DOI:
10.1039/C4RA13258K
(Paper)
RSC Adv., 2015,
5, 3941-3953
Stereoselective synthesis of trans-THF rings using an oxidative cyclisation-radical deoxygenation sequence: application to the formal synthesis of trans-(2R,5R)-linalool oxide†
Received
27th October 2014
, Accepted 5th December 2014
First published on 5th December 2014
Abstract
An efficient stereoselective synthesis of cis-2,5-disubtituted tetrahydrofuran (THF) diols has been achieved using permanganate-mediated oxidative cyclisation of 1,5-diene precursors. The facial selectivity during the course of cyclisation was induced by incorporating conveniently accessible chiral auxiliaries such as (2R)-10,2-camphorsultam, (S)-4-benzyloxazolidin-2-one and (−)-8-phenylmenthol. The use of (2R)-10,2-camphorsultam furnished the desired THF product as a single isolated diastereoisomer. Conformational analyses are presented to rationalize the origin of facial selectivity and synthetic investigation towards successfully accomplished transformation of cis-2,5-disubstituted THF diols into corresponding trans-THF compounds is also described, leading to a stereoselective formal synthesis of trans-(2R,5R)-linalool oxide.
Introduction
From cytotoxic natural products to industrial synthetic analogues, 2,5-disubstituted tetrahydrofurans (THFs) are common motifs present in bioactive molecules.1 WHI-261 (1)2 also named as COBRA-0, and COBRA-1 (2)3 are synthetic anti-cancer drug molecules which are effective against human tumour cell lines; trans-linalool oxide (3)4 is a monoterpenoid present in essential oils and one of the most commonly used compounds in beverages, foods, and perfumery, whilst 14-deacetyl eurylene (4) contains both cis- and trans-2,5-disubstituted THF units and exhibits a significant cytotoxicity against KB cells5 (IC50: 0.52 μg mL−1, Fig. 1). The most widely recognised biosynthetic route to 2,5-disubstituted THF units involves sophisticated enzyme-catalysed epoxidation-ring-opening cascades applied to acyclic polyene precursors,6 and their intrinsic ionophoric nature provides a metal binding tendency with physiologically important metallic cations.7 This ability of polyethers to bind metal ions is recognised as one mechanism of action leading to their notable bioactivity.
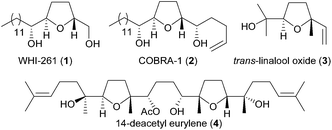 |
| Fig. 1 Structures of representative molecules containing trans-2,5-disubstituted THF rings. | |
Given their distinctive structural features combined with remarkable cytotoxicity, 2,5-disubstituted THFs have attracted an unusually high level of interest from the synthetic community, and much time and efforts to establish new synthetic protocols to obtain adequate quantities for expediting their biological evaluations. Among various established methodologies towards the synthesis of THFs, oxidative cyclisation involving metal-oxo-promoted processes is prevalent and these have been effectively applied to 5-hydroxyalkenes, 1,5-dienes and 5,6-dihydroxyalkenes (Fig. 2). Oxorhenium [Re(VII)]8 and Co(II) (ref. 9) oxidants have been reported to induce trans-selectivity in the incipient THF rings from 5-hydroxyalkenes (Fig. 2a); Mn(VII) (ref. 10), Os(VIII) (ref. 11) and Ru(VIII) (ref. 12) based oxidising agents promote the formation of cis-THF adducts when applied to 1,5-dienes (Fig. 2b), whereas Os(VI) (ref. 13), Cr(VI) (ref. 14) and Ru(VIII) (ref. 15) species provide a convenient access to cis-selective THF rings from 5,6-dihydroxyalkenes (Fig. 2c). Furthermore, Os(VI) chemistry has been extended to 5-hydroxy-6-protected aminoalkenes, 5,7- and 5,8-dihydroxyalkenes to synthesize cis-2,5-disubstituted THF motifs.16 Mechanistically, Co(II) catalysed cyclisation follows a radical pathway,9 whereas other metal-based transformations are believed to involve sequential [3 + 2] cycloadditions.10b However, a Sharpless-type [2 + 2] cycloadditions sequence was also proposed.10c Kinetic isotopic investigations17a and spectroscopic determination of the intermediate species17b reveal that operational mechanism follows [3 + 2] pathway, which was later supported by DFT calculations and labeling studies, confirming that concerted [3 + 2] cycloaddition is favoured by about 40 kcal mol−1 relative to the stepwise [2 + 2] counterpart.17c,d
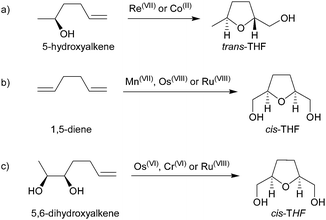 |
| Fig. 2 Synthesis of 2,5-disubstituted THF rings by metal-oxo-promoted cyclisations. | |
During research studies in the domain of oxidative cyclisation of 1,5-dienes and 1,5,9-trienes, it was observed that a direct stereoselective route for the trans-selective permanganate-mediated oxidative cyclisation of 1,5-diene motif is lacking, though the conversion of cis-THF diol into trans-THF product via oxa-carbenium ion intermediate is described.18 A conceptually different methodology involves the formation of a cis-THF diol by oxomanganese [Mn(VII)] promoted cyclisation of 1,5-diene substrate and subsequent conversion into desired trans-THF product via Barton–McCombie radical deoxygenation.19 Herein, a detailed synthetic investigation towards the stereoselective synthesis of cis-THF diols and their conversion into trans-THF motifs is reported.
Results and discussion
Commercially available prenyl bromide (5) was alkylated with the dianion generated from methallyl alcohol to yield allylic alcohol 6 in good yield (Scheme 1).20 Oxidation of allylic alcohol 6 was carried out using Mn-based oxidising agents such as MnO2 and BaMnO4 to furnish the aldehyde 7.21 However, after optimization of experimental procedures, MnO2 was found superior compared to BaMnO4, in terms of yield and shorter reaction time. Treatment of crude aldehyde 7 with NaCN–AcOH in MeOH offered an in situ generation of cyanohydrin, which was readily oxidised by MnO2 to acyl cyanide and subsequent nucleophilic attack of MeOH afforded the diene methyl ester 8.22 For a stereoselective oxidative cyclisation, a chiral auxiliary would be required to direct the metal oxidant to one face of the enoyl alkene. For this purpose, (2R)-10,2-camphorsultam ((−)-9) was selected and synthesized on multi-gram scale (36 g, 54% yield over 4 steps) according to the literature reports.23,24 Coupling of diene ester 8 with a pre-formed aluminium complex of chiral auxiliary (−)-9 provided 1,5-dienoate 10 in good yield,25 which was ready to test for the key oxidative cyclisation. Permanganate oxidative cyclisation of dienoate 10 gave the cis-THF diol 11 as a single diastereoisomer along with recovery of the starting dienoate 10 (up to 45%).19,26 Relative stereochemistry of the cis-THF diol 11 was assigned unequivocally by X-ray crystallography.19b
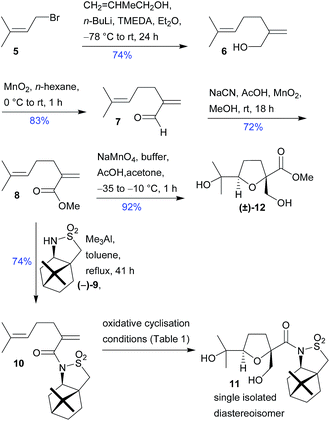 |
| Scheme 1 Permanganate-mediated oxidative cyclisation to prepare cis-THF diols 11 and 12. | |
Sodium permanganate as an oxidising agent in the absence of phase-transfer catalyst (PTC) offered comparatively lower yields of the resultant cis-THF diol 11 (Table 1, entries 1 and 2). However, the best yield was found when cyclisation was performed using potassium permanganate in the presence of PTC, Adogen 464 (Table 1, entry 3).10d Despite of all efforts to increase the conversion proved unsuccessful. Notably, a single diastereoisomeric cis-THF diol 11 was obtained in each reaction, which provides striking evidence for an effective facial selectivity induced by the chiral auxiliary (−)-9 during the course of sequential [3 + 2] permanganate-catalysed cycloadditions. Low reactivity of the dienoate 10 and appearance of single diastereomeric product can be attributed to the effective shielding by the attached chiral auxiliary. This proposal was tested by performing permanganate-mediated oxidative cyclisation for the methyl ester diene 8 under the same reaction conditions, which resulted an highly efficient formation of the racemic cis-THF diol 12 in 92% yield.19b
Table 1 Optimisation studies towards oxidative cyclisation of dienoate 10
Entry |
Oxidative cyclisation conditions |
10 yield (%) |
11 yield (%) |
Numbers in the brackets indicate % yield of cis-THF diol 11 on the basis of recovered starting material 10. |
1 |
NaMnO4 (1.5 eq., 0.4 M aq.), AcOH (3.0 eq.), buffer, acetone, −35 to −10 °C, 1 h. |
45 |
33 (60)a |
2 |
NaMnO4 (1.7 eq., 0.4 M aq.), AcOH (3.0 eq.), buffer, acetone, −40 to 0 °C, 1 h. |
30 |
32 (46)a |
3 |
KMnO4 (2.0 eq., 0.4 M aq.), AcOH (4.0 eq.), Adogen 464 (0.5 eq.), ether, rt, 30 min. |
41 |
38 (65)a |
As the stereoselectivity of the reaction is controlled by a chiral auxiliary (−)-9, the sense of diastereofacial preference observed in the oxidative cyclisation of 1,5-dienoate 10 can be explained by the preferred orientation of enoyl olefin and the relevant arrangement of C
O and NSO2 moieties. It is proposed that based on steric and electronic reasons, s-trans orientation of enoyl olefin27 and an anti-position of C
O and NSO2 groups are favoured,28 as demonstrated by two conformational models A and B (Fig. 3). If the metal atom (M) coordinates with the equatorial oxygen of the sulfonyl moiety and enoyl oxygen atom as shown in the case of conformer B, the Si-face of the electron-deficient alkene is effectively blocked by the camphor structure and the initial attack of permanganate ion (MnO4−) takes place from the Re-face followed by cyclisation. However, if metal-chelation is absent as reflected in the conformation A, the Si-face of enoyl olefin is also blocked; this time by the axial oxygen atom of the camphorsultam auxiliary (−)-9 and approach of MnO4− would take place from the Re-face. Both conformation models favour a Re-face attack of MnO4−, which is consistent with the obtained diastereoselective outcome and proceed via transition state 13 as a result of double [3 + 2] cycloadditions followed by hydrolysis to afford the cis-THF diol 11 as a single diastereoisomer. Low reaction yield and recovery of the unreacted starting material indicate that the Re-face in both conformations also experiences some sterics, which hinders initial attack of MnO4− on the electron-poor enoate alkene.
