DOI:
10.1039/C4AY01919A
(Paper)
Anal. Methods, 2015,
7, 187-196
Development and application of an ELISA method for the analysis of protein-based binding media of artworks
Received
14th August 2014
, Accepted 11th November 2014
First published on 24th November 2014
Abstract
ELISA has been used extensively in scientific research and medical diagnostics since its invention in the 1960s. The application of ELISA to identify proteinaceous materials used in works from cultural heritage is a recent development and presents an important area of further investigation. This study introduces an optimized ELISA with a horse radish peroxidase (HRP) reporting system to identify select proteins in the adhesives and binders used in artworks. The described methodology is successfully applied to samples obtained from three different artworks from the Metropolitan Museum of Art. Furthermore, these experiments use protein quantitation to study the impact of specific pigments/binder combinations on the antigen detection by commercial antibodies. Our results suggest that the aging of specific pigment/binder combinations could impact the ELISA detection of proteins in artworks.
Introduction
Organic materials have been used in cultural heritage since ancient times as binders, adhesives, coatings, and colorants. More specifically, proteinaceous materials derived from animal products (eggs and milk) and tissues (skin, bones, tendons, swim bladder) were common materials used by artists. Their accurate identification is important for conservation practices and for the technical study of artworks. Conventional technologies used by cultural heritage institutions to identify and analyze organic substances present in artworks include spectroscopic techniques such as Fourier transform infrared spectroscopy (FTIR) and chromatographic techniques such as high performance liquid chromatography (HPLC) as well as gas chromatography (GC) and pyrolysis gas chromatography (PyGC) in combination with mass spectrometry (MS).1,2 FTIR can describe, in general, the class of the material (i.e. protein, oil, resin, etc.), whereas chromatographic methods provide more specific information about the identity of the organic material (i.e. animal glue, linseed oil, dammar resin, etc.). However, the limited sample size allowed from artworks coupled with the low concentration of organic materials in paints and preparation layers, the intrinsic complexity of the naturally occurring material, degradation from natural aging, and the presence of inorganic compounds all present technological challenges for the identification of proteinaceous media. In addition, animal-derived materials can be obtained from different animal sources and artists can mix these with other organic materials (i.e. egg and oil, or casein and gum, for example), generating heterogeneous materials.3,4 These challenges make the detection of proteinaceous materials in art difficult.1,5–7
Recently there have been advances in two new directions for protein analysis applied to art: mass-spectrometry and immunology. Developments in matrix assisted laser-desorption ionization (MALDI) and liquid chromatograph (LC) techniques coupled to tandem MS have been used to analyze extracts from art samples where the proteins are cleaved into smaller pieces (peptides) before analysis. These techniques offer an in-depth method of protein and species identification and the ability to assess specific protein degradation pathways.8–12 However, these techniques are not widely available to many cultural institutions due to the high cost of the instrumentation and the high level of expertise required for data analysis.
The application of immunological based techniques has emerged as a specific method for protein and polysaccharide identification in artworks.13–16 One of the most commonly used immunological methods is enzyme-linked immunosorbent assay (ELISA), which uses antibodies coupled to an enzyme reporting system to selectively identify target proteins.17,18 Antibodies are produced by living organisms as part of their immune response and recognize specific recognition sites (epitopes) on foreign molecules (antigens). The antibodies express a high degree of chemical specificity and affinity to their targets19 and coupled with an appropriate reporting system, can be extremely sensitive. Two of the most popular enzyme reporting systems are alkaline phosphatase (AP) and horse radish peroxidase (HRP). While both have been used successfully in the biological community, up until now only the use of AP has been reported in the literature for ELISA applied to cultural heritage.15,16 In this paper we use HRP as a reporting system because its application in biological research is well established,20 it has been reported to be more sensitive than AP, and it offers a greater range of amplifying possibilities.18 Furthermore, in a recent survey it was reported that the HRP reporting system is more commonly used in ELISA assays than the AP reporting system.21 We also found HRP to give more consistent results than AP for our application. The optimized ELISA methodology was used for successful identification of binding media, adhesives and coatings of three samples obtained from artworks at the Metropolitan Museum of Art (MMA): an adhesive sample from a 12–13th century Feathered Tabard from South America, a sized paper sample from an early 20th century Tiffany watercolor and a multilayered paint sample from a 14th century banner painting.
While ELISA has been shown to be able to identify protein and protein mixtures from artworks as old as 200 B.C.,15 there are still important factors that can impact the success of the technique. An important consideration for the application of ELISA to the study of artworks is the influence of specific pigments on the ELISA response. While, past experiments have shown that ELISA can be used successfully to detect small amounts of proteins in mixtures15 and in the presence of specific pigments,16 to the knowledge of the authors, the influence of different pigments on the ELISA response from recent and naturally aged replica paints have not been thoroughly examined. Prior studies with GC-MS suggest that protein modifications such as crosslinking, hydrolysis, and oxidation can be catalyzed by metal cations such as calcium, copper and iron22 and occur at the amino acid level.5 These modifications can cause changes in the protein structure and affect the recognition of a target protein by an antibody. Furthermore, studies on tissue samples by immunohistochemistry have demonstrated that antigens can be masked by the presence of calcium ions and suggest that calcium induced molecular modification can diminish antigen–antibody recognition.23 In order to understand why the ELISA response can be impacted by certain protein/pigment combinations, it is critical to establish if the presence of metal ions arising from certain pigments inhibit the ELISA assay. Toward this end, we have developed a robust ELISA system and have introduced the use of protein quantitation with NanoOrange® to characterize the influence of specific pigments on the ELISA response in both recent and naturally aged replica paints. These studies are a step towards making the ELISA methodology more routine in the identification of proteinaceous materials in the specific context of cultural heritage.
Materials and methods
Materials
Standard and replica samples.
