DOI:
10.1039/C5RA01915J
(Paper)
RSC Adv., 2015,
5, 28879-28884
C–N cross-coupling reaction catalysed by reusable CuCr2O4 nanoparticles under ligand-free conditions: a highly efficient synthesis of triarylamines
Received
30th January 2015
, Accepted 17th March 2015
First published on 17th March 2015
Abstract
A convenient, efficient and ligand-free method for the C–N coupling reaction of anilines and aryl iodides was performed using CuCr2O4 nanoparticles. Copper chromite nanocatalyst improved the rate and facility of the synthesis of triarylamines. The heterogeneous catalyst was fully characterized by scanning electron microscopy, IR and X-ray diffraction techniques. Recyclability, excellent yields of products and short reaction times are the important advantages of this ligand-free procedure by using the CuCr2O4 nanoparticles.
1. Introduction
The formation of C–N bonds is widespread in numerous compounds such as triarylamines which are used in the synthesis of biological,1 pharmaceutical,2 natural products,3 dyes4 and polymers.5–8 The most common synthetic procedure for the preparation of triarylamines is the palladium9,10- or copper11–13-assisted classic Ullman coupling reaction. This reaction often needs harsh conditions such as high temperature (>200 °C) and stoichiometric or greater amount of copper reagent.14–16 Low yield of triarylamines and sensitivity to catalyst type are other limitation of the Ullman reaction. Recently, many researches has been focused to develop catalytic systems to decrease the environmental impact of the methods.17–20 Palladium and complexes with chelating ligands21–25 have been investigated significantly for the cross-coupling of anilines with aryl halides. In these systems, the electron-rich ligand chelated with the metal displays a critical role in the C–N cross coupling. Different chelating ligands were examined for the single step synthesis of triarylamines. Use of chelating ligands leads to the formation of triarylamine derivatives selectively with high catalytic activity. Bidentate ligands shown higher activity.26 Diazabutadienes (DABs) were demonstrated as efficient ligands in the Cu-catalyzed C–N coupling reaction for the synthesis of triarylamines.27 The recent work reported preparation of various triphenylamines in good yields using CuCl with 8-hydroxyquinoline as the chelating ligand.28
Gujadhur et al. reported a synthetic protocol for the synthesis of functionalized diaryl- and triarylamines under mild conditions, using Cu(PPh3)3Br as the catalyst and cesium carbonate as the base. With PPh3 as a ligand the reaction did not proceed efficiently.29 Furthermore, triarylamines was synthesized by ligand-free copper iodide using Cs2CO3 as the base.30 The ligand-free copper-catalysed Ullman reaction was carried out in tetraethyl orthosilicate (TEOS) as the solvent and this method needs high temperature (145 °C) and long reaction time to terminate the reaction.
Also we reported an efficient method for the synthesis of triarylamine derivatives mediated by copper iodide nanoparticles and 1,10-phenanthroline ligand. This system enhanced the rate and ease of reaction and exhibited a high influence in the synthesis of various amine derivatives. But CuI nanoparticle requires a ligand to have effective performance and it is not recyclable.31
Nanoparticles containing high surface-to-volume ratio and reactive morphologies have been used as suitable catalysts for organic reactions.32–34 Moreover the nanomaterial catalyzed synthesis provide the advantages of high atom efficiency, simple separation of product and easy recovery and recyclability of the nanocatalysts. In the recent years, copper chromite (CuCr2O4) has been applied extensively as a heterogeneous catalyst in many reactions. These catalyst are inexpensive, very stable with strong resistance to acids and alkalis, and they have high melting points. These properties make it appropriate for use as solid heterogeneous catalysts in organic reactions.35–37 Copper chromite nanoparticles indicated an important level of performance as catalysts in terms of reactivity and selectivity in improvement of organic synthesis yields.38–41
In this research we reported an efficient catalysis of CuCr2O4 nanoparticles for the C–N cross-coupling reactions of anilines with iodobenzenes. Our procedure is simple, convenient and ligand-free, and the CuCr2O4 nanoparticles can be recycled.
2. Results and discussion
2.1. Characterization of CuCr2O4 nanoparticles
Nano crystalline of Copper chromite was characterized by IR, SEM and XRD analysis. Fig. 1 represents XRD pattern of CuCr2O4 nanoparticles. All of the detected diffraction peaks are indexed by the spinel-phase structure of CuCr2O4 (JCPDS no. 88-0110) demonstrating a high phase purity of the product. The average crystalline size was estimated using the Scherrer formula on the highest intensity peak for each sample. An average size was obtained around 20–30 nm for CuCr2O4 nanoparticles.
