrobis(2-thiopyridone)borate, [{(Bmp)Na(THF)}2]n (1) and the corresponding alkaline earth metal diiodides in toluene at ambient temperature. The solid-state structures of the strontium and barium complexes, complexes 3 and 4 respectively, were established using single-crystal X-ray diffraction analysis. The solid-state structure of sodium complex 1 was also confirmed using X-ray techniques. The solid-state structures of complexes 3 and 4 revealed that the Bmp ligand coordinates through sulphur atoms to the metal ions in κ2 fashion. The strontium ion is attached symmetrically and the barium ion is asymmetrically linked with the Bmp ligand, manifesting the 2-thiopyridone and pyridine-2-thiolate tautomeric form of the Bmp ligand. The strontium ion in complex 3 adopts a distorted octahedral geometry whereas the geometry around the barium ion can best be described as a distorted pentagonal bipyramidal. Both complexes 3 and 4 also have a short B⋯H⋯M interaction due to the presence of the BH2 group in the ligand. In the solid state, sodium complex 1 is polymeric in nature and in the asymmetric unit each sodium ion is bonded to two sulphur atoms through η1 and μ2 modes. The adjacent BH2 group is also linked with each sodium ion through hydrogen atoms via μ2 and η1.
Introduction
The scorpionate ligands such as [hydrotris(pyrazolyl)borate]1 and [dihydrobis(pyrazolyl)borate]2 first reported by Trofimenko were generally thought of as innocent spectator ligands in transition metal chemistry,3,4 modifying the properties of the metal centre without getting directly involved in its reactivity.5 However, the more flexible scorpionate ligand, [hydrotris(methylimidazolyl)-borate], (Tm) and [dihydrobis(methylimidazolyl)-borate] (Bm) had two major advantages when compared to original Trofimenko's scorpionate ligands. The Tm and Bm ligands were based on soft sulfur donor atoms and more significantly, incorporate greater flexibility into the ligand by addition of an extra atom between the boron and the donor atom. The enhanced flexibility of these ligand systems provide the greater potential for activation at the boron bridgehead and formation of metal-borane (metallaboratrane) complexes6 giving rise to reactivity not observed in the analogous Tp compounds.7 Recently, Owen and co-workers have developed a new family of scorpionate ligands based on boro-mercaptopyridine hydrotris(2-thiopyridone)borate (Tmp) and hydrobis(2-thiopyridone)borate (Bmp) to study their properties of coordination to transition metals such as copper, ruthenium, iridium, palladium and platinum.8,9 However, their work has been restricted within the transition metal chemistry. It has been observed that boro-mercaptopyridine based scorpionate ligands coordinate through sulphur atoms and hydrogen atoms present in BH or BH2 groups which point towards the metal centre.10 Some of these B–H⋯metal interactions have been found to be particularly strong and exhibit significant metal-hydride character and thus these complexes can be used for hydride migration between transition metal and boron centres and this process can be applied to metal-mediated transformations.11 However, various factors, such as a transition metal's ability to accept a hydride, the nature of ancillary ligands attached to it and favourable substituents at the metal centre govern the hydride migration between boron and metal centre.12
Another interesting feature of such ligands is that the boro-mercaptopyridine unit can undergo tautomerisation between 2-thiopyridone and pyridine-2-thiolate forms where the electron density moves from the boron atom to the sulphur atom (Scheme 1). It has been reported that although the thione–thiolate tautomeric equilibrium generally favours the thione form, there is a strong dependence on its environment with only a small difference in the energy between the two tautomeric forms.13
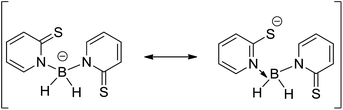 |
| Scheme 1 Tautomeric forms of 2-thiopyridone and pyridine-2-thiolate of Bmp. | |
In our continuous study of the alkaline earth metal chemistry, our work focuses on the exploration of synthetic methodologies allowing for a facile, clean, high-yield production of the required molecules and determination of their molecular structure and function. We have recently introduced various amidophosphine chalcogenide and borane ligands containing N, E (E = O, S, Se, BH3) and P as donor atoms into alkaline earth metal chemistry in order to study their coordination properties.14 These unique ligands are potentially capable of coordinating through hard nitrogen and phosphorus donor atoms along with the soft E donor atom. As the Bmp ligand has some resemblance to amidophosphine chalcogenide ligands in terms of hard and soft donor atoms (N, P, S), we have sought to introduce the Bmp ligand into electropositive alkaline earth metal chemistry in order to understand its thermodynamic stability.
Here, we report the syntheses and structural details of 2-mercaptopyridine based scorpionate calcium, strontium and barium complexes of compositions [κ2-{(2-SC5H4N)2(BH2)}2M(THF)n] [M = Ca (2), Sr (3) n = 2; M = Ba (4), n = 3]. We also report the solid-state structure of the sodium complex [{(2-S-C5H4N)2(BH2)Na(THF)}2]n (1). In addition, we also describe the comparative study among the computed structures and experimental structures of strontium and barium complexes.
Results and discussion
Sodium complex
The Owen group has reported on the sodium salt of the Bmp ligand; however, its solid-state structure has not been established.8a We found this very useful to report the solid-state structure of NaBmp and compare with the solid-state structures of alkaline earth metal complexes. The sodium complex (1) was prepared according to the procedure8a described by Owen et al. involving the reaction of NaBH4 and two equivalents of 2-mercaptopyridine in a mixture of toluene and THF at 80 °C for 12 hours (Scheme 2). The 1H, 13C{1H} and 11B{1H} NMR data for complex 1 are in full agreement with the values in literature. Complex 1 was re-crystallised from concentrated THF solution at 15 °C and the solid-state structure was confirmed using single-crystal X-ray structure analysis.