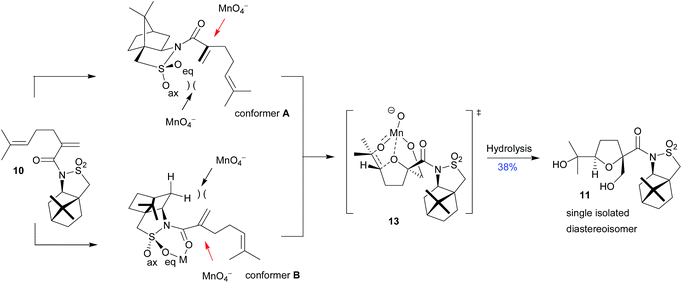 |
| Fig. 3 Rationalization of the diastereoinduction obtained by incorporating (2R)-10,2-camphorsultam ((−)-9) for the oxidative cyclisation of 1,5-dienoate 10. | |
At this juncture, an attempt was made to shorten the synthetic route towards dienoate 10 by subjecting allylic alcohol 6 to one-step MnO2-mediated oxidation approach.29 The desired diene methyl ester 8 was obtained, albeit in poor yield (15%) along with 70% recovery of the starting allylic alcohol 6. Also, efforts were carried out to shorten the reaction time (originally 41 hours) for the coupling reaction of methyl ester 8 with the chiral auxiliary (−)-9. In this context, a model study was performed using microwave irradiation for a commercially available ester 14, which was added to a pre-formed aluminium complex of (2R)-10,2-camphorsultam ((−)-9) in a microwave tube and the reaction mixture was irradiated (Scheme 2). The desired product 15 was isolated in poor yield along with 58% recovery of the auxiliary (−)-9. The starting material 14 could not be recovered, probably because of its high volatility. The same reaction was attempted with another available triene ester 16, which provided the desired trienoate 17 and unreacted chiral auxiliary (−)-9 in 60 and 23% yields respectively. Due to associated difficulties in improving the yield under microwave conditions, it was decided to investigate the effect of other readily available chiral auxiliaries, (S)-4-benzyloxazolidin-2-one ((−)-21)30 and 8-phenylmenthol ((−)-24)31 in the oxidative cyclisation.
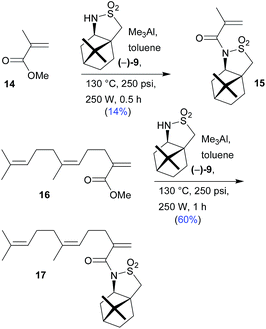 |
| Scheme 2 Microwave studies for the coupling of (2R)-10,2-camphorsultam ((−)-9) with methyl esters 14 and 16. | |
Methyl ester 8 was hydrolysed under basic conditions and the resultant carboxylic acid 18 was converted to its activated ester 19 by treating with pentaflourophenol and DCC (Scheme 3).32 As anticipated, oxidative cyclisation of PFP ester 19 provided cis-THF diol 20 in good yield, though lower in comparison with the oxidative cyclisation of methyl ester 8. Treatment of lithiated salt of oxazolidinone based chiral auxiliary (−)-21 with the ester 19 gave the diene 22, which on oxidative cyclisation afforded the cis-THF diol 23 as a major diastereoisomer (dr ∼ 4
:
1, 1H NMR) in moderate yield.33 Both diastereoisomers were not separable by flash column chromatography. With this moderate levels of yield and diastereoselectivity for the oxidative cyclisation of dienoate 22, application of another commercially available chiral auxiliary was investigated. Coupling of diene ester 19 with sodium salt of cyclohexyl based auxiliary (−)-24 furnished the desired 1,5-dienoate 25, which was subjected to permanganate-mediated oxidative cyclisation to provide cis-THF diol 26 as a major diastereoisomer in an excellent yield and acceptable level of diastereoinduction (dr ∼ 3.5
:
1, 1H NMR).34 Pleasingly, separation of the diastereoisomers was successfully achieved chromatographically by protecting inseparable mixture of diastereomeric oxidative cyclisation products as their bis-TMS ethers 27 and 28, and rest of the investigation was carried out with diastereomerically pure cis-THF adduct 27.
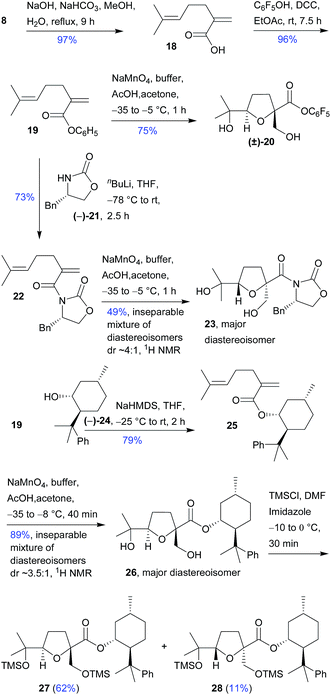 |
| Scheme 3 Oxidative cyclisation of 1,5-dienoates 19, 22 and 25; stereoselective synthesis of bis-protected cis-THF diol 27. | |
It is proposed that the observed stereochemical outcome during the oxidative cyclisation of 1,5-dienoate 22 may be accounted for by the preferential blockage of the rear face of the electron-poor enoate alkene by the phenyl ring present in the chiral auxiliary, as indicated in the s-trans enoate conformer C (Fig. 4). Due to this shielding, attack of MnO4− would take place preferentially from the front face, affording the cis-THF diol 23 as the major diastereoisomer via the transition state 29. The major product 26 from the oxidative cyclisation of dienoate 25 is formed through the transition state 30, which results by the blockage of the front face of the more electron deficient alkene by the phenyl ring as shown in the s-trans enoate conformer D.
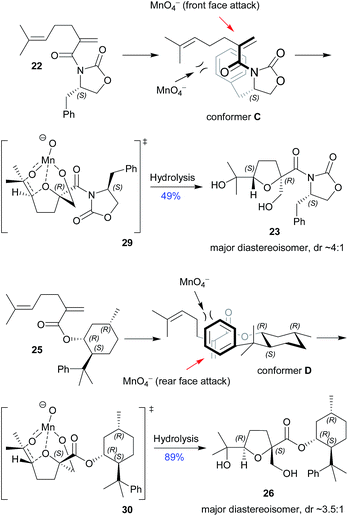 |
| Fig. 4 Origin of facial selectivity during the oxidative cyclisation of 1,5-dienoates 22 and 25 bearing chiral auxiliaries. | |
To provide substrates for the radical deoxygenation, conversion of primary carbinol present in the cis-THF diols 11 and 12 into corresponding thionoformate was envisaged. Treatment of cis-THF precursors 11 and 12 with chlorothionoformate in the presence of DMAP afforded the desired thionoformates 31 and 32 respectively (Scheme 4).35 In case of diastereomerically pure cis-THF diol 11, degradation of the starting material was noticed and the desired product was obtained in 33% yield. Efforts to improve the reaction yield by altering the reaction conditions such as temperature, time and stoichiometric amounts of the reagents made a very little impact. This low yield can be ascribed to the impediment caused by the steric bulk of the attached sultam auxiliary (−)-9 as it was observed during the oxidative cyclisation step. The degradation of the starting material might be triggered by the presence of free tertiary hydroxyl group, especially in a situation when primary hydroxyl group is experiencing steric hindrance. Alternatively, xanthate formation using CS2 and MeI in the presence of NaH and imidazole also proved unsuccessful to provide the desired product when applied to racemic cis-THF diol 12 and the starting material was recovered in 55% yield after flash chromatographic purification.36
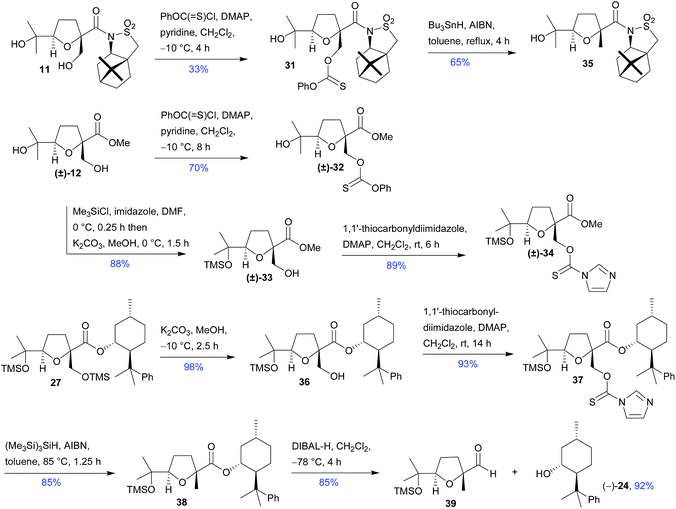 |
| Scheme 4 Synthetic investigations towards the transformation of cis-THF diols into trans-THF adducts via radical deoxygenation. | |
Moving to another approach to provide substrates for the radical deoxygenation, the tertiary hydroxyl group of the racemic cis-THF diol 12 was protected as TMS ether 33 and the product was treated with 1,1′-thiocarbonyl diimidazole in the presence of imidazole to afford the racemic thionocarbamate 34 in an excellent yield (Scheme 4).37 A survey of conditions for the radical deoxygenation revealed that (Bu4N)2S2O8 was a suitable reagent.38 Initial attempts towards the radical cleavage of the substrates 31, 32 and 34 failed to afford the desired trans-THF products on treatment with (Bu4N)2S2O8 and degradation of the starting material to afford intricate reaction mixture was noticed by TLC and crude 1H NMR. Additives such as Na2CO3 and Et3N have also been reported to facilitate the reaction, which also proved ineffective for this particular transformation. Gratifyingly, treatment of the thionoformate 31 with conventional hydride source, Bu3SnH afforded the required trans-THF product 35 in good yield,39 achieving a formal conversion of cis-THF diol 11 into trans-THF 35. Encouraged by successful accomplishment of radical deoxygenation, selective removal of primary TMS ether from bis-TMS protected THF adduct 27 provided mono-protected THF 36, which was subsequently converted to thionocarbamate 37 in an excellent yield. An alternate radical source, tris-trimethylsilyl silane (TTMSS) was also used for radical deoxygenation.40 Pleasingly, an end-game was achieved by treating thionocarbamate 37 with TTMSS/AIBN in hot toluene to afford the desired trans-THF compound 38 in good yield. Finally, reductive cleavage of the chiral auxiliary from the trans-THF compound 38 furnished the THF aldehyde 39 in excellent yield and pure 8-phenylmenthol ((−)-24, 92%), demonstrating the potential to recycle the chiral auxiliary. Stereoselective total synthesis of trans-(2R,5R)-linalool oxide (3), a commercially useful monoterpenoid can easily be achieved by deprotection of the tertiary silyl ether and subsequent methylenation of the aldehyde 39, which highlights the effectiveness of this protocol.