Protein standards used were gelatin from porcine skin type A p300 (Sigma, G2500), casein (Kremer, 63200), albumin from chicken egg white (Sigma, A2512), gum arabic (Kremer), and gum tragacanth (Sigma, G1128). Binders of whole hen egg, animal skin glue, and casein were used to create replica paints that were applied on glass slides, dried naturally, and aged for three years. The pigments used to prepare the paints were lead white (Kremer, 46000), ultramarine (Kremer, 4500), chalk from Bologna (Sinopia), and French ochre JTCLES (Kremer, 40010). It is important to mention that these modern pigments are thought to be more pure than those made by artists in the 15th century. All pigments were characterized with X-ray fluorescence and X-ray diffraction (data not shown). The eggs were purchased from a local market and prepared by passing the entire contents of an egg through a sieve. For the egg paints, the pigments were pre-wetted with water and then mixed with binder until the desirable properties were achieved. The animal skin glue was made by soaking 17.5 g of rabbit skin glue and 17.5 g of bovine hide glue (both from Kremer) in 500 mL of water overnight. The glues were heated at 60 °C until dissolved. The casein binder can be prepared by several methods.24 For these experiments, casein was prepared with the addition of borax and will be referred to as borax casein. These binders are also not chemically pure. The binders extracted from animal skins or processed from bovine milk contain more proteins than collagen type I or casein, respectively. Binders made from whole egg also contain more proteins than ovalbumin. Thus, the replica paints used in these experiments are more similar to authentic paints than model paints prepared from pure chemicals.
Antibodies.
Primary and secondary antibodies and their respective blocking buffers are listed in Table 1. Dilutions for each antibody were determined experimentally based on suggestions provided by the manufacturer. The primary antibodies for collagen type I (Abcam, ab292), casein (GeneTex, GTX77267), and ovalbumin (Chemicon, AB1225) are polyclonal. It is very likely that in materials from cultural heritage there are chemical modifications of the protein of interest. The multiple epitopes recognized by polyclonal antibodies increase the chances of detection of native and modified proteins and offer a clear advantage over monoclonal antibodies. Species specificity is another important considerations. The rabbit anti-collagen type I antibody, for example, recognizes mammalian as well as fish collagen type I (data not shown) and can serve as a general screening antibody for the presence of animal glue. The casein antibody reacts with casein in bovine milk. The rabbit polyclonal antibody to chicken ovalbumin was used to determine the presence of egg white protein. Although the anti-ovalbumin antibody is specific for ovalbumin, present in egg white, we have consistently detected ovalbumin in egg yolk paints because egg yolk cannot be effectively separated from egg white manually. The primary antibodies for arabinogalactan gums (CarboSource, JIM 13) and gum tragacanth (CarboSource, MAC 265) are only available commercially as monoclonal antibodies. JIM 13 antibody reacts with certain carbohydrate epitopes on arabinogalactan, but the precise features of these epitopes have yet to be characterized.25 The MAC 265 antibody was used to determine the presence of gum tragacanth. The product literature from CarboSource indicates that the MAC 265 antibody binds to an epitope found only in gum tragacanth. The HRP-conjugated goat anti-rabbit IgG (H+L) polyclonal secondary antibody (GeneTex, GTX85323) was used for the detection of ovalbumin, casein, and collagen type I. The HRP-conjugated goat anti-rat IgG (H+L) polyclonal antibody (Southern Biotech, 3050-05) was used for the detection of gums.
Table 1 List of primary and secondary antibodies, their corresponding binders, dilutions, and blocking buffers
Primary antibody |
Primary antibody dilution |
Binder recognition |
Primary antibody blocking buffer (in 1× PBS) |
HRP-conjugated secondary antibody |
Secondary antibody dilution |
Secondary antibody blocking buffer (in 1× PBS) |
The anti-ovalbumin antibody detects ovalbumin from egg white (there is no ovalbumin present in egg yolk). However, we have consistently detected ovalbumin in egg yolk samples and paints with the anti-ovalbumin antibody because egg white cannot be cleanly separated by hand from egg yolk.
|
Rabbit anti-collagen type I |
1 : 500 |
Mammalian/fish glues |
10% Sea block |
Goat-anti-rabbit |
1 : 10 000 |
30% Sea block |
Rabbit anti-casein |
1 : 10 000 |
Milk glue |
10% Sea block |
Goat-anti-rabbit |
1 : 10 000 |
30% Sea block |
Rabbit anti-ovalbumin |
1 : 1000 |
Egg white in egg bindera |
5% Newborn calf serum |
Goat-anti-rabbit |
1 : 10 000 |
5% Newborn calf serum |
Rat anti-JIM 13 |
1 : 10 |
Arabinogalactans |
5% Newborn calf serum |
Goat-anti-rat |
1 : 1000 |
5% Newborn calf serum |
Rat anti-MAC 265 |
1 : 10 |
Gum tragacanth |
5% Newborn calf serum |
Goat-anti-rat |
1 : 1000 |
5% Newborn calf serum |
Experimental procedure
Protein extraction.
Three extraction procedures were used to determine the best method for extracting proteinaceous materials from replica paint samples. The first extraction procedure was based on a method used for sample preparation with MALDI.26 The samples were extracted with equal volumes of 50 mM ammonium bicarbonate (Ambi) and trifluoroethanol (TFE) and heated at 60 °C for one hour with repeated vortexing. The samples were then sonicated in a water bath sonicator for approximately 5 minutes and then were heated at 60 °C for an additional 15 minutes. TFE was evaporated and additional 50 mM Ambi was added to restore solutions to the original volume. The samples were heated at 60 °C for a final 30 minutes and spun in a microcentrifuge at 13
600g to remove any insoluble particles from the solution. The other two extraction methods were carried out with 2.5 M NH3 (ref. 7 and 11) and 1% trifluoroacetic acid (TFA),9 respectively. The samples were sonicated in a water bath sonicator for 45 minutes and spun in a microcentrifuge at 13
600g to remove any insoluble particles from the solution.
Protein quantification.
