Does powder and granular activated carbon perform equally in immobilizing chlorobenzenes in soil?†
Received
12th September 2014
, Accepted 30th October 2014
First published on 31st October 2014
Abstract
The objective of this study is to compare the efficacies of powder activated carbon (PAC) and granular activated carbon (GAC) as amendments for the immobilization of volatile compounds in soil. Soil artificially-spiked with chlorobenzenes (CBs) was amended with either PAC or GAC to obtain an application rate of 1%. The results showed that the dissipation and volatilization of CBs from the amended soil significantly decreased compared to the unamended soil. The bioavailabilities of CBs, which is expressed as butanol extraction and earthworm accumulation, were significantly reduced in PAC and GAC amended soils. The lower chlorinated and hence more volatile CBs experienced higher reductions in both dissipation and bioavailability in the amended soils. The GAC and PAC equally immobilized more volatile CBs in soil. Therefore, it could be concluded that along with environmental implication, applying GAC was the more promising approach for the effective immobilization of volatile compounds in soil.
Environmental impact
Immobilization, which sought to reduce the bioavailability of contaminants in soil, has been viewed as an efficient and environmentally friendly remediation strategy. In particular, for volatile compounds, their volatilization from soil into air could be inhibited after immobilization. This is quite an important issue because air contamination results in the direct exposure of humans to pollutants and is much more difficult for remediation. However, the immobilization of volatile compounds in soil by amendments, such as activated carbon (AC), is rarely reported. Whether granular AC provides equivalent effect as powder AC for the immobilization of volatile compounds remains unknown. If so, applying granular AC in soil could be an efficient remediation strategy because it is more environmentally friendly than powder AC.
|
Introduction
Chlorobenzenes (CBs) are used as starting materials and additives in the production of insecticides, fungicides, herbicides, dyes, pharmaceuticals, rubbers, and plastics.1,2 China is an important producer of CBs in the world and accounts for more than 50% of the worldwide production.3 Due to the wide use and production of CBs, they have been detected in soil, water and sediments in many places in China.4–6 All the congeners of CBs, especially trichlorobenzenes (TCBs), have been detected in the soil of vegetable fields within 1 km from a factory that is manufacturing CBs.7 The lower chlorinated CBs are easily volatilized from soil, resulting in air pollution and making it difficult for their remediation.8 Therefore, it is necessary to immobilize volatile CBs to reduce their environmental risks in contaminated soil and prevent air pollution.
It is generally agreed that sorption induced immobilization, which seeks to reduce the bioavailability and mobility of contaminants in soil or sediment, and thus reduce the potential accumulation or uptake of contaminants by biota, is an effective in situ remediation strategy.9,10 Among the amendments, activated carbon (AC) has shown a great potential for immobilizing contaminants, such as PCBs, PAHs and DDTs, in sediments.11–14 Lately, AC is being increasingly used for the immobilization of hydrophobic organic contaminants in soils.15,16 Earthworm accumulation of PCBs and plant uptake of PAHs were significantly decreased in the soils amended with AC.17,18 The strong sorption capacity of AC is because of its high porosity and large surface area.19 Generally, powder AC (PAC) performs better than granular AC (GAC) in reducing the bioavailability of contaminants such as PAHs in soil.18,20 However, reports on whether AC can immobilize volatile compounds are limited.15,21 Moreover, reports on the comparison of the immobilizing potential of PAC and GAC for volatile compounds in soil are rare. Volatile compounds, such as lower chlorinated CBs, show different environmental behavior as compared to less volatile compounds, such as PCDD/Fs or PCBs, in soil.22 One hypothesis is that the volatile compounds in soil could migrate and easily diffuse to the strong sorbents of the large or small AC particles. If so, GAC may perform the equivalent immobilizing effect as PAC for volatile compounds in soil.
The objective of this study was therefore to test whether AC could immobilize the volatile compounds in soil and to compare the immobilizing efficacies of PAC and GAC. A lower chlorinated and easily volatile CB, i.e. 1,2,4-TCB, as well as a higher chlorinated and relatively less volatile CB, i.e. hexachlorobenzene (HCB), were selected as model compounds for this study. The immobilization effectiveness was evaluated by spiking soil with the CBs, followed by determining their residues and volatilization over time and by determining the bioavailability changes of CBs in soil with mild solvent (butanol) extraction and a bioassay (earthworm accumulation) study.