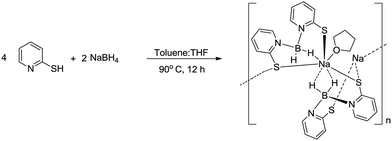 |
| Scheme 2 Synthesis of sodium complex 1. | |
Complex 1 crystallises in the triclinic space group P
, with two molecules in the unit cell. The data collection parameters are set out in Table 1. Fig. 1 shows the molecular structure of complex 1, as well as the grown-up structure. The solid-state structure of sodium complex 1 confirmed the κ2 SS-coordination of the Bmp ligand.
Table 1 Crystallographic data and structure refinement parameters for complexes 1, 3 and 4
Crystal |
1 |
3 |
4 |
CCDC no. |
1049442 |
1049443 |
1049444 |
Empirical formula |
(C28H36B2N4Na2O2S4)n |
C28H36B2N4O2S4Sr |
C32H44B2BaN4O3S4 |
Formula weight |
656.45 |
698.09 |
819.90 |
T (K) |
150(2) |
150(2) |
150(2) |
λ (Å) |
1.54184 |
0.71073 |
0.71073 |
Crystal system |
Triclinic |
Monoclinic |
Monoclinic |
Space group |
P![[1 with combining macron]](https://www.rsc.org/images/entities/char_0031_0304.gif) |
P21/c |
P21/c |
a (Å) |
7.4416(4) |
11.0091(12) |
13.7544(7) |
b (Å) |
11.0214(7) |
11.7200(9) |
17.7876(6) |
c (Å) |
20.0676(13) |
14.443(4) |
20.3426(6) |
α (°) |
83.276(5) |
90 |
90 |
β (°) |
86.959(5) |
119.400(16) |
132.543(2) |
γ (°) |
86.536(5) |
90 |
90 |
V (Å3) |
1629.82(17) |
1623.5(5) |
3666.9(2) |
Z |
2 |
2 |
4 |
Dcalc Mg m−3 |
1.342 |
1.428 |
1.487 |
μ (mm−1) |
3.201 |
1.950 |
1.349 |
F (000) |
692 |
720 |
1676 |
Theta range for data collection |
4.04 to 70.85 |
3.24 to 29.00 |
2.95 to 29.02 |
Limiting indices |
−8 ≤ h ≤ 8, −9 ≤ k ≤ 13, −24 ≤ l ≤ 20 |
−9 ≤ h ≤ 14, −13 ≤ k ≤ 14, −19 ≤ l ≤ 9 |
−18 ≤ h ≤ 12, −22 ≤ k ≤ 8, −18 ≤ l ≤ 27 |
Reflections collected/unique |
11 474/6129 [R(int) = 0.0264] |
7191/3703 [R(int) = 0.0217] |
13 294/7867 [R(int) = 0.0317] |
Completeness to theta |
97.5% |
86.1% |
95.6% |
Absorption correction |
Semi-empirical |
Semi-empirical |
Semi-empirical |
Max. and min. transmission |
0.906 and 0.886 |
1.00000 and 0.91344 |
1.00000 and 0.84776 |
Refinement method |
Full-matrix least-squares on F2 |
Full-matrix least-squares on F2 |
Full-matrix least-squares on F2 |
Data/restraints/parameters |
1.00000 and 0.79802 |
3703/0/187 |
7159/30/416 |
Goodness-of-fit on F2 |
1.090 |
1.040 |
1.038 |
Final R indices [I > 2σ(I)] |
R1 = 0.0738, wR2 = 0.2283 |
R1 = 0.0374, wR2 = 0.0784 |
R1 = 0.0365, wR2 = 0.0596 |
R indices (all data) |
R1 = 0.0785, wR2 = 0.2355 |
R1 = 0.0494, wR2 = 0.0841 |
R1 = 0.0459, wR2 = 0.0691 |
Largest diff. peak and hole |
1.565 and −0.466 e A−3 |
0.566 and −0.594 e A−3 |
1.03 and −1.15 e A−3 |
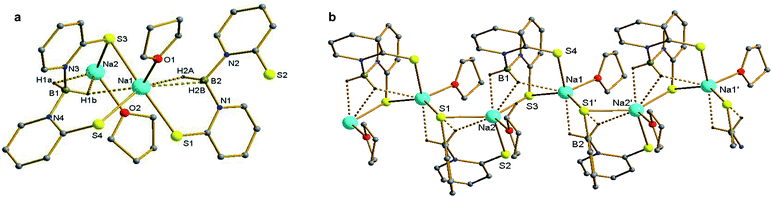 |
| Fig. 1 (a) Solid-state molecular structure of complex 1 (b) fragment of one-dimensional of polymer of complex 1. Hydrogen atoms (except H1A, H1B, H2A and H2B) are omitted for clarity. Colour code: cyan Na, red O, blue N, grey C and black H. | |
In the molecular structure of 1, it is evident that the complex is polymeric and has two different chemical environments for two sodium ions in the asymmetric unit of the molecule. The central sodium ions Na1 and Na2 are ligated from the Bmp ligand through the soft donor sulphur atoms S1, S3 in a κ2 fashion. Moreover, Na1 and Na2 are bonded to third sulphur atom S4 and S2 respectively (Fig. 1b). The other sodium ion Na2 is also bonded with three sulphur atoms (S1, S2 and S3) from another Bmp fragment in the κ2 mode. However, both the sodium atoms (Na1 and Na2) are connected through a sulphur atom (S1) from the second Bmp ligand through a μ bridging mode, with a distance of 3.953 Å between the sodium atoms. The Na–S bond distances [2.882(14) and 2.923(14) Å] are similar, which demonstrates the 2-thiopyridone of the ligand. However, the Na1–S1 and Na2–S3 distances [2.815(1) and 2.789(2) Å] indicate a strong attachment between the sodium ions and adjacent Bmp ligands with a symmetry element [x + 1, y, z] to grow the polymeric chain. Two eight-membered metallocycles Na1–S3–C14–N3–B1–N4–C16–S4 and Na2–S1–C1–N1–B2–N2–C6–S2 are observed due to the coordination of the sulphur atoms of the Bmp ligand to the sodium ions. It is noteworthy that the sulphur atoms are coplanar with their pyridine rings (S1 is coplanar with C1–C2–C3–C5–C6–N1 and S2 is coplanar with C6–C7–C8–C9–C10–N2) and the two planes are almost orthogonal (the dihedral angle is 80.9°), indicating a significant contribution from the 2-thiopyridone tautomeric form (Scheme 1). In addition, each sodium atom is coordinated with one THF molecule and the geometry around the sodium ions can be best described as a distorted tetrahedral. Both the BH2 groups from the Bmp ligands are bonded with the sodium ions through hydrogen atoms via μ (H1B and H2B) and η1 (H1A and H2A) bonds. The short Na⋯H–B distances (Na1–H1B 2.488 Å, Na2–H1B 2.544 Å and Na2–H2B 2.503 Å) are in agreement with the linkage between BH2 and sodium ions.15 The second sodium atom and one sulphur atom of the Bmp fragment leads the polymeric chain. In the NMR spectra of 1, only one set of signals in 1H, 31C{1H} and 11B{1H} was obtained due to the fluxional nature of the molecule in the solution.