Conclusions
In summary, an efficient route to prepare trans-2,5-disubstituted THF rings, a common motif present in numerous bioactive natural products, from cis-2,5-disubstituted diols is described. This route employs permanganate-mediated oxidative cyclisation applied to 1,5-dienoate followed by radical deoxygenation of the resultant primary carbinol. Facial selectivity during the course of oxidative cyclisation was controlled by incorporating readily available external chiral auxiliaries and obtained diastereoinduction is explained by the conformational models. Reductive cleavage of the chiral auxiliary was efficiently carried out using facile conditions to promote functional group transformation for further synthetic manipulations and to demonstrate the potential of recycling chiral auxiliary. Work is ongoing in the area of stereoselective oxidative cyclisation followed by specific transformations and the process can provide a useful route to the synthesis of trans-THF rings containing natural products and synthetic analogues of pivotal importance.
Experimental
Experimental, characterization data and NMR spectra for the compounds 6–8, 10–12, 18 and 19 are reported.19b
General
All air/moisture sensitive reactions were carried out under an inert atmosphere, in an oven dried glassware. The solvents Et2O (from Na–benzophenone), toluene and CH2Cl2 (from CaH2) were distilled before use, and where appropriate, other reagents and solvents were purified by standard techniques.41 TLC was performed on aluminium-pre-coated plates with silica gel 60 with an F254 indicator; visualised under UV light (254 nm) and/or by staining with KMnO4 (10% aq.). Flash column chromatography was performed with Merck Kieselgel 60 silica gel. Fourier-transform infrared (FT-IR) spectra are reported in wavenumbers (cm−1) and were collected on a Nicolet 380 fitted with a Diamond platform, as solids or neat liquids. 1H NMR and 13C NMR spectra were recorded in CDCl3 solution using a Bruker AC300 or and AV300 (300 and 75 MHz respectively) or on a Bruker DPX400 (400 and 100 MHz respectively). Chemical shifts are reported in δ units using CHCl3 as an internal standard (δ 7.27 ppm 1H and δ 77.00 ppm 13C). Coupling constants (J) were recorded in Hz. The following abbreviations for the multiplicity of the peaks are s (singlet), d (doublet), t (triplet), q (quartet), quin (quintet), sxt (sextet), br (broad), and m (multiplet). Optical rotations were measured using PolAAr 2001 polarimeter with 589 nm light source. Melting points were obtained in an open capillary and are uncorrected. Electron impact and chemical ionisation mass spectra were obtained using a Fisons VG platform single quadrapole mass spectrometer. Electrospray mass spectra were obtained using a Micromass platform mass analyser with an electrospray ion source. The buffer solution used in the aq. permanganate-promoted oxidative cyclisation was an aq. 8
:
2 mixture of 1/15 M KH2PO4 and 1/15 M NaH2PO4 at pH 6.5.
General procedure for the microwave conditions of coupling of (2R)-10,2-camphorsultam ((−)-9) with methyl esters
To the solution of (2R)-10,2-camphorsultam ((−)-9, 1.25 mmol) in distilled toluene (0.25 M, on the basis of mmol of methyl ester) was added dropwise (Me)3Al (1.25 mmol) at room temperature. After stirring for 15 min, a solution of methyl ester (1.0 mmol) in distilled toluene (0.5 mL) was added and the resulting mixture was placed in microwave at 130 °C, 250 psi and 250 W for 30–60 min. At this stage, the mixture was taken out from microwave and diluted with CH2Cl2 (5 mL), water (2 mL) and Rochelle salt (2 mL) and stirred for 1 hour at room temperature. The organic phase was separated, dried (Na2SO4), filtered and concentrated in vacuo to afford the crude product.
N-(3-Methyl-2-methylene-propylate)-(2R)-camphor-10,2-sultam (15)
Following the general procedure for the coupling (2R)-10,2-camphorsultam ((−)-9) with methyl esters under microwave conditions, methyl ester 14 (100 mg, 1.0 mmol) provided the crude (267 mg) after 30 min as a yellowish white solid. Purification by column chromatography (SiO2 eluting with 20% EtOAc–hexane) afforded the title product 15 as a yellow oil (45 mg, 0.16 mmol, 14%). The pure chiral auxiliary (−)-9 was also recovered as a white solid (156 mg, 0.72 mmol, 58%). [α]D = −37.0 (c 0.45, CHCl3, 26 °C); FT-IR νmax (neat) 2961, 2919, 2882, 1679, 1334, 1194, 1132, 1063, 976 cm−1; 1H NMR (300 MHz, CDCl3) δ 5.70 (1H, br, C
CHH), 5.66 (1H, d, J = 1.5 Hz, C
CHH), 4.05 (1H, dd, J = 7.5, 5.0 Hz, CHN), 3.52 (1H, d, J = 13.8 Hz, CHHSO2), 3.40 (1H, d, J = 13.5 Hz, CHHSO2), 2.06–1.90 (5H, m, CHH and 2 × CH2), 2.01 (3H, s, CH3), 1.44–1.38 (2H, m, CH2), 1.24 (3H, s, CH3), 1.01 (3H, s, CH3) ppm; 13C NMR (75 MHz, CDCl3) δ 171.25 (CON), 138.98 (C
CH2), 124.31 (C
CH2), 65.44 (CHN), 53.56 (CH2SO2), 48.02 (CCH2), 47.71 (C(CH3)2), 45.26 (CHC(CH3)2), 38.35 (CH2CHN), 33.28 (CH2CH), 26.47 (CH2C), 21.32 (CH3), 19.89 (CH3), 18.71 (CH3) ppm; LRMS (ES+) m/z 306 (100%, [M + Na]+); HRMS (ES+) m/z calculated: 306.1134; found: 306.1132 ([M + H]+).
N-((Z)-Methyl-6,10-dimethyl-2-methyleneundeca-5,9-dienoyl)-(2R)-camphor-10,2-sultam (17)
Following the general procedure for the coupling (2R)-10,2-camphorsultam ((−)-9) with methyl ester under microwave conditions, methyl ester 16 (100 mg, 0.42 mmol) afforded a yellow oil after 60 min as a crude product (177 mg). Purification by column chromatography (SiO2 eluting with 20% EtOAc–hexane) afforded the title trieneoate 17 as a yellow oil (106 mg, 0.25 mmol, 60%). Physical and spectroscopic data were in accordance with the literature.19a The pure chiral auxiliary (−)-9 was also recovered as a white solid (26 mg, 0.12 mmol, 23%). [α]D = −41.8 (c 0.33, CHCl3, 25 °C); FT-IR νmax (neat) 2960, 2914, 1680, 1336, 1196, 1132, 1063, 976 cm−1; 1H NMR (400 MHz, CDCl3) δ 5.76 (1H, s,
CHH), 5.63 (1H, s,
CHH), 5.15 (2H, t, J = 7.0 Hz, 2 × C
CHCH2), 4.05 (1H, dd, J = 7.3, 4.8 Hz, CHN), 3.51 (1H, d, J = 13.6 Hz, CHHSO2), 3.40 (1H, d, J = 13.6 Hz, CHHSO2), 2.39–2.19 (4H, m, 2 × CH2), 2.06 (4H, s, 2 × CH2), 2.05–1.90 (5H, m, CH and 2 × CH2), 1.69 (6H, s, 2 × CH3), 1.61 (3H, s, CH3), 1.44–1.38 (2H, m, CH2), 1.24 (3H, s, CH3), 1.00 (3H, s, CH3) ppm; 13C NMR (100 MHz, CDCl3) δ 171.10 (CON), 143.15 (C
CH2), 135.94 (CH3C), 131.50 ((CH3)2C), 124.34 (C
CH2), 124.12 (C
CHCH2), 123.49 (C
CHCH2), 65.53 (CHN), 53.60 (CCH2), 47.92 (CH2SO2), 47.70 (C(CH3)2), 45.20 (CHC(CH3)2), 38.38 (CH2CHN), 33.21 (CH2C), 32.76 (CH2C
CH2), 31.94 (CH2C
CH), 26.55 (CH2CH2C), 26.48 (CH2CH2), 26.04 (CH2CH2), 25.69 (CH3), 23.33 (CH3), 21.25 (CH3), 19.87 (CH3), 17.63 (CH3) ppm; LRMS 442 (100%, [M + Na]+), 420 (20%, [M + H]+); HRMS (ES+) m/z calculated: 442.2386; found: 442.2380 ([M + Na]+).