Materials and methods
Chemicals
The CBs standards (>99.5% purity) were purchased from Dr Ehrenstorfer (Augsburg, Germany). The physico-chemical properties of HCB and 1,2,4-TCB are shown in Table S1 (ESI†). The solvents and all other chemical reagents, purchased from Nanjing Chemical factory (Nanjing, China), were of analytical grade. Anhydrous sodium sulfate was oven-dried at 400 °C for 4 h.
Soil sampling
An agricultural soil (Ferri-Udic Argosols, as referred to in Chinese Soil Taxonomy) was collected from a vegetable field in Nanjing, China. The soil was sampled from the upper 20 cm layer, and passed through a 2 mm sieve before storage at 4 °C. The soil had a pH of 7.56; total carbon content of 3.10%; clay content of 13.61%, silt content of 63.11% and sand content of 23.28%.23
Activated carbon preparation and characterization
A commercial AC was purchased from Shanghai Chemical Company. The AC was ground and sieved into two parts, powder AC (PAC) with particle size <150 μm, and granular AC (GAC) with particle size between 425 and 850 μm. The elemental C, N and H compositions of AC were determined by a CNH analyzer (Vario MICRO, Germany elementar, Germany), and O content was estimated by mass difference (100% – (C, H, N and ash %)).24 The specific surface areas of PAC and GAC were determined by applying the Brunauer–Emmett–Teller (BET) method to nitrogen adsorption isotherms, whereas the pore volume and size were determined using Barrett–Joyner–Halenda (BJH) adsorption and desorption analysis. The functional groups of AC were determined using a Fourier transform infrared (FTIR) spectrometer (NEXUS 870, Thermo Nicolet, USA). The samples for FTIR analysis were prepared in 2% KBr wagers. The infrared spectra were obtained with 2 cm−1 resolution with 64 scans between wavenumbers of 400–4000 cm−1.
Soil amendment experiment
Note that artificially contaminated soil was used in this experiment. The mixed standards of CBs (HCB and TCB) were dissolved in acetone, and then applied to an aliquot of 15 g (dry weight) of soil in a 50 mL glass beaker. After the evaporation of acetone, 15 g of spiked soil was mixed, and then transferred into a 1000 mL glass beaker, which already contained 282 g (dry weight) of equilibrated soil (20% soil moisture content, 25 °C for 1 week), and the contents were mixed by stirring carefully with a spatula. Then, 3 g each of PAC or GAC (1% amendment rate, w/w) was added into different glass beakers to obtain a total amount of 300 g (dry weight) of amended soil. The spiked soil and amendments were thoroughly mixed with a spatula again and transferred to a 1000 mL incubation flask. The water content was adjusted to 28% soil moisture content. Then, 2 g (dry weight) of the spiked soil was sampled to analyze the initial concentrations of CBs in the soil. The remaining soil was compacted to a volume equivalent to 1.3 g cm−3 of soil density. The flask was tightly closed with a rubber plug, which contained inlet and outlet tubes, and incubated at 25 °C for 48 weeks in the dark. The soil spiked by HCB and TCB but unamended with AC was used as control. Therefore, there were a total of 3 treatments, namely, Control (unamended), PAC (1% PAC amendment) and GAC (1% GAC amendment). The concentrations of CBs in each treatment were 1.72 ± 0.05 μg g−1 and 7.33 ± 0.27 μg g−1 for HCB and TCB, respectively. Data of Control before 24 weeks was obtained from previous studies.23,25 Note that all the treatments were conducted in triplicates.
During the incubation period, the flasks were aerated once in a week for 20 min at an exchange rate of 0.4 L min−1, in a closed laboratory trapping system,2 to flush out and trap the volatilized CBs. After aeration, 10 g of soil was sampled for CBs residues and butanol extraction analysis at a specific pre-determined interval. For earthworm bioassay experiment, 25 g of soil was sampled after 1 week and 48 weeks of incubation.
