DOI:
10.1039/C4RA16342G
(Paper)
RSC Adv., 2015,
5, 12094-12099
Coumarin-based supramolecular gelator: a case of selective detection of F− and HP2O73−†
Received
13th December 2014
, Accepted 9th January 2015
First published on 9th January 2015
Abstract
Coumarin appended 1,2,3-triazole coupled cholesterol 1 which acts as a small molecular gelator has been designed and synthesized. Compound 1 has been noted to form gel from CHCl3–petroleum ether (1
:
1, v/v). The stable gel is anion responsive. The gel state is transformed into the sol state selectively in the presence of F− and hydrogen pyrophosphate and thus validates their visual sensing over a series of other anions. Fluorescence study in CH3CN containing 0.5% DMSO also reveals substantial change in emission of 1 upon addition of both F− and hydrogen pyrophosphate and distinguishes them from other anions studied.
Introduction
The design and synthesis of a low molecular weight organic compound which is capable of forming a supramolecular gel in a solvent and subsequently transformed to the sol state in the presence of ionic analytes has recently been the subject of increasing attention in supramolecular chemistry.1 Supramolecular gels are dimensionally controlled assemblies of low-molecular-weight molecules held together by noncovalent interactions, such as hydrogen bonding, metal coordination, van der Waals interaction, and π–π stacking.2 Perturbation of these weak interactions using various stimuli such as light,3 redox,4 pH5 and different ions6 results in the destruction of supramolecular gel. These are called stimuli responsive gels. The stimuli responsive nature of the supramolecular gels is now-a-days highly attractive for the development of sensor devices. Many research groups in this domain have shown interest, especially, in the construction of anion-responsive supramolecular gels.6a–f,7 Of the different anion responsive gels, fluoride and pyrophosphate-sensing gels have received a great deal of attention. Fluoride is an important anion which is involved in preventing dental caries and in medical treatment for osteoporosis.8 Similarly, pyrophosphate is critically important due to its crucial role in biological system.9
Careful scrutiny of the literature reveals that there are plenty number of fluororeceptors which recognize fluoride10 and pyrophosphate anions in solution.11 In relation to this, supramolecular gelators that are capable of detecting fluoride6c,12 and some phosphate derivatives13 are less in number in the literature. To the best of our knowledge, pyrophosphate sensing gelators are unknown. In this account, we report a simple new architecture 1 (Fig. 1), which forms gel from CHCl3–petroleum ether (1
:
1, v/v) mixture solvent. The gel is anion responsive. It is broken selectively in the presence of F− and hydrogen pyrophosphate (HP2O73−) and validates their visual sensing over a series of other anions. Fluorescence study in CH3CN containing 0.5% DMSO also reveals substantial change in emission of 1 upon addition of both F− and HP2O73−.
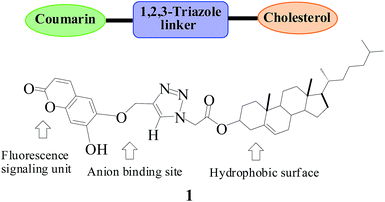 |
| Fig. 1 Structure of compound 1. | |
Results and discussion
Compound 1 was achieved according to the Scheme 1. Cholesterol was initially converted to the chloride 2 (ref. 6c) which on reaction with NaN3 in CH3CN afforded the cholesterol azide 3. Pursuance of click reaction of the azide 3 with propergyl alcohol gave 1,2,3-triazole linked cholesterol alcohol 4. Then the hydroxyl group in 4 was sequentially reacted with mesyl chloride and LiBr to give bromide derivative 6. Subsequent reaction of 6,7-dihydroxycoumarin with bromide 6 in the presence of Cs2CO3 in CH3CN under refluxing condition introduced the compound 1 in appreciable yield.