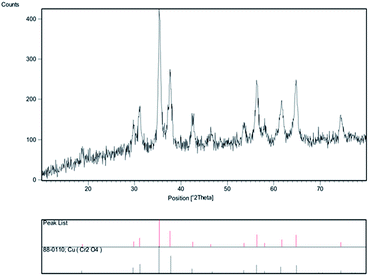 |
| Fig. 1 The XRD pattern of CuCr2O4 nanoparticles. | |
The SEM image of nano CuCr2O4 proves that the nanoparticles have a uniform size and spherical shape as shown in Fig. 2.
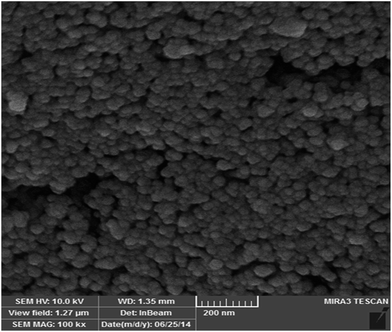 |
| Fig. 2 SEM image of CuCr2O4 nanoparticles. | |
The FT-IR spectra of CuCr2O4 nanoparticles was illustrated in Fig. 3. As shown in this figure, the absorption bands at 615 cm−1 and 514 cm−1 refers to Cr2O42− group. Also, the bands at 911 cm−1 and 999 cm−1 were assigned to Cr–O chromate group.
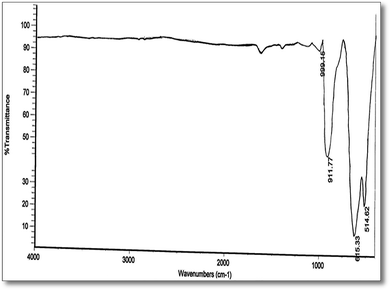 |
| Fig. 3 FT-IR spectrum of CuCr2O4 nanoparticles. | |
2.2. Synthesis of triaryl amines by CuCr2O4 nanoparticle
The C–N cross coupling reaction was performed by the Ullman condensation of aryl amine with aryl iodide (molar ratio 1
:
2) in the presence of potassium hydroxide as the base and CuCr2O4 nano catalyst in toluene at reflux condition under nitrogen atmosphere (Scheme 1).
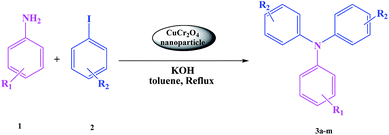 |
| Scheme 1 Synthesis of triarylamine derivatives. | |
Catalytic effects of five types of catalysts including CuBr, CuCl, CuI, CuO, CuCr2O4 were investigated in the reaction of aniline and iodo-benzene as listed in Table 1. The results of this table shows that the reaction was carried out efficiently in the presence of nano CuCr2O4. The highest yield was obtained by 4% mol of copper chromite nanoparticle (Table 1, entry 7). Notably, increasing of this amount did not show any change in yield and time of reaction. Also the reaction was performed by nano CuCr2O4 (4%) and 1,10-phenantroline (4%) as a ligand and it was not obtained better yield (Table 1, entry 8). Entry 9 in Table 1 represent performance of CuI nanoparticles in the synthesis of triphenylamine.28 This system requires 1,10-phenantroline to achieve good yield of product. While CuCr2O4 nano catalyst without 1,10-phenantroline shows high influence in the synthesis of triarylamines with better yields. This behaviour can be described that chromite plays role of ligand in the CuCr2O4. It should be noted that the reaction did not progress in the absence of catalyst (Table 1, entry 10).
Table 1 Optimization of model reaction using various catalysts
Entry |
Catalytic system (mol%) |
Time (h) |
Yielda (%) |
Isolated yield. |
1 |
CuCl (20%) |
48 |
29 |
2 |
CuBr (20%) |
48 |
33 |
3 |
CuI (20%) |
35 |
48 |
4 |
CuO (20%) |
35 |
59 |
5 |
CuCr2O4 (20%) |
30 |
66 |
6 |
Nano CuCr2O4 (3%) |
6 |
92 |
7 |
Nano CuCr2O4 (4%) |
6 |
95 |
8 |
Nano CuCr2O4 (4%), 1,10-phen (4%) |
6 |
93 |
9 |
Nano CuI (3%), 1,10-phen (3%) |
8 |
92 |
10 |
Without catalyst |
48 |
Trace |
Furthermore we have examined various solvents with varying polarity for this reaction. It was demonstrated that toluene was the best choice over any organic solvents such as 1,4-dioxane, THF, acetonitrile and xylene (Table 2).