Earthworm bioassay
The earthworm (Eisenia foetida) uptake of CBs in soil was conducted as a bioassay experiment.27 Briefly, 10 adult worms with clitellum were exposed to 25 g of (dry weight) soils, adjusted to 30% soil moisture content with deionized water in a 100 mL glass jar, and covered with aluminum foil with several holes. The soils were maintained under constant room light at 25 °C for 14 days. After exposure, the worms were rinsed and allowed to purge their gut contents for 48 h on moistened filter papers. The worms were weighed, frozen dried, and ground with 7 times their weight of anhydrous sodium sulphate and an equal weight of quartz sand, followed by accelerated solvent extraction and gas chromatography (GC) analysis.
Accelerated solvent extraction
The residues of CBs in soil were extracted by accelerated solvent extraction (ASE 200, Dionex, USA) and were expressed as the total concentrations of CBs in soil. Briefly, 2 g of soil samples were homogenized with 5 g of diatomaceous earth and extracted with hexane/acetone (4
:
1, v/v) at a temperature of 100 °C and a pressure of 1500 psi. The extracts were rotary evaporated at 45 °C to about 2 mL, and then applied to a silica gel/anhydrous sodium sulfate column, followed by elution with 15 mL hexane/dichloromethane (9
:
1, v/v). Finally, the eluate was rotary evaporated to 1 mL for the subsequent GC analysis.
Mild solvent extraction
A mild solvent extraction, butanol extraction, was used to assess the immobilizing efficiencies of PAC and GAC, and expressed as bioavailable fractions.26 Briefly, 2 g of soil samples were extracted with 15 mL butanol in a glass centrifuge tube by shaking on an orbital shaker at 200 rpm for 2 h, followed by centrifugation at 1448×g for 30 min. The butanol supernatants were discarded and the soil, after extraction, was washed by centrifugation with 10 mL deionized water for 10 s. Then, the washed soil was exhaustively extracted by ASE as described above. The concentrations of CBs in the butanol extract were calculated by subtracting the concentration in soil after butanol extraction from the total concentration in soil before extraction.
Volatilization of CBs from soil
At the various sampling points, the volatile fractions of CBs were trapped in two trapping bottles, each containing 15 mL hexane. The two hexane trapping solvents were then pooled together, dried with anhydrous sodium sulfate and concentrated to 1 mL for subsequent GC analysis.
GC analysis
The concentrations of CBs in all the samples were measured by a GC (Agilent 6890, USA) equipped with a DB-5 capillary column (30 m length × 0.32 mm internal diameter × 0.25 μm film thickness), a 63Ni electron capture detector and an HP 7683 auto-sampler. Nitrogen (purity > 99.999%) was used as the carrier gas at a flow rate of 1.5 mL min−1. The column temperature was programmed from 60 °C (1 min) to 140 °C at 20 °C min−1, and then to 280 °C (5 min) at 8 °C min−1. The injector and detector temperatures were 220 °C and 300 °C, respectively, and the injection volume was 1 μL in the splitless mode.
Data analysis
The dissipation of CBs in soil data was fitted into a modified first-order kinetics equation as follows:23 |
C = C0[λ + (1 − λ)e−kt] | (1) |
where C (μg g−1) and C0 (μg g−1) are the concentrations of CBs in soil at time t and time 0 (initial concentration), respectively; λ is the coefficient of non-bioavailable fraction of CBs in soil; and k is the first-order rate constant (week−1).
The extent of the earthworm accumulation of CBs was expressed using a bioaccumulation factor (BAF) as follows:28
where
Cworm (μg g
−1) and
Csoil (μg g
−1) are the concentrations of CBs in earthworm (dry weight) and in soil (dry weight), respectively.
All the statistical data were compared using analysis of variance (ANOVA) and the least significant difference (LSD) post-hoc comparison testing with statistical product and service solutions (SPSS 17.0), and the significance level was p < 0.05.
