DOI:
10.1039/C4RA15710A
(Review Article)
RSC Adv., 2015,
5, 15233-15266
Pyrrole: a resourceful small molecule in key medicinal hetero-aromatics
Received
3rd December 2014
, Accepted 22nd January 2015
First published on 23rd January 2015
Abstract
Pyrrole is widely known as a biologically active scaffold which possesses a diverse nature of activities. The combination of different pharmacophores in a pyrrole ring system has led to the formation of more active compounds. Pyrrole containing analogs are considered as a potential source of biologically active compounds that contains a significant set of advantageous properties and can be found in many natural products. The marketed drugs containing a pyrrole ring system are known to have many biological properties such as antipsychotic, β-adrenergic antagonist, anxiolytic, anticancer (leukemia, lymphoma and myelofibrosis etc.), antibacterial, antifungal, antiprotozoal, antimalarial and many more. Due to the diversity of these analogs in the therapeutic response profile, many researchers have been working to explore this skeleton to its maximum potential against several diseases or disorders. In this review, attempts have been made to disclose various tactical approaches to synthesize pyrrole and pyrrole containing analogs. The structure–activity relationship studies have been discussed along with their therapeutic applications which have been reported during last decade. Some molecules as the main components of the market and clinical trials have also been discussed.
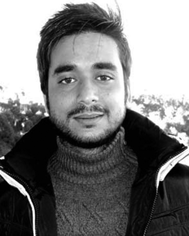 Varun Bhardwaj | Varun Bhardwaj received his bachelor's degree in pharmacy in 2008 and earned a master's degree in pharmacy with specialization in pharmaceutical chemistry in 2010 under the supervision of Prof. Malleshappa N. Noolvi. In early 2011, he joined as JRF and PhD fellow at JUIT, Waknaghat, India in a DST funded project and completed his PhD in 2014 under the supervision of Dr Poonam Sharma and Dr S. Chauhan. In his short research career, he has contributed in two government funded projects. His research interest includes design and synthesis of bioactive heterocycles, physicochemical interactions, nano/microparticulates, topical formulations especially against microbial infections. |
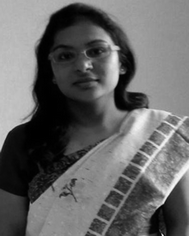 Divya Gumber | Divya Gumber is currently working as a project fellow and PhD fellow in department of pharmaceutical chemistry at Banasthali Vidyapith, Rajasthan. She holds a scholarship awarded by UGC, New Delhi. She has also worked at Discovery Lab, Organic and Biomolecular Chemistry Division, CSIR-Indian Institute of Chemical Technology, Hyderabad during her Post Graduation research project. She is an active researcher in area of synthetic medicinal and organic chemistry. |
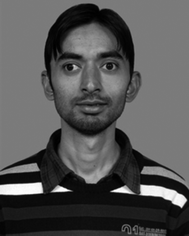 Vikrant Abbot | Vikrant Abbot obtained his Bachelor's degree in Pharmacy from Punjab Technical University in 2005–2009. He started his professional career working as Production Chemist at Comed Pharmaceuticals Ltd. Baddi for 2.5 years. Thereafter, he got the opportunity to work for Sun Pharmaceuticals Ltd. Jammu, as Junior Officer. Thereafter, he completed his Master's degree in medicinal chemistry from JUIT, Waknaghat in 2014. His present research interests include design and synthesis of small molecules with anticancer and antimicrobial profile. |
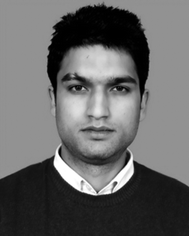 Saurabh Dhiman | Saurabh Dhiman was born in 1990 in Himachal Pradesh, India. He obtained his Bachelor degree from JUIT, waknaghat in 2012. He continued his postgraduate studies with specialization in medicinal chemistry from the same university and worked in the field of hybrid compounds as anticancer and antimicrobial agents. |
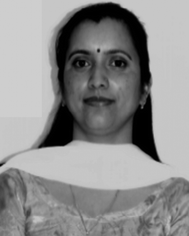 Poonam Sharma | Poonam Sharma obtained her Master's of Philosophy in 2003 with a gold medal and completed her PhD in chemistry in 2006 from Himachal Pradesh University (India). She was awarded with UGC project fellowship for her PhD research. She also got awarded with Fast Track Young Scientist research project by DST in 2010. Presently, she is working as Assistant professor in Jaypee University of Information Technology, Waknaghat, Solan (India). Her research revolves around physicochemical drug interactions, thermodynamics, heterocyclic bioactive analogs, topical drug delivery. |
1. Introduction
Heterocyclic compounds are referred to those cyclic compounds that contain at least two different elements as ‘ring member’ atoms. Heterocyclic compounds may be organic or inorganic, containing one carbon atom, and one or more atoms of elements other than carbon, such as sulphur, oxygen, nitrogen etc. within the ring structure. Thus, the non-carbon elements that replace the carbon atoms in a chemical structure are commonly termed as heteroatoms. Simple N-heterocycles have received considerable attention because of their important biological properties and their role as pharmacophores.1 The synthesis, reactions, and biological activities of pyrrole derivatives stand as an area of research in heteroaromatic chemistry, and this fundamental construction unit appears in a large number of pharmaceutical agents and natural products.2 In addition, biologically pyrroles could construct the structure of porphyrin rings, which act as a key moiety in chlorophyll, heme, vitamin B12, or bile pigments.3 Pyrrole is a colorless volatile liquid that darkens readily upon exposure to air and polymerizes in light. Pyrroles have low basicity than amines and other aromatic compounds like pyridines. This decreased basicity is due to the delocalization of the lone pair of electrons of the nitrogen atom in the aromatic ring. Pyrrole is a very weak base with a pKa of about −3.8 and its protonation results in the loss of aromatic property. Both –NH– and –CH– protons of pyrrole are moderately acidic and can be deprotonated with strong bases rendering the pyrrole nucleophilic. The resonance contributors of pyrrole provide insight to the reactivity of the compound. In simple terms, pyrrole is an aromatic five membered ring with the formula C4H5N, as shown below;
Pyrrole and its derivatives are ever present in nature. Pyrrole subunit has diverse applications in therapeutically active compounds including fungicides, antibiotics, anti-inflammatory drugs,4 cholesterol reducing drugs,5 antitumor agents6 and many more. They are known to inhibit reverse transcriptase [human immunodeficiency virus type 1 (HIV-1)] and cellular DNA polymerases protein kinases. Moreover, they are also a component of polymers,7 indigoid dyes5 and of larger aromatic rings.8 In catalytic reactions, pyrroles are well utilized as catalyst for polymerization process,9 corrosion inhibitor,10 preservative,11 solvent for resin,12 terpenes, in metallurgical process,13 transition metal complex catalyst chemistry for uniform polymerization,14 luminescence chemistry15 and spectrochemical analysis.16 Furthermore, some of these compounds are useful intermediates in the synthesis of biologically important naturally occurring alkaloids17 and synthetic heterocyclic derivatives.18 In this present review, attempt has been made to discuss various synthetic approaches that can be used to synthesize this resourceful moiety, in addition various biological active molecules containing pyrrole has been discussed with regard to structure activity relationship or substitution of different groups on the pyrrole ring.
2. Synthetic routes of pyrrole and its analogs
The thorough literature reports various synthetic routes. A number of methods have been reported till now to synthesize pyrrole and pyrrole containing analogs. One of the synthetic approaches includes the synthesis of pyrrole derivatives and their utilization for the preparation of pyrrolo-pyrimidine derivatives. The reaction of benzoin with antipyrine amine and malononitrile in non-polar solvent gave the pyrrole derivative 1 which was further utilized for the preparation of pyrrole derivatives using appropriate reagents and reaction conditions. The pyrrole derivatives 2a–c were further converted to the corresponding pyrrole [2,3-d] pyrimidines (Scheme 1).19 An another approach includes the synthesis of various 2,5-bis (guanidino-aryl)-1-methyl-1H-pyrroles from 1-methyl-1H-pyrrole and were reported as active antifungal agents. The synthetic route included a six step synthesis reaction. The 1-methyl-1H-pyrrole, 3 on reaction with tri-n-butyltin chloride in the presence of n-butyl lithium and N,N,N′,N′-tetramethylethylenediamine in reflux with hexane gave 2,5-bis(tri-n-butylstannyl)-1-methyl-1H-pyrrole 4.20 This compound on ‘stille’ coupling with substituted bromonitroarene in the presence of tetrakis (triphenylphosphine) palladium (O) gave the corresponding nitro intermediates 5. The nitro derivatives were then reduced with tin(II) chloride dihydrate to obtain the amino compounds 6, which by reaction with Boc-protected S-methylthiourea in the presence of mercury(II) chloride gave the Boc-protected diguanidino analogues 7. Deprotection of the Boc-protected guanidine analogues was carried out using ethanolic–HCl in dichloromethane at 0 °C to give the corresponding 2,5-bis(guanidino-aryl)-1-methyl-1H-pyrrole derivatives 8 in good yield as shown in Scheme 1. The SAR studies of the above synthesized compounds revealed that 4-guanidino compound having methyl substituent on the phenyl ring was the most active compound against Candida albicans, Candida krusei, Candida parapsilosis, Candida glabrata, Candida tropicalis and compound having a chloro substituent on the phenyl ring also showed moderate activity against Candida species.21
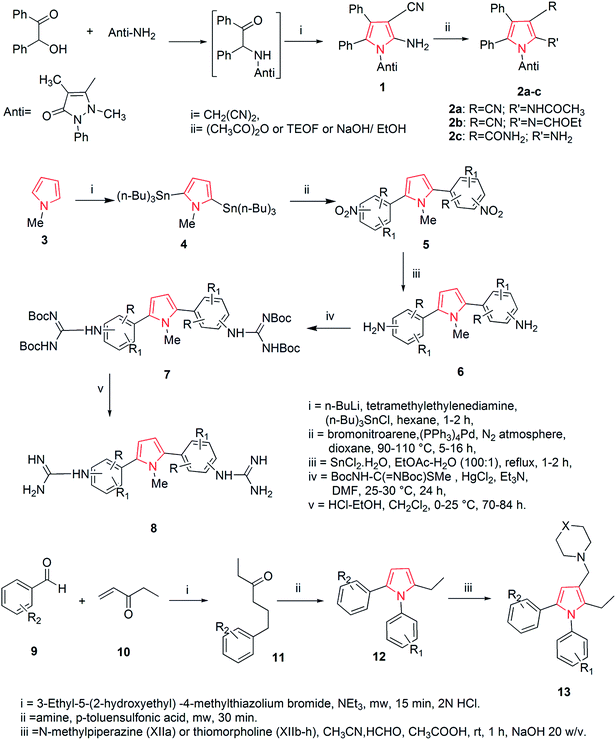 |
| Scheme 1 Systemic route for synthesis of pyrrole incorporated derivatives. | |
During the search of novel antitubercular drugs, Biava et al. designed and synthesized a new series of diarylpyrroles. Structure activity relationship (SAR) studies, along with a pharmacophoric model allowed them to find derivatives in which the following substituents and substitution pattern were responsible for the activity on the pyrrole ring; (i) a substituted phenyl ring at both the positions 1 and 5 (F, Cl, and CH3 were the best substituents) and (ii) an amino methyl group at position 3 (a thiomorpholinomethyl side chain was the optimal moiety). On this basis, the synthesis of a new derivative 13 bearing an ethyl group at position 2 of the pyrrole nucleus was carried out, while keeping the same substituents on both N1 and C5 phenyl rings, gave the best results in terms of activity. This approach includes the reaction of a suitable benzaldehyde 9 with ethyl vinyl ketone 10 that afforded 1,4-diketones 11. In the presence of the appropriate amine, following the Paal–Knoor condensation conditions for 30 min, intermediates 11 were cyclized to yield the expected 1,5-diarylpyrroles 12. Construction of the side chain at C3 was achieved in good yield by reaction with formaldehyde and N-methylpiperazine or thiomorpholine to give the expected derivatives 13 (Scheme 1).22
Based on the structure of HIV-1 gp41 binding site for small molecule inhibitors, He et al. synthesized a new series of 2,5-dimethyl-3-(5-(N-phenylrhodaninyl) methylene)-N-(3-(1H-tetrazol-5-yl) phenyl) pyrrole compounds with improved anti-HIV-1 activity. The Paal–Knorr reaction was performed to synthesize 3-(2,5-dimethyl-1H-pyrrol-1-yl) benzonitrile 15 by the condensation of 3-aminobenzonitrile 14 with 2,5-hexanedione. An aldehyde group was then introduced at the 3-position on the pyrrole ring to obtain the 3-(3-formyl-2,5-dimethyl-1H-pyrrol-1-yl) benzonitrile 16. The cyano group of 16 was further converted into tetrazolyl by treating with sodium azide and triethylamine hydrochloride in refluxed toluene to get the intermediate 2,5-dimethyl-N-(3-(1H-tetrazol-5-yl)phenyl)pyrrole-3-carbaldehyde 17. To expand the structural scaffold of binding site in a more linear manner, a series of N-substituted rhodanine derivatives 19a–i were prepared by treating substituted anilines 18a–i with bis (carboxylmethyl) trithiocarbonate. The intermediate 19j was synthesized by the condensation of 4-trifluoromethylphenyl isothiocyanate with 2-mercaptoacetic acid and intermediates 19k and 19l were prepared by treating an amine with carbon disulfide and 2-bromoacetic acid successively. The rhodanine derivatives 19a–l so prepared were refluxed with ammonium acetate in toluene and methanol (2