Table 2 Optimization of reaction solvent for the synthesis of triphenylamine
Entry |
Solvent/condition |
Time (h) |
Yielda (%) |
Isolated yield. |
1 |
CH3CN/reflux |
8 |
71 |
2 |
THF/reflux |
10 |
62 |
3 |
1,4-Dioxane/reflux |
10 |
65 |
4 |
Xylene/reflux |
6 |
92 |
5 |
Toluene/reflux |
6 |
95 |
6 |
Toluene/80 °C |
8 |
76 |
We then examined the effect of leaving group in aryl halides applied in the C–N cross-coupling reactions using CuCr2O4 nanoparticles. Aryl chloride, aryl bromide and aryl iodide were investigated for this protocol. Aryl iodide is more effective for the synthesis of triarylamines as resulted in Table 3. The reaction of aniline with 2.5 equiv. of bromobenzene or chlorobenzene afforded triphenylamines in lower yield than iodobenzene (Table 3).
Table 3 Investigation of leaving group effect in Ar-X for the synthesis of triphenylamine using CuCr2O4 nanoparticlesa
Entry |
X |
Time (h) |
Yielda (%) |
Isolated yield. |
1 |
Cl |
18 |
43 |
2 |
Br |
15 |
64 |
3 |
I |
6 |
95 |
We also applied CuCr2O4 nano catalyst in synthesis of triarylamine derivatives from various aryl amines and aryl iodides under similar condition as represented in Table 4. The results of this table indicates that the excellent yields were achieved in the C–N cross coupling reaction at the presence of nano CuCr2O4 (4% mol) under reflux. Electron-withdrawing and electron-donating substituents for aryl halide and aniline were given good to excellent yields of triarylamines under above-mentioned conditions. The highest yields of triarylamine were obtained by the electron donating para-methoxy group as substituent for aryl halide and aniline (Table 4, entries 6 and 12). Although ortho-substituted aniline and aryl halide were resulted in good yield (entries 4 and 10 in Table 4) but, due to steric effects, yields are lower than para-substituted aniline. Aryl iodides represent higher reactivity than aryl bromides in the Ullman reaction. The coupling reaction of 1-bromo-4-iodobenzene and aniline derivatives formed the products containing bromide on the aromatic ring (Table 4, entries 2–7).
Table 4 Synthesis of triaryl amines using CuCr2O4 nanoparticlesa
2.3. Proposed mechanism
A probable mechanism for the C–N cross coupling reaction using CuCr2O4 nanoparticle has been illustrated in Scheme 2. The synthesis of triaryl amines occurs by oxidative addition followed by the reductive elimination. These processes performed on the nano Cu Cr2O4 which have highly effective catalytic behaviour in terms of its high surface area.
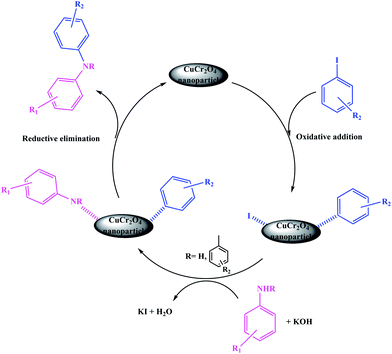 |
| Scheme 2 Proposed reaction mechanism for the preparation of triarylamine by CuCr2O4 nanoparticles. | |
2.4. Reusability experiments
The CuCr2O4 nano catalyst is recyclable without loss of activity (Fig. 4). After completion of the Ullman reaction, the mixture was treated with water and CH2Cl2. The CuCr2O4 nanoparticles were recovered from the aqueous phase by centrifugation. It was reused for the new C–N cross-coupling reaction of aniline with iodobenzene for five runs, and minimal loss of activity was detected providing the products in high yield.
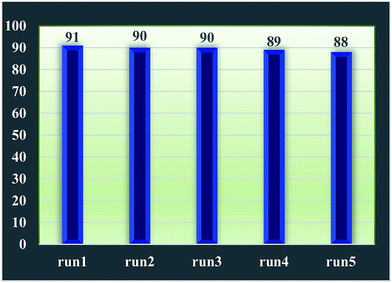 |
| Fig. 4 Reusability of nano CuCr2O4 for model reaction. | |
3. Conclusions
In this research a simple and efficient procedure is developed for the C–N cross coupling reaction of anilines and aryl halides using CuCr2O4 nanoparticles as a highly efficient heterogeneous catalyst under ligand-free conditions. The catalyst is recyclable and provide excellent yields of triarylamines and short reaction times. The conditions was very mild, neutral and environmentally benign.