Results and discussion
Properties of the AC
As shown in Table 1, the AC had a total C content of 89.60%, indicating a high carbonization degree. The high aromaticity of AC could also be ascribed to the low H/C ratio because H is primarily associated with original organic matter.19 The low O/C ratio of AC indicates the low degree of hydrophilicity of the AC.19 As a commercial activated carbon, both PAC and GAC have a large specific surface area and pore volume, indicating that AC has fine-pore structure created through a well-controlled activation process. Due to the high degree of carbonization, in addition to the aromatic rings (1590 cm−1) and aromatic C–H (1200–1110 cm−1) stretching bands, few distinguishable bands for AC were observed between 3600 and 700 cm−1.
Table 1 Physico-chemical properties of powder activated carbon (PAC) and granular activated carbon (GAC)
|
pH |
Elemental composition (%) |
Molar ratio |
Specific surface area (m2 g−1) |
Pore volume (cm3 g−1) |
Pore width (nm) |
C |
H |
O |
N |
H/C |
O/C |
(O + N)/C |
PAC |
7.50 |
89.60 |
3.83 |
4.55 |
0.82 |
0.51 |
0.04 |
0.05 |
796.14 |
0.179 |
3.98 |
GAC |
753.84 |
0.111 |
3.71 |
Dissipation of CBs in soil
To check whether AC could immobilize CBs in soil, the changes in the residues of CBs over time were monitored. As shown in Fig. 1, the dissipations of CBs in PAC or GAC amended treatments were significantly lower than the control (p < 0.05), throughout the whole incubation period. Moreover, the dissipations of CBs in soil fitted well into the modified first-order kinetics model (Table 2). After 48 weeks of incubation, the residues of CBs in soil were in the order of PAC > GAC > Control for each compound. The non-bioavailable coefficient λ in AC amended treatments were significantly higher than that in the Control treatment, indicating that AC amendment reduced the bioavailability of CBs in soil. The value of λ for HCB in PAC treatment was significantly higher than that in GAC treatment, whereas there were no significant differences in the values of λ for TCB between PAC and GAC treatments. This indicates that PAC performed better than GAC in reducing the bioavailability of HCB in soil, while PAC performed equally as GAC in reducing the bioavailability of TCB in soil.
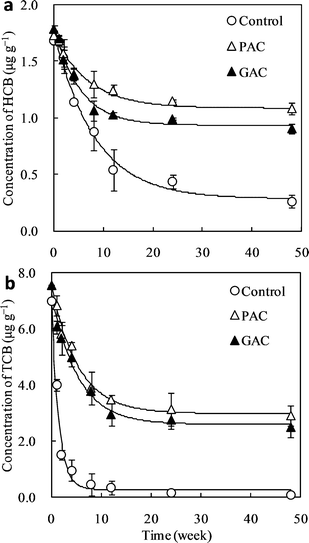 |
| Fig. 1 Time course of the residues of chlorobenzenes in unamended soil (control) or soils amended with powder activated carbon (PAC) and granular activated carbon (GAC) ((a) HCB; (b) TCB; dots: measured data; curves: modified first-order kinetics model fitted; Data of Control before 24 weeks was sourced from previous studies23,25). | |
Table 2 Residues and regression equations of the dissipations of chlorobenzenes from unamended soil (control) or soils amended with powder activated carbon (PAC) and granular activated carbon (GAC)
|
Treatment |
Residue (%) |
Modified first-order kinetics model fitted |
C
0
|
λ
|
k
|
R
2
|
C
0: concentrations of CBs in soil at the start time.
λ: coefficient of non-bioavailable fraction of CBs in soil.
k: first-order rate constant.
|
HCB |
Control |
15.64 ± 3.31 |
1.77 ± 0.16 |
0.16 ± 0.01 |
0.19 ± 0.01 |
0.98 |
PAC |
62.50 ± 2.83 |
1.73 ± 0.09 |
0.63 ± 0.02 |
0.14 ± 0.03 |
0.97 |
GAC |
50.63 ± 2.52 |
1.81 ± 0.08 |
0.52 ± 0.02 |
0.15 ± 0.03 |
0.99 |
TCB |
Control |
1.39 ± 0.80 |
7.06 ± 0.48 |
0.04 ± 0.02 |
0.69 ± 0.08 |
0.99 |
PAC |
38.55 ± 4.53 |
7.52 ± 0.32 |
0.40 ± 0.02 |
0.25 ± 0.02 |
0.99 |
GAC |
32.96 ± 4.49 |
7.27 ± 0.45 |
0.39 ± 0.03 |
0.26 ± 0.03 |
0.98 |
In this study, λ is the non-bioavailable coefficient, which also represents the immobilization ability of the amendments in soil. The lower the chlorination level of CBs, the more hydrophilic and degradable they are in soil under aerobic conditions.29 Therefore, the higher dissipation rate k and lower values of λ were observed for TCB, regardless of the treatment. However, the relative factors of λ in AC amended treatments to unamended treatment were higher for TCB (9.75–10) than that for HCB (3.25–3.94). This indicates that the immobilization abilities of the AC amendment were more pronounced for lower chlorinated (thus volatile) CBs.