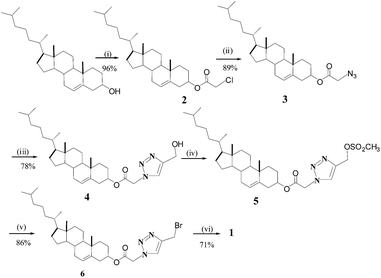 |
| Scheme 1 (i) Chloroacetyl chloride, pyridine, dry CH2Cl2, rt, 10 h; (ii) CH3CN, NaN3, reflux, 5 h; (iii) propergyl alcohol, CuSO4, Cu turning, EtOH–H2O, reflux, 90 °C, 6 h; (iv) dry CH2Cl2, methanesulfonyl chloride, NEt3, rt, 30 min; (v) dry THF, LiBr, stirring, 8 h; (vi) 6,7-dihydroxycoumarin, dry CH3CN, Cs2CO3, reflux, 36 h. | |
Compound 1 consists of different components of which the anion binding site is comprised of phenolic OH and triazole C–H as hydrogen bond donors (Fig. 1). The cholesterol group has been added adjacent to the binding site for its potential for strong hydrophobic interactions.14 Coumarin moiety has been introduced as the fluorescent signalling unit to execute the sensing behavior of compound 1. It is noteworthy that cholesteryl motif bearing compound 1 with such an arrangement is observed to form brown colored gel from chloroform–petroleum ether (1
:
1 v/v) mixture solvent. A range of other solvents or solvent mixtures as summarized in Table 1 were unable to bring gelation of 1. The gel was stable at room temperature and transformed into the sol state at 48 °C (Tgel). Upon cooling the sol is slowly transformed into the gel state and thereby indicated that the gel forming and gel collapsing are thermo reversible. Fig. 2 shows the scanning electron microscopy (SEM) image of the xero gel of 1. Three dimensional network shows fibrous aggregate.
Table 1 Results of gelation test for 1
Solvents |
Resulta (1) |
S = solution; G = transparent gel (minimum gelation concentration); I = insoluble; P = precipitation. |
CHCl3 |
S |
2% CH3OH in CHCl3 |
S |
CHCl3 : petroleum ether (1 : 1, v/v) |
G (18 mg mL−1) |
CH3COCH3 |
S |
DMF |
S |
DMF : H2O (1 : 1, v/v) |
P |
CH3CN |
I |
CH3CN : CHCl3 (1 : 1, v/v) |
S |
CH3OH |
I |
CH3OH : H2O (1 : 1, v/v) |
I |
DMSO |
S |
1% DMSO in CH3CN |
S |
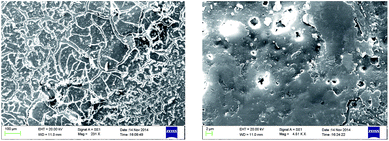 |
| Fig. 2 SEM images of xerogel of 1 prepared from CHCl3–petroleum ether (1 : 1, v/v): (a) in 100 μm and (b) 2 μm scales. | |
To our belief, out of several possibilities, the phenolic OH and the triazole ring nitrogen in 1 may be intimately involved in hydrogen bonding to make an intermolecular association according to the mode shown in Fig. 3. This gives a network into the solution. The hydrophobic–hydrophobic interaction between the cholesterol units and weak π-stacking interaction of coumarins presumably stabilize the hydrogen bonded network. A shouldering at 378 nm in UV-vis spectrum of 1 in the gel state with respect to its sol state is likely to be due to aggregate stacking of the gel (Fig. 4a). In fluorescence, red-shifted emission at 446 nm for the gel state is also in accordance with the proposed π-stacking aggregation (Fig. 4b). In FTIR, while the stretching for –OH in the amorphous state of 1 was noted at 3391 cm−1 as broad signal, in the gel state it appeared at 3393 cm−1 (Fig. 5). This small change in stretching frequency for –OH group is presumably due to its participation in hydrogen bonding. On the other hand, stretching frequency for ester carbonyls of both the coumarin and cholesteryl parts merged together and appeared at 1725 cm−1 as broad signal. In the gel state, this signal was reduced to the lower frequency (1714 cm−1). This lowering of stretching for ester carbonyl in 1 is the consequence of hydrogen bonding.