Alkaline earth metal complexes
Determining the structure and reactivity of alkaline earth metal species is an important step in the design and development of efficient catalysts; however, full realisation of the catalytic potential of these elements requires substantial advances in understanding their basic coordination and organometallic chemistry.16 The heavier alkaline earth metal complexes 2–4 were readily prepared in high yield by the reaction between the sodium salt (1) and corresponding alkaline earth metal diiodides in THF solvent at ambient temperature (Scheme 3). The pure complexes can be obtained from re-crystallisation of the crude compound from a concentrated solution of THF. All the complexes are air- and moisture-sensitive and sparingly soluble in aromatic solvents such as benzene and toluene. All three compounds were characterised using the spectroscopic/analytic technique and the solid-state structure of complexes 3 and 4 were established through single-crystal X-ray diffraction analysis.
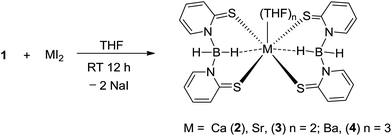 |
| Scheme 3 Synthesis of alkaline earth metal complexes 2–4. | |
The 1H NMR spectra of complexes 2–4 measured in DMSO-d6 was particularly broad, revealing four signals (from δ 8.56 to 6.44 ppm for 2, 8.57 to 6.43 ppm for 3 and 8.77 to 6.43 ppm for 4), each with an integration of three protons corresponding to the pyridine ring protons. A very broad signal for each complex, centred at δ 3.4 ppm (for 2), 3.59 ppm (for 3) and 3.52 ppm (for 4), with integration of two protons, can be assigned to the two B–H protons present in the Bmp ligand. The broad resonances (δ 3.58 and 1.74 ppm for 2, 3.58 and 1.1.74 ppm for 3 and 3.59 and 1.71 ppm for 4) are due to the THF protons, which are coordinated to the metal ions. From the integration it can be calculated that there are two coordinated THF molecules in the calcium and strontium complexes, whereas three THF molecules are attached to the barium ion in complex 4. In the 13C{1H}NMR spectrum, each of the complexes 2–4 displayed five characteristic signals in the low-field region of the spectra. The chemical shift corresponding to the C
S group was found at δ 182.5 (for 2), 182.4 (for 3) and 182.9 (for 4) ppm. Similar chemical shift values were obtained for the complex [Rh{κ3-(Bmp)2}(η3-C8H13)] (179.9 ppm) reported by Owen et al.9a The infrared spectra of complexes 2–4 showed a characteristic absorption band at 2354 (for 2), 2387 (for 3) and 2375 (for 4) cm−1 for the terminal B–H stretch, which is consistent with the reading of 2422 cm−1 reported for [Rh{κ3-(Bmp)2}(η3-C8H13)].9a In the 11B{1H} NMR experiment for each of the complexes 2–4, a broad resonance signal at δ 1.11 (for 2), 0.82 (for 3) and 1.73 (for 4) ppm is observed.
Although there has been continued interest in alkaline earth organo metallics,17a particularly in the cyclopentadienyl chemistry of these elements,17b complexes 2–4 represent, to the best of our knowledge, the first structurally characterised alkaline earth metal complexes of the Bmp ligand. Therefore, their molecular structures in the solid state were determined using X-ray diffraction analysis. X-ray quality single crystals were obtained from the concentrated THF solution of each of the complexes. Complex 2 was found to diffract very weakly under X-ray and data are therefore not publishable. The molecular structures of the strontium and barium complexes are established. Both the complexes 3 and 4 crystallise in the monoclinic space group P21/c, with two and four molecules respectively in the respective unit cell. The data collection parameters are set out in Table 1. Fig. 2 and 3 display the molecular structure of strontium complex 3 and barium complex 4 respectively. The solid-state structures of 3 and 4 confirmed a κ2-SS coordination mode for Bmp. In complexes 3, Sr1 ion is bonded with four sulphur atoms, S1, S2, S1′ (−x + 1, −y, −z) and S2′ (−x + 1, −y, −z) from two Bmp ligands. Ba1 is connected to four crystallographically independent sulphur atoms (Sa, S2, S3 and S4) in complex 4. In addition, in complex 3, the strontium ion is coordinated to two THF molecules to adopt a distorted octahedral geometry. In contrast, the barium ion in complex 4 is ligated with three THF molecules to adopt a distorted pentagonal bipyramidal geometry around it. The symmetric Sr–S bond distances [3.0171(8) and 2.9909(10) Å] in complex 3 are in agreement with reported Sr–S bond lengths [2.951(2) Å] in [Sr{S(2,4,6-tBu3C6H2)}2(THF)4]18 and [3.025(3) Å] in [Sr3(OCSOC2H5)6(C2H5OH)8],19 