4. Experimental
4.1. Chemicals and apparatus
All reagents and solvents were purchased from Merck (Germany) and Sigma-Aldrich and used without more purification. Melting points were determined by Electro thermal 9200.1H NMR and 13C NMR spectra were recorded with a Bruker Avance-400 MHz. The NMR spectra were achieved in CDCl3 and DMSO-d6 solution and are reported as parts per million (ppm) downfield from tetramethylsilane as internal standard. The IR spectra were recorded on FT-IR Magna 550 apparatus using with KBr plates. EIMS (70 eV) was performed by Finnigan-MAT-8430 mass spectrometer in m/z. The elemental analyses (C. H. N) of the samples were carried out using a LECO CHNS 923 analyser.
Powder X-ray diffraction (XRD) of CuCr2O4 nanoparticles was performed on a Philips diffractometer of X'pert Company with monochromatized Cu Kα radiation (λ = 1.5406 Å).
Microscopic morphology was visualized by SEM (MIRA 3 TESCAN).
4.2. Preparation of CuCr2O4 nanoparticles
Copper chromite nanoparticle has been prepared by coprecipitation method. Stoichiometric amount of Cu+2 (0.005 mol, 1.4 g Cu (NO3)2·6H2O) and Cr3+ (0.01 mol, 4 g Cr(NO3)3·9H2O) were poured in 50 ml distilled water. Afterward the mixture was added into 100 ml distilled water containing capping agent (glycerol, 5% in distilled water) under stirring. Subsequently, 1.5 M aqueous solution of precipitating agent (NaOAc) was added drop-wise until the pH value of the solution adjusted to 10. During the procedure, the temperature of the mixture was maintained about 60 °C. Then, the temperature was increased to 80 °C at which the precipitation took place. The precipitates were centrifuged and then washed with distilled water and ethanol several times and then dried at 60 °C in an oven for 2 h. Finally, calcination was carried out at 600 °C for 5 h. CuCr2O4 nanoparticles were produced in 86% yield.
4.3. General procedure for the amination reaction
The preparation of triaryl amines derivatives was performed in a two-necked round bottomed flask under nitrogen atmosphere. A 10 ml toluene solution of anilines (1 mmol), aryl iodides (2.5 mmol), CuCr2O4 nanoparticles (4% mol), potassium hydroxide (0.43 g, 8 mmol) was stirred under nitrogen atmosphere at reflux for a desired time. At the completion of reaction (followed by TLC) the mixture was cooled to room temperature and poured into distilled water. The extraction of products were carried out by CH2Cl2 and the organic layer was dried over anhydrous sodium sulphate (Na2SO4). Then the solvent was evaporated in vacuo, the products were purified using silica column chromatography by normal hexane to afford triarylamines.
4.4. Spectral data for triarylamine derivatives
Triphenylamine (3a). White solid. 1H NMR (400 MHz, CDCl3): δ (ppm) 7.08 (m, 9H), 7.26 (d, 6H), 13C NMR (100 MHz, CDCl3): δ (ppm) 124.82, 125.46, 135.62, 147.65. FT-IR (KBr): 3031, 3016, 1590, 1454, 1276 cm−1. MS (EI, 70 eV): m/z 244 (M+), anal. calcd for C18H15N: C, 88.16; H, 6.12; N, 5.72%. Found: C, 87.95; H, 6.01; N, 5.62%.
N,N-Bis(4-bromophenyl)aniline (3b). Colorless viscous liquid. 1H NMR (400 MHz, CDCl3): δ (ppm) 6.95 (d, 4H), 7.06 (m, 3H), 7.27 (d, 2H), 7.35 (d, 4H). 13C NMR (100 MHz, CDCl3): δ (ppm) 115.53, 123.82, 124.66, 125.46, 129.62, 132.22, 146.57, 146.96. FT-IR (KBr): 3071, 3056, 1579, 1484, 1274, 1070 cm−1. MS (EI, 70 eV): m/z 400 (M+), anal. calcd for C18H13NBr2: C, 53.87; H, 3.24; N, 3.50%. Found: C, 53.61; H, 3.03; N, 3.35%.