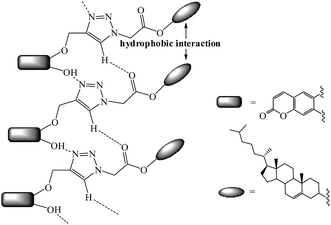 |
| Fig. 3 Probable mode of interaction of 1. | |
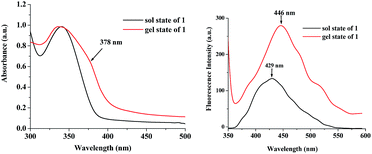 |
| Fig. 4 Comparison of (a) UV-vis and (b) fluorescence spectra of 1 in the sol and gel states. | |
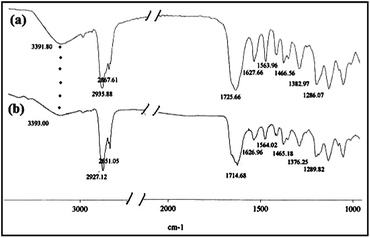 |
| Fig. 5 Partial FTIR spectra of 1 in its (a) amorphous and (b) gel states. | |
In an effort to understand the stimuli responsive nature of the gel, we added different anions as their tetrabutylammonium salts to the gel state of 1. Among the anions taken in the study, only addition of F− and HP2O73− led to the rapid transformation of the gel into solution (Fig. 6). Fluoride being strongly basic deprotonates the phenolic –OH and disrupts the self-assembly of 1. This deprotonation mediated gel disruption was further supported by addition of tetrabutylammonium hydroxide. Similarly, HP2O73− rather than H2PO4− strongly interacts with the triazole coupled phenolic OH motif of 1 via hydrogen bond formation and destroys the network through deprotonation of the phenolic –OH. It is mentionable that hydrogen bonding affinities of triazole motif for HP2O73− is well established.15
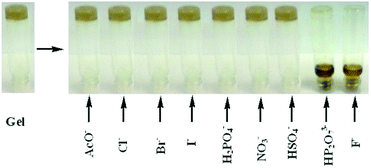 |
| Fig. 6 Photograph showing the changes in the CHCl3–petroleum ether (1 : 1, v/v) gel of 1 (18 mg mL−1) after addition of 1 equiv. amount of different anions (c = 3.5 × 10−2 M). | |
Fluoride induced broken gel was recovered on keeping the sol after addition of either trifluoroacetic acid (TFA) or BF3. Importantly, while TFA recovered the gel state after ∼1 h, BF3 was able to gelate the sol after 1.5 h (Fig. 7). We believe that regeneration of phenolic OH either by protonation using TFA or by scavenging F− ions from the mixture as BF4− enables the compound 1 to attain its original structural feature for which gelation takes place. Likewise, HP2O73− induced broken gel was recovered upon addition of TFA, shown in Fig. 7a. During recovery, the color of the gel became light brown rather than deep brown.
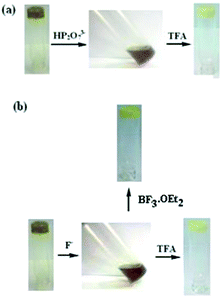 |
| Fig. 7 Phase changes of the gel of 1 [18 mg mL−1 in CHCl3–pet ether (1 : 1, v/v)] on successive addition of (a) HP2O73− (c = 3.5 × 10−2 M) and TFA (The gel to sol conversion was completed within 15 min and reappeared upon addition of TFA after 1 h); (b) TBAF (c = 3.5 × 10−2 M) and TFA (The gel to sol conversion was completed within 10 min and reappeared upon addition of TFA after 1.5 h). | |
As the compound 1 contains coumarin motif, a well defined fluorophore with good quantum yield, we further investigated its interaction with the said anions in CH3CN containing 0.5% DMSO (used for homogeneity of the solution). In fluorescence, while compound 1 showed measurable interaction with F− and HP2O73−, other anions perturbed the emission weakly. Fig. 8 highlights the change in fluorescence ratio of 1 at 428 nm. As can be seen from Fig. 9, the emission at 428 nm is significantly quenched with a red shift of 24 nm upon interaction. This indicated strong interaction of HP2O73− and F− at the binding site of 1 involving the phenolic OH and triazole C–H as the hydrogen bond donors. In presence of both F− and HP2O73−, blue colored solution of 1 became light greenish blue when looked in under UV light (insets of Fig. 9). During interaction with these anions, the phenolic OH of 1 was deprotonated rather than participation in hydrogen bonding when they were present in excess in solution. In 1H NMR, the disappearance of the signal at 6.20 ppm for –OH in the presence of equivalent amount of F− and HP2O73− supported the deprotonation phenomena (Fig. 10). On deprotonation of phenolic OH, the coumarin ring protons He, Hb moved upfield significantly. On the other hand, downfield chemical shift of the proton Hg in triazole ring (Δδ for F− = 0.2 ppm and HP2O73− = 0.15 ppm) indicated its involvement in hydrogen bonding with the anions.