Volatilization of CBs from soil
As shown in Fig. 2, the volatilization ratios of both HCB and TCB in the amended treatments were significantly lower than the control, indicating that sorption reduced the volatilization of CBs. The volatile fraction of CBs in the control was in the order of TCB > HCB, agreeing with the order of their vapor pressures (Table S1†). The lower the chlorination level of CBs, the faster their volatilization from soil occurs. After the amendment, the relative reduced percentages of TCB in both PAC and GAC treatments to control were significantly higher than HCB. This demonstrates that AC amendment could effectively reduce the volatilization of volatile CBs and that with higher volatility of CBs, higher is the reduction in volatilization after amendment. The relative reduced percentage of HCB in PAC treatment was significantly higher than that in GAC treatment, whereas there were no significant differences in the relative reduced percentages of TCB in both PAC and GAC treatments. This indicates that PAC performed better than GAC in reducing the volatilization of HCB from soil, while PAC performed equally as GAC in reducing the volatilization of TCB from soil.
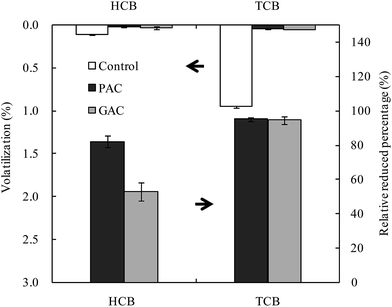 |
| Fig. 2 Cumulative volatilization of chlorobenzenes from unamended soil (control) or soils amended with powder activated carbon (PAC) and granular activated carbon (GAC) after 24 weeks of incubation, and the relative reduced percentages in PAC and GAC treatments to control. Data of control was sourced from previous studies.23,25 | |
Butanol extraction of CBs from soil
The amendment resulted in the high residues of CBs in soils (Fig. 1); however, these total concentrations of CBs extracted by the exhaustive solvent extraction, such as ASE, could not reflect the actual bioavailable concentrations of CBs in soil.30 However, the mild solvent extraction, such as butanol extraction, reflects the changes of the bioavailability of CBs in amended soil.23 The butanol extraction efficiencies of CBs in PAC and GAC treatments were significantly lower than those in the control at each interval (Fig. 3), indicating that amendment with AC could effectively reduce the bioavailability of CBs in soil. There were no significant differences in the butanol extraction efficiencies between PAC and GAC treatments for each compound, indicating the equivalent effect of PAC and GAC. After aging for 1 and 48 weeks of incubation, the relative reduced percentages of HCB in PAC and GAC treatments to control were lower than that for TCB, indicating that the immobilizing effect of the amendments was more apparent for TCB. The lower chlorinated CBs had relatively higher aqueous solubility (Table S1†) and faster mass transfer, which enabled them to easily diffuse to the AC particles in soil and get strongly sorbed. A similar congener effect has also been observed in studies with PCDD/Fs and PCBs in soils.15,17
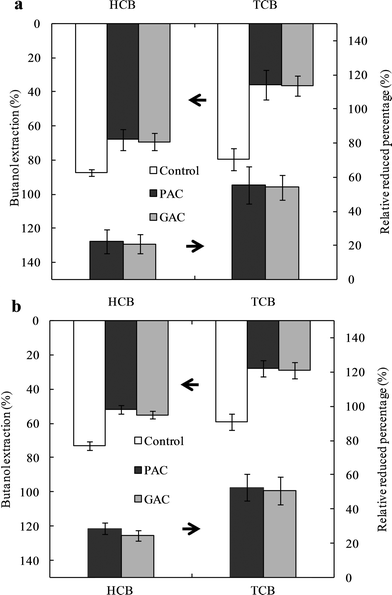 |
| Fig. 3 Butanol extraction of chlorobenzenes from unamended soil (control) or soils amended with powder activated carbon (PAC) and granular activated carbon (GAC) after 1 week (a) and 48 weeks (b) of incubation, and the relative reduced percentages in PAC and GAC treatments to Control. Data of control in (a) was sourced from previous studies.23,25 | |