indicating a major contribution from the 2-thiopyridone form of the Bmp ligands. In the barium complex, the Ba–S distances are not symmetric and a combination of short [Ba1–S1 3.255(1) and Ba1–S4 3.273(1) Å] and long [Ba1–S2 3.346(1) and Ba1–S3 3.449(4) Å] is observed. These sequences of short and long bond distances are in agreement with the Ba–S covalent and coordination bond respectively.20,21 Thus, it is evident that in complex 4, pyridine-2-thiolate tautomeric form of Bmp is the major contributor (Scheme 1). The variation in ion radii of strontium and barium presumably causes one particular tautomeric form of the 2-mercaptopyridine based scorpionate Bmp ligand. Similar observations were reported by Owen et al.9 The larger Ba–S distances, when compared to Sr–S distances, are due to the larger ion radius of the barium ion (1.35 Å) when compared to the strontium ion (1.12 Å).22 In complex 3, two eight-membered metallocycles, Sr1–S1–C1–N1–B1–N2–C10–S2 and Sr1′–S1′–C1′–N1′–B1′–N2′–C10′–S2′, (Ba1–S1–C5–N1–B1–N2–C10–S2 and Ba2–S3–C11–N3–B2–N4–C20–S4 for complex 4), with a twist-boat configuration are formed by the κ2 coordination of each Bmp ligand to the strontium ion. Both the sulphur atoms (S1 and S2) are coplanar with the respective pyridine planes (C1–C20–C3–C4–C5–N1 and C6–C7–C8–C9–C10–N2) and they bisect each other through B1–N1 and B1–N2 bonds to make a dihedral angle of 76.44°. However, the four sulphur atoms in complex 4 are not coplanar with the pyridine planes and lie slightly above or below the planes (S1 0.143 Å above, S2 0.105 Å below, S3 0.137 Å below and S4 0.170 Å below), indicating the formation of thiolate for the Bmp ligands. Two sets of B–N distances [1.56(2) and 1.60(2) Å] in the Bmp ligands in complex 4 are observed, also indicating the asymmetric nature of the ligand backbone. However, only one kind of B–N distance [1.575(3)] is observed to support symmetric nature of the Bmp ligand backbone present in complex 3. Two short Sr⋯H–B contacts (2.806 Å) are observed due to the secondary interaction between the strontium ion and BH2 hydrogen atoms. Analogous short Ba⋯H–B contacts (2.706 and 2.728 Å) are obtained due to the secondary interaction between the barium ion and one hydrogen atom from the BH2 group. Such alkaline earth metal and hydrogen interactions are observed in complex [M{N(2,4,6-Me3C6H2)–SiMe3}2(PMTDA)] (M = Sr, Ba and PMDTA = N,N,N′,N′′,N′′ pentamethyldiethylenetriamine) and reported in literature.23
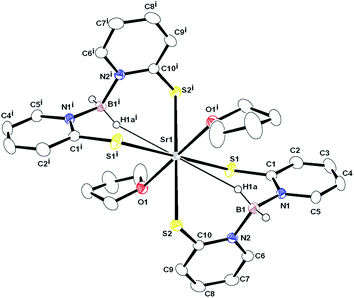 |
| Fig. 2 Solid-state structure of complex 3 and ellipsoids are drawn to encompass 50% probability. Hydrogen atoms (except H1a, H1b, H1ai and H1bi) are omitted for clarity. Selected bond lengths [Å] and bond angles [°]: Sr1–S1 3.0171(8), Sr1–S2 2.991(1), Sr1–O1 2.552(2), Sr1–O1′ 2.552(2), Sr1–S1′ 3.0171(8), Sr1–S2′ 2.991(1). More bond lengths and angles are given in ESI.† | |
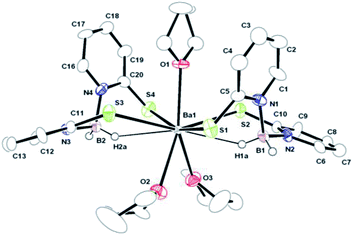 |
| Fig. 3 Solid-state structure of complex 4 and ellipsoids are drawn to encompass 50% probability. Hydrogen atoms are omitted for clarity (except H1b, H1a, H2a and H2b). Selected bond lengths [Å] and bond angles [°]: Ba1–S1 3.255(2), Ba1–S2 3.346(1), Ba1–S3 3.449(1), Ba1–S4 3.273(2), Ba1–O1 2.711(2), Ba1–O2 2.794(4), Ba1–O3 2.797(3). More bond lengths and angles are given in ESI.† | |
Computational analysis
First principles calculations were performed to investigate the structure and coordination properties of various metal complexes described in the Experimental section of the current study. We were also interested in modelling the elusive calcium complex whose crystals weakly diffracted in X-ray. In addition, we wanted more insight about the structures and bonding of the sodium, strontium and barium complexes by optimising their structure computationally. The chemical nature of bonds between metal and ligands, as well as solvent, was investigated to understand the coordinating behaviour of metal ions. All the model structures were drawn in GaussView24 and optimised at B3LYP/LANL2DZ level25 of electronic structure calculation using the Gaussian 09 (Rev B.01) software.26 The chosen method, combining hybrid density functional and potential for the metals studied here, has been used in earlier studies involving alkaline earth metal complexation.27 We computed the structures, shown in Fig. 4, by following the geometry optimisation procedure. The coordinating environments of metal ions are very similar to the experimentally obtained complex structures for ions other than Ca.