:
1, v/v) for 3–5 h to get the corresponding new compounds 20a–l with a yield range of 55–95% (Scheme 2). Among the synthesized rhodanine derivatives, 20a and 20i showed good inhibitory activities against gp416-HB formation and HIV-1 replication.23
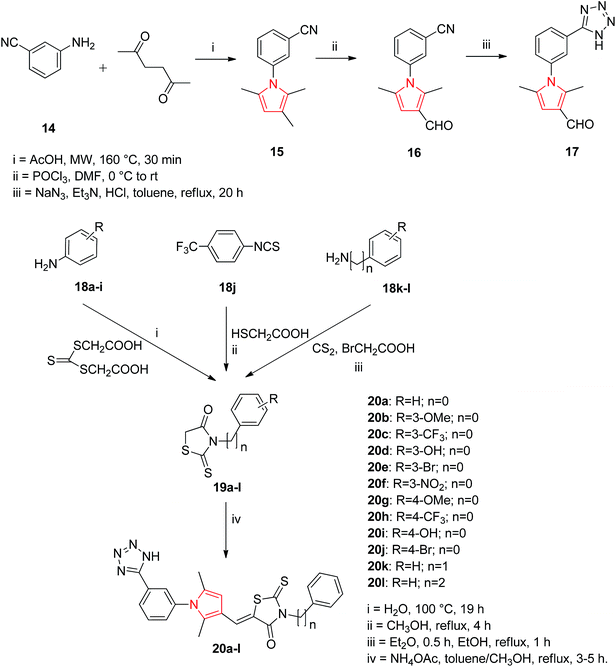 |
| Scheme 2 Synthesis of rhodanine derivatives. | |
Wallace et al. designed various novel derivatives of 3-aminopyrrole compounds 21 that inhibited the MEK allosteric site. The N-acetyl pyrrole compounds 21 showed excellent enzymatic activity but weak micromolar activity in cellular assays due to the inherent chemical instability of pyrrole N-deacetylation during assay incubation. To solve this issue they modified the central core while retaining all of the key binding elements by changing the substitution from position 3 to position 2 i.e. 2-aminopyrrole scaffold24 as presented in Scheme 3. Another series of novel N-substituted pyrrole derivatives were designed and synthesized by an eco-friendly route using ultrasound-assisted bismuth nitrate-catalyzed reaction. Ultrasonic exposure of different amines with 2,5-dimethoxytetrahydrofuran in the presence of catalytic amount (5 mol%) of bismuth nitrate pentahydrate produced the corresponding pyrroles 22 with excellent yield (Scheme 4). In addition, Table 1 represents different synthesized derivatives. The derivatives 22i and 22j exhibited good cytotoxic activity against some cancer cell lines.25
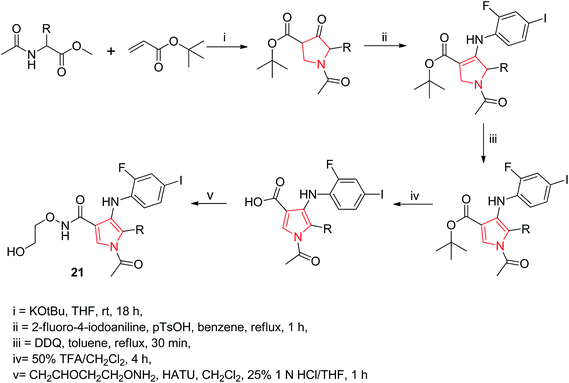 |
| Scheme 3 Synthesis of 2-aminopyrrole scaffold. | |
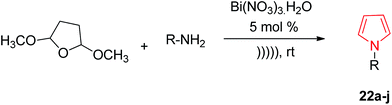 |
| Scheme 4 Synthesis of N-substituted pyrrole derivatives by an eco-friendly route using ultrasound-assisted bismuth nitrate-catalyzed reaction. | |
Table 1 The synthesized N-substituted pyrroles by Bi(NO3)3·5H2O catalyzed ultrasound-induced synthesis as obtained from Scheme 4
Compound code |
Amine |
Product |
Time (min) |
Yield (%) |
22a |
 |
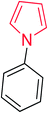 |
5 |
99 |
22b |
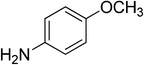 |
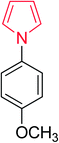 |
5 |
95 |
22c |
 |
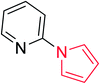 |
5 |
92 |
22d |
 |
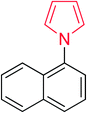 |
5 |
95 |
22e |
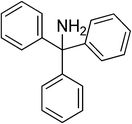 |
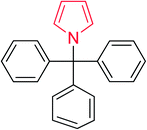 |
30 |
87 |
22f |
 |
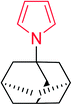 |
35 |
79 |
22g |
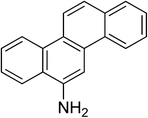 |
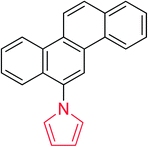 |
10 |
92 |
22h |
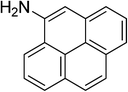 |
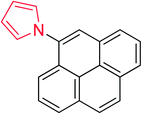 |
15 |
87 |
22i |
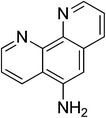 |
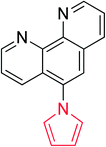 |
60 |
76 |
22j |
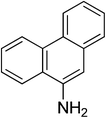 |
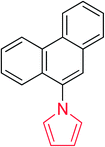 |
5 |
86 |
Yavari et al. reported a solvent-free synthesis of 1,2,3,5-tetrasubstituted pyrroles 23 from enaminones and α-haloketones by simple mixing the starting materials at room temperature for 3 h without the use of any solvent or catalyst. The different substitutions were made as per the required desired product. The preferred substitutions included; R = OEt, R′ = n-Bu and R′′ = 4-Br-C6H4, COOEt, 4-MeO-C6H4.26 Another reaction of 1-aryl-1H-pyrrole-2,5-diones 24 with nonstabilized azomethineylides, which were generated in situ via decarboxylative condensation of isatins 25 and sarcosine 26, afforded only one product i.e. 4′-aryl-5′a,6′-dihydro-1′-methyl-spiro [3H-indole-3,2′(1′H)-pyrrolo [3,4-c] pyrrole]-2,3′,5′(1H,2′aH,4′H)-triones 27 (Scheme 5). The synthesized compound 27 revealed moderate anti-tumor properties against HCT116 (colon), MCF7 (breast) and HEPG2 (liver) human tumor cell lines, as compared to Doxorubicin.27
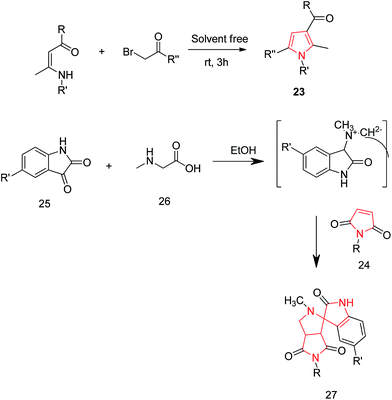 |
| Scheme 5 Synthesis of pyrrole containing analogs. | |
Goel et al. synthesized 2-methyl-3,4,5-triphenyl pyrrole derivatives 28 as antihyperglycemic agents by refluxing a mixture of benzoin, benzyl methyl ketone and ammonium acetate in acetic acid. It was also reported that this reaction also lead to a minor byproduct 29 which was possibly formed due to self-condensation of benzoin with ammonium acetate in presence of acetic acid and air as shown in Scheme 6. This byproduct was avoided by the synthesis of 3,4,5-triphenyl-1H-pyrroles under nitrogen atmosphere using anhydrous ammonium acetate. The synthesized compounds were evaluated for antihyperglycemic activity and the results suggested that unsubstituted-phenyl ring at positions 4 and 5 of the pyrrole reduces elevated blood sugar levels while substitution at positions 3 or 4 of phenyl ring resulted in either reduction or a complete loss of antihyperglycemic activity. A compound with trifluoromethyl group at position 3 of the aryl ring displayed good antidiabetic activity.28 Qin et al. proposed a mild and convenient method for the synthesis of 4(3)-substituted 3-(4)-nitropyrrole 30 from nitro olefins and TosMIC in ionic liquid 1-butyl-3-methylimidazolium bromide ([bmIm] Br).29 Borbas et al. found that bacteriochlorins gets absorbed strongly in the near infrared region and hence are suited for diverse photo medical application. A de novo route was exploited to prepare synthetic bacteriochlorins 31 using TosMIC chemistry (Scheme 6).30
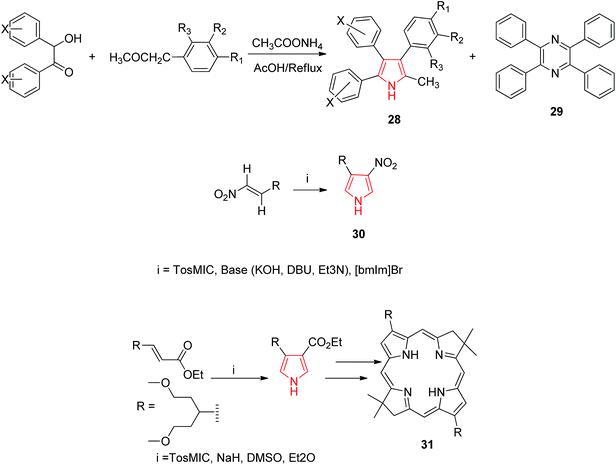 |
| Scheme 6 Synthesis of methyl substituted pyrroles 28, nitropyrrole 30 and synthetic bacteriochlorins 31. | |
Oligofunctional pyrroles play a pivotal role, being basic constituents of numerous natural products, potent pharmaceuticals, molecular sensors and devices. In this context, Larionov et al. developed a method for synthesizing tri-substituted pyrroles. They developed 2,3,4-trisubstituted pyrroles 34 by reacting toluene-4-sulfonylmethyl isocyanide (TosMIC) 32 and substituted acetylenes 33 by cycloaddition method. It involved a formal cycloaddition of an α-methylated isocyanide across a C–C triple bond in an electron-substituted acetylene furnishing a transient 2H-pyrrole which led to a 1,5-hydrogen shift and protonation to give a 2,3,4-trisubtituted pyrrole 34.31 Santo et al. synthesized novel series of N-substituted derivatives of 1-[(aryl) (4-aryl-1H-pyrrolol-3-yl) methyl]-1H-imidazoles using TosMIC protocol and reported their QSAR studies. They developed the key intermediate (2,4-dichlorophenyl)-[4-(naphthalen-1-yl)-1H-pyrrol-3-yl] methanone 35 by using TosMIC chemistry. These compounds were found to be active against Candida species.32 A new class of highly fluorescent low molecular weight water soluble cholephilic compounds were synthesized from dipyrrinones in two steps. The reaction of acetylated β-hydroxynitro product 36 with TosMIC in presence of tetramethylguanidine (TMG) afforded tosyl pyrrole 37, which was later converted to dipyrrinones.33 Airaksinen et al. prepared 3,4-disubstituted pyrroles 39 from 6/7-carboxyethyl-3-phenyl-3-tropen-2-ones 38 regioselectively (Scheme 6). This synthetic procedure provided two distinct substituents of the pyrroles: a phenyl group and a pyrrolidine analogue (Scheme 7).34
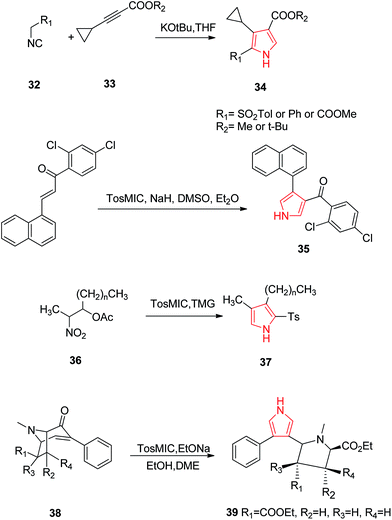 |
| Scheme 7 Synthesis of tri-substituted pyrrole 34, pyrrole containing imidazole 35, cholephilic compound 37 and pyrrolidine analogues 39. | |
Smith et al. reported the synthesis of 3-aryl and 3,4-diaryl-(1H)-pyrroles 41 (Table 2) in one step from TosMIC and commercially available or easily synthesizable aryl alkenes 40, as reported in Scheme 8. The methodology resulted in higher yields (>65%) when electron deficient aryl groups were attached to the alkenes.35
Table 2 Synthesis of aryl pyrroles using TosMIC
Ar1 |
Ar2 |
Temperature (°C) |
Reaction time (h) |
Yield (%) |
 |
 |
25 |
4 h |
91 |
 |
 |
80 |
2 h |
49 |
 |
CH3 |
60 |
3 h |
53 |
 |
H |
25 |
1 h |
76 |
 |
H |
50 |
18 h |
47 |
 |
 |
50 |
1 h |
72 |
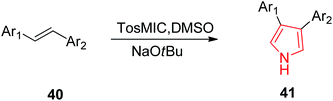 |
| Scheme 8 One step synthesis of 3-aryl and 3,4-diaryl-(1H)-pyrroles using TosMIC. | |
Various substitutions on the pyrrole ring were found to possess diversity of medicinal importance. Azizi et al. reported an operationally simple, practical and economical method for the synthesis of N-substituted pyrroles 43 under very mild reaction conditions in good to excellent yields as shown in Scheme 9. It included the condensation of 2,5-dimethoxytetrahydrofuran 42 with various amines and sulfonamines in the presence of water and a catalytic amount of iron(III) chloride.36 The introduction of acyl group on nitrogen atom of pyrrole possessed medicinal importance. Maehara et al. reported the condensation of carboxylic acid moiety with substituted amine i.e. 2,4,4-trimethoxybutan-1-amine 44 under reflux conditions followed by acid-mediated cyclization, that resulted in the formation of N-acyl derivative of pyrrole 45 as shown in Scheme 9. This method was highly tolerant to various functional groups.37 The highly substituted pyrrole ring was found to be highly stable and depending upon the various functional groups on each substituent, which can act on a variety of receptor sites. 1,2,5 or 3,4 substitutions on pyrrole ring and were found to possess biological importance. The copper catalyzed reaction of amine with 1,4-dihalo-1,3-dienes 46, allowed the synthesis of pyrroles and heteroaryl pyrroles 47 with a wide variety of functional groups and substitution patterns.38 Similarly, the Cu-catalyzed double alkenylation reaction of amide with 1,4-dihalo-1,3-dienes 48 afforded di- or trisubstituted N-acylpyrroles 49 (ref. 39) in good yields using CuI as the catalyst and Cs2CO3 as the base (Scheme 9).
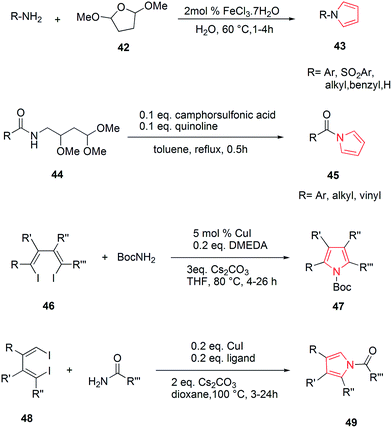 |
| Scheme 9 Synthesis of substituted pyrrole containing analogs. | |
Ozdemir et al. synthesized new Pd–NHC complexes that were used for direct arylation of pyrrole derivatives. Electron-deficient aryl chlorides were used as coupling partners. The desired products 50a–e were obtained in good yields by using 1 mol% of air-stable palladium complexes.40 A three-component reaction of arylglyoxal hydrates was performed with β-dicarbonyl compounds in the presence of ammonium acetate and hydrazine hydrate for the preparation of 5-aryl-4-hydroxy-2-methyl-1H-pyrrole-3-carboxylic acid ester 51 (Scheme 10). Water was used as a solvent and the reaction proceeded under ultrasonic irradiations.41 During samarium di-iodide mediated N–O cleavage of 3,6-dihydro-2H,1,2-oxazine 52; an enantiopure 1,4-amino alcohol 53 and 3-methoxypyrrole derivative 54 were prepared in significant amounts42 as shown in Scheme 10. A sequential multicomponent process was designed to prepare the polysubstituted functionalized pyrroles 55 that involved the high-speed vibration milling of ketones with N-iodosuccinimide and p-toluene sulphonic acid, followed by the addition of mixture of primary amines, β-dicarbonyl compounds, cerium(IV) ammonium nitrate and silver nitrate.43 The dual role of nitriles was exploited during a novel multicomponent reaction that afforded 3H-pyrroles 56 from ketones.44 The reaction revealed that nitriles can act both as nucleophiles and electrophiles. The reaction used two equivalents of malonitrile, the corresponding thiols and was catalyzed by triethylamine in water (Scheme 10).