Earthworm accumulation of CBs in soil
The BAFs of CBs in PAC and GAC treatments were significantly lower than those in the control, regardless of incubation time, indicating that amendment with AC could effectively reduce the earthworm accumulation of CBs (Fig. 4). There were no significant differences of the BAFs between PAC and GAC treatments for each compound, indicating that PAC and GAC performed equally in reducing the accumulation of CBs by earthworms. As shown in Fig. 4, the relative reduced percentages of BAFs in PAC and GAC treatments to control after 1 week of incubation were 48–53%, which were increased to 80–97% after 48 weeks of incubation. This indicates that the long term effect of AC amendments on reducing the bioavailability of CBs in soil was more apparent than the short term effect. This could be ascribed to the fact that the volatile compounds, such as CBs, could diffuse in soil towards amended particles and get strongly sorbed over a period of time.15 Moreover, after 48 weeks of incubation, the reductions in the BAFs of TCB were higher than that for HCB, confirming that the lower chlorinated CBs experienced more efficient immobilization by AC.
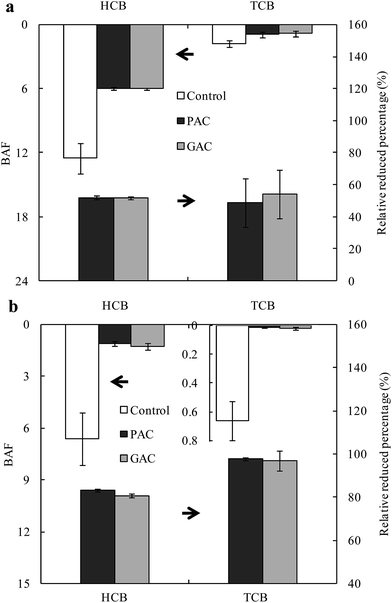 |
| Fig. 4 Bioaccumulation factors (BAF) of chlorobenzenes in earthworm from unamended soil (control) or soils amended with powder activated carbon (PAC) and granular activated carbon (GAC) after 1 week (a) and 48 weeks (b) of incubation, and the reduced percentages in PAC and GAC treatments compared to control. Data of control in (a) was sourced from previous studies.23,25 | |
Environmental implication
The low volatilization (Fig. 2), low butanol extraction (Fig. 3) and low earthworm accumulation (Fig. 4) of CBs in amended treatments, all of these indicate that a low level of AC amendment of soil could effectively immobilize CBs, especially the more volatile CBs. Both in PAC and GAC treatment, the relative reduced volatilization, relative reduced butanol extraction and relative reduced BAFs for HCB were lower than those for TCB. Therefore, for hydrophobic volatile compounds, AC amendment could be an effective in situ soil remediation strategy. Immobilization is a less destructive strategy compared to the existing alternatives of ex situ remediation such as removal and landfill application or incineration. Immobilization results in the reduced bioavailability of contaminants in soil and reduced uptake by both flora and fauna.31 Moreover, it is reported that amendment with AC does not reduce the degradation potential of PAHs in soil.32 It has been shown that a biofilm, which is not influenced by pollutants, could be formed on the surface of AC.33 Black carbon can also be used as a bacteria carrier for bioaugmentation,34 and this offers remediation possibilities for volatile compounds: AC immobilizes the volatile compounds in soil and then the inoculated bacteria degrades them. This is a potentially effective and sustainable approach for the elimination of volatile compounds in soil while avoiding air pollution caused by the soil–air exchange of volatile compounds.