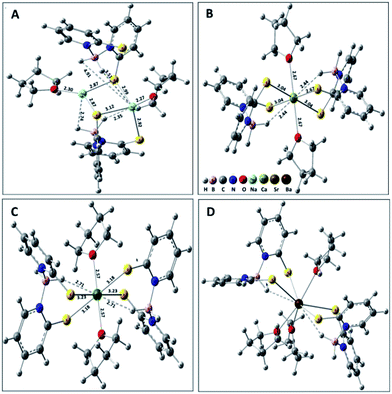 |
| Fig. 4 Computed structures of complexes are shown in A, B, C and D corresponding to Na, Ca, Sr and Ba, respectively. The coordinating bonds around the metals ions are shown in Å unit. | |
A comparison of computed bonds and angles and available experimental data is given in the ESI.† The distances and angles are close to the crystal structure data obtained using X-ray diffraction analysis. For example, calculated Na–S and Na–O distances are 2.99 and 2.27 Å, whereas the experimental values are 2.892 and 2.329 Å, respectively. Table 2 represents the comparison of calculated and experimentally obtained values corresponding to significant coordinating distances around the ions. The symmetric Sr–S bond distances are slightly higher than the experimental values. As observed from earlier discussions, the calculated Ba–S distances in barium complexes are not symmetric and the differences are within 0.2 Å as compared to measured ones. The geometry around calcium shows behaviour similar to that of strontium due to similar ionic radii between the two metal ions. However the Ca–S (3.04–3.10 Å) and Ca–O (2.44 Å) distances fall in the range similar to those [2.818(3) Å and 2.495 Å] obtained for [{2,4,6-(tBu)3C6H2S}Ca(THF)4] reported by Ruhlandt-Senge et al.28 The adopted level of calculation can describe the B–H⋯M interaction satisfactorily. The variation in ionic radii for alkaline earth metal ions plays a major role in the interaction of these metal ions with ligands as well as solvents. The Sr–S distances are symmetric, but only two of the Ba–S distances are the same. Although Ca and Sr show similar interaction towards the solvent THF molecules, the Ba–O distance is the highest among these three. We observed a clear trend of increase in M–S distances as well as M⋯H(BH2) distances with the increase in ionic radii of metal ions. Though experimental data for the Ca complex is not available, our computational calculations reveal that calcium can make shortest B–H⋯M contact among the alkaline earth metal ions. The shortest M⋯H distances are 2.35, 2.67, 2.71, and 2.84 Å for Na, Ca, Sr and Ba, respectively. It is noteworthy that the experimentally obtained value for the Ba complex is between those obtained for the Na and Sr complexes, indicating the stronger M⋯H interactions between barium and hydrogen atoms compared to other analogues. For a particular metal ion, the hydride interactions with two BH2 groups are similar, except for Na, where we observed different types of such interactions due to the formation of polymeric forms.
Table 2 Comparison of computed M–O, M–S and M⋯H distances with experimental values (where applicable) for the sodium, calcium, strontium and barium complexes
Distance (Å) |
M |
Na |
Ca |
Sr |
Ba |
B3LYP |
Expt |
B3LYP |
B3LYP |
Expt |
B3LYP |
Expt |
M–O |
2.27 |
2.329 |
2.44 |
2.57 |
2.552 |
2.75 |
2.702 |
2.30 |
2.330 |
2.44 |
2.57 |
2.552 |
2.80 |
2.805 |
— |
— |
— |
2.80 |
2.813 |
M–S |
2.87 |
2.789 |
3.04 |
3.18 |
3.017 |
3.46 |
3.273 |
2.87 |
2.814 |
3.04 |
3.18 |
3.017 |
3.46 |
3.355 |
2.99 |
2.892 |
3.10 |
3.23 |
2.991 |
3.53 |
3.449 |
3.12 |
2.923 |
3.10 |
3.23 |
2.991 |
3.53 |
3.346 |
M⋯H |
2.74 |
2.497 |
2.67 |
2.71 |
2.806 |
2.84 |
2.729 |
2.35 |
2.488 |
2.67 |
2.71 |
2.806 |
2.84 |
2.743 |
Experimental
General consideration
All manipulations involving air- and moisture-sensitive organometallic compounds were carried out under argon using the standard Schlenk technique or argon-filled glove box. Hydrocarbon solvents (n-pentane, toluene) were distilled under nitrogen from LiAlH4 and stored in the glove box. THF was dried and deoxygenated by distillation over sodium benzophenone ketyl under argon and then distilled and dried over CaH2 prior to storing in the glove box. Dimethylsuphoxide (DMSO-d6) was dried over CaH2, distilled and stored in the glove box. 1H NMR (400 MHz), 13C{1H} NMR (100 MHz) and 11B{1H} (128.3 MHz) spectra were measured on a BRUKER AVANCE III-400 spectrometer. Elemental analyses were performed on a BRUKER EURO EA at the Indian Institute of Technology Hyderabad. Sodium dihydrobis(2-thiopyridone)borate (1) was prepared according to procedures prescribed in the literature.8a
General preparation of [κ2-{(2-SC5H4N)2-(BH2)}2M(THF)n]: M = Ca (2), Sr (3) n = 2; M = Ba (4), n = 3]
A 25 mL Schlenk flask was charged with one equivalent of alkaline earth metal diiodide and two equivalents of sodium dihydrobis(2-thiopyridone)borate (1). 10 mL of THF was added to it and the reaction mixture was kept for stirring at ambient temperature for 12 hours. White precipitate of sodium iodide was separated through a G4 frit. The solvent was evaporated to dryness under vacuo to give a green residue. The title compounds were re-crystallised from THF at −35 °C.
M = Ca (2). Yield 144 mg 65%. 1H NMR (400 MHz, DMSO-d6, 25° C): δ 8.56 [dd, 4H, 3JHH = 6.2 Hz, 4JHH = 1.2 Hz, mpCH-(6)], 7.12 [d, 4H, 3JHH = 8.2 Hz, mpCH-(3)], 7.02 [τd, 4H, 3JHH = 6.8 Hz, 4JHH = 1.8 Hz, mpCH-(4)], 6.44 [τd, 4H, 3JHH = 6.5 Hz, 4JHH = 1.3 Hz, mpCH (5)], 3.58 (THF), 3.40 (br, 2H, (BH2))], 1.74 (THF) ppm. 11B{1H} NMR (128.3 MHz, DMSO-d6, 25 °C): δ 1.11 ppm. 13C{1H} NMR (100.6 MHz, DMSO-d6, 25 °C): δ 182.5 (C
S), 150.5 [mpCH-(6)], 133.8 [mpCH-(3)], 133.1 [mpCH-(4)], 109.8 [mpCH-(5)], 66.9 (THF), 25.0 (THF) ppm. FTIR (selected peaks): 2354 (w, B–H), 1607 (s), 1533 (s), 1259 s, 753 (s), 554 (m) cm−1. Elemental analysis calculated (%) for (C28H36B2N4O2S4Ca) (650.57): C 51.69, H 5.58, N 8.61; found C 51.28, H 5.33, N 8.39.