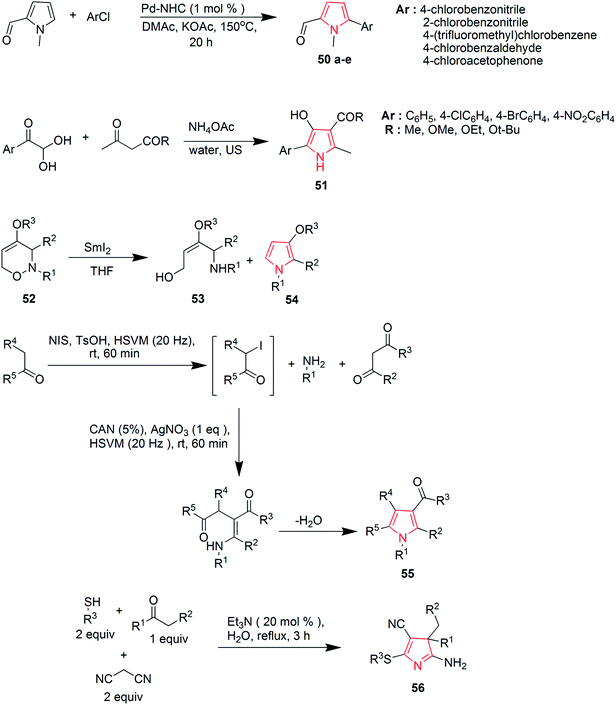 |
| Scheme 10 Systemic approach to synthesize substituted pyrroles. | |
Khulpe et al. synthesized the novel 2-methyl-7-(4-nitrophenyl)-5,6-diphenyl-3,7-dihydro-4-H-pyrrolo-[2,3-d]pyridine-4-one derivatives by the Paal–Knorr condensation reaction. Benzoin 57 was refluxed with primary aromatic amines in the presence of alcohol, which led to the formation of α-aminoketone intermediates 58, which were condensed, without isolation, with malonitrile to yield various pyrrole derivatives 59 (Scheme 11).45 2,3-Fused pyrroles 61 were synthesized from cyclic ketones by rhodium-catalyzed reaction of 4-alkenyl-1-sulfonyl-1,2,3-triazoles 60. The reaction involved an unusual 4Π electrocyclization.46 A new method was developed for the amino-acylation of pyrroles. The procedure involved a multicomponent one-pot cascade reaction between pyrroles, ynol ethers and sulfonyl azides leading to the formation of four different bonds regioselectively through N-sulfonyltriazole intermediates (Scheme 11). The desired oxo-pyrroloethanamines 62 were generated in moderate to high yields.47
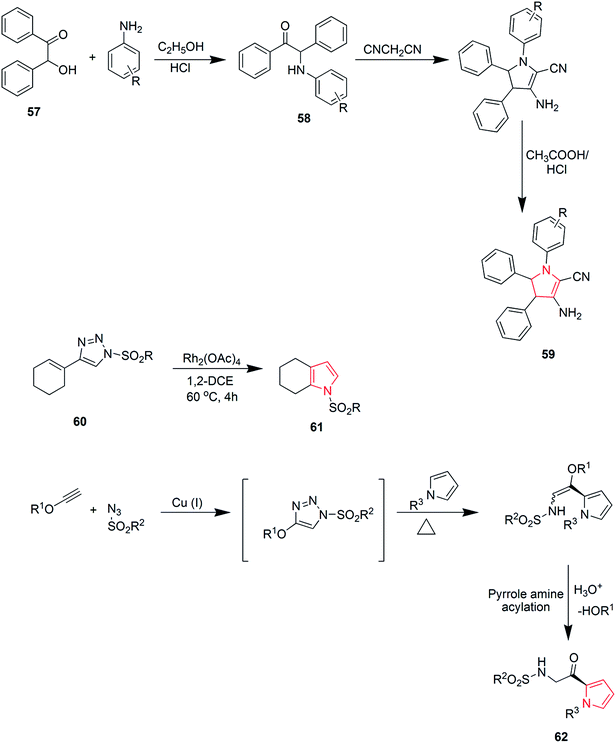 |
| Scheme 11 Synthesis of various catalyzed pyrrole containing products. | |
Blackburn et al. synthesized substituted triarylpyrroles by reacting 1,2-dibenzoylethane 63 with 4-bromoaniline 64 that yielded 2,5-symmetrically substituted pyrroles 65. Trifluoroethanol (TFE) was used as a solvent and trifluoroacetic acid (TFA) was used as a catalyst under microwave conditions. Vilsmeir–Haack conditions were applied for formylation of pyrroles 65 under microwave conditions to give 3-formylpyrroles 66. Further condensation of 66 with barbituric acid or thiobarbituric acid in presence of ethanol at room temperature yielded pyrrole barbiturates 67 (Scheme 12).48 The palladium(II)-catalyzed oxidative cyclization reaction of N-allylimines 68 was reported to form pyrrole. N-Allylimines were derived from methyl ketones, especially acetophenones, which afforded pyrrole derivatives 69 at room temperature in presence of oxygen. The reaction occurred through α-palladation of imine followed by migratory insertion of olefin and β-hydride elimination. This reaction represented a new example of aerobic dehydrogenative Heck cyclization.49 Various polysubstituted pyrroles 71 were synthesized in good yields (Scheme 12) by Ag(I)-mediated conjugated addition and cyclization reaction of terminal alkynes 70 with amines.50 Natural products didebromohamacanthin A 74 and demethylaplysinopsine 73 were synthesized via oxidative nucleophilic addition of ethylenediamine and guanidine derivatives with pyrrole-amino acid diketopiperazines 72 (ref. 51) as shown in Scheme 12. Fesenko and Shutalev synthesized 2-phenyl-3-(phenylthio)-1H-pyrrole-1-carboxamide 76 from 4-phenyl-5-(phenylthio)-1H-1,3-diazepin-2(3H)-one 75 under acidic conditions by refluxing in 95% ethanol and TsOH (0.23 equiv.) for three hours. On increasing the amount of TsOH to 1.01 equivalents, product 76 was obtained in 98% yield.52 Some substituted pyrroles 78 were synthesized by metal-catalyzed heterocyclodehydration of 1-amino-3-yn-2-ol 77. The heterocyclodehydration occurred by 5-exodig intramolecular nucleophilic attack of hydroxyl group to triple bond, coordinated to the metal center, which was followed by protonolysis and aromatization.53 A new and efficient method was developed for the synthesis of uracil fused pyrrole derivative 5-(4-methoxyphenyl)-1,3-dimethyl-1H-pyrrolo[2,3-d]pyrimidine-2,4(3H,7H)-dione 80 as shown in Scheme 13. The process involved a three-component reaction comprising of 1,3-dimethyl-6-aminouracil 79, p-methoxybenzaldehyde and nitromethane, while CuFe2O4 was used as a catalyst. The reaction completed in 4 hours and product was obtained in good yield.54 New three-component coupling process was designed to synthesize pyrroles 82 (Scheme 13). The reaction proceeded by reacting symmetrical vicinal diols 81 with enamines or imines, involving intramolecular ruthenium-catalyzed dehydration and N–H alkylation steps that resulted in substituted pyrroles.55 Novel pyrrole derivatives were synthesized by click reaction using silver-catalyzed cycloaddition of terminal alkynes with isocyanides. Phenylacetylene was reacted with 2-isocyanoacetate in presence of N-methyl-2-pyrrolidone and silver carbonate to give ethyl-3-phenyl-1H-pyrrole-2-carboxylate 83 in 89% yield56 as shown in Scheme 13. A novel method was developed for the synthesis of substituted pyrroles via Paal–Knorr reaction (Scheme 13). The reaction was catalyzed by α-amylase derived from hog pancreas. 4-Chloroanilline was reacted with 2,5-hexanedione in presence of α-amylase to obtain 1-(4-chlorophenyl)-2,5-dimethyl-1H-pyrrole 84 in 94% yield.57
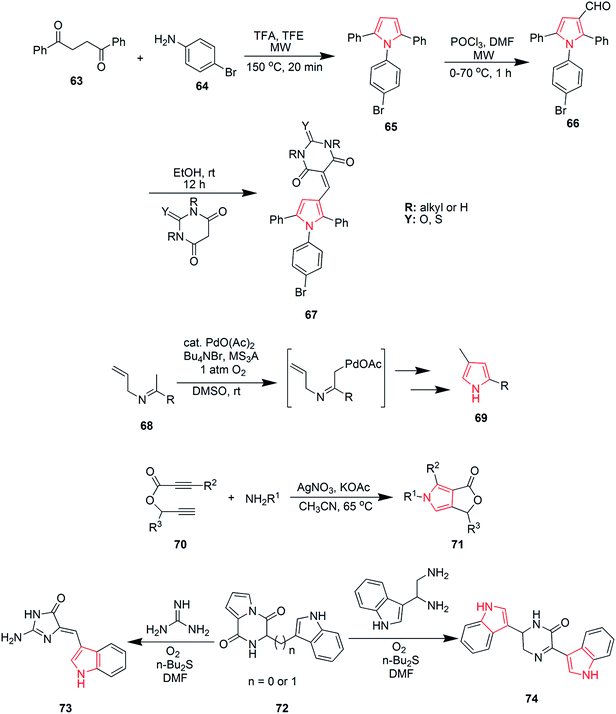 |
| Scheme 12 Synthetic route for catalyzed pyrrole containing analogs. | |
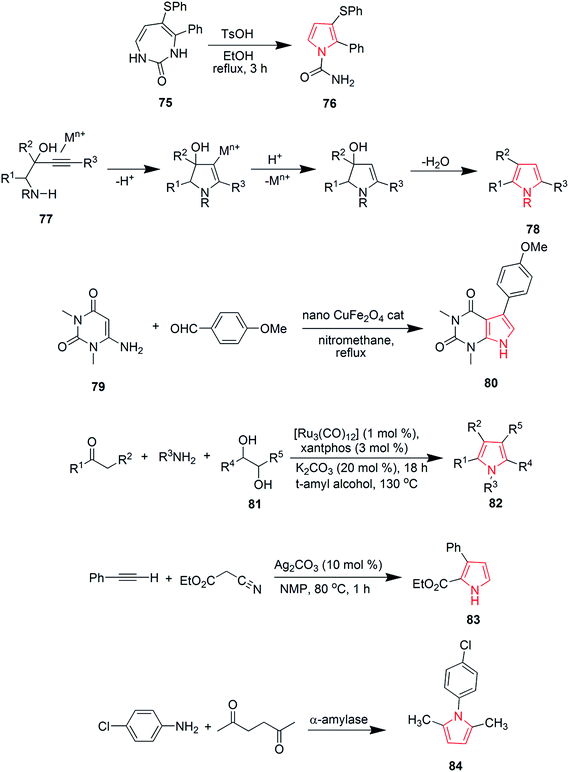 |
| Scheme 13 Different catalyzed synthetic route to synthesize substituted pyrroles. | |
Murugan et al. synthesized various substituted pyrroles via ruthenium-catalyzed oxidative cyclization of enamides with alkynes in water or dimethoxyethane. The product 86 was obtained in 95% yield by refluxing a mixture of ethyl-2-acetamidoacrylate 85, dialkyl acetylene, [Ru(p-cymene) Cl2] (2 mol%), KPF6 and Cu(OAc)2 in water.58 A ruthenium carbene catalyzed ring-closing metathesis reaction and FeCl3·6H2O or CuCl2·2H2O catalyzed in situ oxidative dehydrogenation reaction was implied for synthesis of aryl-substituted pyrrole derivatives 88 and 89 (Scheme 14). Diallylamines 87 was used as a starting material in the presence of oxygen and the reaction was mild, simple and convenient.59 Polysubstituted pyrroles were synthesized from readily available isocyanides, primary or secondary amines and gem-diactivated olefins. The synthesis involved a multicomponent domino reaction to obtain the chemoselective and structurally diverse pyrroles 90 and 91 without the use of any catalyst.60 A novel four-component domino reaction of arylglyoxal monohydrate, aniline, dialkyl but-2-ynedioate and malonitrile was reported for the synthesis of polysubstituted pyrroles 92. The reaction was highly efficient, ethanol was used as a solvent and proceeded without catalyst.61 Novel 1,2,3-trisubstituted pyrroles were synthesized via iodocyclization from acetoacetate (Scheme 15). This one-pot two step reaction involved the treatment of ethyl-3-(propylamino) but-2-enoate 93 with acetaldehyde and iodine in basic conditions to give the desired product ethyl-2-methyl-1-propyl-1H-pyrrole-3-carboxylate 94 as shown in Scheme 15.62
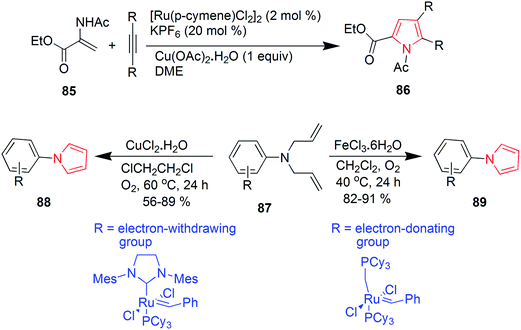 |
| Scheme 14 Synthesis of metal catalyzed pyrroles. | |
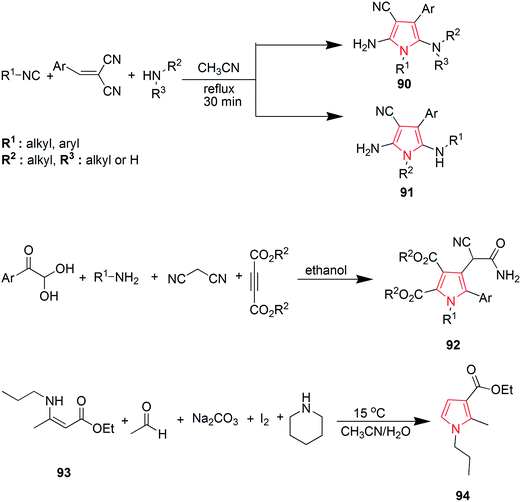 |
| Scheme 15 Synthesis of pyrroles using various solvent systems. | |
Viradiya et al. developed an eco-friendly and highly efficient method for the one-pot synthesis of penta-substituted pyrrole derivatives via a four-component reaction of pyrimidine-2,4,6(1H,3H,5H)-trione 95, 1-(4-fluorophenyl)-2,2-dihydroxyethanone 96, dimethyl but-2-ynedioate and 4-methoxyanilline. This catalyst-free and environmental friendly reaction yielded dimethyl-5-(2,2-dimethyl-4,6-dioxohexahydropyrimidin-5-yl)-4-(4-fluorophenyl)-1-(4-methoxyphenyl)-1H-pyrrole-2,3-dicarboxylate 97 in excellent yield and short time duration63 as shown in Scheme 16. Highly functionalized bicyclic pyrrole derivatives were synthesized through a three-component one pot reaction of 1-(4-fluorophenyl)-2-(tetrahydropyrimidin-2(1H)-ylidene)ethanone 98, 1H-indole and 1-(4-fluorophenyl)-2,2-dihydroxyethanone 99. The reaction proceeded in ethanol medium and was catalyzed by acetic acid to give the pyrrole containing product 100 (Scheme 16).64 The reaction between methyl-2,5-dioxopyrrolidine-1-carboxylate 101 and Petasis reagent led to the formation of dienamine product 102, which was isomerized under mild conditions to give methyl-2,5-dimethyl-1H-pyrrole-1-carboxylate 103 in 96% yield.65 Various trifluoromethyl substituted pyrrole derivatives were prepared. The phosphine-mediated reaction used commercially available trifluoroacetic anhydride as the only trifluoromethyl source. Dimethyl-2-(phenyl(tosylmino)methyl)fumarate 104 was reacted with triphenyl phosphine and dichloromethane to give dimethyl-2-phenyl-1-tosyl-5-(trifluoromethyl)-1H-pyrrole-3,4-dicarboxylate 105 in 48–99% yield.66 A green and rapid strategy was developed for the synthesis of novel pyrroles by using molybdate sulfuric acid (Scheme 16). Pechmann condensation of meta-aminophenol with ethylacetoacetate under solvent-free condition yielded 7-amino-4-methylcoumarin 106, which was reacted with hexane-2,5-dione to give 7-(2,5-dimethylpyrrole-1-yl)-4-methylcoumarin 107 in overall 90% yield67 as shown in Scheme 16.