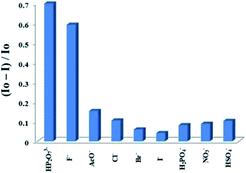 |
| Fig. 8 Fluorescence ratio [I0 − I/I0] of 1 (c = 1.94 × 10−5 M) at 428 nm upon addition of 10 equiv. amounts of a particular anion (c = 7.76 × 10−4 M) in CH3CN containing 0.5% DMSO. | |
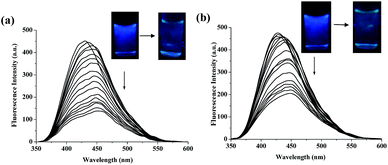 |
| Fig. 9 Change in emission of 1 (c = 1.94 × 10−5 M) upon addition of 10 equiv. amounts of (a) HP2O73−, (b) F− (c = 7.76 × 10−4 M) in CH3CN containing 0.5% DMSO (insets show the change in colour of the solutions under UV radiation). | |
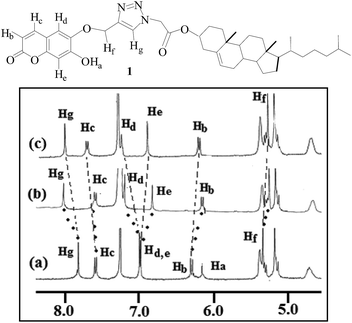 |
| Fig. 10 Partial 1H NMR (400 MHz, CDCl3) of (a) compound 1 (c = 6.54 × 10−3 M), (b) 1 with TBAF (1 : 1) and (c) 1 with TBA-hydrogen pyrophosphate (1 : 1). | |
In UV-vis titration, while in presence of HP2O73− and F− ions the intensity of the peak at 340 nm was decreased, a new peak at 412 nm appeared with significant intensity (Fig. 11). A similar ratiometric change was observed in the presence of tetrabutylammonium hydroxide (ESI†). The peak at 412 nm is due to the formation of phenoxide ion that exerts auxochromic effect in UV-vis spectra. This was not observed for other anions taken in the study (ESI†). Acetate ion being less basic responded weakly in deprotonation as supported by minor change in absorbance of 1 at 412 nm in the presence of AcO− ion (ESI†).
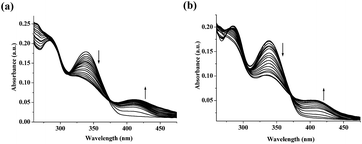 |
| Fig. 11 Change in absorbance of 1 (c = 1.94 × 10−5 M) upon addition of 10 equiv. amounts of (a) HP2O73−and (b) F− in CH3CN containing 0.5% DMSO. | |
To realize the strength of interaction, the emission titration data for HP2O73− and F− with 1 were analysed. The binding constant values16 were observed to be (1.78 ± 0.19) × 104 M−1 and (8.49 ± 1.11) × 103 M−1 for HP2O73− and F−, respectively with 1
:
1 stoichiometries.17 Due to small change in emission the binding constant values for other anions were not determined. In the process, the detection limits18 for F− and HP2O73− were determined to be 1.06 × 10−4 M and 7.51 × 10−5 M, respectively (ESI†). Interference study as shown in Fig. 6S (ESI†) corroborates that F− and HP2O73− are mutually interfering. Other anions in the study were non interfering.
Conclusion
In conclusion, coumarin-based fluorescent organogelator 1 has been designed and synthesized. The gelator forms stable gel from CHCl3–petroleum ether (1
:
1, v/v). Cooperative hydrogen bonding between phenolic –OH and 1,2,3-triazole ring as well as hydrophobic–hydrophobic interaction of the cholesteryl groups in 1 play crucial role in the formation of organogel. The stable gel is anion responsive. The gel phase is rapidly transformed into the sol state selectively in the presence of F− and HP2O73− and thus validates their visual sensing over a series of other anions. Fluorescence study in solution also distinguishes these two anions from the other anions by showing considerable change in emission. In CH3CN containing 0.5% DMSO, substantial quenching of emission of 1 in presence of F− is noticeable and useful in its distinction from other halides. In a similar way greater quenching of emission in presence of HP2O73−ion is mentionable for its diagnosis from H2PO4− ion. Thus the compound 1 in this account is undoubtedly a new system that detects F− and HP2O73− fluorimetrically as well as gel breaking process under appropriate conditions. Further insight along this direction is underway in our laboratory.
Experimental
Syntheses
Chloro-acetic acid 17-(1,5-dimethyl-hexyl)-10,13-dimethyl-2,3,4,7,8,9,10,11,12,13,14,15,16,17-tetradecahydro-1H-cyclopenta[a]phenanthren-3-yl ester (2)6c. To a stirred solution of cholesterol (0.5 g, 1.29 mmol) in 20 mL dry CH2Cl2 was added chloroacetyl chloride (0.16 mL, 1.93 mmol) and pyridine (0.05 mL, 0.65 mmol) under nitrogenous atmosphere. The mixture was allowed to stir for 10 h at room temperature. After completion of reaction, the solvent was evaporated and the crude was extracted with CHCl3 (3 × 30 mL). The organic layer was washed several times with water and separated and dried over Na2SO4. Evaporation of the solvent gave white solid compound. Recrystallization from petroleum ether afforded pure product 2 (0.58 g, yield 96%), mp 148 °C. 1H NMR (400 MHz, CDCl3): δ 5.37 (m, 1H), 4.72 (m, 1H), 4.03 (s, 2H), 2.36 (m, 2H), 2.02–0.85 (m, 38H), 0.67 (s, 3H); FTIR (KBr, cm−1): 2939, 2907, 2821, 1753, 1620, 1195.