Only very small samples are obtained from artworks, therefore a sensitive protein quantitation technique was required that would allow protein quantification at a low concentration. NanoOrange® (Invitrogen),27 a fluorescence based technique with sensitivity between 0.01 and 20 μg mL−1 for protein quantification, fulfilled these requirements. The sensitivity of the assay proved to be between 0.1 and 20 μg mL−1 for the parameters of our instrument. Standard preparation for calibration curves and protein quantification of samples were performed according to the protocol provided by the manufacturer with a volume modification for a 96 black microwell plate (BD Falcon). All measurements were performed in triplicate; 150 μL of standards and samples were used per well. The readings were taken with the following parameters: excitation at 470 nm, emission at 590 nm with the cutoff at 570 nm. A four-parameter (quadratic) curve-fitting algorithm was used to obtain concentrations of the unknown samples.
ELISA.
ELISA was performed in a 96 microwell plate (Nunc MaxiSorp® flat-bottom, Thermo Scientific). All samples and controls were diluted in carbonate–bicarbonate buffer (CBB) (pH 9.6 at 25 °C) (Sigma). Three sets of control wells without the antigen contained CBB, primary antibody, or secondary antibody. The background determined for each primary antibody was the absorbance value of primary and secondary antibodies together in the absence of an antigen. All unknown samples and controls were run in triplicate.
First, 50 μL of the antigen was plated per well and incubated overnight at 37 °C. The unbound antigen was removed by washing the wells with 150 μL of 1× phosphate buffered saline (PBS) five times. One hundred microliters of blocking buffer was then added to each well for one hour at room temperature (RT). The choice of blocking buffer is different for each type of primary antibody as listed in Table 1. A volume of 50 μL of primary antibody was then added to each well and incubated overnight at 4 °C. Unbound primary antibody was removed by washing each well with 150 μL of washing buffer (PBS with 0.1% Tween-20) for five times, and 50 μL of secondary antibody (horseradish peroxidase (HRP) labeled) was added and allowed to incubate at 37 °C for 90 minutes. The wells were washed with 150 μL of washing buffer five times. A final wash with 150 μL of 1× PBS was performed to remove trace amount of Tween-20. For colorimetric development with 3,3′,5,5′-tetramethylbenzidine substrate (TMB) (1-Step™ Ultra TMB, Pierce), 50 μL of the reagent was added per well. The development time ranged from 5 to 15 minutes and depended on the colorimetric development of the unknowns. Equal volume of 1 N sulfuric acid was added to stop the reaction and the optical density (absorbance) was measured with a spectrophotometer (SpectraMax™ M3, Molecular Devices) at 450 nm. For colorimetric development with 2,2′-azino-bis(3-ethylbenzothiazoline-6-sulphonic acid) (ABTS) substrate, ABTS powder (Southern Biotech) was dissolved in phosphate–citrate buffer (pH 4.8) to a final concentration of 0.5 mg mL−1. Right before use, 0.003% hydrogen peroxide was added to the ABTS solution (HRP catalyzes hydrogen peroxide oxidation of the substrates). Fifty microliters of ABTS/hydrogen peroxide solution were added per well. The development time ranged from 10 to 20 minutes. The reaction was stopped with an equal volume of 0.5 mg mL−1 of sodium azide in phosphate–citrate buffer (pH 4.8). The optical density was measured with the spectrophotometer at 410 nm.
ELISA threshold determination.
As mentioned previously, the background for the ELISA was determined by the absorbance of the primary and secondary antibodies without the antigen. The threshold values were calculated as the average background absorbance reading plus three standard deviations (3σ). Threshold values varied for each primary antibody.
Removal of free ions from solution.
Samples of paints prepared with animal skin glue or whole egg mixed with chalk and borax casein mixed with French ochre were selected to test the influence of ion removal in solution on the ELISA response. The samples were extracted with Ambi/TFE conditions to a concentration of 1 μg μL−1 (by weight). The protein concentration measurement was taken with NanoOrange® before and after the clean-up procedure to determine if changes in the protein concentration occurred. Once the samples were cleaned-up, they were diluted in CBB to 0.25 μg mL−1 based on the protein quantification performed before the sample clean-up. The samples were screened for the presence of collagen type I, casein, and ovalbumin with ELISA. Extracted samples were divided in equal volumes to compare five different clean-up procedures.
Amicon® Ultra.
Ambi/TFE extracted samples were added to the Amicon® Ultra 3K (Millipore UFC). The samples were spun at 13
000g for 10 minutes. The volume of the discarded solution was added back to the protein solution with 50 mM Ambi to ensure that the protein concentration remained the same.
OMIX® C4 tips.
TFA was added to the Ambi/TFE extracted samples to a final concentration of 0.1%. The OMIX® C4 (Agilent Technologies) tip was wetted with 50% acetonitrile/water solution and equilibrated with 0.1% TFA solution as specified by the manufacturer protocol. The samples were applied by aspirating and dispensing the extracted protein solution through the tip for 10 cycles. The tip was washed with 0.1% TFA and the bound compounds eluted with 0.1% TFA in 50% acetonitrile solution.
EDTA.
A 0.5 M ethylenediaminetetraacetic acid (EDTA) (Sigma) solution was added to the Ambi/TFE extracted samples to obtain 25 mM and 100 mM final concentration of EDTA.
Zeba™ Spin Desalting Column.
After the storage buffer was removed from the Zeba™ Spin Desalting Column 7K (Thermo) as described by the manufacturer's protocol, Ambi/TFE extracted samples were placed in the column and spun at 1500g for 2 minutes.
Analysis of samples from artworks using ELISA.
ELISA was performed on samples obtained from the three following artworks from the Metropolitan Museum of Art. All the samples were diluted in CBB to a concentration of 100 μg mL−1 (by weight). The antibody dilution and parameters are mentioned in the Materials and methods section and TMB substrate was used for colorimetric development. No protein quantitation was performed in these experiments due to limited availability of sample.
The first art sample analyzed by ELISA was an adhesive sample from a 12–13th century Feathered Tabard (a short sleeved or sleeveless coat) (MMA Accession 59.135.8). It is thought to be from the Ica culture, a cultural region of Chimú in Peru. FTIR showed the sample to be unpigmented and homogenous (data not shown). A 120 μg sample from the Feathered Tabard was dissolved in 1× PBS at 37 °C for 30 minutes and screened for the presence of animal collagen, casein and ovalbumin.