4-Methyl-N,N-bis(4-bromophenyl)aniline (3c). White solid. 1H NMR (400 MHz, CDCl3): δ (ppm) 2.32 (s, 3H), 6.91 (d, 4H), 6.96 (d, 2H), 7.09 (d, 2H), 7.31 (d, 4H). 13C NMR (100 MHz, CDCl3): δ (ppm) 20.89, 114.94, 124.92, 125.20, 130.25, 132.23, 133.86, 144.29, 146.69. FT-IR (KBr): 3033, 2922, 1578, 1483, 1276, 1070 cm−1. MS (EI, 70 eV): m/z 415 (M+), anal. calcd for C19H15NBr2: C, 54.70; H, 3.60; N, 3.36%. Found: C, 54.63; H, 3.49; N, 3.28%.
2-Methoxy-N,N-bis(4-bromophenyl)aniline (3d). White solid. 1H NMR (400 MHz, CDCl3): δ (ppm) 3.69 (s, 3H), 6.93 (d, 4H), 7.01 (d, 1H), 7.12 (m, 3H), 7.32 (d, 4H). 13C NMR (100 MHz, CDCl3): δ (ppm) 56.48, 115.45, 122.32, 124.92, 125.65, 129.25, 132.03, 132.86, 145.12, 145.97. FT-IR (KBr): 3031, 2935, 1585, 1472, 1272, 1072 cm−1. MS (EI, 70 eV): m/z 430 (M+), anal. calcd for C19H15NOBr2: C, 52.68; H, 3.47; N, 3.23%. Found: C, 52.51; H, 3.38; N, 3.21%.
3-Methoxy-N,N-bis(4-bromophenyl)aniline (3e). White solid. 1H NMR (400 MHz, CDCl3): δ (ppm) 3.72 (s, 3H), 6.90 (s, 1H), 6.94 (d, 4H), 7.05 (m, 3H), 7.22 (d, 4H). 13C NMR (100 MHz, CDCl3): δ (ppm) 57.84, 116.14, 120.21, 121.85, 124.36, 131.51, 133.97, 142.65, 144.84, 152.23. FT-IR (KBr): 3033, 2950, 1523, 1454, 1256, 1064 cm−1. MS (EI, 70 eV): m/z 431 (M+), anal. calcd for C19H15NOBr2: C, 52.68; H, 3.47; N, 3.23%. Found: C, 52.57; H, 3.32; N, 3.24%.
4-Methoxy-N,N-bis(4-bromophenyl)aniline (3f). White solid. 1H NMR (400 MHz, CDCl3): δ (ppm) 3.75 (s, 3H), 6.90 (d, 2H), 6.96 (d, 4H), 7.08 (d, 2H), 7.39 (d, 4H). 13C NMR (100 MHz, CDCl3): δ (ppm) 57.98, 115.34, 126.34, 131.50, 133.73, 135.65, 145.67, 146.32, 150.07. FT-IR (KBr): 3056, 2948, 1584, 1457, 1273, 1075 cm−1. MS (EI, 70 eV): m/z 431 (M+), anal. calcd for C19H15NOBr2: C, 52.68; H, 3.47; N, 3.23%. Found: C, 52.54; H, 3.35; N, 3.19%.
4-Bromo-N,N-bis(4-bromophenyl)aniline (3g). Pale green solid. 1H NMR (400 MHz, CDCl3): δ (ppm) 6.97 (d, 6H), 7.08 (d, 6H). 13C NMR (100 MHz, CDCl3): δ (ppm) 115.23, 125.92, 132.43, 146.59. FT-IR (KBr): 3056, 1585, 1483, 1272, 1071 cm−1. MS (EI, 70 eV): m/z 481 (M+), anal. calcd for C18H12NBr3: C, 44.84; H, 2.49; N, 2.91%. Found: C, 44.32; H, 2.31; N, 2.89%.
4-Bromo-N,N-bis(phenyl)aniline (3h). White solid. 1H NMR (400 MHz, CDCl3): δ (ppm) 7.01 (d, 2H), 7.16 (m, 6H), 7.22 (d, 4H), 7.42 (d, 2H). 13C NMR (100 MHz, CDCl3): δ (ppm) 115.35, 120.64, 124.97, 127.79, 128.44, 136.21, 146.69, 147.74. FT-IR (KBr): 3085, 1590, 1483, 1273, 1075 cm−1.
4-Bromo-N,N-bis(4-methylphenyl)aniline (3i). Yellowish solid. 1H NMR (400 MHz, CDCl3): δ (ppm) 2.35 (s, 6H), 6.92 (d, 2H), 7.01 (d, 4H), 7.09 (d, 4H), 7.31 (d, 2H). 13C NMR (100 MHz, CDCl3): δ (ppm) 19.96, 113.07, 124.88, 126.34, 129.52, 132.65, 135.06, 145.84, 146.37. FT-IR (KBr): 3110, 2989, 1568, 1495, 1275, 1069 cm−1. MS (EI, 70 eV): m/z 350 (M+), anal. calcd for C20H18NBr: C, 68.20; H, 5.11; N, 3.98%. Found: C, 68.08; H, 4.96; N, 3.73%.