Azido-acetic acid 17-(1,5-dimethyl-hexyl)-10,13-dimethyl-2,3,4,7,8,9,10,11,12,13,14,15,16,17-tetradecahydro-1H-cyclopenta[a]phenanthren-3-yl ester (3). To a stirred solution of compound 2 (0.5 g, 1.08 mmol) in CH3CN (20 mL) NaN3 was added (0.11 g, 1.6 mmol) and the reaction mixture was refluxed for 5 h. The progress of reaction was monitored by TLC. After the total consumption of the halide, solvent was evaporated off and water was added. The reaction mixture was extracted with CHCl3. Evaporation of the solvent gave the crude azide product 3 (0.45 g, yield 89%, mp 116 °C), which after recrystallization from diethyl ether was directly used in the next step. FTIR (KBr, cm−1): 2938, 2107, 1747, 1213.
(4-Hydroxymethyl-[1,2,3]triazol-1-yl)-acetic acid 17-(1,5-dimethyl-hexyl)-10,13-dimethyl 2,3,4,7,8,9,10,11,12,13,14,15,16,17-tetradecahydro-1H-cyclopenta[a]phenanthren-3-yl ester (4). Compound 3 (0.6 g, 1.28 mmol) was dissolved in ethanol (40 mL) and propergyl alcohol (0.12 mL, 1.92 mmol) was added to this solution followed by addition of 4 mL of saturated CuSO4 solution and Cu turning (0.75 mg). Then the reaction mixture was refluxed for 6 h at 90 °C. After completion, reaction mixture was filtered through celite bed. The filtrate was evaporated off and the crude mass was extracted with 2% CH3OH in CHCl3 and dried over anhydrous Na2SO4. Evaporation of the solvent gave the crude product which was purified by column chromatography using 70% ethyl acetate in petroleum ether to afford compound 4 (0.53 g, yield 78%), mp 178 °C. 1H NMR (400 MHz, CDCl3): δ 7.68 (s, 1H), 5.37 (d, 1H, J = 4 Hz), 5.14 (s, 2H), 4.81 (s, 2H), 4.70 (m, 1H), 2.36 (d, 2H, J = 8 Hz), 1.99–0.85 (m, 38H), 0.67 (s, 3H) (signal for –OH is not found due to broadening); 13C NMR (100 MHz, CDCl3): δ 165.7, 148.2, 138.9, 123.3, 123.2, 76.5, 56.6, 56.4, 56.1, 51.0, 49.9, 42.3, 39.6, 39.5, 37.8, 36.8, 36.5, 36.1, 35.8, 31.88, 31.80, 28.2, 28.0, 27.6, 24.2, 23.8, 22.8, 22.5, 21.0, 19.2, 18.7, 11.8; FTIR (KBr, cm−1): 3487, 3401, 2960, 2939, 1749, 1727, 1468, 1230, 1212.
Compound 1. To a stirred solution of 4 (0.5 g, 0.95 mmol) in 20 mL dry CH2Cl2 was added methanesulfonyl chloride (0.11 mL, 1.42 mmol) and NEt3 (0.26 mL, 1.9 mmol) at 0 °C. The mixture was then allowed to stir for 30 min at room temperature. The progress of the reaction was monitored by TLC. After completion of reaction, solvent was evaporated off and the crude mass was extracted with CHCl3 to afford compound 5. Without characterization compound 5 was dissolved in dry THF (15 mL). To this solution LiBr (0.16 g, 1.9 mmol) was added and the mixture was stirred for 8 h at room temperature. After completion of the reaction, THF was evaporated off and the organic compound was extracted with CHCl3 (3 × 20 mL), dried over Na2SO4. Removal of CHCl3 gave solid product 6 (0.48 g, yield 86%) which was directly used in the next step without characterization.A mixture of 6,7-dihydroxycoumarin (0.1 g, 0.56 mmol) and Cs2CO3 (0.36 g, 1.12 mmol) was refluxed in dry CH3CN for 2 h and then compound 6 (0.49 g, 0.84 mmol) was added to it. The reaction mixture was then allowed to reflux for 36 h. Then the organic solvent was evaporated under reduced pressure and water was added to the crude mass. Then reaction mixture was extracted with 2% CH3OH in CHCl3. Evaporation of the solvent gave the crude product which was purified by column chromatography using CHCl3 to 1% CH3OH in CHCl3 as eluents to afford the pure compound 1 in 71% yield (0.0.27 g), mp 192 °C. 1H NMR (400 MHz, CDCl3): δ 7.87 (s, 1H), 7.60 (d, 1H, J = 8 Hz), 6.99 (s, 1H), 6.98 (s, 1H), 6.30 (d, 1H, J = 8 Hz), 6.20 (brs, 1H), 5.37–5.30 (m, 3H), 5.17 (s, 2H), 4.70 (brs, 1H), 2.36 (d, 2H, J = 8 Hz), 2.03–0.85 (m, 38H), 0.67 (s, 3H); 13C NMR (100 MHz, CDCl3): δ 165.7, 161.4, 149.2, 148.7, 144.9, 143.3, 142.4, 138.8, 124.9, 123.3, 113.8, 112.7, 112.1, 100.8, 62.8, 62.6, 56.6, 56.1, 51.0, 49.9, 42.2, 39.6, 39.5, 37.8, 36.8, 36.5, 36.1, 35.8, 31.8, 31.7, 28.2, 28.0, 27.6, 24.2, 23.8, 22.8, 22.5, 21.0, 19.2, 18.7, 11.8; FTIR (KBr, cm−1): 3391, 2935, 2867, 1725, 1627; HRMS (TOF MS ES+): required 685.4091 (M+), 708.3989 (M + Na+), found 685.3961 (M+), 708.3571 (M + Na+).