In this study, for HCB, differences between PAC and GAC treatments were visible as higher residues and lower volatilization of HCB in PAC than those in GAC, but not for butanol extraction and earthworm accumulation of HCB. However, for TCB, PAC showed no significant differences with GAC in residues, volatilization inhibition and bioavailability reduction, confirming the hypothesis that GAC performed equally as PAC in immobilizing volatile compounds in soil. Some studies reported that with smaller particle size, more effective is AC in reducing the bioavailability of contaminants,13 but these studies were based on low-volatile compounds such as PAHs.18 However, for volatile compounds, such as TCB, they could diffuse in soil towards GAC particles and get sorbed. It has been reported that at longer contact times, the effectiveness of small-sized and large-sized ACs can be compared for reducing the bioavailability of organic contaminants.15 The results suggest that for the immobilization of volatile compounds, 1% application rate of GAC was considerably effective. The addition of PAC to soil may affect the soil structure and ecological environment. For example, PAC amendment had a negative effect on plant growth, whereas GAC increased the growth rate of plants.18 PAC may have clogged the soil pores, which are initially filled with air and water, leading to less aeration and a higher soil density. However, GAC amendment resulted in a coarser and more aerobic soil structure.18 Note that PAC may leach and possibly contaminate ground water.20 Therefore, considering the ecological risk, GAC is better than PAC in immobilizing volatile compounds in soil.
Conclusions
AC could effectively immobilize CBs in soil, and the amendment of soil with AC significantly reduced the volatilization and bioavailability of CBs compared to the unamended soil, especially for the more volatile 1,2,4-TCB. GAC and PAC showed an equivalent efficacy in the immobilization of more volatile compounds.
Acknowledgements
This research was financially supported by the National Natural Science Foundation of China (41301240), the Specific Fund for Agro-Scientific Research in the Public Interest (201203045), the National Key Basic Research Program of China (2014CB441105), and the Natural Science Foundation of Jiangsu Province (BK20131049).
References
- P. Rapp, Appl. Environ. Microbiol., 2001, 67, 3496–3500 CrossRef CAS PubMed.
- R. Schroll, F. Brahushi, U. Dörfler, S. Kuhn, J. Fekete and J. C. Munch, Environ. Pollut., 2004, 127, 395–401 CrossRef PubMed.
- M. Y. Zhang and Y. M. Lv, Chlor-Alkali Ind., 2005, 1, 26–32 Search PubMed.
- J. Y. Zhang, W. Zhao, J. Pan, L. M. Qiu and Y. M. Zhu, Environ. Int., 2005, 31, 855–860 CrossRef CAS PubMed.
- Q. P. Du, X. S. Ra and C. N. Huang, J. Environ. Sci., 2007, 19, 1171–1177 CrossRef CAS.
- X. Zhou, G. Yu, Z. L. Zhang and J. F. Niu, Environ. Sci., 2005, 26, 117–120 CAS.
- Y. Song, F. Wang, Y. Bian, Y. Zhang and X. Jiang, J. Environ. Sci., 2012, 24, 362–368 CrossRef.
- F. O. Kengara, U. Doerfler, G. Welzl, B. Ruth, J. C. Munch and R. Schroll, Environ. pollut., 2013, 173, 168–175 CrossRef CAS PubMed.
- I. Josko, P. Oleszczuk, J. Pranagal, J. Lehmann, B. Xing and G. Cornelissen, Ecol. Eng., 2013, 60, 50–59 CrossRef PubMed.
- T. K. Udeigwe, P. N. Eze, J. M. Teboh and M. H. Stietiya, Environ. Int., 2011, 37, 258–267 CrossRef CAS PubMed.
- R. N. Millward, T. S. Bridges, U. Ghosh, J. R. Zimmerman and R. G. Luthy, Environ. Sci. Technol., 2005, 39, 2880–2887 CrossRef CAS.
- X. Sun and U. Ghosh, Environ. Sci. Technol., 2007, 41, 4774–4780 CrossRef CAS.
- J. E. Tomaszewski, D. Werner and R. G. Luthy, Environ. Toxicol. Chem., 2007, 26, 2143–2150 CAS.