The second sample was a sample of paper attached to an artist’s board obtained from a Tiffany watercolor drawing (MMA Accession 67.654.226). The drawing was executed on an artist board, comprised of a high quality watercolor Whatman paper attached to a highly lignified laminated board. The watercolor painting is thought to be made possibly by the Tiffany Studios (1902-32). Approximately 300 μg of sample was extracted using the Ambi/TFE conditions and screened for the presence of animal collagen and casein.
The third sample was a microscopic detached fragment from a 14th century double-sided processional banner by Spinello Aretino (b. 1345–52, d. 1410); it depicts Saint Mary Magdalen Holding a Crucifix on one side and The Flagellation on the reverse (MMA Accession 13.175). A paint sample fragment containing all of the layers from the ground up to top paint and varnish layers (130 μg) was extracted using the Ambi/TFE conditions and screened for the presence of animal collagen, casein, ovalbumin, and general gums.
Results and discussion
The optimization of ELISA methodology
Samples from artworks are usually small, tens to a few hundred micrograms, and the binder is typically less than 10% of the mass.2 Original paint layers based on proteins or gums can become infiltrated with non-aqueous soluble materials such as natural and synthetic resins and oils from varnishes or paints applied during the artwork's lifetime. Furthermore, it has been suggested that metal cations reduce the solubility of proteinaceous materials.3,22 Thus, a proper extraction method that can be applied to heterogeneous and diverse materials found in artworks is necessary in order to maximize the amount of protein recovered for the ELISA analysis. For these reason, we compared three extraction conditions: 1% TFA, 2.5 M NH3, and Ambi/TFE. Generally, we find that the Ambi/TFE extraction method is the most comprehensive with regards to the extraction of protein, most likely due to the presence of TFE. This is supported in the literature, where it has been suggested that a high concentration of TFE (40–50% v/v) can reduce the dielectric constant of the solubilization medium and improve the protein extraction efficiency.28 TFE has also been shown to be a more effective method to extract proteins from micro- and nanoscale samples than a more traditional detergent based methods.29 Furthermore, the successful use of the Ambi/TFE procedure for the identification of adhesives and binders from diverse and mixed media found in artworks was demonstrated.27 For these reasons, we chose the Ambi/TFE extraction for the subsequent ELISA experiments.
In addition to the extraction protocol, we optimized the ELISA specifically for five primary antibodies that were used to detect collagen type I, casein, ovalbumin, arabinogalactans and gum tragacanth. Ideally, antibody dilutions for both the primary and secondary antibodies should be determined for each new antibody lot and depend on the concentration of the antibodies supplied by the manufacturer. Protein standards were used as target antigens that represent proteins found in artworks. The optimized parameters are listed in Table 1 and were chosen to give high absorbance values and produce the lowest background signal. Other parameters that were evaluated and optimized were antibody incubation times and temperatures, blocking agents, the number and type of washing steps, and even the plate type.
We originally tested both AP and HRP reporting systems, but chose to develop an ELISA with the HRP reporting system because it gave the most consistent results. We wanted to retain the accessibility of a colorimetric assay and initially adopted the popular substrate 2,2′-azino-bis(3-ethylbenzothiazoline-6-sulphonic acid) (ABTS). However, newer substrates are now available and we achieved higher sensitivity in the detection of target antigens by their respective primary antibodies with the substrate 3,3′,5,5′-tetramethylbenzidine (TMB). The sensitivity of both substrates was comparatively measured for the detection of collagen type I, casein, ovalbumin, arabinogalactans and gum tragacanth with their respective primary antibodies (Fig. 1). Protein standards were used as target antigens at the following concentrations: 1, 0.1, 0.01 and 0.001 μg mL−1. Absorbance values obtained for the five selected primary antibodies with TMB were greater than those obtained with ABTS for all antigen concentrations tested (Fig. 1a–e). Absorbance values for each antibody fell as the concentration of the antigen decreased. With the exception of gum tragacanth and anti-collagen type I antibodies, the primary antibodies gave a robust ELISA signal at 0.01 μg mL−1 with the TMB substrate. With the gum tragacanth antibody, a significant decrease in signal occurred from 1 μg mL−1 to 0.1 μg mL−1 (Fig. 1e). These results demonstrate that for these applications the TMB substrate is more sensitive than ABTS for use with the HRP-based ELISA system. It is important to note that because the antigen–antibody recognition varies with different primary antibodies, quantitative comparison of proteins in a sample with different primary antibodies cannot be made.
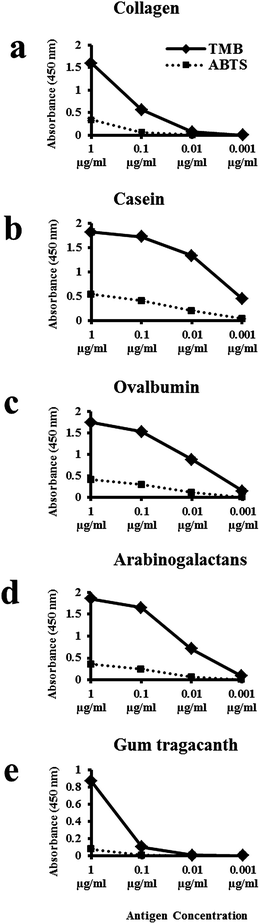 |
| Fig. 1 Sensitivities of two HRP substrates (TMB and ABTS) were tested for (a) collagen type I, (b) casein, (c) ovalbumin, (d) arabinogalactans and (e) gum tragacanth. Results shown in graphs (a) to (e) represent one experiment conducted in triplicate. The standard deviation for the three experiments is too small to be visible on this scale. In general, the TMB substrate is more sensitive to lower concentrations of the five antigens tested than the ABTS substrate. | |
Analysis of artwork samples
To test our optimized ELISA methodology on real artworks, we obtained a small adhesive sample from an archaeological Peruvian Feathered Tabard, 12th–13th century AD and used ELISA to determine if it contained gum or animal-glue based binder (Fig. 2a). Adhesive identification was assessed because prior analysis by FTIR of an adhesive sample obtained from a feathered object from a different region showed it to be animal-based rather than gum-based. Therefore, the determination of the adhesive's source would be important for understanding the temporal and regional differences within the Andean cultural region in crafting these magnificent feathered objects. FTIR analysis indicated the presence of gums (data not shown) however ELISA analysis was also performed as small concentrations of protein could have been masked. When the adhesive sample was screened for the presence of animal glue, arabinogalactan gums and gum tragacanth, the ELISA result indicated that the adhesive is arabinogalactan gum-based and is not animal glue or tragacanth-gum based (Fig. 3a).