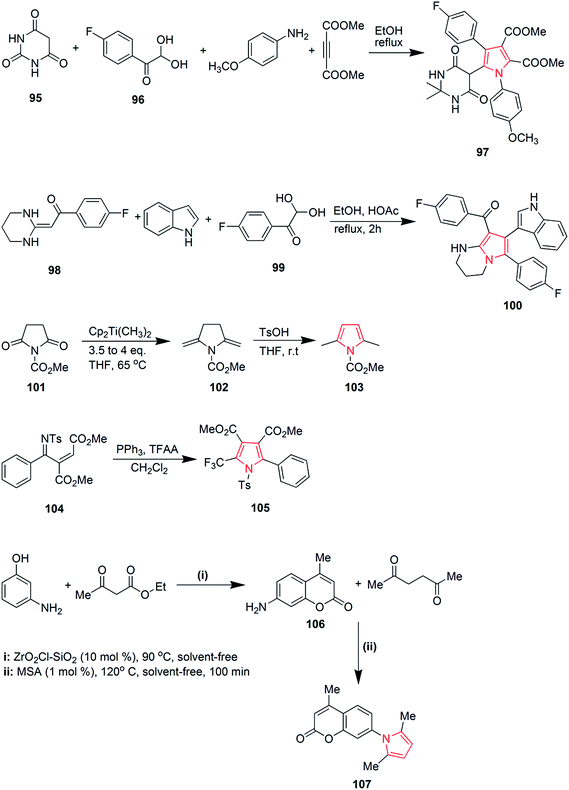 |
| Scheme 16 Various synthetic route to synthesize pyrroles incorporated analogs. | |
Murthi et al. designed a faster and efficient method for the synthesis of polysubstituted pyrrole derivative 108 through a four-component reaction of β-ketoesters, benzylamines, aromatic aldehydes and nitromethane. The reaction was carried out under ultrasound and Amberlyst-15 was used as a catalyst68 as shown in Scheme 17. Novel 3-arylamino substituted fused pyrrole derivatives were prepared through p-TsOH promoted N-arylation of 2,2-dihydroxy-1-(4-methoxyphenyl) ethanone 109, (1-methyl-5-oxo-3-(phenylamino)cyclohex-3-en-1-yl)methylium 110 and 4-nitroanilline. The reaction was carried out in ethanol under microwave heating and the product 111 was formed in 90% yield.69 Polysubstituted pyrrole derivative 1-(1,4-bis(4-methoxyphenyl)-2-methyl-1H-pyrrole-3-yl)ethanone 112 was synthesized by one-pot four-component coupling of 4-methoxyanilline, 4-methoxybenzaldehyde, acetylacetone and nitromethane in gluconic acid aqueous solution (GAAS). Gluconic acid aqueous solution was recycled and reused several times without significant loss of its activity.70 One more novel method was designed for the synthesis of pyrroles via palladium-catalyzed aerobic oxidative intramolecular alkenylation of Csp3–H bond. Ethyl-3-(allylamino)-3-phenylacrylate 113 was treated with palladium catalyst under mild conditions in presence of molecular oxygen as the terminal oxidant, to form ethyl-4-methyl-2-phenyl-1H-pyrrole-3-carboxylate 114.71 Various diversely functionalized pyrroles were synthesized under catalyst-free condition by using ionic liquid as a reaction media from an efficient four-component reaction of prop-2-yn-1-amine, 3-hydroxybenzaldehyde, nitromethane and pentane-2,4-dione (Scheme 17). The reaction completed by using an ionic liquid 1-n-butylimidazolium tetrafluoroborate [Hbim]BF4, that yielded 1-(4-(3-hydroxyphenyl)-2-methyl-1-vinyl-1H-pyrrol-3-yl)ethanone 115 without any additional catalyst or promoter.72 Michlik and Kempe introduced a sustainable iridium-catalyzed synthesis of pyrroles by deoxygenating secondary alcohols and amino alcohols by linking them through the formation of C–N and C–C bonds. Oxidation of commercially available 1-phenylethanol from potassium tertiary butoxide led to the formation of acetophenone, which was fused with 2-aminobutanol to form 2-((1-phenylethylidene) amino) butan-1-ol 116. Further treatment of this intermediate with potassium tertiary butoxide in presence of iridium catalyst yielded 2-ethyl-5-phenyl-1H-pyrrole 117 (Scheme 18).73 A novel and efficient method was developed for the synthesis of substituted pyrroles via palladium(II)-catalyzed alkenyl C–H activation oxidative annulations of enamides with alkynes. N-(1-Phenylvinyl)acetamide 118 was reacted with 1,2-diphenylethyne in presence of tertiary amyl alcohol and palladium catalyst to give 1-(2,3,5-triphenyl-1H-pyrrol-1-yl) ethanone 119 in good yield.74 Highly functionalized pyrroles were synthesized by reaction of rhodium-stabilized imino-carbenes with furans. The reaction of dimethylfuran with N-sulfonyltriazole resulted in the formation of pyrrole 120 in 41% yield.75 Novel 2,4,5-trisubstituted pyrrole derivatives 121 were synthesized through the coupling of α-diazoketones with β-enaminoketones and esters using 10 mol% Cu(OTf)2.76 Various pyrrole derivatives were synthesized from oxazolidines (Scheme 18). On boiling 2-butyl-2-methyl-3-(2-hydroxyethyl)oxazolidine 122 in presence of potassium hydroxide, equimolar amount of pyrrole derivatives 123 and 124 were formed in overall 61% yield (Scheme 18).77
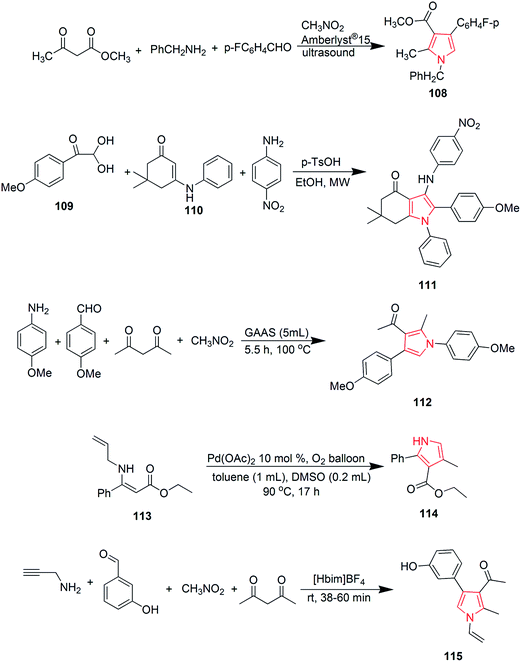 |
| Scheme 17 Synthesis of various catalyzed pyrrole analogs. | |
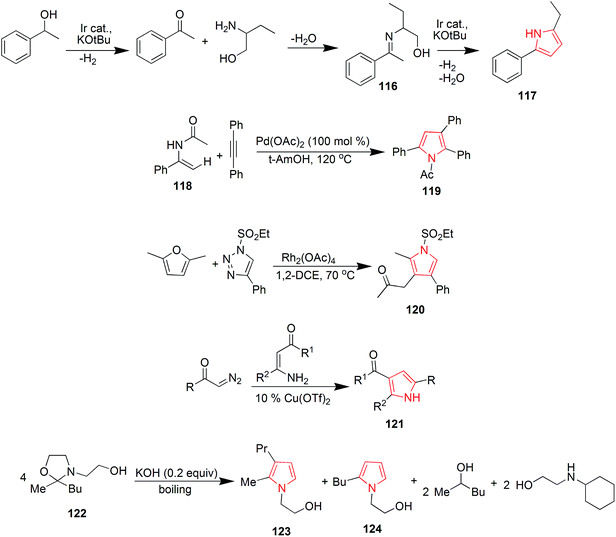 |
| Scheme 18 Synthesis of catalyzed pyrrole molecules. | |
Suresh et al. designed a facile and regioselective method for the synthesis of polysubstituted pyrroles from α-azido chalcones and 1,3-dicarbonyl compounds. The reaction of 2-azido-1,3-diphenylprop-2-en-1-one 125 with pentane-2,4-dione, catalyzed by indium trichloride in water, resulted in formation of (1-(4-benzoyl)-2-methyl-5-phenyl-1H-pyrrol-3-yl) ethanone 126.78 A method was developed to synthesize novel pyrrole ring containing azepine skeleton 128. The reaction was catalyzed by rhodium(II) azavinyl carbene intermediate, which initiated the intramolecular C–H functionalization with pyrrolyl ring of compound 127 (Scheme 19).79 In 1910, Piloty discovered that 2,5-diethyl-3,4-dimethyl pyrrole 130 was formed upon heating diethyl ketone azine 129 at 230 °C. Later on, in 1918, Robinson and Robinson synthesized 2,3,4,5-tetraphenylpyrrole 132 by heating deoxybenzoin azine 131 in hydrogen chloride stream at 180 °C (Scheme 19). These reactions are known as Piloty–Robinson reaction.80 The reaction of diaryl diazomethane with 1,4-dilithio-1,3-diene 133 yielded 2,3,4,5-tetrabutyl-N-(diphenylmethylene)-H-pyrrol-1-amine 134 in high yield. Diaryl diazomethane acted as an electrophile in this reaction.81 A pyrrole-2,3,4,5-tetracarboxylate derivative 135 was obtained during a copper-catalyzed reaction of amine with but-2-ynedioate. The reaction required oxygen atmosphere and three bonds were formed during the process (Scheme 19).82 Zhao et al. studied the palladium-catalyzed direct polyarylation of 1-methylpyrrole. In presence of three equivalents of aryl bromide, 1-(4-(1-methyl-1H-pyrrol-2-yl) phenyl) ethanone 136 and 1,1′-((1-methyl-1H-pyrrole-2,5-diyl)bis(4,1-phenylene)) diethanone 137 were formed. These compounds were formed as the C2 and C5 positions of pyrroles are more reactive for C–H bond functionalization as compared to the C3 and C4 positions. 1 mol% PdCl(C3H5) (dppb) [dppb = 1,4-bis(diphenylphosphino)butane] was used as catalyst, while dimethylacetamide and potassium acetate were the solvents used.83 A new approach was developed for the synthesis of tetrasubstituted pyrroles from the readily available amino acid esters (Scheme 19). Biosynthetic reaction of L-tryptophan 138 with RebO enzyme formed the imine intermediate, which was transformed into chromopyrrolic acid 139 with the action of RebD enzyme via oxidative deamination and cyclization (Scheme 20).84
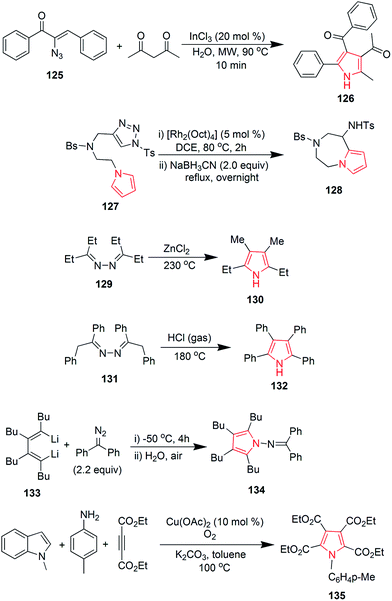 |
| Scheme 19 Substituted pyrrole molecules. | |
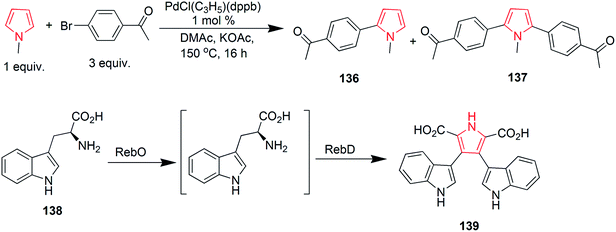 |
| Scheme 20 Catalysis assisted formation of pyrrole containing compound. | |
3. Biological significance of pyrrole containing analogs
Pyrrole, being an important ring structure, has been found to possess a number of biological activities. This ring has a broad range of biologically active compounds, incorporated either as a substituent or with various substitutions on the ring itself. Some of the drugs containing pyrrole moiety are already available in market and some are under clinical trials as presented in Table 3.
Table 3 Marketed pyrrole containing drugs and molecules under clinical trial
S. no. |
Compounds |
Description |
1 |
5-(Methylamino)-2-({(2R,3R,6S,8S,9R,11R)-3,9,11-trimethyl-8-[(1S)-1-methyl-2-oxo-2-(1H-pyrrol-2-yl)ethyl]-1,7-dioxaspiro[5.5]undec-2-yl}methyl)-1,3-benzoxazole-4-carboxylic acid |
• It is produced by fermentation of Streptomyces chartreusensis |
• It possesses antibiotic properties against Gram positive bacteria and fungi |
• It acts as divalent cation inophore and its order of selectivity is: Mn2+ > Ca2+ > Mg2+ > Sr2+ > Ba2+ |
• It inhibits mitochondrial ATPase activity |
• It uncouples oxidative phosphorylation |
• It induces apoptosis in some cells and prevents it in others |
2 |
N-[[(5S,6R,7R)-2-Amino-7-(2-amino-1H-imidazol-5-yl)-5-[[[(4-bromo-1H-pyrrol-2-yl)carbonyl]amino]methyl]-4,5,6,7-tetrahydro-1H-benzimidazol-6-yl]methyl]-4-bromo-1H-pyrrole-2-carboxamide |
• It is a chemical compound produced by some sponges |
• It was first isolated from Caribbean and then Okinawan marine sponges in the genus Agelas |
• It has antibacterial properties |
• It can cause biofilms to dissolve |
3 |
N-[2-(3-Formyl-2,5-dimethylpyrrol-1-yl)ethyl]acetamide |
• It is a neotropic drug of the racetam family |
• It is useful for the treatment of Alzheimer's disease |
4 |
(3R,5R)-7-[2-(4-Fluorophenyl)-3-phenyl-4-(phenylcarbamoyl)-5-propan-2-ylpyrrol-1-yl]-3,5-dihydroxyheptanoic acid |
• It is used for the treatment of dyslipidemia |
• It is useful in preventing cardiovascular diseases |
• It is recommended to be used only after other measures, such as, if diet, exercise and weight reduction have not improved cholesterol levels |
5 |
1-(1-Benzofuran-7-yl)-4-{[5-(4-fluorophenyl)-1H-pyrrol-2-yl]methyl}piperazine |
• It is an antipsychotic drug of the phenylpiperazine class |
6 |
1-(Propan-2-ylamino)-3-(2-pyrrol-1-ylphenoxy)propan-2-ol |
• Isamoltane (CGP-361A) is a drug of scientific research |
• It acts as antagonist at β-adrenergic, 5-HT1A and 5-HT1B receptors |
• It has anxiolytic effect in rodents |
7 |
(5aR,8aS)-3-(2-{4-[3-(Trifluoromethyl)phenyl]piperazin-1-yl}ethyl)-5,5a,6,7,8,8a-hexahydrocyclopenta[3,4]pyrrolo[2,1-c][1,2,4]triazole |
• It is also known as Normarex |
• It is an anxiolytic drug of phenylpiperazine class |
8 |
14-Hydroxy-4-((S)-1-hydrocyethyl)-7-methoxy-1,3,13-trimethyl-6-oxo-3,4,6,7,8,8a,11,12,13,14,14a-dodecahydro-11,14b-epoxynaphthol [2,1-e]oxecin-12-yl 1H-pyrrole-2-carboxylate |
• It is a 28 carbon macrolide with a tricyclic lactone ring and unique ether bridge |
• It is isolated from Nocardia argeninensis |
• It is effective against Gram positive bacteria |
• It induces cell differentiation, thus used for neoplastic diseases |
9 |
2-(2-((3,5-Dimethyl-1H-pyrrol-2-yl)methylene)-3-methoxy-2H-pyrrol-5-yl)-1H-indole |
• It is an experimental drug for the treatment of various types of cancer |
• It is in phase II clinical trials for the treatment of leukemia, lymphoma, myelofibrosis and mastocytosis |
10 |
3-[5-(Aminomethyl)-4-(carboxymethyl)-1H-pyrrol-3-yl]propanoic acid |
• PBG is a drug that involves pyrrole in porphyrin metabolism |
• An acute intermittent porphyria causes an increase in urinary porphobilinogen |
11 |
4-Methoxy-5-[(Z)-(5-methyl-4-pentyl-2H-pyrrol-2-ylidene)methyl]-1H,1′H-2,2′-bipyrrole |
• It possesses antibacterial, antifungal, antiprotozoal, antimalarial, immune suppressive and anticancer properties |
3.1. Anticoccidial activity
A series of various diarylpyrrole derivatives were analyzed for the anticoccidial activity by both in vitro and in vivo assays. The dimethyl amine substituted derivative 140 resulting was found to be the most potent inhibitor against Eimeria tenella (Et) PKG (cGMP-dependent protein kinase).85 Further studies on diarylpyrrole derivatives suggested that the ω-hydroxylated derivatives 141 were more potent inhibitor as compared to their alkyl analogs.86 N-Substituted derivatives of 2,3-diarylpyrrole were evaluated against commercially important strains of Eimeria in chickens. Among these, 5-(N-methyl, N-ethyl, and N-methylazetidine methyl) piperidyl derivatives 142, 143, 144 were found to be most potent with a broad spectrum activity. The N-ethyl piperidine analog 143 had excellent activity when administered at 50–125 ppm levels in an in vivo spectrum model against eight of the most common Eimeria species i.e. E. tenella, E. acervulina, E. necartrix, E. brunetti, E. maxima, E. mitis, E. mivati, and E. praecox.87 N-Alkyl-4-piperidinyl-2,3-diarylpyrrole derivatives with heterocyclic substitutions were also evaluated and found to possess anticoccidial profile. Among the series of compounds evaluated, the azetidine derivative 145 and morpholine derivative 146 showed improvements in potency of Et-PKG inhibition.88
3.2. Anti-inflammatory activity