M = Sr (3). Yield 133 mg 65%. 1H NMR (400 MHz, DMSO-d6, 25 °C): δ 8.57 [d, 4H, 3JHH = 5.7 Hz, mpCH-(6)], 7.19 [d, 4H, 3JHH = 5.7 Hz, mpCH-(3)], 7.12 [d, 4H, 3JHH = 8.0 Hz, mpCH-(4)], 6.43 [dd, 4H, 3JHH = 6.2 Hz, 4JHH = 1.0 Hz, mpCH-(5)], 3.59 (br, 2H, (BH2))], 3.56 (THF), 1.74 (THF) ppm. 11B{1H} NMR (128.3 MHz, DMSO-d6, 25 °C): δ 0.82 ppm. 13C{1H} NMR (100.6 MHz, DMSO-d6, 25 °C): δ 182.4 (C
S), 150.4 [mpCH-(6)], 133.8 [mpCH-(3)], 133.1 [mpCH-(4)], 109.8 [mpCH-(5)], 67.0 (THF), 25.0 (THF) ppm. FTIR selected value: 2496 (w), 2387 (w, B–H), 1608 (s), 1532 (s), 749 (s), 519 (w) cm−1. Elemental analysis calculated (%) for (C28H36B2N4O2S4Sr) (698.11): C 48.17, H 5.20, N 8.03; found C 47.72, H 4.93, N 7.81.
M = Ba (4). Yield 142 mg 68%. 1H NMR (400 MHz, DMSO-d6, 25 °C): δ 8.77 [dd, 4H, 3JHH = 6.3 Hz, 4JHH = 1.2 Hz, mpCH-(6)], 7.13 [d, 4H, 3JHH = 7.9 Hz, mpCH-(3)], 7.01 [d, 4H, 3JHH = 6.0 Hz, mpCH-(4)], 6.43 [d, 4H, 3JHH = 5.1 Hz, mpCH-(5)], 3.59 (THF), 3.52 (br, 2H, (BH2))], 1.71 (THF) ppm. 11B{1H} NMR (128.3 MHz, DMSO-d6, 25 °C): δ 1.73 ppm. 13C{1H} NMR (100.6 MHz, DMSO-d6, 25 °C): δ 182.9 (C
S), 150.6 [mpCH-(6)], 134.0 [mpCH-(3)], 132.8 [mpCH-(4)], 109.6 [mpCH-(5)], 68.2 (THF), 25.7 (THF) ppm. FTIR selected value: 2375 (w, B–H), 1604 (s), 1532 (s), 797 (s), 520 (w) cm−1. Elemental analysis calculated (%) for (C32H44B2N4O3S4Ba) (819.93): C 46.88, H 5.41, N 6.83; found C 46.31, H 5.09, N 6.52.
X-ray crystallographic studies of complexes 1, 3 and 4
X-ray crystallographic analyses. Single crystals of complex 1 were obtained from a concentrated solution of THF at 15 °C while single crystals of complexes 3 and 4 were obtained from their respective concentrated THF solutions under argon atmosphere at a temperature of −35 °C. In each case, a crystal of suitable dimensions was mounted on a CryoLoop (Hampton Research Corp.) with a layer of light mineral oil and placed in a nitrogen stream at 150(2) K. All measurements were made on an Agilent Supernova X-calibur Eos CCD detector with either graphite-monochromatic Cu-Kα (1.54184 Å, for 1) or Mo-Kα (0.71073 Å for 3 and 4) radiation. Crystal data and structure refinement parameters are summarised in Table 1. The structures were solved by direct methods (SIR2004)29 and refined on F2 using the full-matrix least-squares method, using SHELXL-97.30 Non-hydrogen atoms were anisotropically refined. H-atoms were included in the refinement on calculated positions riding on their carrier atoms. The function minimised was [∑w(Fo2 − Fc2)2] (w = 1/[σ2 (Fo2) + (aP)2 + bP]), where P = (Max(Fo2, 0) + 2Fc2)/3 with σ2(Fo2) from counting statistics. The function R1 and wR2 were (Σ||Fo| − |Fc||)/Σ|Fo| and [Σw(Fo2 − Fc2)2/Σ(wFo4)]1/2 respectively. The DIAMOND-3 and ORTEP-III programs were used to draw the molecule (ESI).†
Conclusion
To sum up, we have reported the syntheses of calcium, strontium and barium complexes of the Bmp ligand. In the solid-state structure of the strontium complex 3, a symmetric attachment, κ2, of the Bmp ligand to the metal ion is observed. However, an asymmetric κ2 SS-ligation of Bmp to the barium ion is observed in complex 4. These symmetric and asymmetric attachments are the manifestation of two tautomeric forms, 2-thiopyridone and pyridine-2-thiolate, of the Bmp ligand. We also observed that the sodium complex of the Bmp ligand is polymeric in the solid state. Our computational study also matches well with our experimental observations; in addition, the calculated calcium complex structure is also in agreement with the higher analogues of Sr and Ba complexes.
Acknowledgements
This work is supported by the Ministry of New and Renewable Energy (MNRE), India under project no. 103/209/2013-NT, dated: 29th September, 2014. The instrumental facilities were provided by the Indian Institute of Technology Hyderabad (IITH). K. N. and Th. D. N. R. thank the University Grants Commission (UGC), India, for his PhD fellowship. We sincerely thank Prof. Kazushi Mashima and Dr Hayato Tsurugi, Osaka University, for their generous support. We thank all the reviewers for their valuable comments and special thanks to crystallographic reviewer to help us to improve the crystal structure of complex 4.