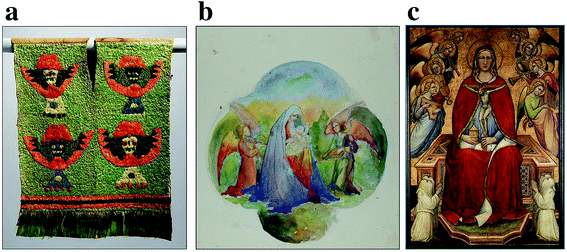 |
| Fig. 2 Works of art from the Metropolitan Museum of Art. (a) Feathered Tabard, Peru, Ica (?) culture; 12th–13th century; cotton and feathers; Fletcher Fund, 1959 (accession number: 59.135.8). (b) Design for window possibly by Tiffany Studios (1902–32). Watercolor and graphite on paper mounted on board; Walter Hoving and Julia T. Weld Gifts and Dodge Fund, 1967 (accession number: 67.654.226). (c) Saint Mary Magdalen Holding a Crucifix; (reverse) The Flagellation by Spinello Aretino, 1395–1400; tempera on canvas, gold ground; gift of the family of Francis M. Bacon, 1914 (13.175). All images copyright The Metropolitan Museum of Art. | |
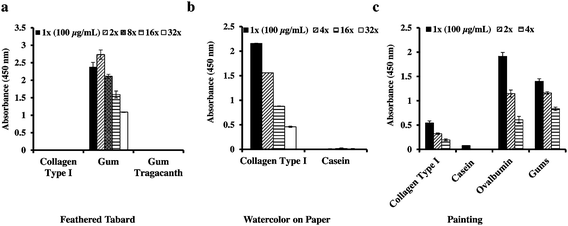 |
| Fig. 3 ELISA analysis of binders or adhesives from (a) a Feathered Tabard (12–13th century), (b) a drawing made possibly by the Tiffany Studios (1902–32), and (c) a multi-layer paint sample from the side of the Magdalen from the 14th-century double-sided processional banner, Saint Mary Magdalen Holding a Crucifix; (reverse) The Flagellation, by Spinello Aretino. The threshold values were subtracted from the absorbance readings obtained in the presence of an antigen. The error bars in graphs represent standard deviation. | |
Next, we examined a sample of paper attached to an artist board that was obtained from a Tiffany watercolor drawing, late 19th-early 20th century, (Fig. 2b). As the adhesive impregnated both the paper and the board, separation of the adhesive from the rest of the material was not possible. A solubility test by a conservator showed that the adhesive between the paper and the board was not soluble in room temperature water. FTIR performed on a sample extracted with Ambi/TFE indicated the presence of protein and the absence of synthetic adhesives (data not shown). The water insoluble nature of the adhesive with the historical use of casein as an adhesive on support boards led to the hypothesis that the adhesive might be casein glue (communication with a paper conservator). Therefore, the ELISA analysis was employed to identify the adhesive used between the paper and the supporting board. When the extracted paper/board sample was screened, it was positive for collagen type I and was negative for casein (Fig. 3b). The result suggests that the adhesive used is not casein glue although the possibility that the protein is too degraded and the concentration is below the detection limit with the ELISA analysis cannot be ruled out. The presence of collagen can be attributed to animal glue adhesive or to the sizing from the paper. Gelatin is used as a sizing reagent, where it provides the paper with resistance to the absorption of water. Our ELISA result demonstrates that the protein detected with FTIR is collagen-based.
The last sample measured was a microscopic detached paint fragment from a 14th-century double-sided processional banner by Spinello Aretino (b. 1345–52, d. 1410); depicting Saint Mary Magdalen Holding a Crucifix on one side and The Flagellation on the reverse (Fig. 2c). In cross-section, the sample appeared to be from the Magdalen's red robe and comprised a gypsum ground (calcium sulfate) beneath multiple red paint layers interspersed with varnish (data not shown). FTIR analysis of scrapings and ATR-FTIR analysis of cross-sections indicated that the lowest paint layer contained protein and oil but the complicated spectra obtained from the other paint layers did not allow differentiation of the binders of the upper layers (data not shown). GC-MS protein analysis of the lower red paint layer showed the presence of egg and possibly collagen, which could be a contamination from the ground layer or a glue applied during the artworks lifetime as a consolidant (data not shown). The paint layers with the ground removed and a separate sample of the ground was screened for the presence of collagen type I, casein, ovalbumin and general gums by ELISA to identify the binders in the ground and paint layers. The ELISA data indicates the presence of collagen type I (animal glue), ovalbumin, and gums (Fig. 3c) and the absence of casein. Collagen type I may be present as the binder for the gypsum ground or in the form of an animal-based consolidant used in a later restoration of the painting. Likewise, the presence of gum may be from a water-based medium, such as watercolor or gouache paint, used in restoration. Egg protein is likely present as the binder in the paint layers, as it is consistent with the painting practice of the period and region.30
The identification and detection of the binders/adhesives used in these artworks with ELISA was successful and unambiguous even though these three works are diverse in material, composition and age. However, as the ELISA method reported here is not quantitative, protein concentration as well as the ratio of different types of protein in a sample cannot be determined. Quantitative protein methods can determine the total amount of protein present but not always the ratios of complicated mixtures. In most cases the accurate identification of the components is of greater importance than the amount present.
