DOI:
10.1039/C4NJ00936C
(Paper)
New J. Chem., 2015,
39, 224-234
C5-curcuminoid-4-aminoquinoline based molecular hybrids: design, synthesis and mechanistic investigation of anticancer activity†
Received
(in Montpellier, France)
8th June 2014
, Accepted 15th October 2014
First published on 16th October 2014
Abstract
The privileged scaffolds of curcumin and 4-aminoquinolines are extensively used in the design and synthesis of biodynamic agents having remarkable efficacy against diseases like cancer and malaria. Therefore, we anticipated that covalent hybridization of these two pharmacophores via the triazole linker may lead to molecules with better anticancer activity. The synthesized hybrid compounds were tested for their anti-cancer activity on 60 human cancer cell lines, which represent diverse histologies. Our study has identified a set of these hybrids that showed excellent growth inhibition at nano-molar concentrations. The mechanistic investigations through a series of assays showed apoptotic induction as a cause for their displayed anticancer activity.
1. Introduction
Cancer is a leading cause of death worldwide, accounting for 7.6 million deaths in 2008 and expected to cross the figure of around 13 million deaths by 2030. About 70% of the cancer related deaths are mainly due to lung, colon, liver, stomach and breast cancers.1,2 It is well established that there is no single treatment for cancer and patients often receive a combination of therapies and palliative care, such as surgery, radiation, immunotherapy, chemotherapy or gene therapy, depending on the type and stage of cancer, the health status, age and personal characteristics.3 Anticancer drugs such as alkylating agents,4–8 antimetabolites,9–11 plant alkaloids,12–15 topoisomerase inhibitors16,17 and cytotoxic antibiotics18 have been used extensively in chemotherapy. Among these, natural products show good promise in the development of anticancer molecules19,20 and curcumin is one such natural product which has been extensively studied over the past few decades.21 These studies revealed that curcumin shows anticancer activity against prostate cancer,22 cervical cancer,23 colorectal carcinoma,24 leukemia25 and human breast cancer cells.26 The clinical use of curcumin has been hampered due to its poor solubility, absorption, bioavailability and rapid metabolism.27–30 The pharmacokinetic studies reveal that the β-diketone functionality of curcumin is a substrate for liver aldoketo reductases and this may be one of the reasons for its rapid in vivo metabolism.31 To overcome these limitations, several approaches have been explored and replacement of central diketo functionality with mono carbonyl has resulted in many compounds with improved anticancer activity, pharmacokinetic properties and bioavailability (Fig. 1).32–36
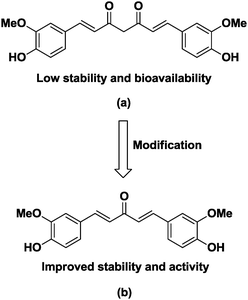 |
| Fig. 1 (a) Curcumin; (b) C5-curcuminoid. | |
In recent years, the concept of molecular hybrids has gained considerable importance due to the problem of drug resistance and it is anticipated that these ‘dual-drugs’ or ‘double-drugs’ synthesised by covalent hybridization of two or more pharmacophores may solve the problem of drug resistance.37–39 In this scenario, the synthesis of molecular hybrids by conjugating a C5-curcuminoid pharmacophore with other biologically active entities, especially those which are known for their anticancer activity may exhibit better anticancer properties in comparison to non-conjugated counterparts, has been reported.40 Quinoline is one such privileged pharmacophore that possesses an array of biological activities including antimalarial, anti-proliferative and antitumor activities. Among the many synthesized molecules containing the quinoline scaffold, camptothecin and its synthetic derivatives topotecan and irinotecan are well known for their antitumor activity and these compounds display their anticancer activity by inhibiting DNA enzyme topoisomerase I (Fig. 2).41 So, based on these observations and in continuation of our efforts of developing structurally diverse molecules of medicinal importance,42–45 we designed molecular hybrids by clubbing together C5-curcuminoids and quinoline nuclei by covalently linking them through the 1,2,3-triazole ring. Our rational for choosing the 1,2,3-triazole linker is based on the fact that triazole shows favourable physiochemical properties in the biological system and can be conveniently synthesized by click chemistry.46,47
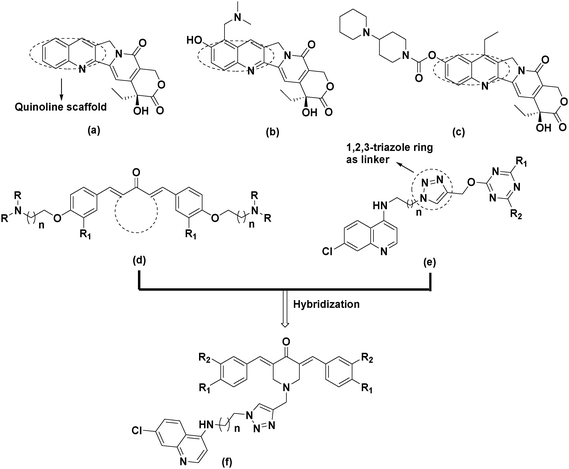 |
| Fig. 2 (a) Camptothecin; (b) topotecan; (c) irinotecan; (d) C5-curcuminoids and (e) 4-aminoquinoline based hybrid molecules reported previously from our research group; (f) C5-curcuminoid-4-aminoquinoline based hybrids synthesized in the present investigation. | |
2. Results and discussion
2.1 Chemistry
The targeted C5-curcuminoid-4-aminoquinoline based hybrids (7a–g and 8a–g) were synthesized as depicted in Scheme 1. To start with, firstly commercially available 4,7-dichloroquinoline (1) was reacted with linear chain aminoalcohols under neat conditions to yield 4-aminoquinolines with a free hydroxyl group at the terminal position (2a–b) in excellent yield.
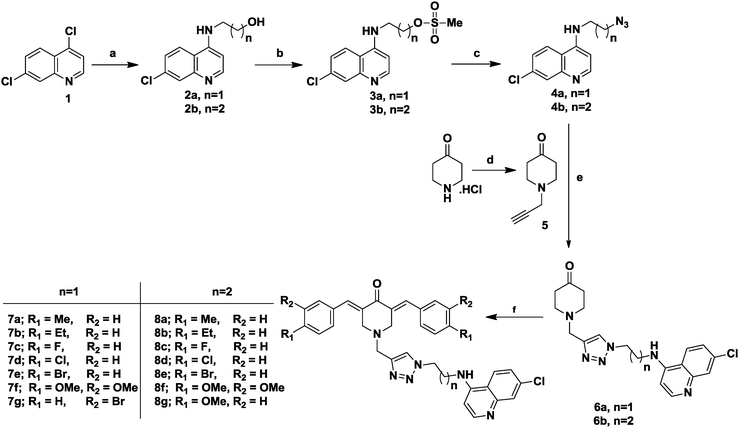 |
| Scheme 1 Reagents and conditions: (a) aminoalcohol, neat, 130–150 °C, under N2, 10–12 h; (b) MsCl, Et3N, THF, 0 °C-rt, under N2, 1 h; (c) NaN3, DMF, 50 °C, 4–5 h; (d) propargyl bromide, K2CO3, CHCl3, H2O, rt, 5–6 h; (e) t-butanol : H2O (1 : 1), CuSO4·5H2O, sodium ascorbate, 40–60 °C, 3 h; (f) Substituted benzaldehydes, 20% aq. NaOH (w/v), ethanol, rt, 4–5 h. | |
The free hydroxyl group was chemoselectively O-mesylated by the literature method45 using mesyl chloride and triethylamine as a base to yield mesylated 4-aminoquinolines (3a–b). Subsequently, the mesylated products (3a–b) were converted into corresponding azides (4a–b) using sodium azide as the nucleophile and DMF as solvent at 50 °C. These azides (4a–b) were reacted with 1-(prop-2-yn-1-yl)piperidin-4-one (5) by click chemistry using standard protocols of sodium ascorbate and copper sulfate as catalysts and an equimolar ratio of water and t-butanol as solvents to yield 1,2,3-triazole linker containing intermediates (6a–b). In the final step, the intermediates (6a–b) were subjected to aldol condensation with substituted benzaldehydes in the presence of NaOH as the base in ethanol to get the final targeted products (7a–g and 8a–g). It may be noted that, the intermediate compound 1-(prop-2-yn-1-yl)piperidin-4-one (5) was synthesized via nucleophilic substitution reaction between 4-piperidone hydrochloride monohydrate and propargyl bromide in the presence of anhydrous K2CO3 in the biphasic system [CHCl3
:
H2O (1
:
1)] at room temperature in good yield.