Notes and references
-
(a) S. Trofimenko, Scorpionates: The Coordination of Poly(pyrazolyl)borate Ligands, Imperial College Press, London, 1999 Search PubMed;
(b) S. Trofimenko, Chem. Rev., 1993, 93, 943 CrossRef CAS;
(c) S. Trofimenko, Polyhedron, 2004, 23, 197 CrossRef CAS;
(d) C. Pettinari, Scorpionates II: Chelating Borate Ligands, Imperial College Press, London, 2008 Search PubMed.
- S. Trofimenko, J. Am. Chem. Soc., 1966, 88, 1842 CrossRef CAS.
- H. Vahrenkamp, Acc. Chem. Res., 1999, 32, 589 CrossRef CAS.
-
(a) C. Slugovc, I. Padilla-Martınez, S. Sirol and E. Carmona, Coord. Chem. Rev., 2001, 213, 129 CrossRef CAS;
(b) C. Slugovc, R. Schmid and K. Kirchner, Coord. Chem. Rev., 1999, 185–186, 109 CrossRef CAS.
- H. V. R. Dias and T. K. H. H. Goh, Polyhedron, 2004, 23, 273 CrossRef CAS.
- A. F. Hill, G. R. Owen, A. J. P. White and D. J. Williams, Angew. Chem., Int. Ed., 1999, 38, 2759 CrossRef CAS.
-
(a) I. Kuzu, I. Krummenacher, F. Armbruster and F. Breher, Dalton Trans., 2008, 5836 RSC;
(b) F.-G. Fontaine, J. Boudreau and M.-H. Thibault, Eur. J. Inorg. Chem., 2008, 35, 5439 CrossRef;
(c) H. Braunschweig, C. Kollann and D. Rais, Angew. Chem., Int. Ed., 2006, 45, 5254 CrossRef CAS PubMed.
-
(a) G. Dyson, A. Hamilton, B. Mitchell and G. R. Owen, Dalton Trans., 2009, 6120 RSC;
(b) G. C. Rudolf, A. Hamilton, A. G. Orpen and G. R. Owen, Chem. Commun., 2009, 553 RSC;
(c) G. R. Owen, H. P. Gould, J. P. H. Charmant, A. Hamilton and S. Saithong, Dalton Trans., 2010, 39, 392 RSC.
-
(a) G. Dyson, A. Zech, B. W. Rawe, M. F. Haddow, A. Hamilton and G. R. Owen, Organometallics, 2011, 30, 5844 CrossRef CAS;
(b) A. Zech, M. F. Haddow, H. Othman and G. R. Owen, Organometallics, 2012, 31, 6753 CrossRef CAS.
-
(a) C. A. Kosky and P. Ganis, Acta Crystallogr., Sect. B: Struct. Crystallogr. Cryst. Chem., 1971, 27, 1859 CrossRef CAS;
(b) F. A. Cotton, M. Jeremic and A. Shaver, Inorg. Chim. Acta, 1972, 6, 543 CrossRef CAS.
-
(a) V. Rodriguez, I. Atheaux, B. Donnadieu, S. Sabo-Etienne and B. Chaudret, Organometallics, 2000, 19, 2916 CrossRef CAS;
(b) S. L. Kuan, W. K. Leong, L. Y. Goh and R. D. Webster, J. Organomet. Chem., 2006, 691, 907 CrossRef CAS;
(c) G. C. Rudolf, A. Hamilton, A. G. Orpen and G. R. Owen, Chem. Commun., 2009, 553 RSC;
(d) G. R. Owen, N. Tsoureas, R. F. Hope, Y.-Y. Kuo and M. F. Haddow, Dalton Trans., 2011, 40, 5906 RSC;
(e) M. D. Spicer and J. Reglinski, Eur. J. Inorg. Chem., 2009, 1553 CrossRef CAS.
-
(a) I. R. Crossley and A. F. Hill, Dalton Trans., 2008, 201 RSC;
(b) N. Tsoureas, M. F. Haddow, A. Hamilton and G. R. Owen, Chem. Commun., 2009, 2538 RSC;
(c) N. Tsoureas, T. Bevis, C. P. Butts, A. Hamilton and G. R. Owen, Organometallics, 2009, 28, 5222 CrossRef CAS.
-
(a) E. Khaskin, P. Zavalij and A. N. Vedernikov, Angew. Chem., Int. Ed., 2007, 46, 6309 CrossRef CAS PubMed;
(b) E. Khaskin, P. Y. Zavalij and A. N. Vedernikov, J. Am. Chem. Soc., 2008, 130, 10088 CrossRef CAS PubMed.
-
(a) K. Naktode, R. K. Kottalanka and T. K. Panda, New J. Chem., 2012, 36, 2280 RSC;
(b) R. K. Kottalanka, K. Naktode and T. K. Panda, J. Mol. Struct., 2013, 1036, 188 CrossRef CAS;
(c) R. K. Kottalanka, K. Naktode, S. Anga, H. P. Nayek and T. K. Panda, Dalton Trans., 2013, 42, 4947 RSC;
(d) R. K. Kottalanka, S. Anga, S. K. Jana and T. K. Panda, J. Organomet. Chem., 2013, 740, 104 CrossRef CAS;
(e) R. K. Kottalanka, S. Anga, K. Naktode, P. Laskar, H. P. Nayek and T. K. Panda, Organometallics, 2013, 32, 4473 CrossRef CAS;
(f) R. K. Kottalanka, K. Naktode, S. Anga and T. K. Panda, Phosphorus, Sulfur Silicon Relat. Elem., 2014, 189, 1624 CrossRef CAS;
(g) R. K. Kottalanka, A. Harinath, J. Bhattacharjee, H. V. Babu and T. K. Panda, Dalton Trans., 2014, 43, 8757 RSC;
(h) J. Bhattacharjee, R. K. Kottalanka, H. Adimulam and T. K. Panda, J. Chem. Sci., 2014, 126, 1463–1475 CrossRef CAS.