Impact of pigments on ELISA analysis
The successful application of the ELISA method to identify proteins in real artworks shows that ELISA has a great potential to be used as an alternative or a complementary tool with the existing techniques available at cultural institutions for the identification and differentiation of binders and adhesives in artworks. However, for the ELISA to become a routine technique in the field of cultural heritage it is important to establish conditions and experimental parameters that impact the assay. To assess if protein detection with ELISA is influenced by the presence of pigments that contain biologically active cations, naturally aged three year old replica paints of whole egg, animal skin glue and borax casein, unpigmented and mixed with different pigments (lead white, ultramarine, chalk, French ochre) were used for these experiments (Fig. 4). Three of these paints contain reactive cations (Pb2+ in lead white, Ca2+ in chalk, and Fe3+ in French ochre) while ultramarine (a sulfur-containing sodium aluminum silicate compound) is relatively unreactive. It is important to mention that we have tested our ELISA methodology on real artworks that contained non-proteinaceous materials and we do not observe a significant background for the ELISA response. Another important consideration for these experiments was to perform protein quantitation with NanoOrange® after the extraction to determine the concentration of the protein present. The samples were then diluted to a protein concentration of 1 μg mL−1 for ELISA analysis to show trends in ELISA response that were influenced by protein/antibody recognition and not fluctuations in protein concentration.
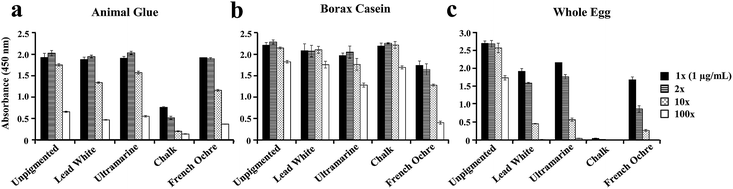 |
| Fig. 4 Impact of pigments on ELISA detection of proteins extracted from (a) animal glue, (b) borax casein, and (c) whole egg paints. The protein was quantified with NanoOrange® and diluted to a concentration of 1 μg mL−1 prior to ELISA analysis. The graphs represent one experiment conducted in triplicate. The threshold values determined for each binder were respectively subtracted from the absorbance values for each antigen. The error bars in all graphs represent standard deviation. | |
For naturally aged animal glue paints (Fig. 4a), only extracted protein from chalk paint showed suppressed ELISA response in comparison to protein detected from unpigmented, lead white, ultramarine and French ochre paints. For naturally aged borax casein paints (Fig. 4b), only casein extracted from French ochre paint showed a slight decrease in ELISA response compared to protein extracted from unpigmented, lead white, ultramarine, and chalk paints. Naturally aged whole egg paints showed the most pronounced pigment influence on the detection of protein with ELISA (Fig. 4c). Protein extracted from lead white, ultramarine, and French ochre paints showed a diminished ELISA response. Protein extracted from chalk paint showed a significant suppression of the ELISA response.
It is important to note that several pigments contain metal ions such as copper, iron, and cobalt, which are known to inhibit HRP activity.31 However, it is unlikely that the inhibition of HRP activity with metal ions can explain the observed dampening of the ELISA because the decrease in the ELISA signal is not observed for all of the extracted proteins from paints with the same pigment. For example, casein extracted from chalk paint shows no suppressed ELISA response, unlike collagen type I and ovalbumin extracted from chalk paint that both show a suppressed ELISA response. Also, only ovalbumin extracted from lead white and ultramarine paints shows a slight suppression in the ELISA response; while collagen type I and casein extracted from paints with these two pigments do not. These observations suggest that, within our experimental constraints, these metal containing pigments do not affect HRP activity. It is more likely that the diminished signal observed with certain pigments is due to antibody recognition of the antigen-binding domain of the target protein.
We also examined whether the aging of the paint can influence the observed ELISA suppression for specific pigments. For these experiments we prepared fresh paints from pure ovalbumin (purchased from Sigma-Aldrich) and whole egg because the naturally aged whole egg paints showed the most dramatic pigment effect compared to other protein binders (Fig. 4). The ovalbumin fresh paints (Fig. 5a) and whole egg fresh paints (Fig. 5b) were prepared two weeks prior to ELISA analysis. The naturally aged paints (Fig. 5c) were approximately 3 years old. For freshly prepared ovalbumin paints, only the protein extracted from chalk paint showed a slight suppression of the ELISA signal. For whole egg freshly prepared paints, the protein extracted from ultramarine, chalk, and French ochre paints showed a slight decrease in the ELISA signal compared to the unpigmented binder. However, no significant suppression of the ELISA response was observed for the freshly prepared ovalbumin and whole egg paints, in comparison to the naturally aged whole egg paints (Fig. 5c). As previously discussed, the naturally aged whole egg paints showed a decrease in the ELISA response for ultramarine and French ochre paints, and showed almost complete suppression of the ELISA response for the paints prepared with chalk. These results support the hypothesis that structural and or chemical modifications of the protein could occur in the paint film from interactions with chalk over time. Furthermore, these experiments show that aging of the paint is an important factor to consider for the detection of ovalbumin with ELISA, especially from paints prepared with specific pigments. While less likely, it is also possible that the presence of ions from pigments in solution or bound to the antigen sites could interfere with the antigen–antibody recognition in the ELISA analysis.