4-Bromo-N,N-bis(2-methoxyphenyl)aniline (3j). Yellowish solid. 1H NMR (400 MHz, CDCl3): δ (ppm) 3.64 (s, 6H), 7.01 (d, 2H), 7.12 (m, 4H), 7.21 (m, 4H), 7.31 (d, 2H). 13C NMR (100 MHz, CDCl3): δ (ppm) 61.54, 120.32, 122.64, 125.49, 129.18, 132.78, 135.63, 136.13, 145.22, 146.39, 150.79. FT-IR (KBr): 3051, 2964, 1598, 1422, 1265, 1049 cm−1. MS (EI, 70 eV): m/z 383 (M+), anal. calcd for C20H18NO2Br: C, 62.52; H, 4.69; N, 3.65%. Found: C, 62.43; H, 4.49; N, 3.51%.
4-Bromo-N,N-bis(3-methoxyphenyl)aniline (3k). Yellow solid. 1H NMR (400 MHz, CDCl3): δ (ppm) 3.75 (s, 6H), 6.98 (m, 4H), 7.02 (m, 3H), 7.09 (m, 3H), 7.24 (d, 2H). 13C NMR (100 MHz, CDCl3): δ (ppm) 61.89, 112.21, 119.82, 124.46, 125.97, 130.52, 132.79, 134.23, 135.17, 145.91, 148.64, 155.01. FT-IR (KBr): 3064, 2922, 1581, 1462, 1272, 1081 cm−1. MS (EI, 70 eV): m/z 382 (M+), anal. calcd for C20H18NO2Br: C, 62.52; H, 4.69; N, 3.65%. Found: C, 62.41; H, 4.56; N, 3.48%.
4-Bromo-N,N-bis(4-methoxyphenyl)aniline (3l). Yellow solid. 1H NMR (400 MHz, CDCl3): δ (ppm) 3.81 (s, 6H), 6.98 (d, 2H), 7.21 (d, 4H), 7.28 (d, 2H), 7.43 (d, 4H). 13C NMR (100 MHz, CDCl3): δ (ppm) 59.36, 115.07, 124.48, 133.97, 134.58, 138.27, 146.19, 147.41, 152.63. FT-IR (KBr): 3088, 2921, 1579, 1475, 1277, 1073 cm−1. MS (EI, 70 eV): m/z 383 (M+), anal. calcd for C20H18NO2Br: C, 62.52; H, 4.69; N, 3.65%. Found: C, 62.35; H, 4.51; N, 3.49%.
4-Methyl-N,N-bis(4-nitrophenyl)aniline (3m). Yellow solid. 1H NMR (400 MHz, DMSO-d6): δ (ppm) 2.31 (s, 3H), 7.16 (d, 2H), 7.18 (d, 4H), 7.33 (d, 2H), 8.05 (d, 4H). 13C NMR (100 MHz, DMSO-d6): δ (ppm) 21.36, 122.34, 125.36, 126.27, 130.58, 132.60, 139.04, 147.23, 147.69. FT-IR (KBr): 3063, 3043, 1548, 1508, 1452, 1335, 1273 cm−1. MS (EI, 70 eV): m/z 348 (M+), anal. calcd for C19H15N3O4: C, 65.32; H, 4.33; N, 12.03%. Found: C, 65.06; H, 4.12; N, 12.15%.
4-Methyl-N,N-bis(4-carbomethoxy)aniline (3n). Yellow solid. 1H NMR (400 MHz, DMSO-d6): δ (ppm) 2.35 (s, 3H), 3.61 (s, 6H), 7.06 (d, 2H), 7.21 (d, 4H), 7.32 (d, 2H), 7.94 (d, 4H). 13C NMR (100 MHz, DMSO-d6): δ (ppm) 20.05, 60.21, 121.43, 126.54, 127.78, 130.08, 132.52, 138.41, 147.35, 147.74, 167.49. FT-IR (KBr): 3061, 3045, 1735, 1542, 1503, 1459, 1332, 1271 cm−1. MS (EI, 70 eV): m/z 375 (M+), anal. calcd for C23H21NO4: C, 73.60; H, 5.60; N, 3.73%. Found: C, 73.35; H, 5.46; N, 3.75%.