Tolmetin 147 and Zomepirac 148 are two pyrrole acetic acid derivatives that have now gained a degree of success in treatment of rheumatoid arthritis and pain. Wong and coworker reported that methylation of Zomiperac in the acetic acid chain 149 markedly increased the anti-inflammatory potency as measured by the rat paw kaolin edema assay. Benzoylpyrrolopyrrole carboxylic acid series of compounds also possess high anti-inflammatory and analgesic activity among which p-methoxy derivative of 5-benzoyl-1,2-dihydro-3H-pyrrolo-[1,2-α] pyrrole-1-carboxylic acid and 4-vinylbenzoyl derivatives were most potent (150 and 151)89 as shown in Fig. 1. Bimetopyrol 152, a 2-substituted-4,5-diarylpyrrole derivative, was also reported to show anti-inflammatory and analgesic properties. A series of 2-substituted-4,5-diarylpyrroles as potent anti-inflammatory agents among which 2-[(trifluoromethyl) thio]-4-(4-fluorophenyl)-5-[4-(methylsulfonyl)phenyl] pyrrole 153 (Fig. 1) was reported as potent anti-inflammatory agent against paw edema produced in the adjuvant arthritis rat model.90 A series of 1,2-diarylpyrroles were also found to be selective inhibitors of COX-2 and evaluated for anti-inflammatory activity by adjuvant induced arthritis rat model, among which 1-(4-fluorophenyl)-2-methyl-5-[4-(methylsulfonyl)phenyl]-1H-pyrrole-4-[1-(4-fluorophenyl)-5methyl-1H-2-pyrrolyl] phenylmethylsulfone 154 and 2-(4-fluorophenyl)-5-methyl-1-[4-(methylsulfonyl)phenyl]-1H-pyrrole-4-[2-(4-fluorophenyl)-5-methyl-1H-pyrrolyl] phenyl methyl sulfone 155 (Fig. 1) showed excellent potency for in vivo testing in the carrageenan induced paw edema model in the rat.91
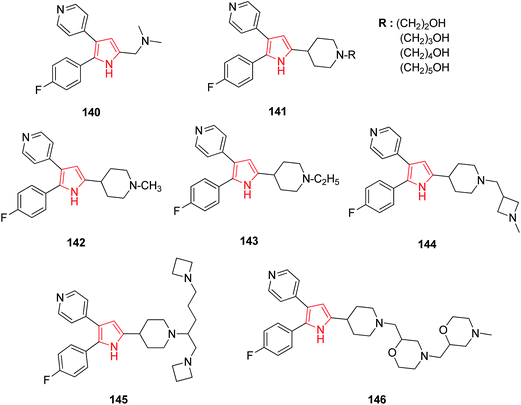 |
| Fig. 1 Pyrrole containing compounds with anticoccidal activity. | |
On the other side, 1,3,4-thiadiazoles when reacted with pyrrole-3-carboxamide moiety were found to hold anti-inflammatory activity. The synthesized compounds were evaluated for their anti-inflammatory activity against carrageenan-induced acute paw edema in Wistar albino rats among which nitro 156 and fluoro 157 substituted derivatives (Fig. 2) were found to be potent showing high activity profile.92 The new class of pyrrole derivatives was synthesized containing a small appendage fragment (carbaldehyde, oxime, nitrile) on the central core. The compound 158 was most effective in vivo and showed a significant profile when compared to the already marketed reference compound. When compared to celecoxib, this compound was more efficient and potent inducing a percentage of writhes reduction.93 The novel pyrrolo [2,3-d] pyrimidine and pyrrolo [1,2,4] triazolo[1,5-c] pyrimidine derivatives were synthesized as anti-inflammatory agents. When compared to the standard drug, ibuprofen, it was observed that compounds 159, 160 and 161 showed increased activity (Fig. 2) due to the introduction of hydrazine group, thione group and phenyl group respectively.19
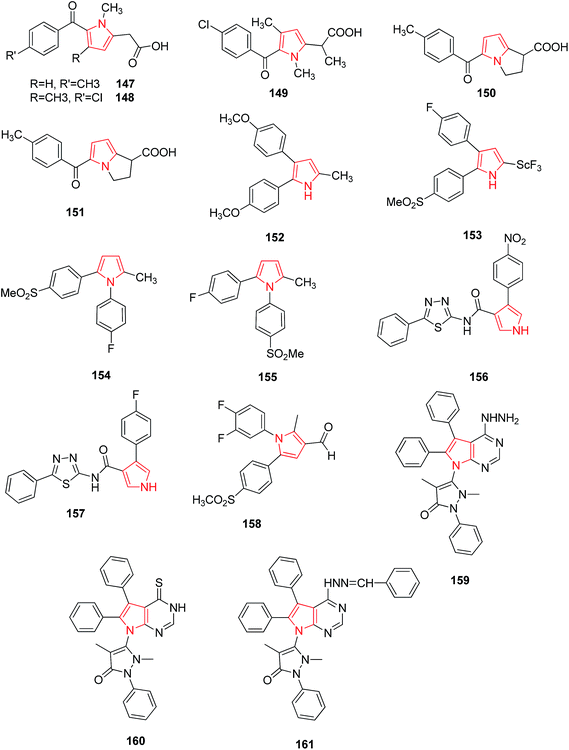 |
| Fig. 2 Pyrrole containing compounds with anti-inflammatory activity. | |
3.3. EP1 receptor antagonist
Synthesized 1,5-biarylpyrroles were found to exhibit potential EP1 receptor antagonist activity. The compound 162 was found to be the most potent among a synthesized series of 1,5-biarylpyrrole derivatives containing two fluorine atoms.94 It was reported that the substitution of benzoic acid moiety at the 5-position by electron donating group such as NH2 163 increased the affinity towards EP1 receptors.95 The substitution of benzoic acid moiety at 6-position and the 5,6-disubstitution also influenced the in vitro affinity profile. The substitution of –F–(164), –OMe–(165),–OCHF2–(166) and –NHAc–(167) at 6-position showed exceptionally high affinities.96 The replacement of carboxylic acid in the side chain of 1,5-biarylpyrroles 72 led to the discovery of novel non-acidic antagonists such as sulfonamide 77, amide 168 and benzimidazole 169 derivatives.97 The respective compounds have been presented in Fig. 3.
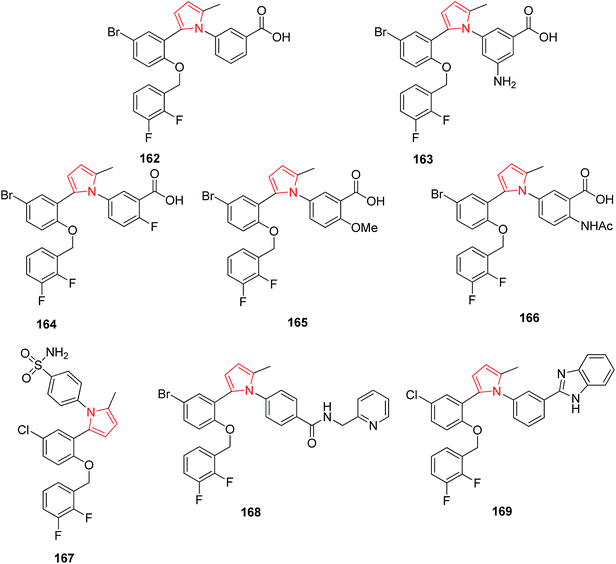 |
| Fig. 3 Pyrrole containing compounds as EP1 receptor antagonist. | |
3.4. Antipsychotic and anticonvulsant activity
2,5-Disubstituted-1H-pyrrole derivatives were synthesized and the modification of basic side chain was carried out by introducing piperidine 170 and 2-phenylazacycloheptane 171 (Fig. 4). The new compounds formed by this reaction showed D3 antagonist activity with 30 fold selectivity for the D3 receptor over the D2 receptor.98 Further modifications of the ethyl sulfone substituent to either phenyl sulfonate 172 or sulfonamides 173, 174 and 175 (Fig. 4) showed high affinities and selectivity for the dopamine D3 receptor over the D2 receptor. These compounds therefore represent valuable pharmacological tools for the characterization of the role of the dopamine D3 receptor in central nervous system.99 Boyfield et al. modified 2,5-disubstitued pyrroles by introducing different substituent in the side chain of phenyl group. The introduction of α-methylbenzyl 176 and aminoindane 177 side chains retained high affinity for the dopamine D3 receptor.100 Similarly, synthesized 2-aroyl-4-(ω-aminoacyl)-1-(1-piperidinyl-acetyl) – 1,3,5-trimethylpyrrole derivatives represent a new, structurally novel class of anticonvulsant agents among which 2-(4-chlorobenzoyl) derivative i.e. RWJ-37868 178, showed better potency and therapeutic index in comparison to those of phenytoin and carbamezipine moreover greater than sodium valproate. This compound blocked bicuculline induced seizures, and did not elevate seizure threshold following i.v. infusion of metrazole and blocked influx of Ca2+ ions into cerebellar granule cells induced by K+ or veratridine.101 Pyrrole ring has also been found to possess biological activity when incorporated as a substituent. A number of novel pyrrole [1,2-a] pyrazine compounds displayed promising seizure protection in the maximal electroshock seizure (MES), subcutaneous metrazol seizure (scMET) and pilocarpine induced status prevention (PISP) tests in epileptic models comparable to the reference anticonvulsant drugs. Among the synthesized pyrrole [1,2-a] pyrazine derivatives, the 4S,8aS diastereomer 179, its ethoxycarbonylmethyl 180 and 2-phenylethyl 181 derivatives (Fig. 4) were found to be highly potent anticonvulsant agents.102
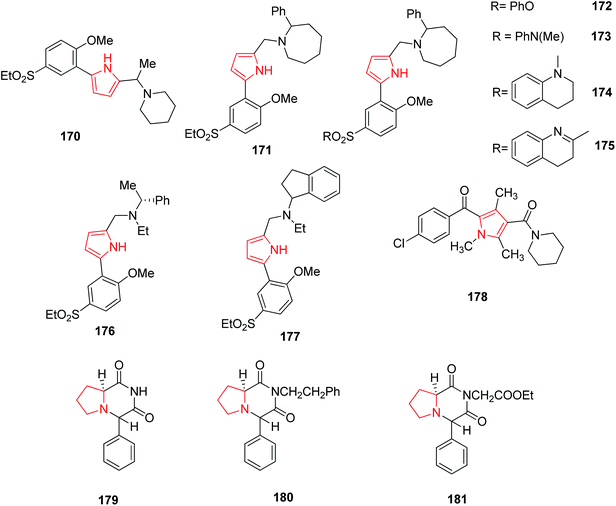 |
| Fig. 4 Pyrrole containing compounds with antipsychotic and anticonvulsant activity. | |
3.5. Antifungal and antibacterial activity
Pyrrolomycins are natural antibiotics and contain nitropyrrole nucleus which is stable and chemically reactive for antifungal activity. N-Alkylation of pyrrolomycin A 182 caused reduction of antimicrobial activity against Candida albicans and Trichophyton mentagophytes strains, but N-iodoalkylation caused an enhancement of the biological potency. N-(iodopropargyl) pyrrolomycin A 183 and N-(triiodoallyl) pyrrolomycin A 184 derivative exhibited more antifungal activity than pyrrolomycin A and a known antifungal agent i.e. clotrimazole.103 The compounds with antifungal and antibacterial activity have been presented in Fig. 5.
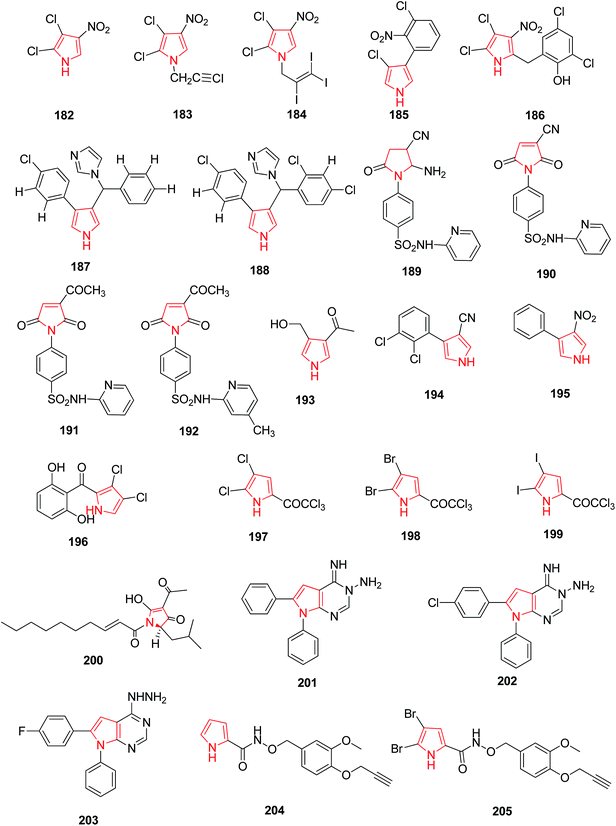 |
| Fig. 5 Pyrrole containing compounds with antifungal and antibacterial activity. | |
Pyrrolnitrin 185 and Pyrrolomycim B 186 are synthetic pyrroles that possess antifungal activity, indicating that the bulky substituents on the pyrrole ring weakens the activity whereas nitro group has a potential enhancing affect. It was further concluded that the antifungal activity of nitropyrrole was due to electro-negativity offered by nitro group transmitted through the pyrrole ring. The compounds 3-aryl-4-[α-(1H-imidazol-l-yl) arylmethyllpyrroles, when related to bifonazole and pyrrolnitrin were discovered as a new class of potential antifungal agents. Among the 3-aryl pyrrole derivatives that contains an (arylmethyl) imidazole moiety, two derivatives 187 and 188 (Fig. 5) were found to be highly active in vitro against C. albicans.104 Sulfonamide is well known to possess a variety of biological activities. The introduction of a heterocyclic sulfonamide in the pyrrole ring increases antifungal activity. On the other hand, lack of any substitutent of sulfonamide caused partial or complete reduction in antifungal activity. Among a series of sulfonamide containing pyrroles, compounds 189, 190, 191 and 192 (Fig. 5) exhibited a remarkable antifungal activity compared with the standard fungicide mycostatine.105 Encyclopedia of organic reagents reported that pyrrole containing Verrucarine E 193 and Fenpiclonil 194 are therapeutically useful antibacterial compounds and presence of nitro group at position 3 in 3-nitro-4-phenyl-1H-pyrrole 195 increases the antibacterial activity of the compound. A naturally occurring halogenated pyrrole derivative i.e. pyoluteorin 196 was found to posses antibacterial activity. Synthesized 4,5-dihalopyrrole derivatives were also reported as potential antibacterial agents derived from the modification of naturally occurring antibiotic pyoluteorin. Substitution of trichloroacetylated pyrrole with chloro 197, bromo 198 and iodo 199 group led to the improvement in antibacterial activity.106 (R)-Reutericyclin,(2R)-4-acetyl-1,2-dihydro-5-hydroxy-2-(2-methyl-propyl)-1-[(2E)-1-oxodec-2-enyl]-3H-pyrrol-3-one 200 is a bactericidal natural compound with a trisubstituted tetramic acid moiety which acts against a broad spectrum of Gram-positive bacteria.107 Hilmy et al. synthesized a series of new pyrrole derivatives and pyrrolo[2,3-d]pyrimidine derivatives. Compounds 201, 202 and 203 (Fig. 5) displayed best antifungal activity against Staphylococcus aureus with MIC 0.31 μg mL−1 when compared with the standard drug ampicillin, with MIC 0.62 μg mL−1. These compounds also showed antibacterial activity against Gram negative Escherichia coli similar to the standard drug.108 A facile method was used for the design and synthesis of a series of novel pyrrole alkaloid analogs. Compounds 204 and 205 exhibited good antifungal activity against Psylla piricola at low dosage.109
3.6. Antiviral activity
Migawa et al. synthesized several heterocyclic analogs of antibiotic toyocamycin and tricyclic nucleoside triciribine. They reported that 4-amino-1-(β-D-ribofuranosyl) pyrrole [2,3-d] [1,2,3] triazine-5-carboxamidrazone 206 and 4-amino-1-(β-D-ribofuranosyl) pyrrole [2,3-d] [1,2,3] triazine-5-carboxamidoxime 207 (Fig. 6) were active against Human Cytomegalo Virus (HCMV) and Herpes Simplex Virus type I (HSV–I) but their activity was poorly separated from cytotoxicity.110 In another study, 1-arylsulfonyl-1H-pyrroles were identified as a novel class of non-nucleoside HIV-1 reverse transcriptase inhibitors because of the presence of a specific chemical feature i.e. diarylcarbinol moiety which correlated with anti HIV-1 activity. Among the synthesized derivatives, pyrrolyl aryl sulfones (PAS) 208 and 1-benzenesulfonyl-3-(α-hydroxy-2,4-dichlorobenzyl) pyrrole 209 showed the highest activity when tested in MT-4 cells infected with HIV-1.111 SAR studies conducted on PAS derivatives revealed that the presence of a p-chloroaniline moiety and an ethoxycarbonyl group at position 2 of the pyrrole nucleus led to enhanced activity.112 The new substituent on the amino group at position 2 of the aryl moiety led to the formation of acylamino pyrryl aryl sulfones (APASs) 210 which resulted as active as PAS. Further substitutions were done to synthesize PAS derivatives among which the highest antiviral activity within the series was found to be 2-methylpropyl (sec-butyl) ester of 1-[(2-amino-5 chlorophenyl)sulfonyl]-1H-pyrrole-2-carboxylic acid 211 (Fig. 6).113 The SAR performedin the central core of NS5B polymerase inhibitors led to the discovery of a novel series of thieno[3,2-b]pyrroles that are potentallosteric inhibitors of the Hepatitis C virus (HCV) NS5B RNA-dependent polymerase. Introduction of a polar substituent led to compound 212 which efficiently blocked subgenomic HCV RNA replication in HUH-7 cells at low micromolar concentration.114 The chemical structures of compounds with antiviral activity have been shown in Fig. 6.