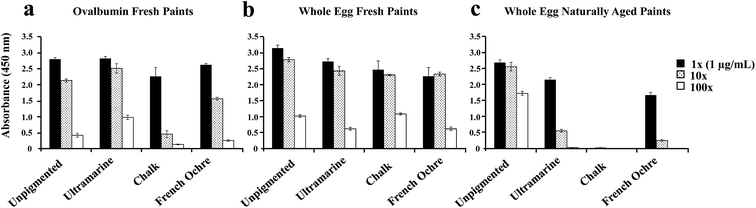 |
| Fig. 5 Impact of pigments and aging on ELISA detection of ovalbumin extracted from (a) ovalbumin fresh paints, (b) whole egg fresh paints, and (c) whole egg naturally aged paints. The protein was quantified with NanoOrange® and diluted to a concentration of 1 μg mL−1 prior to ELISA analysis. The threshold values determined for each binder were respectively subtracted from the absorbance values for each antigen. The graphs represent one experiment conducted in triplicate The error bars in all graphs represent standard deviation. | |
Effects of ion removal on samples prior to ELISA analysis
Studies have shown that pigments that contain reactive cations such as lead, copper, calcium, and iron can interfere with the derivatization process of amino acids in preparation for quantitative analysis by GC-MS and therefore can impair identification of proteins.6,7,22 Removal or sequestration of metal ions with a clean-up procedure that involves micro-column tips7 and EDTA (a metal ion chelator)32 before the derivatization procedure has been explored and reported to be effective.6 In addition, EDTA has been shown to be effective in antigen-unmasking by chelating calcium,23 but can compete with bound metal ions on proteins.31 The aim of these experiments is to determine if the removal of ions associated with pigments from the extracted protein solution could recover the ELISA response for problematic paint samples. To accomplish this, sample clean-up methods were used on paints made with pigments that resulted in significantly diminished protein detection with ELISA (Fig. 6).
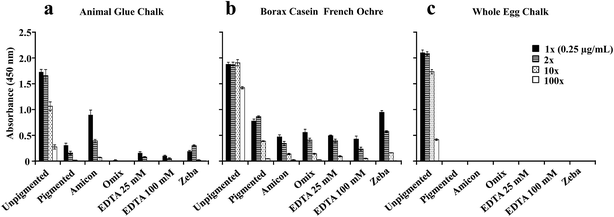 |
| Fig. 6 ELISA absorbance values measured for protein extracted from (a) animal glue mixed with chalk, (b) borax casein mixed with French ochre and (c) whole egg mixed with chalk. Five clean-up methods (Amicon®, OMIX®, 25 mM EDTA, 100 mM EDTA, and Zeba® columns) were used to remove potential ions from each protein solution. The protein was quantified with NanoOrange® and diluted to a concentration of 0.25 μg mL−1 prior to ELISA analysis. Graphs represent one experiment conducted in triplicate. The threshold values determined for each binder were respectively subtracted from the absorbance values obtained for each antigen. The error bars in all graphs represent standard deviation. | |
Protein quantitation with NanoOrange® was performed on samples before and after each cleaning procedure. It is important to note that each clean-up procedure was first performed on the three unpigmented samples to determine protein loss (data not shown). Only the clean-up with OMIX® C4 resulted in decreased protein concentration for the three unpigmented protein binders. Since whole egg binders contain multiple proteins, we tested protein loss with OMIX® C4 on pure ovalbumin (Sigma-Aldrich), which is our target for ELISA analysis, mixed with chalk, ultramarine, lead white, and French ochre, (data not shown). Protein loss was the same for all of the pigments tested and it is estimated to be less than one order of magnitude. The lowest protein concentration detected with NanoOrange® is approximately 0.1 μg mL−1, and ELISA is protein dependent and ranges approximately between 0.01–0.001 μg mL−1 (Fig. 1). For ovalbumin (Sigma-Aldrich), while no protein was detected with NanoOrange® after OMIX® C4 clean-up, we were still able to detect ovalbumin with the ELISA (data not shown). For these reasons, dilutions for ELISA analysis were based on protein concentrations determined before the clean-up procedure.
As shown in Fig. 6, most of the clean-up methods used on the three binders did not enhance the ELISA response. Only a slight increase in the ELISA response was observed for animal skin glue mixed with chalk after it was passed through the Amicon® filter (Fig. 6a). A small decrease in the ELISA response is also observed for the animal glue chalk passed through OMIX® C4 filter due to slight protein losses discussed above. For casein, removal of iron ions did not lead to a significant increase in the ELISA signal (Fig. 6b). For whole egg binder, the removal of calcium ions with different methods did not increase ELISA detection (Fig. 6c).
These results suggest that the overall minimization/removal of cations in the protein solution does not enhance the ELISA signal. It is possible that the concentration of EDTA used might be sufficient to chelate metal ions in solution as well as those bound to protein. Unless metal ions are tightly bound to the protein, they should chelate to EDTA. However, we do not observe significant ELISA enhancement with either of the clean-up methods. It is likely that it is the pigment interaction with the protein and not the metal ions in solution that cause ELISA suppression for specific paints. Since protein conformation and amino acid sequence are essential for specific antigen–antibody recognition,17,19 it is likely that chemical and physical modifications influenced by certain pigments, at a structural and amino acid level, affect the recognition of each antigen by their respective antibodies.
Conclusions
This current study establishes experimental parameters to apply ELISA as a consistent and reliable analytical tool to identify select proteins in adhesives and binders used in artworks. We have optimized conditions for the HRP-based ELISA system and applied the optimized methodology to selected artworks from a range of ages. As we continue to probe the reliability and reproducibility of the ELISA, our results suggest that certain pigments combined with aging could impact the ELISA detection and this could affect protein identification. Removal of pigment ions in solution does not recover the ELISA signal, which suggests that the ions from pigments do not interfere with the ELISA assay directly but interact with the antigens (proteins) over time to disrupt antibody–antigen recognition. While we continue to characterize this phenomenon it is important to remember that a positive identification may still be possible with our ELISA methodology even if such interference occurs. Though the ELISA method is not always quantitative for samples from artworks, this ELISA methodology can provide an accurate and unambiguous identification of proteinaceous binders which can be employed together with traditional techniques used in cultural heritage institutions, such as GC-MS and FTIR.
Acknowledgements
The authors gratefully acknowledge the following conservators at the Metropolitan Museum of Art for sharing their vast knowledge, and their support and involvement: Christine Giuntini, Arts of Africa, Oceania, and the Americas (Feathered Tabard); Marina Ruiz-Molina, Sherman Fairchild Center for Works on Paper and Photograph Conservation (Tiffany's Watercolor); Sarah Kleiner, Sherman Fairchild Foundation Fellow, Paintings Conservation, (Spinello). Also, James Boothe, volunteer, Department of Scientific Research, for the XRF and XRD analysis of the pigments. This material is based upon work supported by the National Science Foundation under CHE-1041839.