- J. R. Zimmerman, U. Ghosh, R. N. Millward, T. S. Bridges and R. G. Luthy, Environ. Sci. Technol., 2004, 38, 5458–5464 CrossRef CAS.
- Y. Chai, R. J. Currie, J. W. Davis, M. Wilken, G. D. Martin, V. N. Fishman and U. Ghosh, Environ. Sci. Technol., 2012, 46, 1035–1043 CrossRef CAS PubMed.
- M. J. Denyes, A. Rutter and B. A. Zeeb, Environ. Pollut., 2013, 182, 201–208 CrossRef CAS PubMed.
- P. Paul and U. Ghosh, Environ. Pollut., 2011, 159, 3763–3768 CrossRef CAS PubMed.
- L. Jakob, T. Hartnik, T. Henriksen, M. Elmquist, R. C. Brandli, S. E. Hale and G. Cornelissen, Chemosphere, 2012, 88, 699–705 CrossRef CAS PubMed.
- Y. Chun, G. Y. Sheng, C. T. Chiou and B. S. Xing, Environ. Sci. Technol., 2004, 38, 4649–4655 CrossRef CAS.
- S. E. Hale, M. Elmquist, R. Brandli, T. Hartnik, L. Jakob, T. Henriksen, D. Werner and G. Cornelissen, Chemosphere, 2012, 87, 177–184 CrossRef CAS PubMed.
- K. M. Bushnaf, S. Puricelli, S. Saponaro and D. Werner, J. Contam. Hydrol., 2011, 126, 208–215 CrossRef CAS PubMed.
-
H. M. Malcolm, P. D. Howe and S. Dobson, Concise International Chemical Assessment Document 60, World Health Organization, 2004 Search PubMed.
- Y. Song, F. Wang, Y. Bian, F. O. Kengara, M. Jia, Z. Xie and X. Jiang, J. Hazard. Mater., 2012, 217–218, 391–397 CrossRef CAS PubMed.
- X. Chen, G. Chen, L. Chen, Y. Chen, J. Lehmann, M. B. McBride and A. G. Hay, Bioresour. Technol., 2011, 102, 8877–8884 CrossRef CAS PubMed.
- Y. Song, F. Wang, F. O. Kengara, X. Yang, C. Gu and X. Jiang, J. Agric. Food Chem., 2013, 61, 4210–4217 CrossRef CAS PubMed.
- Y. Song, F. Wang, X. Yang, C. Liu, F. O. Kengara, X. Jin and X. Jiang, J. Soils Sediments, 2011, 11, 1345–1354 CrossRef CAS PubMed.
- J. X. Tang, B. K. Robertson and M. Alexander, Environ. Sci. Technol., 1999, 33, 4346–4351 CrossRef CAS.
- B. Wen, R. J. Li, S. Z. Zhang, X. Q. Shan, J. Fang, K. Xiao and S. U. Khan, Environ. Pollut., 2009, 157, 968–974 CrossRef CAS PubMed.
- J. A. Field and R. Sierra-Alvarez, Biodegradation, 2008, 19, 463–480 CrossRef CAS PubMed.
- M. Alexander, Environ. Sci. Technol., 2000, 34, 4259–4265 CrossRef CAS.
- B. J. Reid, K. C. Jones and K. T. Semple, Environ. Pollut., 2000, 108, 103–112 CrossRef CAS.
- P. Meynet, S. E. Hale, R. J. Davenport, G. Cornelissen, G. D. Breedveld and D. Werner, Environ. Sci. Technol., 2012, 46, 5057–5066 CrossRef CAS PubMed.
- A. Mercier, G. Wille, C. Michel, J. Harris-Hellal, L. Amalric, C. Morlay and F. Battaglia-Brunet, J. Soils Sediments, 2013, 13, 793–800 CrossRef CAS.
- B. Chen, M. Yuan and L. Qian, J. Soils Sediments, 2012, 12, 1350–1359 CrossRef CAS.
Footnote |
† Electronic supplementary information (ESI) available. See DOI: 10.1039/c4em00486h |
|
This journal is © The Royal Society of Chemistry 2015 |
Click here to see how this site uses Cookies. View our privacy policy here.