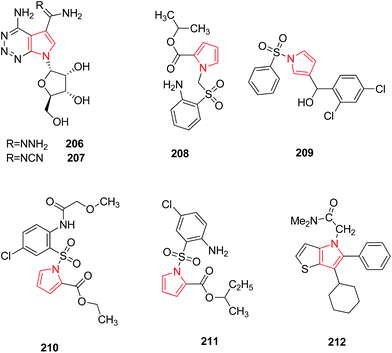 |
| Fig. 6 Pyrrole containing compounds with antiviral activity. | |
3.7. Antimycobacterial activity
A pyrrole derivative BM212 213 (Fig. 7) as the most active and potential antimycobacterial agent, was also found active against drug resistant mycobacteria of clinical origin including strains resistant to Etambutol, Isoniazid and also against Candida albicans but also found to posses cytotoxicity. To improve the antimycobacterial activity and to reduce cytotoxicity, various derivatives of BM212 were prepared. Among them, 4-substituted pyrrole with N-methyl piperaginyl 214 was found more active against Candida species as compared to thiomorpholinyl containing derivative 215.115 A program was further followed to systematically modify BM212 which led to individuate the importance of the substitutions at C5, N1 and C3. The microbiological results showed the importance of the presence of the thiomorpholine at C3 of the pyrrole and the p-chlorophenyl substituent in N1 and C5. The introduction of p-fluorophenyl at N1, phenyl at C5 and thiomorpholone at C3 216 was found to be more active than the corresponding derivatives.116 To establish the role of the pyrrole ring as a pharmacophoric group and hence, its influence on the antimycobacterial activity, 3-D-QSAR studies were undertaken on a set of pyrrole derivatives. As no information regarding their putative receptor was available, classical quantitative structure–activity relationships (QSAR) and comparative molecular field analysis (CoMFA) were used to correlate the anti-mycobacterial activity of compounds against M. tuberculosis. Among the synthesized compounds using QSAR studies, compound 217 (Fig. 7) showed the highest potency.117
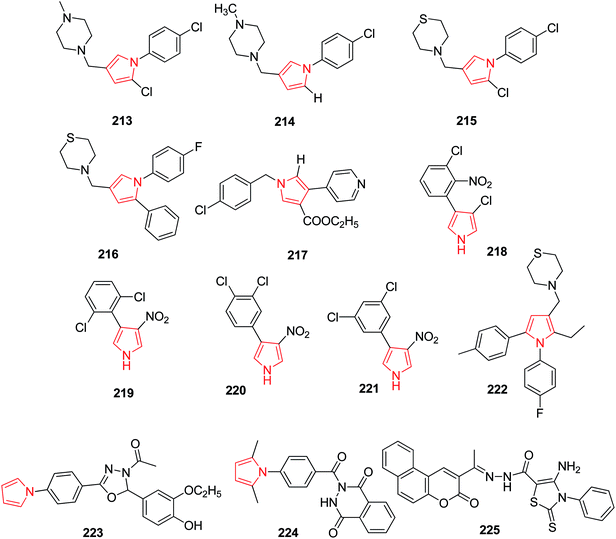 |
| Fig. 7 Pyrrole containing compounds with antimycobacterial activity. | |
Pyrrolnitrin, 218 a natural antibiotic used in topical antifungal diseases, was found to possess antimycobacterial activity also. Keeping in view its structure, derivatives of 4-aryl-3-nitropyrrole were prepared and evaluated for antimycobacterial activity. Three among other synthesized derivatives, 219, 220 and 221 (Fig. 7) showed appreciable activity against M. tuberculosis.118 The new diarylpyrroles were designed and synthesized via structure–activity relationship analysis of the already designed pyrroles. Compound 222 was found to be most active antitubercular agent having better MIC and PI value than the reference compound, moreover it displayed very low cytotoxicity. This compound was found active against both MTB H37 Rv and MTB rifampicin-resistant strains, with MIC value of 0.25 μg mL−1 in both cases.21 Joshi et al. synthesized a new series of pyrrolyl-Schiff base derivatives. The in vitro synthesis revealed that compounds 223 and 224 exhibited promising antitubercular activity with less toxicity. On assessment of these compounds against mammalian vero cell lines and A549 (lung adeno carcinoma) cell lines, it was found that these compounds were active at non-cytotoxic concentrations. By molecular modeling and docking studies, it was revealed that 224 interacted with InhA enzyme more efficiently.119 While exploring the benzochromenone based pyrrole derivatives, Refat et al. identified a novel compound 225 with significant antimicrobial activity. This compound was highly active against all the tested bacteria, due to the presence of electron withdrawing group attached to the benzochromenone ring.120
3.8. Antitumor activity
Roseophilin 226 and Prodigiosins 227 are the natural products of pyrrolo-alkaloids that exhibit a broad range of activity. Furstner reported that roseophilin exhibits higher cytotoxicity against several cancer cells. In vivo studies suggests that prodigiosins acts synergistically with cyclosporine A which is a reference immunosuppressive agent.121 A series of synthesized aryl pyrroles were evaluated in vivo and in vitro for antitumor activity among which [1-{[1-(1,3-benzodoxol-5-ylmethyl)-1H-imidazole-5-yl]-methyl}-4-(1-naphthyl)-1H-pyrrol-3-yl](4-methyl-1-piprazyryl) methanone (LB42908) 228 was found as a highly active antitumor agent, and currently undergoing in preclinical studies as inhibitor of RAS farnesyl transferase (FTase).6 Cdc7 serine/threonine kinase is a key regulator of DNA synthesis in eukaryotic organisms. Cdc7 inhibition through siRNA or prototype small molecules causes p53 independent apoptosis in tumor cells while reversibly arresting cell cycle progression in primary fibroblasts. This implies that Cdc7 kinase could be considered a potential target for anticancer therapy. A new chemical class of 5-heteroaryl-3-carboxamido-2-substituted pyrrole derivative 229 was synthesized by introducing a variety of substituents at position 2 of pyrrole ring. The compound 230 with phenyl substituent at 2 position of pyrrole and 2-amino-4-pyrimidin-4-yl chain at position 5 represented a novel prototype Cdc7 kinase inhibitor.122 Similarly, Siddiqui et al. synthesized compound 231 that showed promising anticancer activity against human leukemia cell line (HL-60) by MTT assay. The presence of [3′,5′-dimethylpyrazole-1-yl] carbonylmethoxy moiety attached at 3β position was responsible for this enhanced activity.123 Compounds with antitumor activity have been presented in Fig. 8.
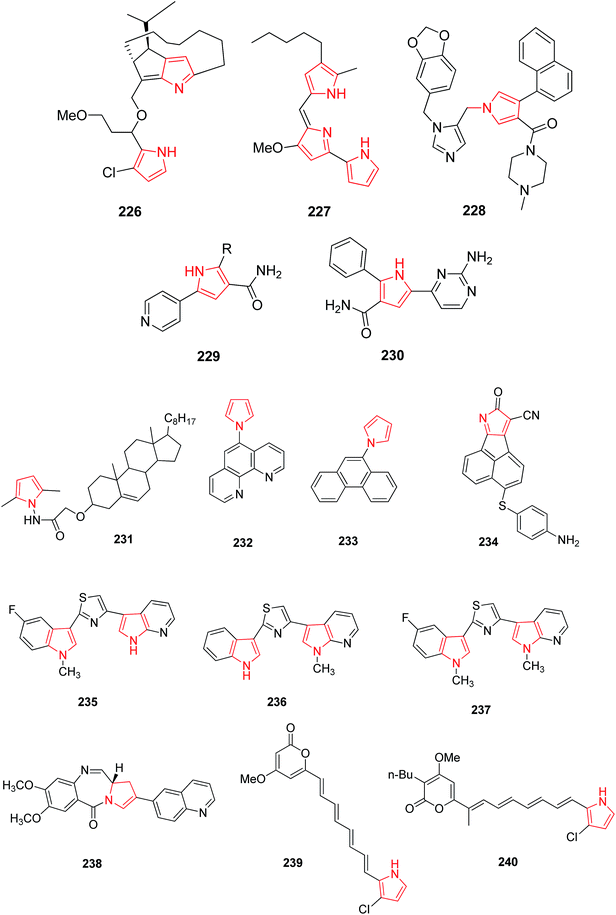 |
| Fig. 8 Pyrrole containing compounds with anti tumor activity. | |
The ultrasound assisted and bismuth nitrate catalyzed eco-friendly route was developed to synthesize a series of novel N-substituted pyrrole derivatives. Compounds 232 and 233 were highly cytotoxic against some cancer cell lines. When compared with normal hepatocytes in vitro, these compounds were selectively cytotoxic against hepatic cancer cell lines. The study suggested that N-substituted pyrrole exhibits different mechanism of cytotoxicity as compared to other polyaromatic derivatives.25 The dual inhibitors of Bcl-2 and Mcl-1,3-thiomorpholine-8-oxo-8H-acenaphtho [1,2-b] pyrrole-9-carbonitrile were developed. The novel dual inhibitor 234 was obtained by various SAR studies to exploit the difference in the p2 binding pocket of Bcl-2 and Mcl-1. This compound was more effective, having 10 fold lower IC50 as compared to other synthesized compounds that enhanced the affinity to Mcl-1 as well as maintained the affinity to Bcl-2.124 The novel 1H-pyrrolo [2,3-b]pyridine derivatives were synthesized for the treatment of DMPM. Compounds 235, 236 and 237 (Fig. 8) consistently reduced DMPM cell proliferation by inducing a caspase-dependent apoptotic response with a concomitant reduction of the expression of active Thr34-phosphorylated form of the anti-apoptotic protein surviving.125 The SAR investigation studies were done on the C2-position of PBD monomer antitumor agents. Compound 238 delayed tumor growth in HCT-116 (bowel) human tumor xenograft model. The study demonstrated that the cytotoxicity and DNA binding affinity of PBD conjugates can be enhanced by introducing C2-quinolinyl substituent. Moreover, this compound delayed tumor growth in HCT-116 colon cancer xenographt model without causing weight loss or other adverse effects, which suggested that C2-aryl PBD monomers be used as potential agents in the treatment of human disease.126
A hit compound identified in a fungus was used to design a class of polyenyl pyrroles and their analogues. Two compounds 239 and 240 exhibited potential cytotoxicity against human non-small cell lung carcinoma cell lines A549 with IC50 of 0.6 and 0.01 μM, respectively. Anticancer activity of compounds could be attributed to the induction of caspases activation dependent apoptosis through loss of mitochondrial membrane potential, followed by release of cytochrome-c and increase in B-cell lymphoma-2-associated X protein (Bax) level, and decrease in B-cell lymphoma-2 (Bcl-2) level.127
3.9. Histone deacetylase inhibitors
SAR studies performed on some portions (pyrrole-C4, pyrrole-N1, and hydroxamate group) of 3-(4-benzoyl-1-methyl-1H-pyrrol-2-yl)-N-hydroxy-2-propenamide highlighted its 4-phenylacetyl 241 and 4-cynnamoyl 242 analogues as more active compounds as HD2 active inhibitors in vitro. Other homologues of 241 were prepared by varying hydrocarbon spacer length ranging from two to five methylenes between benzene and carbonyl groups at the pyrrole C4 position. Compounds with two methylenes 243 and five methylenes 244 were most potent while the introduction of higher number of methylene units decreased the inhibitory activities of derivatives.128 Aroyl-pyrrolyl-hydroxy-amides (APHAs) are a class of synthetic HDAC inhibitors. Their derivatives were prepared by applying chemical modifications on the benzoyl moiety among which 245 and 246 were found to inhibit class IIb and class I histone deacetylase inhibitors.129 The chemical structures have been provided in Fig. 9.
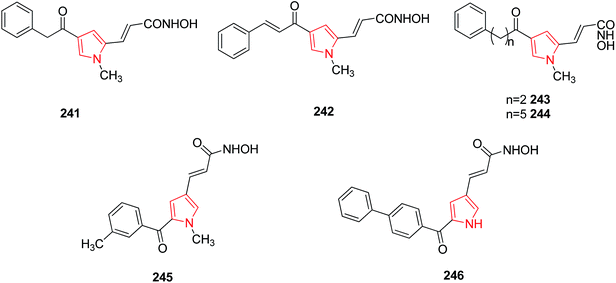 |
| Fig. 9 Pyrrole containing compounds as Histone deacetylase inhibitors. | |
3.10. CDK inhibitors (CDKs)
Wang et al. reported that various substitutions on 2-anilino-4-(1H-pyrrol-3-yl) pyrimidine showed inhibition of cyclic dependent kinase enzyme which is key regulators of cell cycle progression. The introduction of a nitrile group at position 5 of the pyrrole ring 247 found to possess 10-fold higher cellular activity as compared to halogenated derivative 248 against tumor cells.130 Hymenialdisine, a marine natural product, was originally isolated from the sponges Axinella verrucosa and Acantella aurantiaca and found to possess inhibitory activity against members of CDK family. The inhibition activity of diacetyl hymenialdisine 249 is 2-fold higher against CDK5 than that of debromodiacetyl hymenialdisine 250 (Fig. 10).