2.2 Biological studies
2.2.1 Anticancer activity.
In the present study, a series of C5-curcuminoid-4-aminoquinoline based molecular hybrids (7a–g and 8a–g) were synthesized and in order to explore the therapeutic potential of these hybrids, a representative subset of these compounds was screened for their growth inhibitory activity and cytotoxicity against 60 different human cancer cell lines. The screening was carried out by following a standard procedure described earlier.48 In the screening panel, 60 human cancer cell lines are organized into nine subpanels representing diverse histologies: leukemia, melanoma, and cancers of lung, colon, kidney, ovary, breast, prostate, and the central nervous system. The cells were grown in supplemented RPM1 1640 medium for 24 h. The test compounds were dissolved in DMSO and incubated with cells at five concentrations with 10-fold dilutions, the highest concentration being 10−4 M and the others 10−5, 10−6, 10−7, and 10−8 M. The assay was terminated by the addition of cold trichloroacetic acid, and the cells are fixed and stained with sulforhodamine B. The bound stain was solubilized, and the absorbance was read on an automated plate reader. The cytostatic parameter i.e. 50% growth inhibition (GI50) was calculated from time zero, control growth, and the five concentration level absorbance. The cytotoxic parameter i.e. inhibitory concentrations (LC50) represent the average of two independent experiments. The screening process was a two-stage process starting with the evaluation of the compound against the 60 human tumor cell lines with a single dose of 10.0 μM, which was carried out by following the same protocol as for five dose screening. Only the compound which showed more than 60% of growth inhibition in at least 8 tumor cell lines was selected for further testing and the others were assumed to be inactive. The initial testing at a single dose of 10 μM found compounds 7d and 7f causing significant inhibition of several cell lines. Both compounds were further tested for the dose–response effect at five concentration levels (100, 10, 1.0, 0.1 and 0.01 μM). The mean values for GI50 and LC50 on all 60 cell lines are given in Table 1. Additional data for both compounds i.e. one dose results, mean graphs, drug response curves, five dose mean graphs and GI50 and LC50 values are given in the ESI.† The dose–response curves of 7d and 7f for all 60 cell lines are illustrated in Fig. 3.
Table 1 Antitumor activity (GI50/μM)a and toxicity (LC50/μM)b data of compounds 7d and 7f selected for five dose studies for theNCI60-cell line screen
|
GI50 (μM) (7d) |
LC50 (μM) (7d) |
GI50 (μM) (7f) |
LC50 (μM) (7f) |
GI50: 50% Growth inhibition; concentration of the drug resulting in 50% reduction in the net protein increase compared with control cells.
LC50: lethal concentration; concentration of the drug lethal to 50% of the cells.
|
Leukemia |
CCRF-CEM |
0.309 |
>100 |
2.54 |
>100 |
HL-60(TB) |
0.373 |
>100 |
2.42 |
>100 |
K-562 |
0.317 |
>100 |
3.21 |
>100 |
MOLT-4 |
0.499 |
>100 |
3.50 |
>100 |
RPMI-8226 |
0.306 |
>100 |
3.00 |
>100 |
SR |
0.280 |
>100 |
2.04 |
>100 |
|
Non-small cell lung cancer |
A549/ATCC |
0.329 |
5.15 |
4.73 |
>100 |
HOP-62 |
0.316 |
3.31 |
3.90 |
>100 |
HOP-92 |
0.989 |
6.47 |
2.09 |
>100 |
NCI-H226 |
1.920 |
5.96 |
4.04 |
>100 |
NCI-H23 |
1.580 |
7.89 |
7.45 |
>100 |
NCI-H322M |
1.290 |
5.77 |
8.25 |
64.6 |
NCI-H460 |
0.250 |
3.79 |
2.22 |
87.2 |
NCI-H522 |
1.410 |
5.86 |
2.24 |
>100 |
|
Colon cancer |
COLO 205 |
0.354 |
3.35 |
1.99 |
7.65 |
HCC-2998 |
0.313 |
2.83 |
1.96 |
6.68 |
HCT-116 |
0.205 |
0.77 |
1.95 |
7.57 |
HCT-15 |
0.308 |
7.23 |
6.87 |
>100 |
HT 29 |
0.241 |
1.61 |
3.13 |
>100 |
KM 12 |
0.231 |
1.36 |
1.87 |
6.94 |
SW-620 |
0.295 |
4.55 |
2.32 |
>100 |
|
CNS cancer |
SF-268 |
0.335 |
4.28 |
3.36 |
>100 |
SF-295 |
0.228 |
2.50 |
2.41 |
>100 |
SF-539 |
0.289 |
3.29 |
2.58 |
43.4 |
SNB-19 |
0.300 |
3.85 |
3.59 |
>100 |
SNB-75 |
0.936 |
5.17 |
6.67 |
>100 |
U 251 |
0.199 |
1.37 |
3.20 |
>100 |
|
Melanoma |
LOX IMVI |
0.189 |
0.651 |
2.10 |
>100 |
MALME-3M |
1.85 |
NA |
2.78 |
>100 |
M 14 |
0.689 |
8.50 |
3.35 |
>100 |
MDA-MB-435 |
0.373 |
4.56 |
3.01 |
>100 |
SK-MEL-2 |
2.01 |
6.55 |
3.88 |
>100 |
SK-MEL-28 |
1.67 |
5.59 |
2.29 |
21.2 |
SK-MEL-5 |
1.12 |
4.91 |
1.72 |
8.15 |
UACC-257 |
1.64 |
6.02 |
4.80 |
88.6 |
UACC-62 |
0.512 |
5.14 |
2.14 |
>100 |
|
Ovarian cancer |
IGROV1 |
0.316 |
4.36 |
2.68 |
>100 |
OVCAR-3 |
0.383 |
3.50 |
2.80 |
33.0 |
OVCAR-4 |
0.297 |
7.49 |
4.07 |
>100 |
OVCAR-5 |
1.22 |
7.12 |
4.55 |
>100 |
OVCAR-8 |
0.367 |
5.35 |
3.94 |
>100 |
NCI/ADR-RES |
0.673 |
NA |
>100 |
>100 |
SK-OV-3 |
1.26 |
5.45 |
4.66 |
>100 |
|
Renal cancer |
786-0 |
0.316 |
7.31 |
3.35 |
>100 |
A498 |
1.26 |
5.08 |
2.53 |
3.92 |
ACHN |
0.414 |
4.13 |
5.25 |
6.26 |
CAKI-1 |
0.362 |
>100 |
7.22 |
>100 |
RXF 393 |
0.190 |
0.920 |
1.81 |
7.27 |
SN 12C |
1.08 |
>100 |
2.36 |
>100 |
TX-10 |
0.603 |
4.41 |
4.96 |
>100 |
UO-31 |
0.352 |
4.01 |
14.50 |
66.20 |
|
Prostate cancer |
PC-3 |
0.315 |
8.04 |
3.57 |
>100 |
DU-145 |
0.580 |
4.30 |
3.48 |
35.40 |
|
Breast cancer |
MCF7 |
0.318 |
3.80 |
3.30 |
43.00 |
MDA-MB-231/ATCC |
0.303 |
>100 |
2.55 |
>100 |
HS 578T |
0.436 |
>100 |
6.49 |
>100 |
BT-549 |
0.792 |
5.36 |
2.16 |
16.80 |
T-47D |
1.53 |
37.3 |
5.05 |
>100 |
MDA-MB-468 |
2.17 |
4.51 |
1.51 |
>100 |
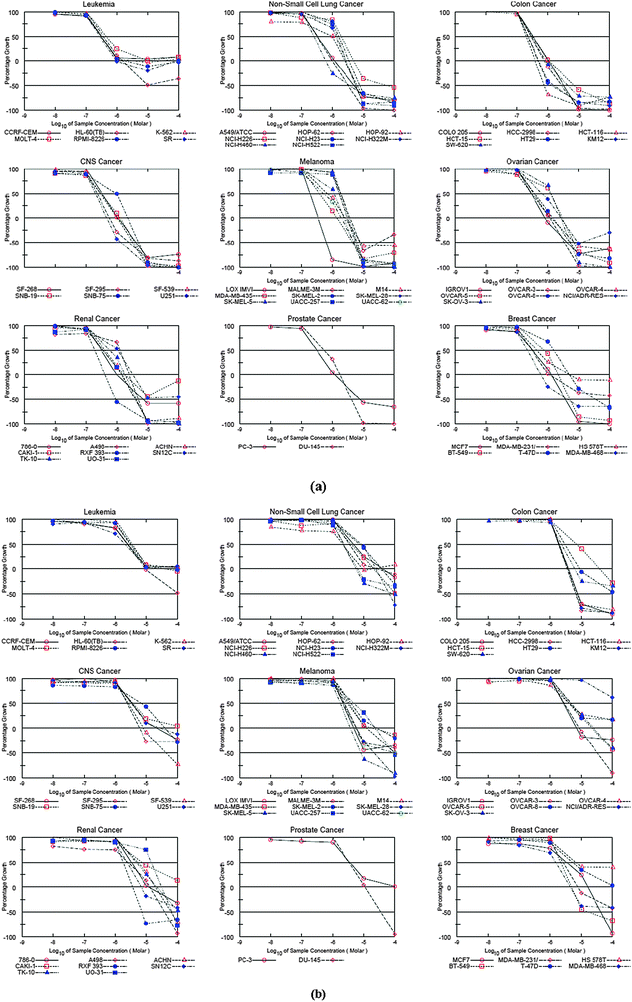 |
| Fig. 3 (a) Dose–response curves for the individual cancer panel (compound 7d); (b) dose–response curves for the individual cancer panel (compound 7f). | |
Mean graph patterns reflect the mechanism of action in vitro. The graph of a dose–response curve helps in determining effective concentrations of an agent. Fig. 3 show the dose–response curves for each of the nine cancer panels. These graphs display the effective variations in sensitivity of cell lines in different cancer panels. As can be seen from these graphs, both compounds have a similar effect on the cell lines suggesting that a class of molecular hybrids apparently attacks the same protein target.