Acknowledgements
We thank DST, New Delhi, India for providing facilities in the university under DST-PURSE program. SP thanks CSIR, New Delhi, India for a fellowship.
References
-
(a) N. M. Sangeetha and U. Maitra, Chem. Soc. Rev., 2005, 34, 821 RSC;
(b) J. W. Steed, Chem. Soc. Rev., 2010, 39, 3686 RSC;
(c) G. O. Lloyd and J. W. Steed, Nat. Chem., 2009, 1, 437 CrossRef CAS PubMed;
(d) M.-O. M. Piepenbrock, N. Clarke and J. W. Steed, Langmuir, 2009, 25, 8451 CrossRef CAS;
(e) H. Maeda, Chem.–Eur. J., 2008, 14, 11274 CrossRef CAS PubMed;
(f) J. W. Steed, Chem. Soc. Rev., 2009, 38, 506 RSC;
(g) P. Terech and R. G. Weiss, Chem. Rev., 1997, 97, 3133 CrossRef CAS PubMed;
(h) G. Yu, X. Yan, C. Han and F. Huang, Chem. Soc. Rev., 2013, 42, 6697 RSC.
-
(a) J. H. van Esch and B. L. Feringa, Angew. Chem., Int. Ed., 2000, 39, 2263 CrossRef CAS;
(b) M. George and R. G. Weiss, Acc. Chem. Res., 2006, 39, 489 CrossRef CAS PubMed;
(c) H. Danjo, K. Hirata, S. Yoshigai, I. Azumaya and K. Yamaguchi, J. Am. Chem. Soc., 2009, 131, 1638 CrossRef CAS PubMed;
(d) A. Ajayaghosh and V. K. Praveen, Acc. Chem. Res., 2007, 40, 644 CrossRef CAS PubMed;
(e) N. M. Sangeetha and U. Maitra, Chem. Soc. Rev., 2005, 34, 821 RSC;
(f) I. Hwang, W. S. Jeon, H.-J. Kim, D. Kim, H. Kim, N. Selvapalam, N. Fujita, S. Shinkai and K. Kim, Angew. Chem., Int. Ed., 2007, 46, 210 CrossRef CAS PubMed;
(g) H. Danjo, K. Hirata, S. Yoshigai, I. Azumaya and K. Yamaguchi, J. Am. Chem. Soc., 2009, 131, 1638 CrossRef CAS PubMed.
-
(a) Y. Huang, Z. Qiu, Y. Xu, J. Shi, H. Lina and Y. Zhang, Org. Biomol. Chem., 2011, 9, 2149 RSC;
(b) R. Yang, S. Peng, W. Wan and T. C. Hughes, J. Mater. Chem. C, 2014, 2, 9122 RSC;
(c) M. J. Clemente, R. M. Tejedor, P. Romero, J. Fitremann and L. Oriol, RSC Adv., 2012, 2, 11419 RSC;
(d) P. Xing, X. Chu, M. Ma, S. Li and A. Hao, Phys. Chem. Chem. Phys., 2014, 16, 8346 RSC.
-
(a) X. Sui, X. Feng, M. A. Hempenius and G. Julius Vancso, J. Mater. Chem. B, 2013, 1, 1658 RSC;
(b) H. Svobodova, V. N. Oponen, E. Kolehmainen and E. Sievanen, RSC Adv., 2012, 2, 4985 RSC;
(c) Y. Gao, J. Lu, J. Wu, J. Hu and Y. Ju, RSC Adv., 2014, 4, 63539 RSC;
(d) B. Zoetebier, M. A. Hempenius and G. J. Vancso, Chem. Commun., 2015, 51, 636 RSC;
(e) T. He, K. Li, N. Wang, Y. X. Liao, X. Wang and X. Q. Yu, Soft Matter, 2014, 10, 3755 RSC.