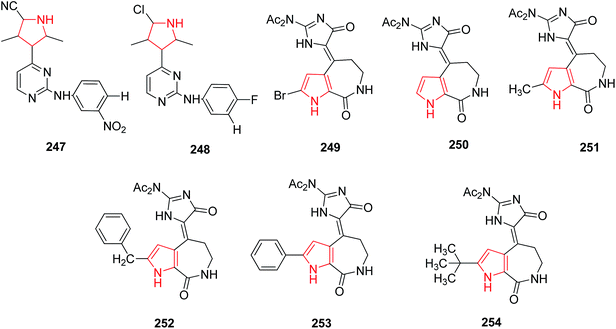 |
| Fig. 10 Pyrrole containing compounds as CDK inhibitors. | |
X-ray structure of hymenialdisine-CDK2 complex reveals some hydrophobic interaction between the bromine atom and the hydrophobic backbone of CDK thus showing the key role of bulky and lipophilic effects of the bromo atom of hymenialdisine against CDK inhibition. To further understand its role, various 2-substituted endo-hymenialdisine derivatives were synthesized by substituting the bromo atom with methyl 251, benzyl 252, phenyl 253 and tert-butyl groups 254 (Fig. 10). Thus, a variety of derivatives with substitutions at the α-position of the pyrrolyl ring were obtained with promising kinase inhibitory activity.131
3.11. Monoamine oxidase inhibitors
A series of substituted pyrrolylethanoneamine derivatives were evaluated for monoamine oxidase enzyme inhibition activity and SAR studies revealed that aminoketone derivative 255 (Fig. 11) was potentially active and selective inhibitor of MAO-A enzyme where as methylation of this compound results in N,N-dimethylamino derivative 256 which was less potent.132 Bruyne et al. demonstrated the study of radio labelling of [11C]-labelled pyrrole-2-carboxamide derivative 257 and its in vivo properties were categorized. Specific binding was observed in MAO-A when blocking and imaging study was performed. It was observed by in vivo studies that this compound penetrated rapidly in the brain, followed by an efficient washout.133 A novel series of 5-substituted 3-(1-alkyl-1H-pyrrol-3-yl)-2-oxazolidinones were synthesized as reversible, highly active and selective MAO-A inhibitors. Compound 258 showed 380 times higher MAO-A inhibitory activity with respect to toloxatone. When compared to befloxatone, this compound showed similar MAO-A activity but there was an increase in A-selectivity ratio.134
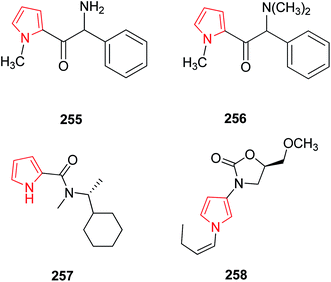 |
| Fig. 11 Pyrrole containing compounds as monoamine oxidase inhibitors. | |
3.12. EGFR tyrosine kinases inhibitors
Over expression of the epidermal growth factor-R tyrosine kinase has been implicated in many disease indications such as tumor and psoriasis. A series of 4,5-disubstitued-5,7-dihydropyrrole [2,3-d] pyrimidine-6-ones were found as potentially active and selective inhibitors of the EGFR tyrosine kinase family. The core molecule (5,7-diazaindolinone) without substitution at the C-3 position 259 was inactive against any kinases where as condensation of core with substituted pyrrole-2-carboxaldehyde 260 was observed to be active against the EGF-R kinase.135 The novel series of chiral and non-chiral 4-N-substituted 6-aryl-pyrrolo pyrimidines were synthesized and tested for their in vitro EGFR-TK inhibitor properties. Compound 261 (Fig. 12) was found to be most active EGFR-TK inhibitor with IC50 of 2.0.136
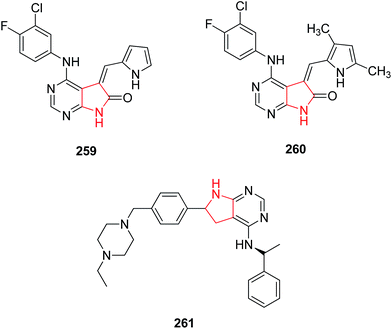 |
| Fig. 12 Pyrrole containing compounds as EGFR inhibitors. | |
3.13. As diagnostic and therapeutic agents
Bacteriochlorins bear a germinal dimethyl group in each pyrroline ring and a symmetrically branched 1,5-dimethoxypentyl group is also attached to each pyrrole ring. Both these groups are well required for the stability and solubility in lipophilic media. Bacteriochlorins absorb strongly in near infra red spectral region and thus, are best suited for photo-medical purposes including optical imaging and photodynamic therapy (PDT). As they possess diverse applications in photo-medicine, they were synthesized synthetically from swallowtail dihydropyrrin which in turn were obtained from swallowtail pyrrole. The swallowtail groups include all hydrocarbon units that afford increased solubility in organic media and polar terminated analogues (e.g. 1,5-diphosphonopent-3-yl) that afford solubility in aqueous media. The synthesized swallowtail bacteriochlorins 262 and 263 (Fig. 13) display strong absorption bands in UV.30 The natural pigment bilirubin, is a bichromophoric structure comprising two Z-dipyrrinones bearing intramolecularly hydrogen-bonded propionic acid. Bilirubin and its analogues are useful probes for hepatobiliary dysfunction. Based on their structure, fluorinated fluorescent dipyrrinones were synthesized. The fluorinated and sulphonated N,N′-carbonyl-bridged dipyrrinones 264 and 265 as their sodium salts possess strong fluorescence and thus used as 19F MRI imaging agents for use in probing liver and biliary metabolism.137
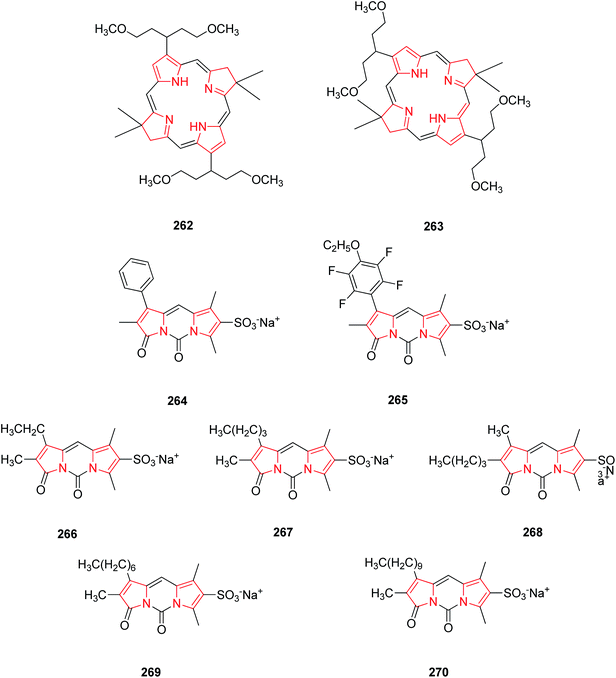 |
| Fig. 13 Pyrrole containing compounds as diagnostic and therapeutic agents. | |
A new class of highly fluorescent and low molecular weight water soluble sulphonated N,N′-carbonyldipyrrinone (3H,5H-dipyrrolo[1,2-c:2′,1′-f] pyrimidine-3,5-dione) derivatives 266–270 were synthesized and isolated as their sodium salts. The alkyl substituent of the lactam ring was lengthened from ethyl to decyl showing an increase in lipophilicity thus excreted more selectively in bile. They are thus useful in clinical diagnosis as they will appear in urine when hepatic elimination is impaired by cholestatic liver disease.33
4. Conclusion
Conclusively, the synthesis of pyrrole analogs is still an active field in medicinal research and development industries. Various new methods are being employed for its preparation and it will continue to be an important area for research in future. By presenting this review, we hope that the scientific community will be beneficial in developing new synthetic routes for the preparation of this resourceful heterocyclic system with better biological outcomes.
Conflict of interest
The authors declare no conflict of interest.
References
- F. Hartner Jr, A. Katritzky, C. Rees and E. Scriven, Comprehensive Heterocyclic Chemistry II, ed. I. Shinkai, Pergamon, Oxford, 1996, 3, p. 4 Search PubMed.
- R. A. Jones, Pyrroles: The synthesis and the physical and chemical aspects of the pyrrole ring, Wiley-Interscience, 1990 Search PubMed.
- H. Nakano, S. Umio, K. Kariyone, K. Tanaka, T. Kishimoto, H. Noguchi, I. Ueda, H. Nakamura and T. Morimoto, Tetrahedron Lett., 1966, 7, 737–740 CrossRef CAS.
- W. W. Wilkerson, R. A. Copeland, M. Covington and J. M. Trzaskos, J. Med. Chem., 1995, 38, 3895–3901 CrossRef CAS.
- R. P. Wurz and A. B. Charette, Org. Lett., 2005, 7, 2313–2316 CrossRef CAS PubMed.
- H. Lee, J. Lee, S. Lee, Y. Shin, W. Jung, J.-H. Kim, K. Park, K. Kim, H. S. Cho and S. Ro, Bioorg. Med. Chem. Lett., 2001, 11, 3069–3072 CrossRef CAS.
- C. Piliego, T. W. Holcombe, J. D. Douglas, C. H. Woo, P. M. Beaujuge and J. M. Fréchet, J. Am. Chem. Soc., 2010, 132, 7595–7597 CrossRef CAS PubMed.
- A. R. Katritzky, P. Barczynski, G. Musumarra, D. Pisano and M. Szafran, J. Am. Chem. Soc., 1989, 111, 7–15 CrossRef CAS.
- B. P. Etherton, R. Krishnamurti and S. Nagy, U.S. Patent no. 5,554,775, 1996.
- V. J. Gelling, M. M. Wiest, D. E. Tallman, G. P. Bierwagen and G. G. Wallace, Prog. Org. Coat., 2001, 43, 149–157 CrossRef CAS.
- R. Advincula and R. B. Pemites, Types of electrodeposited polymer coatings with reversible wettability and electro-optical properties, US Patent Application 13/179,515, 2011.
- A. Kałędkowski and A. W. Trochimczuk, React. Funct. Polym., 2006, 66, 740–746 CrossRef PubMed.
- R. D. Rieth, N. P. Mankad, E. Calimano and J. P. Sadighi, Org. Lett., 2004, 6, 3981–3983 CrossRef CAS PubMed.
- A. Deronzier and J.-C. Moutet, Coord. Chem. Rev., 1996, 147, 339–371 CrossRef CAS.
- Y. Zhu, A. Rabindranath, T. Beyerlein and B. Tieke, Macromolecules, 2007, 40, 6981–6989 CrossRef CAS.
- V. Sreenivasan, in Developments in Applied Spectroscopy, Springer, 1971, pp. 217–234 Search PubMed.
- D. O’Hagan, Nat. Prod. Rep., 2000, 17, 435–446 RSC.
- R. Chinchilla, C. Nájera and M. Yus, Chem. Rev., 2004, 104, 2667–2722 CrossRef CAS PubMed.
- M. S. Mohamed, R. Kamel and S. S. Fatahala, Eur. J. Med. Chem., 2011, 46, 3022–3029 CrossRef CAS PubMed.
- G. H. Jana, S. Jain, S. K. Arora and N. Sinha, Bioorg. Med. Chem. Lett., 2005, 15, 3592–3595 CrossRef CAS PubMed.
- M. Biava, G. C. Porretta, G. Poce, A. De Logu, R. Meleddu, E. De Rossi, F. Manetti and M. Botta, Eur. J. Med. Chem., 2009, 44, 4734–4738 CrossRef CAS PubMed.
- K. Liu, H. Lu, L. Hou, Z. Qi, C. Teixeira, F. Barbault, B.-T. Fan, S. Liu, S. Jiang and L. Xie, J. Med. Chem., 2008, 51, 7843–7854 CrossRef CAS PubMed.
- X.-Y. He, P. Zou, J. Qiu, L. Hou, S. Jiang, S. Liu and L. Xie, Bioorg. Med. Chem., 2011, 19, 6726–6734 CrossRef CAS PubMed.
- M. B. Wallace, M. E. Adams, T. Kanouni, C. D. Mol, D. R. Dougan, V. A. Feher, S. M. O'Connell, L. Shi, P. Halkowycz and Q. Dong, Bioorg. Med. Chem. Lett., 2010, 20, 4156–4158 CrossRef CAS PubMed.
- D. Bandyopadhyay, S. Mukherjee, J. C. Granados, J. D. Short and B. K. Banik, Eur. J. Med. Chem., 2012, 50, 209–215 CrossRef CAS PubMed.
- I. Yavari, M. Ghazvini, L. Azad and T. Sanaeishoar, Chin. Chem. Lett., 2011, 22, 1219–1222 CAS.
- A. S. Girgis, J. Stawinski, N. S. Ismail and H. Farag, Eur. J. Med. Chem., 2012, 47, 312–322 CrossRef CAS PubMed.
- A. Goel, N. Agarwal, F. V. Singh, A. Sharon, P. Tiwari, M. Dixit, R. Pratap, A. K. Srivastava, P. R. Maulik and V. J. Ram, Bioorg. Med. Chem. Lett., 2004, 14, 1089–1092 CrossRef CAS PubMed.
- J. Qin, J. Zhang, B. Wu, Z. Zheng, M. Yang and X. Yu, Chin. J. Chem., 2009, 27, 1782–1788 CrossRef CAS.
- K. E. Borbas and J. S. Lindsey, Org. Lett., 2008, 10, 1931–1934 CrossRef CAS PubMed.
- O. V. Larionov and A. de Meijere, Angew. Chem., Int. Ed., 2005, 44, 5664–5667 CrossRef CAS PubMed.
- R. Di Santo, A. Tafi, R. Costi, M. Botta, M. Artico, F. Corelli, M. Forte, F. Caporuscio, L. Angiolella and A. T. Palamara, J. Med. Chem., 2005, 48, 5140–5153 CrossRef CAS PubMed.
- Z. R. Woydziak, S. E. Boiadjiev, W. S. Norona, A. F. McDonagh and D. A. Lightner, J. Org. Chem., 2005, 70, 8417–8423 CrossRef CAS PubMed.
- A. J. Airaksinen, M. Ahlgren and J. Vepsäläinen, J. Org. Chem., 2002, 67, 5019–5021 CrossRef CAS PubMed.
- N. D. Smith, D. Huang and N. D. Cosford, Org. Lett., 2002, 4, 3537–3539 CrossRef CAS PubMed.
- N. Azizi, A. Khajeh-Amiri, H. Ghafuri, M. Bolourtchian and M. R. Saidi, Synlett, 2009, 2009, 2245–2248 CrossRef PubMed.
- T. Maehara, R. Kanno, S. Yokoshima and T. Fukuyama, Org. Lett., 2012, 14, 1946–1948 CrossRef CAS PubMed.
- R. Martín, C. H. Larsen, A. Cuenca and S. L. Buchwald, Org. Lett., 2007, 9, 3379–3382 CrossRef PubMed.
- X. Yuan, X. Xu, X. Zhou, J. Yuan, L. Mai and Y. Li, J. Org. Chem., 2007, 72, 1510–1513 CrossRef CAS PubMed.
- I. Özdemir, N. Gürbüz, N. Kaloğlu, Ö. Doğan, M. Kaloğlu, C. Bruneau and H. Doucet, Beilstein J. Org. Chem., 2013, 9, 303–312 CrossRef PubMed.
- B. Eftekhari-Sis and S. Vahdati-Khajeh, Curr. Chem. Lett., 2013, 2, 85–92 CrossRef CAS.
- M. Jasiński, T. Watanabe and H. U. Reissig, Eur. J. Org. Chem., 2013, 2013, 605–610 CrossRef.
- V. Estévez, M. Villacampa and J. C. Menéndez, Chem. Commun., 2013, 49, 591–593 RSC.
- V. Estévez, M. Villacampa and J. C. Menéndez, Chem. Soc. Rev., 2014, 43, 4633–4657 RSC.
- P. Khulpe and S. Mohite, Int. J. Curr. Pharm. Res., 2014, 4, 1181–1185 CAS.
- J. S. Alford, J. E. Spangler and H. M. Davies, J. Am. Chem. Soc., 2013, 135, 11712–11715 CrossRef CAS PubMed.
- J. S. Alford and H. M. Davies, J. Am. Chem. Soc., 2014, 136, 10266–10269 CrossRef CAS PubMed.
- T. J. Blackburn, S. Ahmed, C. R. Coxon, J. Liu, X. Lu, B. T. Golding, R. J. Griffin, C. Hutton, D. R. Newell and S. Ojo, Med. Chem. Commun., 2013, 4, 1297–1304 RSC.
- Z. Chen, B. Lu, Z. Ding, K. Gao and N. Yoshikai, Org. Lett., 2013, 15, 1966–1969 CrossRef CAS PubMed.
- Q. Chong, X. Xin, C. Wang, F. Wu and B. Wan, Tetrahedron, 2014, 70, 490–494 CrossRef CAS PubMed.
- L. Ermolenko, H. Zhaoyu, C. Lejeune, C. Vergne, C. l. Ratinaud, T. B. Nguyen and A. Al-Mourabit, Org. Lett., 2014, 16, 872–875 CrossRef CAS PubMed.
- A. A. Fesenko and A. D. Shutalev, Tetrahedron Lett., 2014, 55, 1416–1420 CrossRef CAS PubMed.
- B. Gabriele, L. Veltri, P. Plastina, R. Mancuso, M. V. Vetere and V. Maltese, J. Org. Chem., 2013, 78, 4919–4928 CrossRef CAS PubMed.
- S. Paul, G. Pal and A. R. Das, RSC Adv., 2013, 3, 8637–8644 RSC.
- M. Zhang, H. Neumann and M. Beller, Angew. Chem., 2013, 125, 625–629 CrossRef.
- M. Gao, C. He, H. Chen, R. Bai, B. Cheng and A. Lei, Angew. Chem., 2013, 125, 7096–7099 CrossRef.
- H. Zheng, Q. Shi, K. Du, Y. Mei and P. Zhang, Mol. Diversity, 2013, 17, 245–250 CrossRef CAS PubMed.
- K. Murugan and S.-T. Liu, Tetrahedron Lett., 2013, 54, 2608–2611 CrossRef CAS PubMed.
- W. Chen and J. Wang, Organometallics, 2013, 32, 1958–1963 CrossRef CAS.
- X. Wang, X.-P. Xu, S.-Y. Wang, W. Zhou and S.-J. Ji, Org. Lett., 2013, 15, 4246–4249 CrossRef CAS PubMed.
- X. Feng, Q. Wang, W. Lin, G.-L. Dou, Z.-B. Huang and D.-Q. Shi, Org. Lett., 2013, 15, 2542–2545 CrossRef CAS PubMed.
- P. Xu, K. Huang, Z. Liu, M. Zhou and W. Zeng, Tetrahedron Lett., 2013, 54, 2929–2933 CrossRef CAS PubMed.
- D. J. Viradiya, B. H. Baria, R. Kakadiya, V. C. Kotadiya and A. Shah, Int. Lett. Chem., Phys. Astron., 2014, 11, 265–276 Search PubMed.