General procedure for the permanganate-mediated oxidative cyclization of 1,5-dienoates
To a vigorously stirred mixture of 1,5-dienoate (1 mmol) and phosphate buffer (0.5 M, on the basis of mmol of 1,5-dienoate) in acetone (0.05 M, on the basis of mmol of 1,5-dienoate) at −35 °C was added a solution of NaMnO4 (1.5 mmol) containing AcOH (3 mmol). The purple mixture was stirred rapidly for 40–60 min, during which time the temperature of the acetone cooling bath had raised to −8 to −5 °C and the reaction mixture had turned dark brown. At this, the reaction was quenched with sat. aq. Na2S2O5 (30 mL) to dissolve all of the precipitated manganese salt and then repeatedly extracted using CH2Cl2 (4 × 60 mL). The organic extracts were combined, dried (Na2SO4), filtered and concentrated in vacuo to give the crude product.
Rac. (2S,5R)-Perfluorophenyl tetrahydro-2-(hydroxymethyl)-5-(2-hydroxypropan-2-yl)furan-2-carboxylate (20)
Following the general procedure for the permanganate-mediated oxidative cyclization of 1,5-dienoate, diene ester 19 (735 mg, 2.30 mmol) afforded the crude as a viscous oil (765 mg). Purification by column chromatography (SiO2 eluting with EtOAc–hexane (5–40%)) afforded racemic THF diols 20 as a white solid (638 mg, 1.72 mmol, 75%). mp = 73–75 °C; FT-IR νmax (neat) 3337, 2977, 2933, 2877, 1781, 1519, 1471, 1102, 1055, 996 cm−1; 1H NMR (400 MHz, CDCl3) δ 4.14 (1H, t, J = 7.5 Hz, CH, THF), 4.08 (1H, d, J = 11.3 Hz, CHHOH), 3.90 (1H, d, J = 11.3 Hz, CHHOH), 2.82 (1H, br, OH), 2.35 (2H, t, J = 7.5 Hz, CH2, THF), 2.10–1.96 (2H, m, CH2, THF), 1.35 (3H, s, CH3), 1.26 (1H, s, OH), 1.19 (3H, s, CH3) ppm; 13C NMR (100 MHz, CDCl3) δ 170.01 (COO), 87.97 (CH, THF), 86.26 (CCH2OH, THF), 71.60 ((CH3)2C), 65.97 (CH2OH), 32.40 (CH2, THF), 27.42 (CH2, THF), 25.94 (CH3), 25.28 (CH3) ppm (aromatic carbons were not observed); LRMS 393 (100%, [M + Na]+); HRMS (ES+) m/z calculated: 393.0737; found: 393.0736 ([M + Na]+).
(S)-3-(6-Methyl-2-methylenehept-5-enoyl)-4-benzyloxazolidin-2-one (22)
To a solution of (S)-benzyloxazolidinone ((−)-21, 75.5 mg, 0.43 mmol) in dry THF (5 mL) was added n-BuLi (0.2 mL of 2.5 M in hexane, 0.49 mmol) at −78 °C. The solution was allowed to warm to −60 °C over 1 hour whereupon a solution of the diene ester 19 (130 mg, 0.41 mmol) in dry THF (5 mL) was added dropwise. The solution was then allowed to warm to room temperature. After 1 hour stirring at room temperature, the reaction was diluted in Et2O (20 mL) and quenched with NH4Cl (sat. aq. sol., 25 mL). The organic phase was washed with NaHCO3 (sat. aq. sol., 2 × 20 mL), dried (Na2SO4) and concentrated in vacuo to give yellow oil (147 mg). Purification by column chromatography (SiO2 eluting with EtOAc–hexane (5–20%)) afforded the title product 22 as a yellow oil (93 mg, 0.23 mmol, 73%). [α]D = 56.8 (c 1.25, CHCl3, 25 °C); FT-IR νmax (neat) 2954, 2920, 2862, 1785, 1694, 1517, 1176, 1129, 763, 699 cm−1; 1H NMR (400 MHz, CDCl3) δ 7.36–7.22 (5H, m, 5 × CH, Ar), 5.45 (1H, s, C
CHH), 5.42 (1H, s, C
CHH), 5.15 (1H, tt, J = 7.0, 1.5 Hz,
CHCH2), 4.79–4.72 (1H, m, CHN), 4.29 (1H, t, J = 8.5 Hz, CH2O), 4.21 (1H, dd, J = 8.5, 4.5 Hz, CH2O), 3.42 (1H, dd, J = 13.6, 3.5, Hz, CCHPh), 2.85 (1H, dd, J = 13.6, 9.5 Hz, CCHPh), 2.46 (2H, t, J = 7.5 Hz, CH2), 2.45 (2H, q, J = 7.5 Hz, CH2), 1.73 (3H, s, CH3), 1.66 (3H, s, CH3) ppm; 13C NMR (100 MHz, CDCl3) δ 171.03 (CONCO), 152.85 (COOCH2), 143.93 (CCH2), 135.06 (C, Ar), 132.61 (CCHCH2), 129.39 (2 × CH, Ar(meta)), 128.96 (2 × CH, Ar(ortho)), 127.40 (CH, Ar(para)), 123.08 (CHCH2), 119.45 (CH2), 66.43 (OCH2), 55.30 (NCHCH2), 37.70 (CH2C), 33.05 (CH2CCH2), 26.53 (CH2CH2), 25.65 (CH3), 17.72 (CH3) ppm; LRMS (ES+) m/z 336 (100%, [M + Na]+); HRMS (ES+) m/z calculated: 336.1570; found: 336.1565 ([M + Na]+).
(2R,5S)-((S)-4-Benzyloxazolidin-2-one)tetrahydro-2-(hydroxymethyl)-5-(2-hydroxypropan-2-yl)furan-2-carboxylate (23, major diastereoisomer); (2S,5R)-((S)-4-benzyloxazolidin-2-one)tetrahydro-2-(hydroxymethyl)-5-(2-hydroxypropan-2-yl)furan-2-carboxylate (minor diastereoisomer)
Following the general procedure for the permanganate-mediated oxidative cyclization of 1,5-dienoate, diene ester 22 (81 mg, 0.26 mmol) afforded the crude as a yellow oil (80 mg, dr ∼ 4
:
1, 1H NMR). Purification by column chromatography (SiO2 eluting with EtOAc–hexane (5–40%)) afforded an inseparable diastereomeric mixture of THF diols 23 along with the minor diastereoisomer as a colorless oil (46 mg, 0.126 mmol, 49%). [α]D = 11.6 (c 3.1, CHCl3, 25 °C); FT-IR νmax (neat) 3436, 2972, 2923, 2871, 1779, 1694, 1457, 1089, 1051, 765, 732, 701 cm−1; 1H NMR (400 MHz, CDCl3) δ 7.30–7.12 (5H, m, 5 × CH, Ar), 4.70–4.64 (1H, m, CHN), 4.24 (1H, d, J = 11.6 Hz, CHHOCO), 4.16 (1H, d, J = 11.6 Hz, CHHOCO), 4.13 (1H, dd, J = 9.0, 3.0 Hz, CH, THF), 3.96maj and 3.91min (1H, dd J = 9.0, 7.0 Hz, CHHOH), 3.86maj and 3.76min (1H, d, J = 11.6 Hz, CH2O), 3.23 (1H, dd, J = 13.5, 3.0 Hz, CHHC), 2.74 (1H, dd, J = 13.5, 9.0 Hz, CHHC), 2.33–2.21 (2H, m, CHH, THF), 1.90–1.78 (3H, m, OH and CHH, THF), 1.28maj and 1.25min (3H, s, CH3), 1.11maj and 1.10min (3H, s, CH3) ppm (an OH was not observed); 13C NMR (100 MHz, CDCl3) δ 173.47 (CON), 152.30 (COO), 135.13 (C, Ar), 129.43 (2 × CH, Ar(meta)), 128.94 (2 × CH, Ar(ortho)), 127.35 (CH, Ar(para)), 90.23min and 89.91maj (CCH2OH, THF), 87.24maj and 87.05min (CH, THF), 71.20 (CCH), 66.76min and 66.55maj (OCH2), 56.98min and 56.70maj (CH2OH), 37.75 (CHCH2), 31.93min and 31.62maj (CH2), 27.51maj and 27.36min (CH2, THF), 26.40maj and 26.21min (CH2, THF), 24.82maj and 24.54min (2 × CH3) ppm; LRMS (ES+) m/z 386 (100%, [M + Na]+); HRMS (ES+) m/z calculated: 386.1574; found: 386.1578 ([M + Na]+).