-
(a) Y. Huang, Z. Qiu, Y. Xu, J. Shi, H. Lina and Y. Zhang, Org. Biomol. Chem., 2011, 9, 2149 RSC;
(b) R. Yang, S. Peng, W. Wan and T. C. Hughes, J. Mater. Chem. C, 2014, 2, 9122 RSC;
(c) S. C. Lange, J. Unsleber, P. Drücker, H. J. Galla, M. P. Wallera and B. J. Ravoo, Org. Biomol. Chem., 2015, 13, 561 RSC.
-
(a) H. Maeda, Chem.–Eur. J., 2008, 14, 11274 CrossRef CAS PubMed;
(b) J. W. Steed, Chem. Soc. Rev., 2010, 39, 3686 RSC;
(c) K. Ghosh and D. Kar, Org. Biomol. Chem., 2012, 10, 8800 RSC;
(d) M. Park, D. Jang, S. Y. Kim and J. I. Hong, New J. Chem., 2012, 36, 1145 RSC;
(e) P. A. Gale, N. Busschaert, C. J. E. Haynes, L. E. Karagiannidis and I. L. Kirby, Chem. Soc. Rev., 2014, 43, 205 RSC;
(f) K. Ghosh, D. Kar, S. Panja and S. Bhattacharya, RSC. Adv., 2014, 4, 3798 RSC;
(g) J. Feng and H. Zhang, Chem. Soc. Rev., 2013, 42, 387 RSC;
(h) Y. R. Liu, L. He, J. Zhang, X. Wang and C. Y. Su, Chem. Mater., 2009, 21, 557 CrossRef CAS;
(i) T. Ogawa, J. Yuasa and T. Kawai, Angew. Chem., Int. Ed., 2010, 49, 5110 CrossRef CAS PubMed.
-
(a) M.-O. M. Piepenbrock, G. O. Lioyd, N. Clarke and J. W. Steed, Chem. Rev., 2010, 110, 1960 CrossRef CAS PubMed;
(b) H. Maeda, Chem.–Eur. J., 2008, 14, 11274 CrossRef CAS PubMed.
-
(a) Y. Qu, J. L. Hua and H. Tian, Org. Lett., 2010, 12, 3320 CrossRef CAS PubMed;
(b) H. S. Horowitz, J. Publ. Health Dent., 2003, 63, 3 CrossRef PubMed;
(c) P. P. Singh, M. K. Barjatiya, S. Dhing, R. Bhatnagar, S. Kothari and V. Dhar, Urol. Res., 2001, 29, 238 CrossRef CAS;
(d) B. L. Riggs, Bone and Mineral Research, Annual 2, Elsevier, Amster-dam, 1984, pp. 366–393 Search PubMed;
(e) M. Kleerekoper, Endocrinol. Metab. Clin. North Am., 1998, 27, 441 CrossRef CAS.
-
(a) C. P. Mathews and K. E. van Hold, Biochemistry, The Benjamin/Cummings Publishing Company, Inc., Redwood City, CA, 1990 Search PubMed;
(b) M. Ronaghi, S. Karamohamed, B. Pettersson, M. Uhlen and P. Nyren, Anal. Biochem., 1996, 242, 84 CrossRef CAS PubMed;
(c) S. Xu, M. He, H. Yu, X. Cai, X. Tan, B. Lu and B. Shu, Anal. Biochem., 2001, 299, 188 CrossRef CAS PubMed;
(d) T. Tabary and L. Ju, J. Immunol. Methods, 1992, 156, 55 CrossRef CAS.
-
(a) S. Kondo and M. Sato, Tetrahedron, 2006, 62, 4844 CrossRef CAS PubMed;
(b) D. H. Lee, H. Y. Lee, K. H. Lee and J. Hong, Chem. Commun., 2001, 1188 RSC;
(c) A.-F. Li, J.-H. Wang, F. Wang and Y.-B. Jiang, Chem. Soc. Rev., 2010, 39, 3729 RSC;
(d) M. Cametti and K. Rissanen, Chem. Soc. Rev., 2013, 42, 2016 RSC and references cited therein;
(e) T. Mizuno, W.-H. Wei, L. R. Eller and J. L. Sessler, J. Am. Chem. Soc., 2002, 124, 1134 CrossRef CAS PubMed.