2.2.2 Mechanism of action study.
In the initial screening, compounds 7d and 7f showed a significant effect on the colon cancer panel. Therefore, to understand the mode of their action, subsequent studies were carried out using COLO205 cell lines. These cells provide a system for careful analysis of the immunobiology and antigenicity of human adenocarcinoma of the colon without the complications of histocompatibility differences. The details of our experiments are given below.
2.2.2.1 Annexin V.
Annexin V screening was performed to assess apoptotic cells. The values obtained from the percentage of apoptotic cells were 97% for compound 7f and 63.5% for compound 7d. Results obtained revealed statistically significant (P < 0.05) activity when compared to the negative control suggesting apoptosis activity (Fig. 4). Compound 7d presented 30% of cells at the late apoptotic stage (Fig. 5) whereas compound 7f presented a majority of cells (79%) at an early apoptotic stage. These results indicated that both compounds are apoptosis inducers although with varying degrees since there are differences between both experimental compounds indicated by the early/late apoptotic stages.
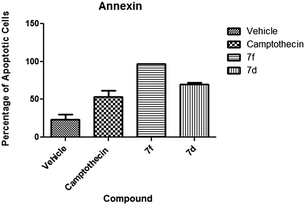 |
| Fig. 4 Annexin V. | |
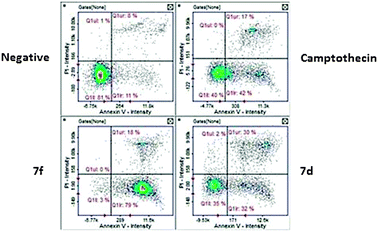 |
| Fig. 5 Distribution of apoptotic cells. | |
2.2.2.2 DNA fragmentation.
Cells with fragmented DNA are characteristic of an apoptotic death mechanism. This event is measured as a confirmatory event in the apoptotic pathway. Our experimental data present a statistically significant (P < 0.05) activity of both experimental compounds. Compound 7f presented 47% of cells with fragmented DNA while it was 54% in the case of compound 7d. These results are significant when compared to the negative control which presented 8.5% of cells with fragmented DNA (Fig. 6).
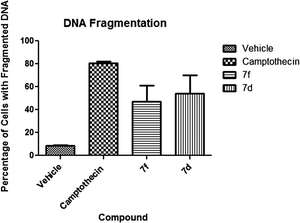 |
| Fig. 6 DNA fragmentation on COLO 205 cells. | |
2.2.2.3 Caspase activation.
(a) Caspase 3 and 7.
The activation of effector caspases is one of the key late events in apoptosis. Our test compounds demonstrated high activation of these caspases. Compound 7f gave the highest activation at 98% of cells while compound 7d presented 59.5% of cells with activated caspases (Fig. 7). Although both compounds caused significant activation, results of compound 7f were comparable (P < 0.05) to the camptothecin positive control. A key difference between these two compounds is the apoptotic stage after the 24 hour exposure. Compound 7f exposed cells were present at the early apoptotic stage while the majority of compound 7d exposed cells are at the late apoptotic stage (Fig. 8).
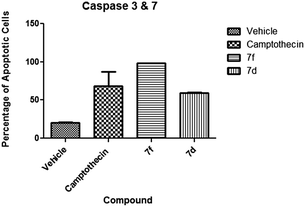 |
| Fig. 7 Caspase 3 and 7 activation. | |
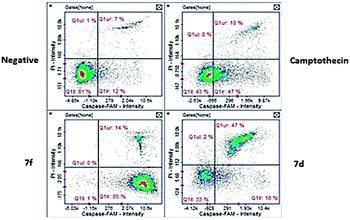 |
| Fig. 8 Distribution of effector caspases. | |
(b) Caspase 8.
Caspase 8 activation is one of the key effects in extrinsic mechanism apoptosis.49 Our experiments presented varied activity among the test compounds. Compound 7f presented the highest activation at 86.5% of cells with activated caspase 8 (Fig. 9). The majority of these cells were at the early apoptotic stage (Fig. 10). Compound 7d presented moderate induction of caspase 8 at 41% with the majority of apoptotic cells at the late apoptotic stage. Both compounds caused higher induction than the camptothecin positive control.
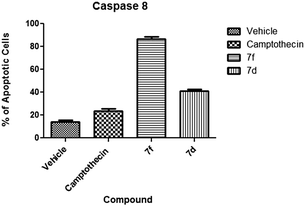 |
| Fig. 9 Caspase 8 activation. | |
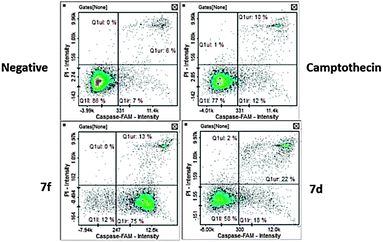 |
| Fig. 10 Caspase 8 apoptotic cell distribution. | |
(c) Caspase 9.
Caspase 9 activation is characteristic of the intrinsic apoptotic pathway.50 Our compounds caused distinct activation of caspase 9. Compound 7f caused the highest activation of caspase 9 with an average at 91.5% of cells (Fig. 11) with apoptotic cells at the early apoptotic stage (Fig. 12). Compound 7d did not activate caspase 9 in a significant manner suggesting an extrinsic mechanism.
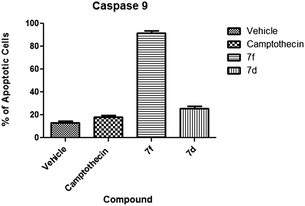 |
| Fig. 11 Caspase 9 activation. | |
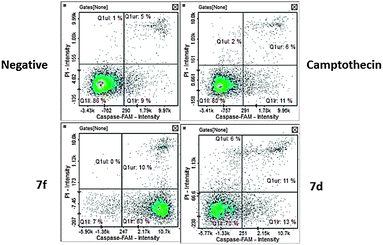 |
| Fig. 12 Apoptotic cell distribution. | |
3. Conclusion
We have synthesized a new class of C5-curcuminoid-4-aminoquinoline molecular hybrid and detailed screening of representative compounds has demonstrated their potential as anticancer agents. Mechanistic studies of test compounds 7d and 7f presented positive annexin V staining, which is characteristic of apoptotic cell death after chemical insult.51 After initial screening, we decided to explore further markers for apoptosis to determine any additional mechanistic insights, and tested for DNA fragmentation which is another hallmark of apoptosis. This nuclease mediated event occurs after activation of DNase by effector caspases.52 Our results confirmed that indeed both compounds (7d and 7f) cause apoptotic cell death since the cells exposed to them presented fragmentation of DNA and activation of effector caspases in a significant manner. Interestingly, both compounds caused activation of caspase 8 while compound 7f also caused activation of caspase 9. This simultaneous activation of both extrinsic and intrinsic apoptosis has been observed in heterocyclic compounds such as quinoline derivatives,53 where an increase in the pro apoptotic protein Bax causes the release of cytochrome C activation of caspase 9 and 8 followed by the effector caspase 3. These results have established the anticancer activity of our newly synthesized molecular hybrids, which merits their development as potential drug agents.