(1R,2S,5R)-5-Methyl-2-(2-phenylpropan-2-yl)cyclohexyl-6-methyl-2-methylenehept-5-enoate (25)
To a solution of 8-phenylmenthol ((−)-24, 303 mg, 1.30 mmol) in dry THF (40 mL) was added NaHMDS (1.03 mL, 1.0 M in THF, 1.03 mmol) at −25 °C. The solution was allowed to warm to −25 °C over 1.5 hours whereupon a solution of the diene ester 19 (418 mg, 1.30 mmol) in dry THF (2 mL) was added dropwise. The solution was then allowed to warm to room temperature. After stirring at room temperature for 30 min, the reaction was diluted in Et2O (25 mL) and quenched with sat. aq. NH4Cl (15 mL). The organic phase was separated, washed with sat. aq. NaHCO3 (2 × 20 mL), dried (Na2SO4) and concentrated in vacuo to give a yellow oil as the crude product as a yellow oil (425 mg). Purification by column chromatography (SiO2 eluting with 5% EtOAc–hexane) afforded the title diene 25, bearing chiral auxiliary 8-phenylmenthol ((−)-24), as a yellow oil (380 mg, 1.03 mmol, 79%). [α]D = −31.1 (c 1.13, CHCl3, 30 °C); FT-IR νmax (neat) 2954, 2920, 2862, 1706, 1176, 1129, 763 cm−1; 1H NMR (400 MHz, CDCl3) δ 7.28–7.10 (5H, m, 5 × CH, Ar), 5.69 (1H, d, J = 1.5 Hz, C
CHH), 5.30 (1H, d, J = 1.5 Hz, C
CHH), 5.11–5.06 (1H, m,
CHCH2), 4.92 (1H, td, J = 10.8, 4.3 Hz, OCH), 2.11–2.05 (6H, m, 3 × CH2), 1.70 (3H, s, CH3), 1.70–1.32 (2H, m, CH2), 1.62 (3H, s, CH3), 1.33 (3H, s, CH3), 1.27 (3H, s, CH3), 1.17–0.91 (4H, m, 2 × CH2), 0.90 (3H, d, J = 6.5 Hz, CH3CH) ppm; 13C NMR (100 MHz, CDCl3) δ 166.26 (COO), 151.43 (CCH, Ar), 140.57 (C
CH2), 131.95 ((CH3)2C), 127.97 (2 × CH, Ar(meta)), 125.43 (2 × CH, Ar(ortho)), 125.03 (CH, Ar(para)), 124.38 (
CH2), 123.60 (C
CHCH2), 74.61 (OCH), 50.55 (CHCPh), 41.78 (CPh), 39.83 (CH2CH(CH3)), 34.62 (CH2C
CH2), 31.65 (CHCH3), 31.33 (CH2CH2CH), 26.93 (CH2CH2), 26.85 (2 × CH3), 26.44 (CH2CH), 25.65 (CH3), 21.78 (CH3), 17.71 (CH3) ppm; LRMS (ES+) m/z 391 (100%, [M + Na]+); HRMS (ES+) m/z calculated: 391.2608; found: 391.2605 ([M + Na]+).
(2S,5R)-((1R,2S,5R)-5-Methyl-2-(2-phenylpropan-2-yl)cyclohexyl) tetrahydro-2-(hydroxymethyl)-5-(2-hydroxypropan-2-yl)furan-2-carboxylate (26, major diastereoisomer); (2R,5S)-((1R,2S,5R)-5-methyl-2-(2-phenylpropan-2-yl)cyclohexyl) tetrahydro-2-(hydroxymethyl)-5-(2-hydroxypropan-2-yl)furan-2-carboxylate (minor diastereoisomer)
Following the general procedure for the permanganate-mediated oxidative cyclization of 1,5-dienoate, diene ester 25 (375 mg, 1.02 mmol) afforded the crude as a yellow oil (411 mg, dr ∼ 3.5
:
1, 1H NMR). Purification by column chromatography (SiO2 eluting with EtOAc–hexane (5–40%)) afforded an inseparable diastereomeric mixture of THF diols 26 along with the minor diastereoisomer as a yellow oil (378 mg, 0.90 mmol, 89%). [α]D = −15.4 (c 0.915, CHCl3, 28 °C); FT-IR νmax (neat) 3390, 2957, 2923, 2871, 1721, 1457, 1089, 1051, 765, 732, 701 cm−1; 1H NMR (400 MHz, CDCl3) δ 7.31–7.16 (5H, m, 5 × CH, Ar), 4.92min and 4.80maj (1H, td, J = 10.7, 4.4 Hz, OCH), 4.05maj and 3.93min (1H, t, J = 7.1 Hz, CH, THF), 3.74–3.47 (2H, m, CH2OH), 2.73 (1H, br, OH), 2.34 (1H, br, OH), 2.05–1.88 (5H, m, CH and 2 × CH2, THF), 1.49–1.26 (5H, m, CH and 2 × CH2), 1.37 (3H, s, CH3), 1.29 (3H, s, CH3), 1.27 (3H, s, CH3), 1.25 (3H, s, CH3), 1.15–90.75 (2H, m, CH2), 0.86 (3H, d, J = 6.5 Hz, CH3CH) ppm; 13C NMR (100 MHz, CDCl3) δ 173.59maj and 173.09min (COO), 150.76maj and 150.51min (CCH, Ar), 128.03 (2 × CH, Ar(meta)), 125.66maj and 125.58min (2 × CH, Ar(ortho)), 125.34 (CH, Ar(para)), 87.67maj and 87.35min (CH, THF), 86.46maj and 85.06min (CCH2OH, THF), 76.18 (OCH), 71.41maj and 71.30min (COH), 65.96maj and 65.59min (CH2OH), 50.33maj and 49.96min (CHCPh), 41.59maj and 41.42min (CPh), 40.12maj and 38.17min (CH2CH(CH3)), 34.50maj and 34.43min (CH2CH2CH), 32.11maj and 31.30min (CH2, THF), 28.75 (CHCH3), 27.54maj and 27.42min (CH2, THF), 27.50 (CH3), 27.16 (CH3), 26.09 (CH2C), 25.66maj and 25.47min (CH3), 25.17 (CH3), 21.72maj and 14.19min (CH3) ppm; LRMS (ES+) m/z 441 (40%, [M + Na]+), 859 (100%, [2M + Na]+); HRMS (ES+) m/z calculated: 441.2611; found: 441.2607 ([M + Na]+).
(2S,5R)-((1R,2S,5R)-5-Methyl-2-(2-phenylpropan-2-yl)cyclohexyl)tetrahydro-2-(methoxytrimethylsilyl)-5-(2-trimethylsiloxy-propan-2-yl)furan-2-carboxylate (27); (2R,5S)-((1R,2S,5R)-5-methyl-2-(2-phenylpropan-2-yl)cyclohexyl) tetrahydro-2-(methoxytrimethylsilyl)-5-(2-trimethylsiloxy-propan-2-yl)furan-2-carboxylate (28)
To a solution of inseparable diastereomeric mixture of cis-THF diol 26 and it's minor diastereoisomer (378 mg, 0.90 mmol) in DMF (10 mL) was added imidazole (735 mg, 10.8 mmol), followed by the addition of Me3SiCl (1.14 mL, 9.0 mmol) at −5 °C. The reaction mixture was stirred for 1 hour, during which the temperature raised to 0 °C. The reaction was quenched with sat. aq. NH4Cl (3 mL) and H2O (5 mL). The aqueous phase was extracted with Et2O (2 × 20 mL) and combined organic phases were dried (Na2SO4), filtered and concentrated in vacuo to give the crude as a yellow oil (412 mg). Purification by column chromatography (SiO2 eluting with CH2Cl2–hexane (10–30%)) afforded the title bis-protected THF 27 (single major diastereoisomer by 1H NMR) as a yellow oil (315 mg, 0.56 mmol, 62%). The more polar minor diastereoisomer 28 was also isolated as a yellow oil (54 mg, 0.10 mmol, 11%). 27, major diastereoisomer: [α]D = −16.1 (c 1.01, CHCl3, 30 °C); FT-IR νmax (neat) 2954, 2915, 2865, 1726, 1249, 1094, 1039, 872, 836, 751, 700 cm−1; 1H NMR (400 MHz, CDCl3) δ 7.32–7.16 (5H, m, 5 × CH, Ar), 4.85 (1H, td, J = 10.6, 4.3 Hz, OCH), 4.00 (1H, m, CH, THF), 3.83 (1H, d, J = 10.1 Hz, CHHOSi(CH3)3), 3.57 (1H, d, J = 10.1 Hz, CHHOSi(CH3)3), 2.05–1.79 (5H, m, CH and 2 × CH2, THF), 1.70–1.24 (5H, m, CH and 2 × CH2), 1.41 (3H, s, CH3), 1.28 (3H, s, CH3), 1.23 (6H, s, 2 × CH3), 1.15–0.79 (2H, m, CH2), 0.85 (3H, s, d, J = 6.5 Hz, CH3CH), 2.59 (9H, s, COSi(CH3)3), −0.48 (9H, s, CH2OSi(CH3)3) ppm; 13C NMR (100 MHz, CDCl3) δ 173.50 (COO), 150.85 (CCH, Ar), 127.96 (2 × CH, Ar(meta)), 125.73 (2 × CH, Ar(ortho)), 125.22 (CH, Ar(para)), 87.80 (CCH2O, THF), 87.58 (CH, THF), 75.87 (OCH), 75.00 (COSi(CH3)3), 66.73 (CH2OSi(CH3)3), 50.41 (CHCPh), 41.61 (CPh), 40.31 (CH2CH(CH3)), 34.59 (CH2CH2CH), 31.50 (CH2, THF), 31.29 (CHCH3), 29.77 (CH3), 27.94 (CH3), 27.34 (CH2, THF), 25.52 (CH2C), 25.14 (CH3), 24.27 (CH3), 21.77 (CH3), 2.59 (3C, COSi(CH3)3), −0.48 (3C, CH2OSi(CH3)3) ppm; LRMS (ES+) m/z 585 (65%, [M + Na]+), 1148 (100%, [2M + Na]+); HRMS (ES+) m/z calculated: 585.3407; found: 585.3405 ([M + Na]+). 28, minor diastereoisomer: [α]D = −15.5 (c 1.405, CHCl3, 27 °C); 1H NMR (400 MHz, CDCl3) δ 7.32–7.16 (5H, m, 5 × CH, Ar), 4.86 (1H, td, J = 10.6, 4.3 Hz, OCH), 3.89 (1H, m, CH, THF), 3.75 (1H, d, J = 10.1 Hz, CHHOSi(CH3)3), 3.69 (1H, d, J = 10.1 Hz, CHHOSi(CH3)3), 2.17–1.79 (5H, m, CH and 2 × CH2, THF), 1.52–1.1.21 (3H, m, CH and CH2), 1.44 (3H, s, CH3), 1.31 (3H, s, CH3), 1.23 (3H, s, CH3), 1.21 (3H, s, CH3), 1.03–0.79 (4H, m, CH2), 0.84 (3H, d, J = 6.3 Hz, CH3CH), 0.12 (9H, s, COSi(CH3)3), 0.10 (9H, s, CH2OSi(CH3)3) ppm; 13C NMR (100 MHz, CDCl3) δ 172.80 (COO), 150.41 (CCH, Ar), 127.94 (2 × CH, Ar(meta)), 125.92 (2 × CH, Ar(ortho)), 125.29 (CH, Ar(para)), 87.74 (CCH2O, THF), 87.54 (CH, THF), 75.97 (OCH), 74.92 (COSi(CH3)3), 66.38 (CH2OSi(CH3)3), 50.60 (CHCPh), 41.63 (CPh), 40.51 (CH2CH(CH3)), 34.55 (CH2CH2CH), 31.34 (CHCH3), 31.29 (CH2, THF), 30.73 (CH3), 27.82 (CH3), 27.49 (CH3), 25.59 (CH2, THF), 25.42 (CH2C), 23.34 (CH3), 21.75 (CH3), 2.57 (3C, COSi(CH3)3), −0.54 (3C, CH2OSi(CH3)3) ppm.