(4,6-Dimethyl-pyrimidin-2-yl)-diphenyl-amine (3o). White solid; 1H NMR (400 MHz, DMSO-d6): δ (ppm) 2.15 (s, 6H), 6.39 (s, 1H), 7.05 (m, 2H), 7.14 (m, 8H). 13C NMR (100 MHz, DMSO-d6): δ (ppm) 24.84, 115.03, 125.45, 128.71, 129.59, 145.89, 163.01, 167.75. FT-IR (KBr): 3063, 3042, 1545, 1451, 1332, 1275 cm−1. MS (EI, 70 eV): m/z 275 (M+), anal. calcd for C18H17N3: C, 78.55; H, 6.18; N, 15.27%. Found: C, 78.36; H, 6.09; N, 15.25%.
Acknowledgements
The authors are grateful to University of Kashan for supporting this work by Grant no.: 159196/XXI.
References
- G. Evano, N. Blanchard and M. Toumi, Chem. Rev., 2008, 108, 3054 CrossRef CAS PubMed.
- J. P. Corbet and G. Mignani, Chem. Rev., 2006, 106, 2651 CrossRef CAS PubMed.
- B. Schlummer and U. Scholz, Adv. Synth. Catal., 2004, 346, 1599 CrossRef CAS.
- Pigment Handbook, ed. P. A. Lewis, John Wiley & Sons, New York, 1988 Search PubMed.
- M. Takeuchi, M. Kobayashi, R. Shishikawa, T. Sakai, H. Nakamura and H. Konuma, Jpn. Kokai Tokkyo Koho, JP Pat. 61279061, 1986.
- K. Kaeriyama, M. Suda, M. Sato, Y. Osawa, M. Ishikawa and M. Kawai, Jpn. Kokai Tokkyo Koho, JP Pat. 63168974, 1988.
- Y. Nishikitani, M. Kobayashi, S. Uchida and T. Kubo, Electrochim. Acta, 2001, 46, 2035 CrossRef CAS.
- W. Shi, L. Wang, M. Umar, T. Awut, H. Mi, C. Tana and I. Nurullaa, Polym. Int., 2009, 58, 800 CrossRef CAS.
- J. P. Wolfe, H. Tomori, J. P. Sadighi, J. J. Yin and S. L. Buchwald, J. Org. Chem., 2000, 65, 1158 CrossRef CAS PubMed.
- J. F. Hartwig, Angew. Chem., Int. Ed. Engl., 1998, 37, 2046 CrossRef CAS.
- H. B. Goodbrand and N. X. Hu, J. Org. Chem., 1999, 64, 670 CrossRef CAS.
- J. Hassan, M. Sevignon, C. Gozzi, E. Schulz and M. Lemaire, Chem. Rev., 2002, 102, 1359 CrossRef CAS PubMed.
- M. P. Nandkumar, A. K. Ashutosh and V. C. Raghunath, J. Mol. Catal. A: Chem., 2004, 223, 45 CrossRef PubMed.
- F. Ullmann, Ber. Dtsch. Chem. Ges., 1903, 36, 2382 CrossRef.
- J. Hassan, M. Sevignon, C. Gozzi, C. Schulz and M. Lemaire, Chem. Rev., 2002, 102, 1359 CrossRef CAS PubMed.
- R. Frlan and D. Kikelj, Synthesis, 2006, 2271 CAS.
- R. A. Sheldon, Pure Appl. Chem., 2000, 72, 1233 CrossRef CAS.
- T. Punniyamurthy and L. Rout, Coord. Chem. Rev., 2008, 252, 134 CrossRef CAS PubMed.
- J. F. Hartwig, Angew. Chem., Int. Ed., 1998, 37, 2046 CrossRef CAS.
- V. Farina, Adv. Synth. Catal., 2004, 346, 1553 CrossRef CAS.
- M. H. Ali and S. L. Buchwald, J. Org. Chem., 2001, 66, 2560 CrossRef CAS PubMed.
- X. Huang, K. W. Anderson, D. Zim, L. Jiang, A. Klapars and S. L. Buchwald, J. Am. Chem. Soc., 2003, 125, 6653 CrossRef CAS PubMed.
- R. K. Gujadhur, C. G. Bates and D. Venkataraman, Org. Lett., 2001, 3, 4315 CrossRef CAS PubMed.
- H. Zhang, Q. Cai and D. Ma, J. Org. Chem., 2005, 70, 5164 CrossRef CAS PubMed.