4. Experimental section
4.1 Chemistry
4.1.1. Instrumentation and chemicals.
All the starting materials used in the synthesis were purchased from Sigma-Aldrich (Bangalore, Karnataka, India) and were used as such. Thin layer chromatography (E. Merck Kieselgel 60 F254) was used to monitor the progress of the reactions. The synthesized compounds were purified using the silica gel column. Distilled solvents were used for purification purposes. Melting points were measured in open capillary tubes on an ERS automated melting point apparatus. IR spectra were recorded using a Perkin-Elmer FT-IR spectrophotometer and the values are expressed as λmax cm−1. The 1H and 13C NMR spectra were recorded on a Jeol Spectrospin spectrometer at 400 MHz and 100 MHz, respectively, using TMS as an internal standard. The chemical shift values are recorded on the δ scale, and the coupling constants (J) are in Hz. Mass spectral data were recorded on a Jeol-AccuTOF JMST100LC and micromass LCT Mass Spectrometer–Data system. Elemental analyses were performed on a Carlo Erba Model EA-1108 elemental analyzer and data of C, H and N are within ±0.4% of calculated values.
Procedure for synthesis of 1-((1-(2-((7-chloroquinolin-4-yl)amino)ethyl)-1H-1,2,3-triazol-4-yl)methyl)piperidin-4-one (6a) and a similar compound (6b).
To a stirred solution of compound 5 (2 g, 14.59 mmol) and compound 4a (3.60 g, 14.59 mmol) in t-butanol (40 mL) at room temperature, a solution of CuSO4·5H2O (0.7279 g, 2.918 mmol) and sodium ascorbate (1.1566 g, 5.836 mmol) in water (40 mL) was added. The temperature was increased to 40–60 °C and the reaction mixture was stirred for 3–4 h. After completion of the reaction as evident from TLC, CHCl3 (100 mL) was added and the organic layer was washed with water (3 × 500 mL) and finally with brine (200 mL). The organic layer was then dried over Na2SO4 and excess of solvent was evaporated to dryness to get the pure product. Yield: 72%; mp: 161–162 °C; IR (cm−1, Film): 3233, 3065, 2950, 1715, 1609, 1579, 1544, 1354; 1H NMR (400 MHz; CDCl3; Me4Si): 2.40 (t, 4H, J = 5.95 Hz), 2.76 (t, 4H, J = 5.95 Hz), 3.75 (s, 2H), 3.87–3.91 (m, 2H), 4.72 (t, 2H, J = 5.50 Hz), 5.96 (br s, 1H), 6.39 (d, 1H, J = 5.50 Hz), 7.35 (dd, 1H, J12 = 2.29 Hz, J13 = 8.70 Hz), 7.52 (s, 1H), 7.70 (d, 1H, J = 8.70 Hz), 7.94 (d, 1H, J = 1.83 Hz), 8.52 (d, 1H, J = 5.50 Hz); 13C NMR (100 MHz; CDCl3; Me4Si): 41.0, 42.7, 48.6, 52.2, 52.7, 98.7, 117.1, 121.1, 123.5, 125.8, 128.6, 135.3, 144.8, 148.9, 149.0, 151.7, 208.3; ESI-MS (m/z): 385.15 (M + H)+, 386.15 (M + 2)+; Anal. calcd for C19H21ClN6O: C, 59.29; H, 5.50; N, 21.84; found: C, 59.30; H, 5.51; N, 21.83%.
1-((1-(3-((7-Chloroquinolin-4-yl)amino)propyl)-1H-1,2,3-triazol-4-yl)methyl)piperidin-4-one (6b).
Yield: 71%; mp: 120–121 °C; IR (cm−1, Film): 3338, 3054, 2923, 1717, 1610, 1581, 1050, 730; 1H NMR (400 MHz; CDCl3; Me4Si): 2.36 (p, 2H, J = 6.41 Hz), 2.42 (t, 4H, J = 5.95 Hz), 2.77 (t, 4H, J = 5.95 Hz), 3.41–3.47 (m, 2H), 3.75 (s, 2H), 4.54 (t, 2H, J = 5.95 Hz), 5.84 (br s, 1H), 6.36 (d, 1H, J = 5.50 Hz), 7.35 (dd, 1H, J12 = 2.29 Hz, J13 = 8.70 Hz), 7.55 (s, 1H), 7.75 (d, 1H, J = 8.70 Hz), 7.92 (d, 1H, J = 1.83 Hz), 8.47 (d, 1H, J = 5.50 Hz); 13C NMR (100 MHz; CDCl3; Me4Si): 28.3, 40.1, 40.9, 47.9, 52.2, 52.7, 98.7, 117.1, 121.4, 122.9, 125.5, 128.2, 135.1, 144.9, 148.6, 149.6, 151.4, 208.4; ESI-MS (m/z): 399.16 (M + H)+, 400.17 (M + 2)+; Anal. calcd for C20H23ClN6O: C, 60.22; H, 5.81; N, 21.07; found: C, 60.23; H, 5.80; N, 21.09%.
Procedure for the synthesis of (3E,5E)-1-((1-(2-((7-chloroquinolin-4-yl)amino)ethyl)-1H-1,2,3-triazol-4-yl)methyl)-3,5-bis(4-methylbenzylidene)piperidin-4-one (7a) and similar compounds (7b–g and 8a–g).
To a well stirred solution of compound 6a (0.2 g, 0.51 mmol) and 4-methylbenzaldehyde (0.131 g, 1.09 mmol) in ethanol (10 mL), aqueous NaOH solution (2 mL, 20% w/v) was added drop-wise while stirring at rt for 4–5 h. After completion of the reaction as evident from TLC, a saturated solution of NH4Cl was added to quench the reaction followed by extraction of the reaction mixture with ethylacetate (2 × 20 mL). The organic layer was dried with anhydrous Na2SO4 and the crude product obtained was further purified by column chromatography. Yield: 70%; mp: 230–232 °C; IR (cm−1, Film): 3351, 3067, 2922, 1609, 1583, 1440, 1266, 1179, 1058, 877, 512; 1H NMR (400 MHz; CDCl3; Me4Si): 2.34 (s, 6H), 3.71–3.75 (m, 2H), 3.86 (s, 6H), 4.59 (t, 2H, J = 5.86 Hz), 5.84 (br s, 1H), 6.34 (d, 1H, J = 5.13 Hz), 7.18 (d, 4H, J = 8.05 Hz), 7.25 (d, 4H, J = 8.05 Hz), 7.31 (dd, 1H, J12 = 1.46 Hz, J13 = 8.79 Hz), 7.37 (s, 1H), 7.62 (d, 1H, J = 8.79 Hz), 7.75 (s, 2H), 7.92 (d, 1H, J = 2.2 Hz), 8.48 (d, 1H, J = 5.13 Hz); 13C NMR (100 MHz; CDCl3; Me4Si): 21.3, 42.7, 48.5, 52.3, 54.6, 98.7, 117.0, 121.2, 123.6, 125.9, 128.2, 129.3, 130.5, 132.1, 132.2, 135.4, 136.5, 139.5, 144.9, 148.5, 149.1, 151.3, 187.2; ESI-MS (m/z): 589.25 (M + H)+, 590.24 (M + 2)+; Anal. calcd for C35H33ClN6O: C, 71.36; H, 5.65; N, 14.27; found: C, 71.38; H, 5.66; N, 14.26%.
(3E,5E)-1-((1-(2-((7-Chloroquinolin-4-yl)amino)ethyl)-1H-1,2,3-triazol-4-yl)methyl)-3,5-bis(4-ethylbenzylidene)piperidin-4-one (7b).
Yield: 66%; mp: 181–183 °C; IR (cm−1, Film): 3357, 3056, 2963, 1604, 1578, 1447, 1196, 1140, 1074, 1056, 553; 1H NMR (400 MHz; CDCl3; Me4Si): 1.22 (t, 6H, J = 8.05 Hz), 2.64 (q, 4H, J = 8.05 Hz), 3.72–3.78 (m, 2H), 3.87 (s, 6H), 4.61 (t, 2H, J = 5.49 Hz), 6.02 (br s, 1H), 6.32 (d, 1H, J = 5.86 Hz), 7.21 (d, 4H, J = 8.05 Hz), 7.27 (d, 4H, J = 8.05 Hz), 7.30 (s, 1H), 7.41 (s, 1H), 7.66 (d, 1H, J = 8.79 Hz), 7.75 (s, 2H), 7.90 (d, 1H, J = 1.46 Hz), 8.49 (d, 1H, J = 5.13 Hz), 13C NMR (100 MHz; CDCl3; Me4Si): 15.2, 28.7, 42.7, 48.4, 52.3, 54.6, 98.6, 116.9, 121.5, 123.7, 126.0, 128.2, 127.7, 130.6, 132.1, 132.4, 135.6, 136.5, 144.8, 145.8, 147.9, 149.5, 150.7, 187.2; ESI-MS (m/z): 617.29 (M + H)+, 618.28 (M + 2)+; Anal. calcd for C37H37ClN6O: C, 72.00; H, 6.04; N, 13.62; found: C, 72.02; H, 6.05; N, 13.62%.