General procedure for the preparation of thionoformate from cis-THF diols
To a solution of cis-THF diol (1 mmol), pyridine (10 mmol) and catalytic DMAP (0.2 mmol) in CH2Cl2 (0.02 M) was added phenyl chlorothionoformate (10 mmol) at 0 °C. The bright yellow mixture was stirred for 4 hours at room temperature, diluted with CH2Cl2 (50 mL) and washed with HCl (2 N, 20 mL) and water (2 × 20 mL). The organic phase was dried (Na2SO4), filtered and concentrated in vacuo to afford the crude product as a bright yellow oil.
N-[O-((2S,5R)-2-Methyl-O-phenylcarbonothioatyl) tetrahydro-5-(2-hydroxypropan-2-yl)furan-2-yl]-2-(2R)-camphor-10,2-sultam (31)
Following the general procedure to prepare thionoformate, diastereomerically pure cis-THF diol 11 (235 mg, 0.59 mmol) afforded the crude product as a yellow oil (220 mg). Purification by column chromatography (SiO2 eluting with EtOAc–hexane (10–25%)) afforded the thionoformate 31 as a yellow oil (104 mg, 0.19 mmol, 33%). [α]D = −37.0 (c 0.7, CHCl3, 24 °C); FT-IR νmax (neat) 3535, 2967, 2950, 2870, 1677, 1340, 1287, 1200, 1051 cm−1; 1H NMR (400 MHz, CDCl3) δ 7.41 (2H, t, J = 8.0 Hz, 2 × CH, Ar(meta)), 7.29 (1H, m, CH, Ar(para)), 7.11 (2H, t, J = 8.0 Hz, 2 × CH, Ar(ortho)), 4.98 (1H, d, J = 11.0 Hz, CHHO), 4.85 (1H, d, J = 11.0 Hz, CHHO), 4.16 (1H, t, J = 7.0 Hz, CHN), 4.10 (1H, dd, J = 7.5, 4.5 Hz, CH, THF), 3.55 (1H, d, J = 13.4 Hz, CHHSO2), 3.46 (1H, d, J = 13.4 Hz, CHHSO2), 2.84 (1H, br, OH), 2.50–1.87 (8H, m, 2 × CH2 and 2 × CH2, THF), 1.45–1.28 (3H, m, CH and CH2), 1.28 (3H, s, CH3), 1.23 (3H, s, CH3), 1.18 (3H, s, CH3), 1.01 (3H, s, CH3) ppm; 13C NMR (100 MHz, CDCl3) δ 195.08 (C
S), 175.27 (CON), 153.49 (CCH, Ar), 129.47 (2 × CH, Ar(meta)), 126.53 (CH, Ar(para)), 121.93 (2 × CH, Ar(ortho)), 88.89 (CCH2, THF), 87.46 (CH, THF), 75.42 (CH2O), 71.29 ((CH3)2C), 67.62 (CHN), 54.59 (CH2S), 47.92 (CCH2S), 47.57 (C(CH3)2), 45.48 (CHC(CH3)2), 39.24 (CH2CHN), 35.30 (CH2C), 33.69 (CH2, THF), 27.31 (CH2CH2C), 26.16 (CH2, THF), 25.98 (CH3), 23.98 (CH3), 21.77 (CH3), 19.91 (CH3) ppm; LRMS (ES+) m/z 560 (100%, [M + Na]+); HRMS (ES+) m/z calculated: 560.1747; found: 560.1749 ([M + Na]+).
Rac. O-((2S,5R)-2-(Methoxycarbonyl)tetrahydro-5-(2-hydroxypropan-2-yl)furan-2-yl)methyl-O-phenyl carbonothioate (32)
Following the general procedure for the preparation of thionoformate, racemic cis-THF diol 12 (145 mg, 0.66 mmol) afforded the crude product as a yellow oil (215 mg). Purification by column chromatography (SiO2 eluting with CH2Cl2–hexane (40–90%)) afforded the thionoformate 32 as a yellow oil (164 mg, 0.46 mmol, 70%). FT-IR νmax (neat) 3383, 2971, 2877, 1728, 1295, 1201, 1042, 772, 690 cm−1; 1H NMR (400 MHz, CDCl3) δ 7.45–7.35 (2H, m, 2 × CH, Ar(meta)), 7.27 (1H, m, CH, Ar(para)), 7.10–7.06 (2H, m, 2 × CH, Ar(ortho)), 4.84 (1H, d, 9J = 11.3 Hz, CHHO), 4.79 (1H, d, J = 11.3 Hz, CHHO), 4.12 (1H, t, J = 7.3 Hz, CH, THF), 3.78 (3H, s, OCH3), 2.29–2.12 (3H, m, OH and CH2, THF), 2.07–1.89 (2H, m, CH2, THF), 1.29 (3H, s, CH3), 1.15 (3H, s, CH3) ppm; 13C NMR (100 MHz, CDCl3) δ 194.98 (C
S), 172.73 (COO), 153.31 (CCH, Ar), 129.45 (2 × CH, Ar(meta)), 126.55 (CH, Ar(para)), 121.75 (2 × CH, Ar(ortho)), 88.23 (CH, THF), 84.53 (CCH2O, THF), 75.29 (CH2O), 71.04 ((CH3)2C), 52.57 (OCH3), 32.84 (CH2, THF), 27.31 (CH2, THF), 25.61 (CH3), 24.66 (CH3) ppm; LRMS (ES+) m/z 377 (100%, [M + Na]+); HRMS (ES+) m/z calculated: 377.1029; found: 377.1026 ([M + Na]+).
Rac. (2S,5R)-Methyl tetrahydro-2-(hydroxymethyl)-5-(2-trimethylsiloxy-2-yl)furan-2-carboxylate (33)
To a stirred solution of racemic THF diol 12 (397 mg, 1.68 mmol) and imidazole (1.14 g, 16.8 mmol) in DMF (3 mL) was added Me3SiCl (0.65 mL, 5.04 mmol) at 0 °C. The mixture was stirred at this temperature for 15 min. After this, anhydrous MeOH (2 mL) was added in the reaction mixture followed by the addition of K2CO3 (2 mg in 1 mL of anhydrous MeOH). The reaction mixture was stirred at 0 °C for 1.5 hours, whereupon it was quenched by acetic acid (5% aq., 5 mL). The organic phase was separated and the aqueous phase was re-extracted with Et2O (2 × 20 mL). The combined organic phases were dried (Na2SO4), concentrated in vacuo to afford the crude as a pale yellow oil (443 mg). Purification by column chromatography (SiO2 eluting with EtOAc–hexane (10–20%)) afforded the title racemic carbinol 33 as a pale yellow oil (428 mg, 1.47 mmol, 88%). FT-IR νmax (neat) 3465, 2950, 2890, 2868, 1732, 1242, 1169, 1037, 837 cm−1; 1H NMR (400 MHz, CDCl3) δ 3.96 (1H, t, J = 7.3 Hz, CH, THF), 3.84 (1H, dd, J = 11.3, 4.0 Hz, CHHOH), 3.74 (3H, s, OCH3), 3.71 (1H, dd, J = 11.3, 9.0 Hz, CHHOH), 2.91 (1H, dd, J = 9.0, 4.0 Hz, OH), 2.27–1.86 (4H, m, 2 × CH2), 1.37 (3H, s, CH3), 1.18 (3H, s, CH3), 0.15 (9H, s, Si(CH3)3) ppm; 13C NMR (100 MHz, CDCl3) δ 174.48 (COO), 88.56 (CCH2OH, THF), 86.81 (CH, THF), 75.18 ((CH3)2C), 66.14 (CH2OH), 52.16 (OCH3), 32.41 (CH2, THF), 27.37 (CH2, THF), 26.97 (CH3), 26.30 (CH3), 2.33 (3C, Si(CH3)3) ppm; LRMS (ES+) m/z 313 (100%, [M + Na]+); HRMS (ES+) m/z calculated: 313.1442; found: 313.1442 ([M + Na]+).
General procedure for the preparation of thionocarbamate
To a solution of mono-protected THF carbinol (1.0 mmol) in CH2Cl2 (0.05 M) was added DMAP (0.30 mmol) and thiocarbonyl diimidazole (3.0 mmol). The resultant bright yellow solution was stirred at room temperature for 8 hours. The reaction was concentrated in vacuo to give the crude product.