-
(a) S. K. Kim, D. H. Lee, J.-I. Hong and J. Yoon, Acc. Chem. Res., 2009, 42, 23 CrossRef CAS PubMed;
(b) C. Lincheneau, R. M. Duke and T. Gunnlaugsson, Org. Biomol. Chem., 2012, 10, 6069 RSC;
(c) M. Wenzel, J. R. Hiscock and P. A. Gale, Chem. Soc. Rev., 2012, 41, 480 RSC;
(d) M. J. Kim, K. M. K. Swamy, K. M. Lee, A. R. Jagdale, Y. Kim, S.-J. Kim, K. H. Yoo and J. Yoon, Chem. Commun., 2009, 7215 RSC;
(e) S. Y. Kim and J.-I. Hong, Tetrahedron Lett., 2009, 50, 1951 CrossRef CAS PubMed;
(f) D. Curiel, G. Sanchez, C. R. De Arellano, A. Tarraga and P. Molina, Org. Biomol. Chem., 2012, 10, 1896 RSC;
(g) K. Ghosh, A. R. Sarkar, A. Samadder and A. R. Khuda-Bukhsh, Org. Lett., 2012, 14, 4314 CrossRef CAS PubMed;
(h) A. E. Hargrove, S. Nieto, T. Zhang, J. L. Sessler and E. V. Anslyn, Chem. Rev., 2011, 111, 6603 CrossRef CAS PubMed;
(i) C. Yin, F. Huo and P. Yang, Sens. Actuators, B, 2005, 109, 291 CrossRef CAS PubMed;
(j) C. Yin, F. Gao, F. Huo and P. Yang, Chem. Commun., 2004, 934 RSC.
-
(a) M. Park, D. Jang, S. Y. Kim and J.-I. Hong, New J. Chem., 2012, 36, 1145 RSC;
(b) J. Krishnamurthi, T. Ono, S. Amemori, H. Komatsu, S. Shinkai and K. Sada, Chem. Commun., 2011, 47, 1571 RSC;
(c) J. Zhou and H. Li, ACS Appl. Mater. Interfaces, 2012, 4, 721 CrossRef CAS PubMed;
(d) J.-W. Liu, Y. Yang, C.-F. Chen and J.-T. Ma, Langmuir, 2010, 26, 9040 CrossRef CAS PubMed;
(e) T. H. Kim, M. S. Choi, B. H. Sohn, S. Y. Park, W. S. Lyoo and T. S. Lee, Chem. Commun., 2008, 20, 2364 RSC;
(f) K. Ghosh, D. Kar and S. Bhattacharya, Supramol. Chem., 2014, 26, 313 CrossRef CAS and references cited therein;
(g) H. Yang, T. Yi, Z. G. Zhou, J. C. Wu, M. Xu, F. Y. Li and C. H. Huang, Langmuir, 2007, 23, 8224 CrossRef CAS PubMed;
(h) T. H. Kim, N. Y. Kwon and T. S. Lee, Tetrahedron Lett., 2010, 51, 5596 CrossRef CAS PubMed;
(i) M. Yamanaka, T. Nakamura, T. Nakagawa and H. Itagaki, Tetrahedron Lett., 2007, 48, 8990 CrossRef CAS PubMed.
-
(a) J.-S. Shen, Q.-G. Cai, Y.-B. Jiang and H.-W. Zhang, Chem. Commun., 2010, 46, 6786 RSC;
(b) K. Ghosh, A. R. Sarkar and A. P. Chattopadhyay, Eur. J. Org. Chem., 2012, 1311 CrossRef CAS;
(c) S. Yamaguchi, I. Yoshimura, T. Kohira, S.-I. Tamaru and I. Hamachi, J. Am. Chem. Soc., 2005, 127, 11835 CrossRef CAS PubMed.
- M. Zinic, F. Vogtle and F. Fages, Top. Curr. Chem., 2005, 256, 39 CrossRef CAS.
- Y. H. Lau, P. J. Rutledge, M. Watkinson and M. H. Todd, Chem. Soc. Rev., 2011, 40, 2848 RSC.
- P. T. Chou, G. R. Wu, C. Y. Wei, C. C. Cheng, C. P. Chang and F. T. Hung, J. Phys. Chem. B, 2000, 104, 7818 CrossRef CAS.
- P. Job, Ann. Chim., 1928, 9, 113 CAS.
- A. Caballero, R. Martinez, V. Lloveras, I. Ratera, J. Vidal-Gancedo, K. Wurst, A. Tarraga, P. Molina and J. Vaciana, J. Am. Chem. Soc., 2005, 127, 15666 CrossRef CAS PubMed.
Footnote |
† Electronic supplementary information (ESI) available: Figures showing the change in fluorescence and UV-vis titrations of 1 with various anions, Job plots, binding constant and detection limit plots, spectral data. See DOI: 10.1039/c4ra16342g |
|
This journal is © The Royal Society of Chemistry 2015 |