(3E,5E)-1-((1-(2-((7-Chloroquinolin-4-yl)amino)ethyl)-1H-1,2,3-triazol-4-yl)methyl)-3,5-bis(4-fluorobenzylidene)piperidin-4-one (7c).
Yield: 54%; mp: 217–219 °C; IR (cm−1, Film): 3246, 3065, 2928, 1610, 1581, 1266, 1187, 1104, 1079; 1H NMR (400 MHz; CDCl3; Me4Si): 3.75–3.78 (m, 2H), 3.83 (s, 4H), 3.86 (s, 2H), 4.64 (t, 2H, J = 5.49 Hz), 5.93 (br s, 1H), 6.34 (d, 1H, J = 5.86 Hz), 7.06 (d, 4H, J = 8.05 Hz), 7.29–7.34 (m, 5H), 7.43 (s, 1H), 7.65 (d, 1H, J = 8.79 Hz), 7.72 (s, 2H), 7.90 (d, 1H, J = 2.20 Hz), 8.46 (d, 1H, J = 5.13 Hz), 13C NMR (100 MHz; CDCl3; Me4Si): 42.3, 48.0, 51.2, 53.2, 98.5, 115.6, 115.8, 116.9, 121.2, 123.8, 126.0, 128.12, 131.1, 132.3, 132.4, 135.6, 135.4, 144.6, 148.3, 151.1, 161.6, 186.3; ESI-MS (m/z): 597.20 (M + H)+, 598.20 (M + 2)+; Anal. calcd for C33H27ClF2N6O: C, 66.38; H, 4.56; N, 14.08; found: C, 66.40; H, 4.54; N, 14.09%.
(3E,5E)-3,5-Bis(4-chlorobenzylidene)-1-((1-(2-((7-chloro-quinolin-4-yl)amino)ethyl)-1H-1,2,3-triazol-4-yl)methyl)piperidin-4-one (7d).
Yield: 74%; mp: 229–231 °C; IR (cm−1, Film): 3368, 3065, 2924, 1611, 1585, 1404, 1095, 525; 1H NMR (400 MHz; DMSO-d6; Me4Si): 3.63 (s, 4H), 3.72 (q, 2H, J = 5.95 Hz), 3.78 (s, 2H), 4.58 (t, 2H, J = 5.95 Hz), 6.41 (d, 1H, J = 5.50 Hz), 7.29 (dd, 1H, J12 = 2.29, J13 = 8.70 Hz), 7.39–7.41 (m, 5H), 7.47(d, 4H, J = 8.70 Hz), 7.52 (s, 2H), 7.65 (d, 1H, J = 2.29 Hz), 8.00 (s, 1H), 8.07 (d, 1H, J = 9.16 Hz), 8.29 (d, 1H, J = 5.04 Hz); 13C NMR (100 MHz; DMSO-d6; Me4Si): 42.3, 48.0, 51.1, 53.1, 98.6, 117.2, 123.6, 124.3, 124.7, 127.4, 128.7, 132.1, 133.3, 133.5, 133.8, 134.1, 141.9, 148.8, 149.7, 151.7, 186.3; ESI-MS (m/z): 629.14 (M + H)+, 630.13 (M + 2)+; Anal. calcd for C33H27Cl3N6O: C, 62.92; H, 4.32; N, 13.34; found: C, 62.93; H, 4.34; N, 13.35%.
(3E,5E)-3,5-Bis(4-bromobenzylidene)-1-((1-(2-((7-chloro-quinolin-4-yl)amino)ethyl)-1H-1,2,3-triazol-4-yl)methyl)piperidin-4-one (7e).
Yield: 71%; mp: 225–227 °C; IR (cm−1, Film): 3423, 3056, 2926, 1604, 1581, 1458, 1138, 1073, 522; 1H NMR (400 MHz; CDCl3; Me4Si): 3.76–3.80 (m, 2H), 3.82 (s, 4H), 3.85 (s, 2H), 4.63 (t, 2H, J = 6.59 Hz), 5.90 (br s, 1H), 6.35 (d, 1H, J = 5.86 Hz), 7.19 (d, 4H, J = 8.05 Hz), 7.32 (dd, 1H, J12 = 2.2 Hz, J13 = 8.79 Hz), 7.39 (s, 1H), 7.51 (d, 4H, J = 8.05 Hz), 7.64 (d, 1H, J = 8.79 Hz), 7.68 (s, 2H), 7.91 (d, 1H, J = 2.20 Hz), 8.48 (d, 1H, J = 5.27 Hz); ESI-MS (m/z): 717.03 (M + H)+, 718.03 (M + 2)+, 720.04 (M + 4)+; Anal. calcd for C33H27Br2ClN6O: C, 55.14; H, 3.79; N, 11.69; found: C, 55.15; H, 3.77; N, 11.70%.
(3E,5E)-1-((1-(2-((7-Chloroquinolin-4-yl)amino)ethyl)-1H-1,2,3-triazol-4-yl)methyl)-3,5-bis(3,4-dimethoxybenzylidene)piperidin-4-one (7f).
Yield: 76%; mp: 153–154 °C; IR (cm−1, Film): 3370, 3054, 2929, 1583, 1510, 1458, 1021,541; 1H NMR (400 MHz; CDCl3; Me4Si): 3.71–3.76 (m, 2H), 3.86–3.89 (m, 18 H), 4.58 (t, 2H, J = 5.50 Hz), 5.92 (br s, 1H), 6.32 (d, 1H, J = 5.50 Hz), 6.84–6.95 (m, 6H), 7.25–7.28 (m, 1H), 7.41 (s, 1H), 7.62 (d, 1H, J = 8.70 Hz), 7.71 (s, 2H), 7.88 (d, 1H, J = 1.83 Hz), 8.46 (d, 1H, J = 5.5 Hz); 13C NMR (100 MHz; CDCl3; Me4Si): 42.7, 48.5, 52.3, 54.6, 55.8, 55.9, 98.6, 110.9, 113.6, 117.0, 121.2, 123.8, 124.0, 125.8, 127.9, 128.2, 131.1, 135.3, 136.4, 144.5, 148.5, 148.7, 149.1, 150.0, 151.3, 186.8; ESI-MS (m/z): 681.25 (M + H)+, 682.26 (M + 2)+; Anal. calcd for C37H37ClN6O5: C, 65.24; H, 5.47; N, 12.34; found: C, 65.25; H, 5.45; N, 12.32%.
(3E,5E)-3,5-Bis(3-bromobenzylidene)-1-((1-(2-((7-chloro-quinolin-4-yl)amino)ethyl)-1H-1,2,3-triazol-4-yl)methyl)piperidin-4-one (7g).
Yield: 68%; mp: 218–220 °C; IR (cm−1, Film): 3233, 3058, 2965, 1608, 1587, 1448, 1320, 1178, 855, 568; 1H NMR (400 MHz; CDCl3; Me4Si): 3.74–3.79 (m, 2H), 3.81 (s, 4H), 3.86 (s, 2H), 4.6 (t, 2H, J = 5.55 Hz), 5.87 (br s, 1H), 6.34 (d, 1H, J = 5.13 Hz), 7.20–7.23 (m, 4H), 7.31 (dd, 1H, J12 = 2.2 Hz, J13 = 8.79 Hz), 7.41–7.44 (m, 5H), 7.63 (d, 1H, J = 8.79 Hz), 7.67 (s, 2H), 7.92 (d, 1H, J = 2.20 Hz), 8.49 (d, 1H, J = 5.13 Hz); 13C NMR (100 MHz; CDCl3; Me4Si): 42.7, 48.6, 52.0, 54.1, 98.7, 117.0, 121.1, 122.6, 123.6, 126.0, 128.6, 130.1, 131.9, 132.9, 133.7, 135.2, 135.4, 136.9, 139.1, 142.4, 144.4, 151.4, 186.5; ESI-MS (m/z): 717.03 (M + H)+, 718.04 (M + 2)+, 720.05 (M + 4)+; Anal. calcd for C33H27Br2ClN6O: C, 55.14; H, 3.79; N, 11.69; found: C, 55.16; H, 3.78; N, 11.68%.