Rac. (2S,5R)-Methyl tetrahydro-2-(O-methoxy-1H-imidazol-1-carbothioyl)-5-(2-trimethylsiloxy-2-yl) furan-2-carboxylate (34)
Following the general method for the preparation of thionocarbamate, racemic mono-protected THF carbinol 33 (320 mg, 1.10 mmol) afforded crude as a yellow oil (445 mg). Purification by column chromatography (SiO2 eluting with EtOAc–hexane (10–20%)) afforded the title racemic thionocarbamate 34 as a yellow oil (409 mg, 1.02 mmol, 89%). FT-IR νmax (neat) 2965, 2953, 2840, 1737, 1390, 1330, 1286, 1246, 1173, 1037, 955, 839 cm−1; 1H NMR (400 MHz, CDCl3) δ 8.30 (1H, s, NCHN), 7.60 (1H, t, J = 1.4 Hz, CHN), 7.03 (1H, dd, J = 1.4, 0.8 Hz, NCHCH), 4.91 (1H, d, J = 10.8 Hz, CHHO), 4.73 (1H, d, J = 10.8 Hz, CHHO), 4.04 (1H, m, CH, THF), 3.79 (3H, s, OCH3), 2.28–1.93 (4H, m, 2 × CH2, THF), 1.27 (3H, s, CH3), 1.19 (3H, s, CH3), 0.12 (9H, s, Si(CH3)3) ppm; 13C NMR (100 MHz, CDCl3) δ 183.69 (C
S), 173.01 (COO), 136.87 (NCHN), 130.86 (NCHCH), 117.88 (CHN), 89.14 (CH, THF), 84.76 (CCH2O, THF), 75.84 (CH2OCS), 74.80 ((CH3)2C), 52.68 (OCH3), 33.12 (CH2, THF), 27.26 (CH2, THF), 26.18 (CH3), 25.47 (CH3), 2.48 (3C, Si(CH3)3) ppm; LRMS (ES+) m/z 423 (100%, [M + Na]+); HRMS (ES+) m/z calculated: 423.1386; found: 423.1392 ([M + Na]+).
N-[(2S,5R)-Tetrahydro-5-(2-hydroxypropan-2-yl)-2-methylfuranoyl]-2-(2R)-camphor-10,2-sultam (35)
To a solution of thionoformate 31 (45 mg, 0.08 mmol) in toluene (2.0 mL) was added AIBN (7 mg, 0.04 mmol), followed by dropwise addition of Bu3SnH (67 μL, 0.24 mmol). The resultant solution was heated to reflux for 4 hours. The reaction was concentrated in vacuo to afford crude product as a yellow oil (25 mg). Purification by column chromatography (SiO2 eluting with CH2Cl2–hexane (10–30%)) afforded the trans-THF product 35 as a yellow oil (19 mg, 0.05 mmol, 65%). [α]D = −32.97 (c 0.37, CHCl3, 26 °C); FT-IR νmax (neat) 3516, 2962, 2960, 2879, 1677, 1456, 1335, 1163, 1134, 1062, 912, 728 cm−1; 1H NMR (300 MHz, CDCl3) δ 4.08 (1H, m, CHN), 4.00 (1H, t, J = 7.0 Hz, CH, THF), 3.51 (1H, d, J = 13.4 Hz, CHHSO2), 3.40 (1H, d, J = 13.4 Hz, CHHSO2), 2.75 (1H, br, OH), 2.32 (1H, dd, J = 12.6, 8.0 Hz, CHH, THF), 2.10–1.69 (7H, m, 2 × CH2, CHH and CH2, THF), 1.53 (3H, s, CH3), 1.42–1.25 (3H, m, CHH and CH2), 1.19 (3H, s, CH3), 1.21 (3H, s, CH3), 1.13 (3H, s, CH3), 0.99 (3H, s, CH3) ppm; 13C NMR (75 MHz, CDCl3) δ 178.45 (CON), 87.77 (CH, THF), 87.46 (CCH3, THF), 71.29 ((CH3)2C), 67.68 (NCH), 54.59 (CH2S), 47.80 (C(CH3)2), 47.48 (CCH2S), 45.58 (CHC(CH3)2), 39.47 (CH2CHN), 39.34 (CH2C), 33.82 (CH2, THF), 26.80 (CH3), 26.13 (CH2, THF), 25.86 (CH2CH2C), 24.46 (CH3), 23.51 (CH3), 21.88 (CH3), 19.90 (CH3) ppm; LRMS (ES+) m/z 408 (100%, [M + Na]+); HRMS (ES+) m/z calculated: 408.1815; found: 408.1808 ([M + Na]+).
(2S,5R)-((1R,2S,5R)-5-Methyl-2-(2-phenylpropan-2-yl)cyclohexyl)tetrahydro-2-(hydroxymethyl)-5-(2-trimethylsiloxy-propan-2-yl)furan-2-carboxylate (36)
To a solution of bis-protected THF 27 (307 mg, 0.55 mmol) in MeOH (10 mL) was added dried powdered K2CO3 (83 mg) at −10 °C. The resultant milky mixture was stirred for 2.5 hours, while maintaining the temperature. The reaction was quenched by adding H2O (2 mL) and MeOH was evaporated. The resultant residue was partitioned between Et2O (30 mL) and H2O (15 mL), and the organic phase was separated. The aqueous phase was re-extracted with Et2O (2 × 30 mL), and the combined organic phases were dried (Na2SO4), filtered and concentrated in vacuo to give the crude as a yellow oil (270 mg). Purification by column chromatography (SiO2 eluting with CH2Cl2–hexane (60–100%)) afforded the title mono-TMS protected THF carbinol 36 as a pale yellow oil (262 mg, 0.53 mmol, 98%). [α]D = −7.34 (c 1.07, CHCl3, 26 °C); FT-IR νmax (neat) 3478, 2955, 2922, 2871, 1725, 1251, 1172, 1050, 841, 761, 701 cm−1; 1H NMR (400 MHz, CDCl3) δ 7.28–7.13 (5H, m, 5 × CH, Ar), 4.75 (1H, td, J = 10.6, 4.3 Hz, OCH), 3.94 (1H, m, CH, THF), 3.68 (1H, dd, J = 11.3, 3.7 Hz, CHHOH), 3.53 (1H, dd, J = 11.3, 9.4 Hz, CHHOH), 2.89 (1H, dd, J = 9.4, 3.7 Hz, OH), 2.15–1.84 (5H, m, CH and 2 × CH2, THF), 1.56–1.20 (5H, m, CH and 2 × CH2), 1.36 (3H, s, CH3), 1.33 (3H, s, CH3), 1.27 (3H, s, CH3), 1.17 (3H, s, CH3), 1.00–0.74 (2H, m, CH2), 0.84 (3H, s, d, J = 6.4 Hz, CH3CH), 0.14 (9H, s, COSi(CH3)3) ppm; 13C NMR (100 MHz, CDCl3) δ 173.49 (COO), 150.39 (CCH, Ar), 128.01 (2 × CH, Ar(meta)), 125.68 (2 × CH, Ar(ortho)), 125.37 (CH, Ar(para)), 88.59 (CCH2OH, THF), 86.13 (CH, THF), 75.91 (OCH), 75.02 (COSi(CH3)3), 65.92 (CH2OH), 50.42 (CHCPh), 41.41 (CPh), 40.19 (CH2CH(CH3)), 34.53 (CH2CH2CH), 32.55 (CH2, THF), 31.28 (CHCH3), 29.32 (CH3), 27.37 (CH3), 27.23 (CH2, THF), 27.07 (CH2C), 26.45 (CH3), 24.93 (CH3), 21.73 (CH3), 2.35 (3C, COSi(CH3)3) ppm; LRMS (ES+) m/z 513 (100%, [M + Na]+); HRMS (ES+) m/z calculated: 513.3007; found: 513.3020 ([M + Na]+).
(2S,5R)-((1R,2S,5R)-5-Methyl-2-(2-phenylpropan-2-yl)cyclohexyl)tetrahydro-2-(O-methoxy-1H-imidazol-1-carbothioyl)-5-(2-trimethylsiloxypropan-2-yl)furan-2-carboxylate (37)
Following the general procedure for the preparation of thionocarbamate, mono-protected THF carbinol 36 (260 mg, 0.53 mmol) afforded crude as a yellow oil (310 mg). Purification by column chromatography (SiO2 eluting with EtOAc–hexane (5–25%)) afforded the title thiocarbonyl imidazolide 37 as a yellow oil (297 mg, 0.49 mmol, 93%). [α]D = +7.85 (c 1.21, CHCl3, 27 °C); FT-IR νmax (neat) 2955, 2915, 2865, 1730, 1463, 1391, 1332, 1287, 1248, 1176, 1037, 955, 840, 764, 701 cm−1; 1H NMR (300 MHz, CDCl3) δ 8.31 (1H, t, J = 1.0 Hz, NCHN), 7.60 (1H, t, J = 1.0 Hz, CHN), 7.30–7.12 (5H, m, 5 × CH, Ar), 6.98 (1H, dd, J = 1.6, 1.0 Hz, NCHCH), 4.82 (1H, td, J = 10.6, 4.3 Hz, OCH), 4.71 (1H, d, J = 10.8 Hz, CHHO), 4.39 (1H, d, J = 10.8 Hz, CHHO), 4.04 (1H, t, J = 6.5 Hz, CH, THF), 2.04–1.73 (5H, m, CH and 2 × CH2, THF), 1.55–1.36 (5H, m, CH and 2 × CH2), 1.34 (3H, s, CH3), 1.21 (3H, s, CH3), 1.20 (3H, s, CH3), 1.19 (3H, s, CH3), 1.03–0.69 (2H, m, CH2), 0.73 (3H, d, J = 6.4 Hz, CH3CH), 0.07 (9H, s, COSi(CH3)3) ppm; 13C NMR (75 MHz, CDCl3) δ 183.78 (C
S), 171.89 (COO), 150.84 (CCH, Ar), 137.01 (NCHN), 130.72 (NCHCH), 128.14 (2 × CH, Ar(meta)), 125.45 (2 × CH, Ar(ortho)), 125.32 (CH, Ar(para)), 117.88 (CHN), 89.17 (CH, THF), 84.45 (CCH2OH, THF), 76.61 (OCH), 75.87 (CH2OCS), 74.64 (COSi(CH3)3), 49.88 (CHCPh), 41.40 (CPh), 39.99 (CH2CH(CH3)), 34.33 (CH2CH2CH), 32.77 (CH2, THF), 31.17 (CHCH3), 27.92 (CH3), 27.40 (CH3), 26.99 (CH2, THF), 25.98 (CH3), 25.92 (CH2C), 25.59 (CH3), 21.53 (CH3), 2.46 (3C, COSi(CH3)3) ppm; LRMS (ES+) m/z 623 (80%, [M + Na]+), 601 (550%, [M + H]+); HRMS (ES+) m/z calculated: 601.3126; found: 601.3111 ([M + H]+).