References
- R. J. Meilunas, J. G. Bentsen and A. Steinberg, Stud. Conserv., 1990, 35, 33–51 CrossRef CAS PubMed.
- M. P. Colombini and F. Modugno, J. Sep. Sci., 2004, 27, 147–160 CrossRef CAS PubMed.
-
J. Mills and R. White, The Organic Chemistry of Museum Objects, Butterworth-Heinemann, London, 2nd edn, 2000 Search PubMed.
-
M. Doerner, The Materials of the Artist and Their Use in Painting with Notes on the Techniques of the Old Masters, Hancourt Brace and Company, California, 3rd edn, 1984 Search PubMed.
- M. R. Schilling and H. P. Khanjian, J. Am. Inst. Conserv., 1996, 35, 123–144 CrossRef PubMed.
- J. De al Cruz-Cañizares, M. T. Doménech-carbó, J. V. Gimeno-adelantado, R. Mateo-Castro and F. Bosh-Reig, J. Chromatogr., 2004, 1025, 277–285 CrossRef PubMed.
- G. Gautier and M. P. Colombini, Talanta, 2007, 73, 95–102 CrossRef CAS PubMed.
- S. Dallongeville, M. Koperska, N. Garnier, G. Reille-Taillefert, C. Rolando and C. Tokarski, Anal. Chem., 2011, 83, 9431–9437 CrossRef CAS PubMed.
- C. Tokarski, E. Martin, C. Rolando and C. Cren-Olive, Anal. Chem., 2006, 78, 1494–1502 CrossRef CAS PubMed.
- D. P. Kirby, M. Buckley, E. Promise, S. A. Trauger and T. R. Holdcraft, Analyst, 2013, 138, 4849–4858 RSC.
- G. Leo, L. Cartechini, P. Pucci, A. Sgamellotti, G. Marino and L. Birolo, Anal. Bioanal. Chem., 2009, 395, 2269–2280 CrossRef CAS PubMed.
- W. Fremout, M. Dhaenens, S. Saverwyns, J. Sanyova, P. Vandenabeele, D. Deforce and L. Moens, Anal. Chim. Acta, 2010, 658, 156–162 CrossRef CAS PubMed.
-
G. Hodgins and R. Hedges, In the 6th International Conference on Non-Destructive Testing and Microanalysis for the Diagnostics and Conservation of the Cultural and Environmental Heritage, Rome, 1999, pp. 1795–1810 Search PubMed.
- A. Heginbotham, V. Millay and M. Quick, J. Am. Inst. Conserv., 2006, 45, 89–10 CrossRef PubMed.
- L. Cartechini, M. Vagnini, M. Palmieri, L. Pitzurra, T. Mello, J. Mazurek and G. Chiari, Acc. Chem. Res., 2010, 43, 867–876 CrossRef CAS PubMed.
- M. Palmieri, M. Vagnini, L. Pitzurra, B. G. Brunetti and L. Cartechini, Anal. Bioanal. Chem., 2013, 405, 6365–6371 CrossRef CAS PubMed.
- G. L. Bratthauer, Methods Mol. Biol., 2010, 588, 231–241 CAS.
- T. Porstmann and S. T. Kiessig, J. Immunol. Methods, 1992, 150, 5–21 CrossRef CAS.
- N. S. Lipman, L. R. Jackson, L. J. Trudel and F. Weis-Garcia, ILAR J., 2005, 46, 258–268 CrossRef CAS.
- A. Azevedo, V. Martins, D. Prazeres, V. Vojinović, J. Cabral and L. Fonseca, Biotechnol. Annu. Rev., 2003, 9, 199–247 CAS.
- Trends 2012 Report published by HTStec Limited, Cambridge, UK, June 2012.
- M. P. Colombini, A. Andreotti, I. Bonaduce, F. Modugno and E. Ribechini, Acc. Chem. Res., 2010, 43, 715–727 CrossRef CAS PubMed.
-
M. A. Hayat, Microscopy, Immunohistochemistry, and Antigen Retrieval Methods, Kluwer Academic/Plenum Publisher, New York, 2002 Search PubMed.
-
K. Wehlte, The Materials and Techniques of Painting, Van Nostrand Reinhold, New York, 1982 Search PubMed.
- J. P. Knox, P. J. Linstead, J. Peart, C. Cooper and K. Roberts, Plant J., 1991, 1, 317–326 CrossRef CAS PubMed.
-
D. P. Kirby, http://www.ndt.net/article/art2011/papers/KIRBY%20-%20M%201.pdf.
- C. J. Jones, R. P. Haugland and V. L. Singe, BioTechniques, 2003, 34, 850–854 Search PubMed.
- J. M. Deshusses, J. A. Burgess, A. Scherl, Y. Wenger, N. Walter, V. Converset, S. Paesano, G. L. Corthals, D. F. Hochstrasser and J. C. Sanchez, Proteomics, 2003, 3, 1418–1424 CrossRef CAS PubMed.
- H. Wang, W. J. Qian, H. M. Mottaz, T. R. Clauss, D. J. Anderson, R. J. Moore, D. G. Camp 2nd, A. H. Khan, D. M. Sforza, M. Pallavicini, D. J. Smith and R. D. Smith, J. Proteome Res., 2005, 4, 2397–2403 CrossRef CAS PubMed.
-
C. Cennini, The Craftsman's Handbook, translated by D. V. Thompson, Dover, New York, 1960 Search PubMed.
-
H. Zollner, Handbook of Enzyme Inhibitors, Wiley-VCH, Weimheim, 3rd edn, 1999 Search PubMed.
- J. K. Nyborg and O. B. Peersen, Biochem. J., 2004, 381, e3–e4 CrossRef CAS PubMed.
Footnote |
† Department of Pediatrics, Weill Cornell Medical College, New York, NY, USA. |
|
This journal is © The Royal Society of Chemistry 2015 |