(3E,5E)-1-((1-(3-((7-Chloroquinolin-4-yl)amino)propyl)-1H-1,2,3-triazol-4-yl)methyl)-3,5-bis(4-methylbenzylidene)piperidin-4-one (8a).
Yield: 73%; mp: 159–161 °C; IR (cm−1, Film): 3346, 3068, 2953, 1610, 1583, 1440, 1079, 537; 1H NMR (400 MHz; CDCl3; Me4Si): 2.17 (p, 2H, J = 5.86 Hz), 2.32 (s, 6H), 3.14 (q, 2H, J = 5.86 Hz), 3.86 (s, 2H), 3.88 (s, 4H), 4.37 (t, 2H, J = 6.59 Hz), 5.56 (br s, 1H), 6.29 (d, 1H, J = 4.39 Hz), 7.18 (d, 4H, J = 8.05 Hz), 7.26 (d, 4H, J = 7.32 Hz), 7.36 (m, 2H), 7.70 (d, 1H, J = 8.79 Hz), 7.77 (s, 2H), 7.94 (d, 1H, J = 2.20 Hz), 8.48 (d, 1H, J = 5.37 Hz); 13C NMR (100 MHz; DMSO-d6; Me4Si): 21.3, 28.1, 30.9, 39.4, 47.5, 52.3, 54.6, 98.6, 117.1, 121.2, 122.9, 125.5, 128.6, 129.3, 130.5, 132.1, 135.0, 136.6, 139.5, 145.1, 149.1, 149.2, 151.8, 187.0; ESI-MS (m/z): 603.25 (M + H)+, 604.24 (M + 2)+; Anal. calcd for C36H35ClN6O: C, 71.69; H, 5.85; N, 13.93; found: C, 71.70; H, 5.86; N, 13.92%.
(3E,5E)-1-((1-(3-((7-Chloroquinolin-4-yl)amino)propyl)-1H-1,2,3-triazol-4-yl)methyl)-3,5-bis(4-ethylbenzylidene)piperidin-4-one (8b).
Yield: 71%; mp: 148–150 °C; IR (cm−1, Film): 3336, 3041, 2963, 1602, 1580, 1431, 1176, 1137, 1002, 805, 505; 1H NMR (400 MHz; DMSO-d6; Me4Si): 1.10 (t, 6H, J = 7.33 Hz), 2.08 (p, 2H, J = 6.87 Hz), 2.54 (q, 4H, J = 7.33 Hz), 3.11 (q, 2H, J = 5.95 Hz), 3.78 (s, 6H), 4.38 (t, 2H, J = 6.87 Hz), 6.28 (d, 1H, J = 5.50 Hz), 7.22 (d, 4H, J = 8.24 Hz), 7.28 (br s, 1H), 7.32 (d, 4H, J = 7.79 Hz), 7.40 (dd, 1H, J12 = 1.83 Hz, J13 = 9.16 Hz), 7.52 (s, 2H), 7.73 (d, 1H, J = 2.75 Hz), 7.99 (s, 1H), 8.18 (d, 1H, J = 8.70 Hz), 8.28 (d, 1H, J = 5.48 Hz); 13C NMR (100 MHz; DMSO-d6; Me4Si): 15.2, 28.0, 28.4, 40.0, 47.2, 51.5, 53.8,98.6, 117.4, 124.1, 124.2, 124.3, 127.3, 128.2, 130.6, 132.0, 132.9, 133.5, 134.8, 143.0, 145.4, 148.8, 150.0, 151.7, 186.6; ESI-MS (m/z): 631.28 (M + H)+, 632.30 (M + 2)+; Anal. calcd for C38H39ClN6O: C, 72.31; H, 6.23; N, 13.31; found: C, 72.30; H, 6.25; N, 13.33%.
(3E,5E)-1-((1-(3-((7-Chloroquinolin-4-yl)amino)propyl)-1H-1,2,3-triazol-4-yl)methyl)-3,5-bis(4-fluorobenzylidene)piperidin-4-one (8c).
Yield: 58%; mp: 166–168 °C; IR (cm−1, Film): 3248, 3065, 2929, 1611, 1582, 1276, 1188, 1104, 1077, 850; 1H NMR (400 MHz; DMSO-d6; Me4Si): 2.08 (p, 2H, J = 6.4 Hz), 3.10 (m, 2H), 3.73 (s, 2H), 3.77 (s, 4H), 4.40 (t, 2H, J = 6.41 Hz), 6.27 (d, 1H, J = 5.50 Hz), 6.95–6.97 (m, 4H), 7.20–7.25 (m, 2H), 7.37–7.41 (m, 4H), 7.51 (s, 2H), 7.73 (d, 1H, J = 2.29 Hz), 8.01 (s, 1H), 8.18 (d, 1H, J = 9.6 Hz), 8.29 (d, 1H, J = 5.50 Hz); ESI-MS (m/z): 611.20 (M + H)+, 612.22 (M + 2)+; Anal. calcd for C34H29ClF2N6O: C, 66.83; H, 4.78; N, 13.75; found: C, 66.84; H, 4.79; N, 13.75%.
(3E,5E)-3,5-Bis(4-chlorobenzylidene)-1-((1-(3-((7-chloro-quinolin-4-yl)amino)propyl)-1H-1,2,3-triazol-4-yl)methyl)piperidin-4-one (8d).
Yield: 74%; mp: 212–213 °C; IR (cm−1, Film): 3245, 3065, 2924, 1611, 1579, 1458, 1094, 525; 1H NMR (400 MHz; CDCl3; Me4Si): 2.18 (p, 2H, J = 6.22 Hz), 3.23 (q, 2H, J = 5.49 Hz), 3.84 (s, 4H), 3.85 (s, 2H), 4.41 (t, 2H, J = 6.22 Hz), 5.44 (br s, 1H), 6.31 (d, 1H, J = 5.86 Hz), 7.28 (d, 4H, J = 8.05 Hz), 7.35–7.39 (m, 6H), 7.67 (d, 1H, J = 8.79 Hz), 7.73 (s, 2H), 7.94 (d, 1H, J = 1.10 Hz), 8.49 (d, 1H, J = 5.13 Hz); 13C NMR (100 MHz; CDCl3; Me4Si): 28.3, 39.7, 47.7, 52.2, 54.3, 98.8, 117.1, 121.0, 122.8, 125.6, 128.8, 128.9, 131.5, 133.1, 133.3, 135.1, 135.2, 135.4, 144.8, 149.1, 149.2, 151.8, 186.7; ESI-MS (m/z): 643.15 (M + H)+, 644.15 (M + 2)+; Anal. calcd for C34H29Cl3N6O: C, 63.41; H, 4.54; N, 13.05; found: C, 63.40; H, 4.55; N, 13.07%.
(3E,5E)-3,5-Bis(4-bromobenzylidene)-1-((1-(3-((7-chloro-quinolin-4-yl)amino)propyl)-1H-1,2,3-triazol-4-yl)methyl)piperidin-4-one (8e).
Yield: 72%; mp: 213–215 °C; IR (cm−1, Film): 3400, 3065, 2929, 1610, 1579, 1485, 1072, 523; 1H NMR (400 MHz; CDCl3; Me4Si): 2.17 (p, 2H, J = 6.35 Hz), 3.23 (q, 2H, J = 5.86 Hz), 3.83 (s, 4H), 3.85 (s, 2H), 4.41 (t, 2H, J = 6.59 Hz), 5.42 (br s, 1H), 6.32 (d, 1H, J = 4.39 Hz), 7.21 (d, 4H, J = 8.05 Hz), 7.34 (s, 1H), 7.39 (dd, 1H, J12 = 2.93 Hz, J13 = 9.52 Hz), 7.52 (d, 4H, J = 8.05 Hz), 7.68 (d, 1H, J = 9.52 Hz), 7.71 (s, 2H), 7.95 (d, 1H, J = 2.20 Hz), 8.50 (d, 1H, J = 5.13 Hz); 13C NMR (100 MHz; DMSO-d6; Me4Si): 28.3, 40.0, 47.2, 51.3, 53.5, 98.6, 117.4, 122.8, 124.1, 127.4, 131.6, 131.7, 132.3, 13.4, 133.7, 133.8, 134.2, 142.9, 148.8, 149.9, 151.7, 186.4; ESI-MS (m/z): 731.06 (M + H)+, 732.05 (M + 2)+, 734.05 (M + 4)+; Anal. calcd for C34H29Br2ClN6O: C, 55.72; H, 3.99; N, 11.47; found: C, 55.70; H, 3.97; N, 11.45%.