- H. Rao, Y. Jin, H. Fu, Y. Jiang and Y. Zhao, Chem.–Eur. J., 2006, 12, 3636 CrossRef CAS PubMed.
- A. A. Kelkar, N. M. Patil and R. V. Chaudhari, Tetrahedron Lett., 2002, 43, 7143 CrossRef CAS.
- Y.-H. Liu, C. Chena and L.-M. Yang, Tetrahedron Lett., 2006, 47, 9275 CrossRef CAS PubMed.
- C. Qian, S. Xu, Q. Zong and D. Fang, Chin. J. Chem., 2012, 30, 1881 CrossRef CAS.
- R. Gujadhur, D. Venkataraman and J. T. Kintigh, Tetrahedron Lett., 2001, 42, 4791 CrossRef CAS.
- D. Wright, U. Gubler and W. E. Moerner, J. Phys. Chem. B, 2003, 107, 4732 CrossRef CAS.
- J. Safaei-Ghomi, Z. Akbarzadeh and A. Ziarati, RSC Adv., 2014, 4, 16385 RSC.
- D. Astruc, F. Lu and J. R. Aranzaes, Angew. Chem., Int. Ed., 2005, 44, 7852 CrossRef CAS PubMed.
- L. D. Pachon, M. B. Thathagar, F. Hartl and G. Rothenberg, Phys. Chem. Chem. Phys., 2006, 8, 151 RSC.
- F. Z. Su, Y. M. Liu, L. C. Wang, Y. Cao, H. Y. He and K. N. Fan, Angew. Chem., Int. Ed., 2008, 47, 334 CrossRef CAS PubMed.
- H. Adkins and R. Connor, J. Am. Chem. Soc., 1931, 53, 1091 CrossRef CAS.
- A. M. Kawamoto, L. C. Pardini and L. C. Rezende, Aerosp. Sci. Technol., 2004, 8, 591 CrossRef CAS PubMed.
- P. S. Sathiskumar, C. R. Thomas and G. Madras, Ind. Eng. Chem. Res., 2012, 51, 10108 CrossRef CAS.
- S. S. Acharyya, S. Ghosh and R. Bal, ACS Sustainable Chem. Eng., 2014, 2, 584 CrossRef CAS.
- D. Liu, D. Zemlyanov, T. Wu, R. J. Lobo-Lapidus, J. A. Dumesic, J. T. Miller and C. L. Marshall, J. Catal., 2013, 299, 336 CrossRef CAS PubMed.
- L. B. Madhavi, V. Sadasivam and B. Sivasankar, Catal. Commun., 2007, 8, 1070 CrossRef PubMed.
- L. Ping, Z. Zhen, X. Hongbin and Z. Yi, Thermochim. Acta, 2012, 544, 71 CrossRef PubMed.
- W. Shi, S. Fan, F. Huang, W. Yang, R. Liu and Y. Cao, J. Mater. Chem., 2006, 16, 2387 RSC.
- Q. Wang, Z. He, A. Wild, H. Wu, Y. Cao, U. S. Schubert, C. H. Chui and W. Y. Wong, Chem.–Asian J., 2011, 6, 1766 CrossRef CAS PubMed.
- C. Quinton, V. Alain-Rizzo, C. Dumas-Verdes, G. Clavier, F. Miomandre and P. Audebert, Eur. J. Org. Chem., 2012, 7, 1394 CrossRef.
- J. H. Cho, Y. S. Ryu, S. H. Oh, J. K. Kwon and E. K. Yum, Bull. Korean Chem. Soc., 2011, 32, 2461 CrossRef CAS.
- J. H. Seok, S. H. Park, M. E. El-Khouly, Y. Araki, O. Ito and K. Y. Kay, J. Organomet. Chem., 2009, 694, 1818 CrossRef CAS PubMed.
- M. Planells, N. Robertson, A. Abate, D. J Hollman, H. J. Snaith, S. D Stranks, V. Bharti, S. Chand, J. Gaur and D. Mohanty, J. Mater. Chem. A, 2013, 1, 6949 CAS.
- K.-L. Wang, S.-T. Huang, L.-G. Hsieh and G.-S. Huang, Polymer, 2008, 49, 4087 CrossRef CAS PubMed.
- C. Lambert, J. Schelter, T. Fiebig, D. Mank and A. Trifonov, J. Am. Chem. Soc., 2005, 127, 10600 CrossRef CAS PubMed.
- Y. Zhao, Y. Wang, H. Sun, L. Li and H. Zhang, Chem. Commun., 2007, 3186 RSC.
|
This journal is © The Royal Society of Chemistry 2015 |