- Selected references for Na⋯H interactions
(a) H. Noth and S. Thomas, Eur. J. Inorg. Chem., 1999, 1373 CrossRef CAS;
(b) D. L. Reger, J. E. Collins, W. A. Flomer, A. L. Rheingold, C. Incarvito and L. A. Guzei, Polyhedron, 2001, 20, 2491 CrossRef CAS;
(c) H.-H. Giese, T. Habereder, H. Noth, W. Ponikwar, S. Thomas and M. Warchhold, Inorg. Chem., 1999, 38, 4188 CrossRef CAS;
(d) J. C. G. Ruiz, H. Noth and M. Warchhold, Eur. J. Inorg. Chem., 2008, 251 CrossRef CAS;
(e) L. Deng, H.-S. Chan and Z. Xie, J. Am. Chem. Soc., 2006, 128, 5219 CrossRef CAS PubMed.
-
(a) S. Harder, Chem. Rev., 2010, 110, 3852 CrossRef CAS PubMed;
(b) S. Kobayashi and Y. Yamashita, Acc. Chem. Res., 2011, 44, 58 CrossRef CAS PubMed.
-
(a) T. P. Hanusa, in Comprehensive Organometallic Chemistry III, ed. R. H. Crabtree and M. P. Mingos, Elsevier, Oxford, 2007, vol. 2, p. 67 Search PubMed;
(b) T. P. Hanusa, Organometallics, 2002, 21, 2559 CrossRef CAS;
(c) T. P. Hanusa, Chem. Rev., 2000, 100, 1023 Search PubMed;
(d) T. P. Hanusa, Coord. Chem. Rev., 2000, 210, 329 CrossRef CAS.
- K. Ruhlandt-Senge, K. Davis, S. Dalal, U. Englich and M. O. Senge, Inorg. Chem., 1995, 34, 2587 CrossRef CAS.
- I. K. Bezougli, A. Bashall, M. McPartlin and D. M. P. Mingos, J. Chem. Soc., Dalton Trans., 1997, 287 RSC.
- P. Mikulcik, P. R. Raithby, R. Smith and D. S. Wright, Angew. Chem., Int. Ed. Engl., 1991, 30, 428 CrossRef.
-
(a) A. Cetin and C. J. Ziegler, Dalton Trans., 2006, 1006 RSC;
(b) M. G. Davidson, C. T. O'Hara, M. D. Jones, C. G. Keir, M. F. Mahon and G. K. Köhn, Inorg. Chem., 2007, 46, 7686 CrossRef CAS PubMed.
- R. D. Shannon, Acta Crystallogr., Sect. A: Cryst. Phys., Diffr., Theor. Gen. Crystallogr., 1976, 32, 751 CrossRef.
- M. G. Kunnath, W. Teng, W. Vargas and K. Ruhlandt-Senge, Inorg. Chem., 2005, 44, 4862 CrossRef PubMed.
- R. Dennington, T. Keith and J. Millam, GaussView, Version 5, Semichem Inc., Shawnee Mission KS, 2009 Search PubMed.
- M. J. Frisch, G. W. Trucks, H. B. Schlegel, G. E. Scuseria, M. A. Robb, J. R. Cheeseman, G. Scalmani, V. Barone, B. Mennucci, G. A. Petersson, H. Nakatsuji, M. Caricato, X. Li, H. P. Hratchian, A. F. Izmaylov, J. Bloino, G. Zheng, J. L. Sonnenberg, M. Hada, M. Ehara, K. Toyota, R. Fukuda, J. Hasegawa, M. Ishida, T. Nakajima, Y. Honda, O. Kitao, H. Nakai, T. Vreven, J. A. Montgomery Jr, J. E. Peralta, F. Ogliaro, M. Bearpark, J. J. Heyd, E. Brothers, K. N. Kudin, V. N. Staroverov, T. Keith, R. Kobayashi, J. Normand, K. Raghavachari, A. Rendell, J. C. Burant, S. S. Iyengar, J. Tomasi, M. Cossi, N. Rega, J. M. Millam, M. Klene, J. E. Knox, J. B. Cross, V. Bakken, C. Adamo, J. Jaramillo, R. Gomperts, R. E. Stratmann, O. Yazyev, A. J. Austin, R. Cammi, C. Pomelli, J. W. Ochterski, R. L. Martin, K. Morokuma, V. G. Zakrzewski, G. A. Voth, P. Salvador, J. J. Dannenberg, S. Dapprich, A. D. Daniels, O. Farkas, J. B. Foresman, J. V. Ortiz, J. Cioslowski and D. J. Fox, Gaussian 09, Revision B.01, Gaussian, Inc., Wallingford CT, 2010 Search PubMed.
-
(a) D. Becke, J. Chem. Phys., 1993, 98, 5648 CrossRef;
(b) C. Lee, W. Yan and R. G. Parr, Phys. Rev. B: Condens. Matter Mater. Phys., 1988, 37, 785 CrossRef CAS.
- M. F. Bush, J. Oomens, R. J. Saykally and E. R. Williams, J. Am. Chem. Soc., 2008, 130, 6463 CrossRef CAS PubMed.
-
(a) U. Englich and K. Ruhlandt-Senge, Z. Anorg. Allg. Chem., 2001, 627, 851 CrossRef CAS;
(b) S. Chadwick, U. Englich, B. Noll and K. Ruhlandt-Senge, Inorg. Chem., 1998, 37, 4718 CrossRef CAS PubMed.
- M. Sheldrick, SHELXS-97, Program of Crystal Structure Solution, University of Göttingen, Germany, 1997 Search PubMed.
- G. M. Sheldrick, Acta Crystallogr., Sect. A: Found. Crystallogr., 2008, 64, 112 CrossRef CAS PubMed.
Footnote |
† Electronic supplementary information (ESI) available. CCDC 1049442–1049444. For ESI and crystallographic data in CIF or other electronic format see DOI: 10.1039/c5ra04696c |
|
This journal is © The Royal Society of Chemistry 2015 |
Click here to see how this site uses Cookies. View our privacy policy here.