(3E,5E)-1-((1-(3-((7-Chloroquinolin-4-yl)amino)propyl)-1H-1,2,3-triazol-4-yl)methyl)-3,5-bis(3,4-dimethoxybenzylidene)piperidin-4-one (8f).
Yield: 76%; mp: 185–186 °C; IR (cm−1, Film): 3312, 3065, 2958, 1611, 1597, 1458, 1084, 804, 618, 548; 1H NMR (400 MHz; CDCl3; Me4Si): 2.14(p, 2H, J = 5.86 Hz), 3.17 (q, 2H, J = 5.86 Hz), 3.85 (s, 4H), 3.87 (s, 2H), 3.90 (s, 12H), 4.39 (t, 2H, J = 6.59 Hz), 5.51 (br s, 1H), 6.29 (d, 1H, J = 5.13 Hz), 6.86 (s, 1H), 6.90 (m, 3H), 6.96–6.99 (m, 2H), 7.36–7.39 (m, 2H), 7.72–7.75 (m, 3H), 7.94 (d, 1H, J = 2.20 Hz), 8.49 (d, 1H, J = 5.13 Hz) 13C NMR (100 MHz; CDCl3; Me4Si): 28.2, 39.5, 47.5, 52.4, 54.6, 55.3, 98.7, 114.1, 117.1, 121.1, 122.9, 125.5, 127.7, 128.7, 131.0, 132.3, 135.0, 136.2, 145.3, 149.1, 149.2, 151.9, 160.3, 187.0; ESI-MS (m/z): 695.25 (M + H)+, 696.24 (M + 2)+; Anal. calcd for C38H39ClN6O5: C, 65.65; H, 5.65; N, 12.09; found: C, 65.66; H, 5.66; N, 12.10%.
(3E,5E)-1-((1-(2-((7-Chloroquinolin-4-yl)amino)ethyl)-1H-1,2,3-triazol-4-yl)methyl)-3,5-bis(4-methoxybenzylidene)piperidin-4-one (8g).
Yield: 70%; mp: 190–192 °C; IR (cm−1, Film): 3356, 3052, 2928, 1600, 1583, 1327, 1081, 1060, 831, 530; 1H NMR (400 MHz; CDCl3; Me4Si): 2.14 (p, 2H, J = 6.22 Hz), 3.15 (q, 2H, J = 6.59 Hz), 3.78 (s, 6H), 3.82–3.88 (m, 6H), 4.39 (t, 2H, J = 6.59 Hz), 5.46 (br s, 1H), 6.30 (d, 1H, J = 5.86 Hz), 6.90 (d, 4H, J = 8.79 Hz), 7.33 (d, 4H, J = 8.79 Hz), 7.36–7.37 (m, 2H), 7.70 (d, 1H, J = 9.52 Hz), 7.75 (s, 2H), 7.94 (d, 1H, J = 2.20 Hz), 8.49 (d, 1H, J = 5.13 Hz) 13C NMR (100 MHz; CDCl3; Me4Si): 28.2, 39.5, 47.5, 52.4, 54.6, 55.2, 98.7, 114.0, 117.1, 121.1, 122.9, 125.5, 127.7, 128.7, 131.0, 132.3, 135.0, 136.2, 145.2, 149.1, 151.8, 160.2, 187.0; ESI-MS (m/z): 635.24 (M + H)+, 636.25 (M + 2)+; Anal. calcd for C36H35ClN6O3: C, 68.08; H, 5.55; N, 13.23; found: C, 68.10; H, 5.56; N, 13.22%.
4.2 Anticancer screening
The anticancer screening was carried out following the procedure described earlier.48
4.3 Mechanism of action studies
Materials and methods.
Compound stock solutions.
Compounds were prepared at a 3 mM stock concentration in DMSO culture grade under sterile conditions. All experiments were done at the 10 μM dose as determined by the NCI 60 cell line screening protocol previously performed for these compounds.
Cell culture.
The cell line used in this study was COLO-205 human colorectal adenocarcinoma (ATCC CCL-222). These cells were maintained in RPMI 1640 (ATCC, Manassas VA) supplemented with 10% fetal bovine serum (ATCC). Cultures were maintained at 37 °C with a humidified atmosphere of 95% air/5% CO2.
Annexin V.
The annexin V assay has been used as an imaging tool for the detection and indication of phosphatidylserine (PS) on the surface of cells; a key event in apoptotic cells.54 Approximately 3 × 106 cells were treated with the IC50 dose of each compound and controls (vehicle DMSO and camptothecin). After exposure, cells were stained with the annexin V conjugate and propidium iodide (Biotium, Hayward, CA). Samples were then analysed using the Nucleo Counter NC3000 (Chemometec, Allerød, Denmark). A one way ANOVA was performed. If significant results were found in the ANOVA, Tukey's posthoc test was also performed.
DNA fragmentation.
DNA fragmentation as an apoptosis marker is a commonly used assay in drug-cell interaction studies.55,56 This nuclease mediated event can be quantified using the DNA content and measuring cells containing less than 1 DNA equivalent known as Sub-G1. The NC3000 system was used for this experiment. The NC3000 assay is based on removal of small DNA fragments and retention of 4′,6-diamidino-2-phenylindole (DAPI) stained higher weight fragments. After treatment with the tested compounds at the dose and conditions described above, cells were fixed with ethanol 70%, stained with 1 μg ml−1 DAPI, and analysed by differential image analysis using the NC3000 instrument measuring DAPI intensity. A one way ANOVA was performed. If significant results were found in the ANOVA, Tukey's posthoc test was also performed.
Caspase activation.
Activation of effector caspases is a key event in the progression of apoptotic cell death.57 This activation was measured using the Fluorescent Labeled Inhibitors of Caspase (FLICA) probes that bind covalently with active caspase effector enzymes. After treatment as described above, cells were harvested and stained using the green FAM FLICA kit (Immunochemistry Technologies, Bloomington Min.). Samples were then analysed using the Nucleocounter NC3000 instrument. A one way ANOVA was performed. If significant results were found in the one way ANOVA, Tukey's post-hoc test was also performed.
Acknowledgements
DSR acknowledges University Grant Commission [41-202/2012 (SR)], New Delhi, India and DU-DST PURSE, University of Delhi, Delhi, India for financial support. S. K. K. and S. M. are thankful to CSIR for the award of junior and senior research fellowship. S. V. M. would like to acknowledge the support from National Cancer Institute, National Institutes of Health, under Contract No. HHSN261200800001E. Authors are also thankful to CIF-USIC, University of Delhi, Delhi for NMR spectral data and RSIC, CDRI, Lucknow for mass data. We thank Alfredo Blakeley, Julie Early, Stephanie Florio, and Juliane Ollinger for technical assistance.
References
- WHO (World Health Organization); World Cancer Report, 2008, 1–26.
- F. Bray, A. Jemal, N. Grey, J. Ferlayand and D. Forman, Lancet Oncol., 2012, 13, 790–801 CrossRef.
-
http://www.medicalnewstoday.com/info/cancer-oncology (accessed May 2013).
-
S. Dhar and S. J. Lippard, in Bioinorganic Medicinal Chemistry, ed. E. Alessio, Wiley-VCH Verlag GmbH & Co. KGaA, Weinheim, 2011, pp. 79–95 Search PubMed.
- R. Ralhan and J. Kaur, Expert Opin. Ther. Pat., 2007, 17, 1061–1075 CrossRef CAS.
- T. A. Connors, Dev. Pharmacol., 1983, 3, 47–57 CAS.
- J. M. Wilbur Jr, J. Med. Chem., 1978, 21, 1168–1171 CrossRef CAS.
- L. H. Schmidt, Cancer Res., 1963, 23, 1311–1314 CAS.
- G. H. Elgemeie, Curr. Pharm. Des., 2003, 9, 2627–2642 CrossRef CAS.
- J. Scaife and D. Kerr, Anticancer Ther., 2008, 91–110 CAS.
- S. B. Kaye, Br. J. Cancer, 1998, 78, 1–7 CrossRef CAS.
- V. D. Nikolic, I. M. Savic, I. M. Savic, L. B. Nikolic, M. Z. Stankovic and V. D. Marinkovic, Cent. Eur. J. Med., 2011, 6, 527–536 CrossRef CAS PubMed.
- A. L. Demain and P. Vaishnav, Microb. Biotechnol., 2011, 4, 687–699 CrossRef PubMed.
-
M. Ghosh, M. Thapliyal and K. Gurumurthi, Novel Therapeutic Agents from Plants, 2009, ch. 1, pp. 1–35 Search PubMed.
- H. Savel, Prog. Exp. Tumor Res., 1966, 8, 189–224 CAS.