- X.-B. Chen, X.-Y. Wang, D.-D. Zhu, S.-J. Yan and J. Lin, Tetrahedron, 2014, 70, 1047–1054 CrossRef CAS PubMed.
- M. Kobeissi, O. Yazbeck and Y. Chreim, Tetrahedron Lett., 2014, 55, 2523–2526 CrossRef CAS PubMed.
- X. Dou, B. Zhou, W. Yao, F. Zhong, C. Jiang and Y. Lu, Org. Lett., 2013, 15, 4920–4923 CrossRef CAS PubMed.
- B. Karami, S. Khodabakhshi and M. Jamshidi, J. Chin. Chem. Soc., 2013, 60, 1103–1106 CrossRef CAS.
- P. Radha Krishna Murthi, D. Rambabu, M. Basaveswara Rao and M. Pal, Tetrahedron Lett., 2014, 55, 507–509 CrossRef CAS PubMed.
- Y. Li, Q.-Y. Li, H.-W. Xu, W. Fan, B. Jiang, S.-L. Wang and S.-J. Tu, Tetrahedron, 2013, 69, 2941–2946 CrossRef CAS PubMed.
- B.-L. Li, P.-H. Li, X.-N. Fang, C.-X. Li, J.-L. Sun, L.-P. Mo and Z.-H. Zhang, Tetrahedron, 2013, 69, 7011–7018 CrossRef CAS PubMed.
- L. Meng, K. Wu, C. Liu and A. Lei, Chem. Commun., 2013, 49, 5853–5855 RSC.
- H. Meshram, B. Madhu Babu, G. Santosh Kumar, P. B. Thakur and V. M. Bangade, Tetrahedron Lett., 2013, 54, 2296–2302 CrossRef CAS PubMed.
- S. Michlik and R. Kempe, Nat. Chem., 2013, 5, 140–144 CrossRef CAS PubMed.
- M.-N. Zhao, Z.-H. Ren, Y.-Y. Wang and Z.-H. Guan, Org. Lett., 2014, 16, 608–611 CrossRef CAS PubMed.
- B. T. Parr, S. A. Green and H. M. Davies, J. Am. Chem. Soc., 2013, 135, 4716–4718 CrossRef CAS PubMed.
- B. S. Reddy, M. R. Reddy, Y. G. Rao, J. Yadav and B. Sridhar, Org. Lett., 2013, 15, 464–467 CrossRef CAS PubMed.
- E. K. Sadykov, V. Stankevich, N. Lobanova and G. Klimenko, Russ. J. Org. Chem., 2014, 50, 219–224 CrossRef CAS.
- R. Suresh, S. Muthusubramanian, M. Nagaraj and G. Manickam, Tetrahedron Lett., 2013, 54, 1779–1784 CrossRef CAS PubMed.
- J. M. Yang, C. Z. Zhu, X. Y. Tang and M. Shi, Angew. Chem., Int. Ed., 2014, 53, 5142–5146 CAS.
- M. Yurovskaya and R. Alekseyev, Chem. Heterocycl. Compd., 2014, 49, 1400–1425 CrossRef CAS PubMed.
- M. Zhan, S. Zhang, W.-X. Zhang and Z. Xi, Org. Lett., 2013, 15, 4182–4185 CrossRef CAS PubMed.
- L. Zhang, X. Wang, S. Li and J. Wu, Tetrahedron, 2013, 69, 3805–3809 CrossRef CAS PubMed.
- L. Zhao, C. Bruneau and H. Doucet, ChemCatChem, 2013, 5, 255–262 CrossRef CAS.
- N. Zhou, T. Xie, L. Liu and Z. Xie, J. Org. Chem., 2014, 79, 6061–6068 CrossRef CAS PubMed.
- X. Qian, G.-B. Liang, D. Feng, M. Fisher, T. Crumley, S. Rattray, P. M. Dulski, A. Gurnett, P. S. Leavitt and P. A. Liberator, Bioorg. Med. Chem. Lett., 2006, 16, 2817–2821 CrossRef CAS PubMed.
- G.-B. Liang, X. Qian, T. Biftu, D. Feng, M. Fisher, T. Crumley, S. J. Darkin-Rattray, P. M. Dulski, A. Gurnett and P. S. Leavitt, Bioorg. Med. Chem. Lett., 2005, 15, 4570–4573 CrossRef CAS PubMed.
- T. Biftu, D. Feng, M. Ponpipom, N. Girotra, G.-B. Liang, X. Qian, R. Bugianesi, J. Simeone, L. Chang and A. Gurnett, Bioorg. Med. Chem. Lett., 2005, 15, 3296–3301 CrossRef CAS PubMed.
- G.-B. Liang, X. Qian, D. Feng, M. Fisher, T. Crumley, S. J. Darkin-Rattray, P. M. Dulski, A. Gurnett, P. S. Leavitt and P. A. Liberator, Bioorg. Med. Chem. Lett., 2008, 18, 2019–2022 CrossRef CAS PubMed.
- J. M. Muchowski, S. H. Unger, J. Ackrell, P. Cheung, J. Cook, P. Gallegra, O. Halpern, R. Koehler and A. F. Kluge, J. Med. Chem., 1985, 28, 1037–1049 CrossRef CAS.
- W. W. Wilkerson, W. Galbraith, K. Gans-Brangs, M. Grubb, W. E. Hewes, B. Jaffee, J. Kenney, J. Kerr and N. Wong, J. Med. Chem., 1994, 37, 988–998 CrossRef CAS.
- I. K. Khanna, R. M. Weier, Y. Yu, P. W. Collins, J. M. Miyashiro, C. M. Koboldt, A. W. Veenhuizen, J. L. Currie, K. Seibert and P. C. Isakson, J. Med. Chem., 1997, 40, 1619–1633 CrossRef CAS PubMed.
- S. Maddila, S. Gorle, C. Sampath and P. Lavanya, J. Saudi Chem. Soc., 2012 DOI:10.1016/jscs. 2012.11.007.
- C. Battilocchio, G. Poce, S. Alfonso, G. C. Porretta, S. Consalvi, L. Sautebin, S. Pace, A. Rossi, C. Ghelardini and L. Di Cesare Mannelli, Bioorg. Med. Chem., 2013, 21, 3695–3701 CrossRef CAS PubMed.
- A. Hall, S. Atkinson, S. H. Brown, I. P. Chessell, A. Chowdhury, N. M. Clayton, T. Coleman, G. M. Giblin, R. J. Gleave and B. Hammond, Bioorg. Med. Chem. Lett., 2006, 16, 3657–3662 CrossRef CAS PubMed.
- A. Hall, S. H. Brown, I. P. Chessell, A. Chowdhury, N. M. Clayton, T. Coleman, G. M. Giblin, B. Hammond, M. P. Healy and M. R. Johnson, Bioorg. Med. Chem. Lett., 2007, 17, 732–735 CrossRef CAS PubMed.
- A. Hall, S. H. Brown, I. P. Chessell, A. Chowdhury, N. M. Clayton, T. Coleman, G. M. Giblin, B. Hammond, M. P. Healy and M. R. Johnson, Bioorg. Med. Chem. Lett., 2007, 17, 916–920 CrossRef CAS PubMed.
- A. Hall, S. Atkinson, S. H. Brown, I. P. Chessell, A. Chowdhury, G. M. Giblin, P. Goldsmith, M. P. Healy, K. S. Jandu and M. R. Johnson, Bioorg. Med. Chem. Lett., 2007, 17, 1200–1205 CrossRef CAS PubMed.
- D. Bolton, I. Boyfield, M. C. Coldwell, M. S. Hadley, M. A. Healy, C. N. Johnson, R. E. Markwell, D. J. Nash, G. J. Riley and G. Stemp, Bioorg. Med. Chem. Lett., 1996, 6, 1233–1236 CrossRef CAS.
- D. Bolton, I. Boyfield, M. C. Coldwell, M. S. Hadley, A. Johns, C. N. Johnson, R. E. Markwell, D. J. Nash, G. J. Riley and E. E. Scott, Bioorg. Med. Chem. Lett., 1997, 7, 485–488 CrossRef CAS.
- I. Boyfield, M. C. Coldwell, M. S. Hadley, M. A. Healy, C. N. Johnson, D. J. Nash, G. J. Riley, E. E. Scott, S. A. Smith and G. Stemp, Bioorg. Med. Chem. Lett., 1997, 7, 327–330 CrossRef CAS.
- J. R. Carson, R. J. Carmosin, P. M. Pitis, J. L. Vaught, H. R. Almond, J. P. Stables, H. H. Wolf, E. A. Swinyard and H. S. White, J. Med. Chem., 1997, 40, 1578–1584 CrossRef CAS PubMed.
- M. Dawidowski, F. Herold, A. Chodkowski, J. Kleps, P. Szulczyk and M. Wilczek, Eur. J. Med. Chem., 2011, 46, 4859–4869 CrossRef CAS PubMed.
- M. Koyama, N. Ohtani, F. Kai, I. Moriguchi and S. Inouye, J. Med. Chem., 1987, 30, 552–562 CrossRef CAS.
- M. Artico, R. Di Santo, R. Costi, S. Massa, A. Retico, M. Artico, G. Apuzzo, G. Simonetti and V. Strippoli, J. Med. Chem., 1995, 38, 4223–4233 CrossRef CAS.
- M. El-Gaby, A. Gaber, A. Atalla and K. Abd Al-Wahab, Il Farmaco, 2002, 57, 613–617 CrossRef CAS.
- D. M. Bailey and R. E. Johnson, J. Med. Chem., 1973, 16, 1300–1302 CrossRef CAS.
- R. Böhme, G. Jung and E. Breitmaier, Helv. Chim. Acta, 2005, 88, 2837–2841 CrossRef.
- K. M. H. Hilmy, M. Khalifa, M. A. Allah Hawata, R. M. A. A. Keshk and A. A. El-Torgman, Eur. J. Med. Chem., 2010, 45, 5243–5250 CrossRef PubMed.
- M.-Z. Wang, H. Xu, T.-W. Liu, Q. Feng, S.-J. Yu, S.-H. Wang and Z.-M. Li, Eur. J. Med. Chem., 2011, 46, 1463–1472 CrossRef CAS PubMed.
- M. T. Migawa, J. C. Drach and L. B. Townsend, J. Med. Chem., 2005, 48, 3840–3851 CrossRef CAS PubMed.
- M. Artico, R. D. Santo, R. Costi, S. Massa, F. Scintu, A. G. Loi, A. De Montis and P. La Colla, Bioorg. Med. Chem. Lett., 1997, 7, 1931–1936 CrossRef CAS.
- M. Artico, R. Silvestri, S. Massa, A. G. Loi, S. Corrias, G. Piras and P. La Colla, J. Med. Chem., 1996, 39, 522–530 CrossRef CAS PubMed.
- R. Silvestri, M. Artico, G. La Regina, G. De Martino, M. La Colla, R. Loddo and P. La Colla, Il Farmaco, 2004, 59, 201–210 CrossRef CAS PubMed.
- J. M. Ontoria, J. I. Martìn Hernando, S. Malancona, B. Attenni, I. Stansfield, I. Conte, C. Ercolani, J. Habermann, S. Ponzi and M. Di Filippo, Bioorg. Med. Chem. Lett., 2006, 16, 4026–4030 CrossRef CAS PubMed.
- M. Biava, R. Fioravanti, G. C. Porretta, D. Deidda, C. Maullu and R. Pompei, Bioorg. Med. Chem. Lett., 1999, 9, 2983–2988 CrossRef CAS.
- M. Biava, G. C. Porretta, D. Deidda, R. Pompei, A. Tafi and F. Manetti, Bioorg. Med. Chem., 2004, 12, 1453–1458 CrossRef CAS PubMed.
- R. Ragno, G. R. Marshall, R. Di Santo, R. Costi, S. Massa, R. Rompei and M. Artico, Bioorg. Med. Chem., 2000, 8, 1423–1432 CrossRef CAS.
- R. Di Santo, R. Costi, M. Artico, S. Massa, G. Lampis, D. Deidda and R. Pompei, Bioorg. Med. Chem. Lett., 1998, 8, 2931–2936 CrossRef CAS.
- S. D. Joshi, U. A. More, K. Pansuriya, T. M. Aminabhavi and A. K. Gadad, J. Saudi Chem. Soc., 2013 DOI:10.1016/jscs.2013.09.002.
- H. M. Refat and A. Fadda, Eur. J. Med. Chem., 2013, 70, 419–426 CrossRef CAS PubMed.
- A. Fürstner, Angew. Chem., Int. Ed., 2003, 42, 3582–3603 CrossRef PubMed.
- M. Menichincheri, C. Albanese, C. Alli, D. Ballinari, A. Bargiotti, M. Caldarelli, A. Ciavolella, A. Cirla, M. Colombo and F. Colotta, J. Med. Chem., 2010, 53, 7296–7315 CrossRef CAS PubMed.
- T. Siddiqui, M. G. Alam and A. M. Dar, J. Saudi Chem. Soc., 2012 DOI:10.1016/jscs.2012.04.009.
- Z. Zhang, G. Wu, F. Xie, T. Song and X. Chang, J. Med. Chem., 2011, 54, 1101–1105 CrossRef CAS PubMed.
- A. Carbone, M. Pennati, B. Parrino, A. Lopergolo, P. Barraja, A. Montalbano, V. Spanò, S. Sbarra, V. Doldi and M. De Cesare, J. Med. Chem., 2013, 56, 7060–7072 CrossRef CAS PubMed.
- D. Antonow, M. Kaliszczak, G.-D. Kang, M. Coffils, A. C. Tiberghien, N. Cooper, T. Barata, S. Heidelberger, C. H. James and M. Zloh, J. Med. Chem., 2010, 53, 2927–2941 CrossRef CAS PubMed.
- Z. Fang, P.-C. Liao, Y.-L. Yang, F.-L. Yang, Y.-L. Chen, Y. Lam, K.-F. Hua and S.-H. Wu, J. Med. Chem., 2010, 53, 7967–7978 CrossRef CAS PubMed.
- A. Mai, S. Massa, I. Cerbara, S. Valente, R. Ragno, P. Bottoni, R. Scatena, P. Loidl and G. Brosch, J. Med. Chem., 2004, 47, 1098–1109 CrossRef CAS PubMed.
- A. Mai, S. Valente, A. Nebbioso, S. Simeoni, R. Ragno, S. Massa, G. Brosch, F. De Bellis, F. Manzo and L. Altucci, Int. J. Biochem. Cell Biol., 2009, 41, 235–247 CrossRef CAS PubMed.
- S. Wang, G. Wood, C. Meades, G. Griffiths, C. Midgley, I. McNae, C. McInnes, S. Anderson, W. Jackson and M. Mezna, Bioorg. Med. Chem. Lett., 2004, 14, 4237–4240 CrossRef CAS PubMed.
- Q. He, W. Chen and Y. Qin, Tetrahedron Lett., 2007, 48, 1899–1901 CrossRef CAS PubMed.
- R. Di Santo, R. Costi, A. Roux, M. Artico, O. Befani, T. Meninno, E. Agostinelli, P. Palmegiani, P. Turini and R. Cirilli, J. Med. Chem., 2005, 48, 4220–4223 CrossRef CAS PubMed.
- S. De Bruyne, G. La Regina, S. Staelens, L. Wyffels, S. Deleye, R. Silvestri and F. De Vos, Nucl. Med. Biol., 2010, 37, 459–467 CrossRef CAS PubMed.
- S. Valente, S. Tomassi, G. Tempera, S. Saccoccio, E. Agostinelli and A. Mai, J. Med. Chem., 2011, 54, 8228–8232 CrossRef CAS PubMed.
- S.-S. Kang, H.-L. Li, H.-S. Zeng and H.-B. Wang, Acta Crystallogr., Sect. E: Struct. Rep. Online, 2008, 64, o1125 CAS.
- S. J. Kaspersen, C. Sørum, V. Willassen, E. Fuglseth, E. Kjøbli, G. Bjørkøy, E. Sundby and B. H. Hoff, Eur. J. Med. Chem., 2011, 46, 6002–6014 CrossRef CAS PubMed.
- S. E. Boiadjiev, Z. R. Woydziak, A. F. McDonagh and D. A. Lightner, Tetrahedron, 2006, 62, 7043–7055 CrossRef CAS PubMed.
|
This journal is © The Royal Society of Chemistry 2015 |