- S. Marsh and J. M. Hoskins, Pharmacogenomics, 2010, 11, 1003–1010 CrossRef CAS PubMed.
- D. S. Thakur, Int. J. Pharm. Sci. Nanotechnol., 2011, 3, 1173–1181 CAS.
-
D. Wright, Biology of Cancer, 2nd edn, 2007, ch. 5, pp. 45–53 Search PubMed.
- A. C. S. Souza, A. de Fatima, R. B. da Silveira and G. Z. Justo, Curr. Drug Targets, 2012, 13, 1072–1082 CrossRef CAS.
- E. H. Liu, L. W. Qi, Q. Wu, Y. B. Peng and P. Li, Mini-Rev. Med. Chem., 2009, 9, 1547–1555 CrossRef CAS.
- A. Shehzad, F. Wahid and Y. S. Lee, Arch. Pharm., 2010, 343, 489–499 CrossRef CAS PubMed.
- S. Shankar, Q. Chen, K. Sarva, I. Siddiqui and R. K. Srivastava, J. Mol. Signaling, 2007, 2, 10 CrossRef PubMed.
- C. S. Beevers, F. Li, L. Liu and S. Huang, Int. J. Cancer, 2006, 119, 757–764 CrossRef CAS PubMed.
- S. M. Johnson, P. Gulhati, I. Arrieta, X. Wang, T. Uchida, T. Gao and B. M. Evers, Anticancer Res., 2009, 29, 3185–3190 CAS.
- A. R. Hussain, M. Al-Rasheed, P. S. Manogaran, K. A. Al-Hussein, L. C. Platanias, K. A. Kuraya and S. Uddin, Apoptosis, 2006, 11, 245–254 CrossRef CAS PubMed.
- C. P. Prasad, G. Rath, S. Mathur, D. Bhatnagar and R. Ralhan, Chem.-Biol. Interact., 2009, 181, 263 CrossRef CAS PubMed.
- S. Manohar, S. I. Khan, S. K. Kandi, K. Raj, G. Sun, X. Yang, A. D. C. Molina, N. Ni, B. Wang and D. S. Rawat, Bioorg. Med. Chem. Lett., 2013, 23, 112–116 CrossRef CAS PubMed.
- F. H. Sarkar and Y. Li, Cancer Treat. Rev., 2009, 35, 597–607 CrossRef CAS PubMed.
- P. Anand, A. B. Kunnumakkara, R. A. Newman and B. B. Aggarwal, Mol. Pharmaceutics, 2007, 4, 807–818 CrossRef CAS PubMed.
- E. Burgos-Moron, J. M. Calderon-Montano, J. Salvador, A. Robles and M. Lopez-Lazaro, Int. J. Cancer, 2010, 126, 1771–1775 CAS.
- M. J. Rosemond, L. St John-Williams, T. Yamaguchi, T. Fujishita and J. S. Walsh, Chem.-Biol. Interact., 2004, 147, 129–139 CrossRef CAS PubMed.
- M. V. Makarov, E. S. Leonova, E. Y. Rybalkina, P. Tongwa, V. N. Khrustalev, T. V. Timofeeva and I. L. Odinets, Eur. J. Med. Chem., 2010, 45, 992–1000 CrossRef CAS PubMed.
- B. Yadav, S. Taurin, R. J. Rosengren, M. Schumacher, M. Diederich, T. J. Somers-Edgar and L. Larsen, Bioorg. Med. Chem., 2010, 18, 6701–6707 CrossRef CAS PubMed.
- A. M. Katsori, M. Chatzopoulou, K. Dimas, C. Kontogiorgis, A. Patsilinakos, T. Trangas and D. Hadjipavlou-Litina, Eur. J. Med. Chem., 2011, 46, 2722–2735 CrossRef CAS PubMed.
- P. Lagisetty, P. Vilekar, K. Sahoo, S. Anant and V. Awasthi, Bioorg. Med. Chem., 2010, 18, 6109–6120 CrossRef CAS PubMed.
- B. K. Adams, E. M. Ferstl, M. C. Davis, M. Herold, S. Kurtkaya, R. F. Camalier, M. G. Hollingshead, G. Kaur, E. A. Sausville, F. R. Rickles, J. P. Snyder, D. C. Liotta and M. Shoji, Bioorg. Med. Chem., 2004, 12, 3871–3883 CrossRef CAS PubMed.
- B. Meunier, Acc. Chem. Res., 2008, 41, 69–77 CrossRef CAS PubMed.
- V. V. Kouznetsova and A. Gomez-Barrio, Eur. J. Med. Chem., 2009, 44, 3091–3113 CrossRef PubMed.
- M. Getlik, C. Grutter, J. R. Simard, S. Kluter, M. Rabiller, H. B. Rode, A. Robubi and D. Rauh, J. Med. Chem., 2009, 52, 3915–3926 CrossRef CAS PubMed.
- R. Kakadiya, H. Dong, A. Kumar, D. Narsinh, X. Zhang, T. C. Chou, T. C. Lee, A. Shah and T. L. Su, Bioorg. Med. Chem., 2010, 18, 2285–2299 CrossRef CAS PubMed.
- V. Srivastava, A. S. Negi, J. K. Kumar, M. M. Gupta and S. P. Khanuja, Bioorg. Med. Chem., 2005, 13, 5892–5908 CrossRef CAS PubMed.
- S. Manohar, S. I. Khan and D. S. Rawat, Chem. Biol. Drug Des., 2013, 81, 625–630 CAS.
- S. Manohar, U. C. Rajesh, S. I. Khan, B. L. Tekwani and D. S. Rawat, ACS Med. Chem. Lett., 2012, 3, 555–559 CrossRef CAS PubMed.
- S. Manohar, S. I. Khan and D. S. Rawat, Bioorg. Med. Chem. Lett., 2010, 20, 322–325 CrossRef CAS PubMed.
- S. Manohar, S. I. Khan and D. S. Rawat, Chem. Biol. Drug Des., 2011, 78, 124–136 CAS.
- S. G. Agalave, S. R. Maujan and V. S. Pore, Chem. – Asian J., 2011, 6, 2696–2718 CrossRef CAS PubMed.
- V. V. Rostovtsev, L. G. Green, V. V. Fokin and K. B. Sharpless, Angew. Chem., Int. Ed., 2002, 4, 2596–2599 CrossRef.
-
http://htttp://dtp.nci.nih.gov/branches/btb/ivclsp.html
.
- M. Kruidering and G. I. Evan, IUBMB Life, 2000, 50, 85–90 CrossRef CAS PubMed.
- S. Elmore, Toxicol. Pathol., 2007, 35, 495–516 CrossRef CAS PubMed.
- A. M. Alabsi, R. Ali, A. M. Ali, S. A. R. Al-Dubai, H. Harun, N. H. A. Kasim and A. Alsalahi, Asian Pac. J. Cancer. Prev., 2012, 13, 5131–5136 CrossRef.
- D. McIlroy, H. Sakahira, R. V. Talanian and S. Nagata, Oncogene, 1999, 18, 4401–4408 CrossRef CAS PubMed.
- Y. Ding and T. A. Nguyen, Apoptosis, 2013, 18, 1071–1082 CrossRef CAS PubMed.
- K. Schutters and C. Reutelingsperger, Apoptosis, 2010, 15, 1072–1082 CrossRef CAS PubMed.
- J. C. Lin, Y. S. Ho, J. J. Lee, C. L. Liu, T. L. Yang and C. H. Wu, Food Chem. Toxicol., 2007, 45, 935–944 CrossRef CAS PubMed.
- H. M. Lien, P. T. Kuo, C. L. Huang, J. Y. Kao, H. Lin, D. Y. Yang and Y. Y. Lai, J. Evidence-Based Complementary Altern. Med., 2011, 450529 Search PubMed.
- Y. R. Liao, C. C. Lu, K. C. Lai, J. S. Yang, S. C. Kuo, Y. F. Wen, S. Fushiya and T. S. Wu, Mol. Med. Rep., 2013, 7, 1539–1544 CAS.
Footnote |
† Electronic supplementary information (ESI) available: Single dose data of compounds 7d and 7f, one dose mean graph (compound 7d), five dose results (compound 7d), one dose mean graph (compound 7f), five dose results (compound 7f), 1H and 13C NMR of representative compounds (6a–b, 7d and 7f). See DOI: 10.1039/c4nj00936c |
|
This journal is © The Royal Society of Chemistry and the Centre National de la Recherche Scientifique 2015 |
Click here to see how this site uses Cookies. View our privacy policy here.