(2R,5R)-((1R,2S,5R)-5-Methyl-2-(2-phenylpropan-2-yl)cyclohexyl) tetrahydro-2-methyl-5-(2-trimethylsiloxypropan-2-yl)furan-2-carboxylate (38)
To a solution of thionocarbamate 37 (292 mg, 0.49 mmol) in toluene (20 mL) was added AIBN (20.1 mg, 0.13 mmol), followed by dropwise addition of (Me3Si)3SiH (0.6 mL, 1.96 mmol). The resultant solution was stirred at 85 °C for 75 min, then concentrated in vacuo to afford crude as a yellow oil (235 mg). Purification by column chromatography (SiO2 eluting with EtOAc–hexane (5–15%)) afforded the title THF adduct 38 as a yellow oil (196 mg, 0.42 mmol, 85%). [α]D = −18.03 (c 1.37, CHCl3, 28 °C); FT-IR νmax (neat) 2954, 2918, 2865, 1725, 1456, 1371, 1248, 1169, 1092, 1041, 837, 762, 700 cm−1; 1H NMR (400 MHz, CDCl3) δ 7.28–7.14 (5H, m, 5 × CH, Ar), 4.76 (1H, td, J = 10.7, 4.3 Hz, OCH), 3.90 (1H, dd, J = 7.5, 6.0 Hz, CH, THF), 1.99–1.79 (5H, m, CH and 2 × CH2, THF), 1.69–1.36 (5H, m, CH and 2 × CH2), 1.35 (3H, s, CH3), 1.33 (3H, s, CH3), 1.26 (3H, s, CH3), 1.20 (3H, s, CH3), 1.18 (3H, s, CH3), 1.00–0.70 (2H, m, CH2), 0.83 (3H, d, J = 6.4 Hz, CH3CH), 0.10 (9H, s, COSi(CH3)3) ppm; 13C NMR (100 MHz, CDCl3) δ 175.10 (COO), 150.59 (CCH, Ar), 127.98 (2 × CH, Ar(meta)), 125.71 (2 × CH, Ar(ortho)), 125.29 (CH, Ar(para)), 87.45 (CH, THF), 83.96 (CCH3 THF), 75.63 (OCH), 74.77 (COSi(CH3)3), 50.41 (CHCPh), 41.52 (CPh), 40.27 (CH2CH(CH3)), 36.56 (CH2CH2CH), 34.56 (CH2, THF), 31.29 (CHCH3), 29.65 (CH3), 27.86 (CH3), 27.31 (CH2, THF), 26.11 (CH2C), 25.28 (CH3), 24.53 (CH3), 23.96 (CH3), 21.76 (CH3), 2.57 (3C, COSi(CH3)3) ppm; LRMS (ES+) m/z 497 (100%, [M + Na]+); HRMS (ES+) m/z calculated: 497.3058; found: 497.3058 ([M + Na]+).
(2R,5R)-Tetrahydro-2-methyl-5-(2-trimethylsilyl-oxy-propan-2-yl)furan-2-carbaldehyde (39)
To a solution of mono-protected THF compound 38 (189 mg, 0.40 mmol) in CH2Cl2 (6 mL) was added DIBAL-H (1.2 mL, 1.2 mmol) at −78 °C and the resultant solution was stirred for 4 hours, while keeping the temperature at −78 °C. The reaction was quenched at −78 °C by adding sat. aq. NH4Cl (5 mL) and the temperature was allowed to rise to room temperature. Rochelle's salt (5 mL, aq. sat.) was added and the solution was stirred for 30 min at room temperature. The solution was diluted with CH2Cl2 (10 mL) and the organic phase was separated. The aqueous phase was re-extracted with CH2Cl2 (2 × 10 mL), and the combined organic phases were washed with brine (10 mL), dried (Na2SO4), filtered and concentrated in vacuo to give the crude as a yellow oil (175 mg). Purification by column chromatography (SiO2 eluting with EtOAc–hexane (5–15%)) afforded the title aldehyde 39 as a pale yellow oil (83 mg, 0.34 mmol, 85%), followed by the commercially available pure chiral auxiliary (−)-24 as a yellow oil (86 mg, 0.37 mmol, 92%). 39: [α]D = −1.46 (c 1.265, CHCl3, 29 °C); FT-IR νmax (neat) 2975, 2888, 2786, 1736, 1249, 1174, 1061, 1041, 839, 753 cm−1; 1H NMR (400 MHz, CDCl3) δ 9.58 (1H, s, CHO), 3.81 (1H, t, J = 7.0 Hz, CH, THF), 2.09 (1H, ddd, J = 12.0, 8.0, 6.8 Hz, CH, THF), 1.96 (1H, ddt, J = 12.0, 8.0, 6.8 Hz, CH, THF), 1.83 (1H, m, CH, THF), 1.68 (1H, ddd, J = 12.0, 8.0, 6.8 Hz, CH, THF), 1.30 (3H, s, CH3), 1.26 (3H, s, CH3), 1.20 (3H, s, CH3), 0.12 (9H, s, COSi(CH3)3) ppm; 13C DEPT135 NMR (100 MHz, CDCl3) δ 204.06 (CHO), 88.60 (CH, THF), 33.27 (CH2, THF), 27.92 (CH3), 26.58 (CH2, THF), 26.18 (CH3), 21.35 (CH3), 2.95 (3C, COSi(CH3)3) ppm; LRMS (ES+) m/z 267 (100%, [M + Na]+); HRMS (ES+) m/z calculated: 267.1387; found: 267.1385 ([M + Na]+).
Acknowledgements
The author gratefully acknowledges the invaluable guidance and generous financial support received from his mentor, Richard C. D. Brown (University of Southampton, UK), during his academic visits to the University of Southampton in 2012 and 2014. Also, thanks to the King Faisal University, Saudi Arabia for the support. The research described here was carried out by the author in the laboratory of Richard C. D. Brown (University of Southampton, UK) and is being published with his consent.
Notes and references
- For a selection of reviews concerning the synthesis of 2,5-disubstituted THF rings, see:
(a) T. L. B. Boivin, Tetrahedron, 1987, 43, 3309–3362 CrossRef CAS;
(b) J.-C. Harmange and B. Figadere, Tetrahedron: Asymmetry, 1993, 4, 1711–1754 CrossRef CAS;
(c) E. Keinan and S. C. Sinha, Pure Appl. Chem., 2002, 74, 93–105 CrossRef CAS;
(d) J. Hartung and M. Greb, J. Organomet. Chem., 2002, 661, 67–84 CrossRef CAS;
(e) J. P. Wolfe and M. B. Hay, Tetrahedron, 2007, 63, 261–290 CrossRef CAS PubMed;
(f) V. Piccialli, Synthesis, 2007, 17, 2585–2607 CrossRef PubMed;
(g) G. Jalce, X. Franck and B. Figadére, Tetrahedron: Asymmetry, 2009, 20, 2537–2581 CrossRef CAS PubMed;
(h) B. S. Pilgrim and T. J. Donohoe, J. Org. Chem., 2013, 78, 2149–2167 CrossRef CAS PubMed;
(i) V. Piccialli, Molecules, 2014, 19, 6534–6582 CrossRef CAS PubMed;
(j) N. S. Sheikh, Nat. Prod. Rep., 2014, 31, 1088–1100 RSC;
(k) N. S. Sheikh, Org. Biomol. Chem., 2014, 12, 9492–9504 RSC.
- F. M. Uckun, C. Mao, A. O. Vassilev, C. S. Navara, R. K. S. Narla and S.-T. Jan, Bioorg. Med. Chem. Lett., 2000, 10, 1015–1018 CrossRef CAS.
- S.-T. Jan, C. Mao, A. O. Vassilev, C. S. Navara and F. M. Uckun, Bioorg. Med. Chem. Lett., 2000, 10, 1193–1197 CrossRef CAS.
-
(a) B. Liu, S. Duan, A. C. Sutterer and K. D. Moeller, J. Am. Chem. Soc., 2002, 124, 10101–10111 CrossRef CAS PubMed;
(b) F. Volz, S. H. Wadman, A. Hoffmann-Röder and N. Krause, Tetrahedron, 2009, 65, 1902–1910 CrossRef CAS PubMed.
- H. Morita, E. Kishi, K. Takeya, H. Itokawa and Y. Iitaka, Phytochemistry, 1993, 34, 765–771 CrossRef CAS.
-
(a) D. E. Cane, W. D. Celmer and J. W. Westley, J. Am. Chem. Soc., 1983, 105, 3594–3600 CrossRef CAS;
(b) J. J. Fernandez, M. L. Souto and M. Norte, Nat. Prod. Rep., 2000, 17, 235–246 RSC;
(c) Y. Kashman and A. Rudi, Phytochem. Rev., 2004, 3, 309–323 CrossRef CAS;
(d) A. R. Gallimore, Nat. Prod. Rep., 2009, 26, 266–280 RSC.
-
(a) W. J. Schultz, M. C. Etter, A. V. Pocius and S. Smith, J. Am. Chem. Soc., 1980, 102, 7981–7982 CrossRef CAS;
(b) H. Tsukube, K. Takagi, T. Higashiyama, T. Iwachido and N. Hayama, Inorg. Chem., 1994, 33, 2984–2987 CrossRef CAS;
(c) Y. Morimoto, T. Iwai, T. Yoshimura and T. Kinoshita, Bioorg. Med. Chem. Lett., 1998, 8, 2005–2010 CrossRef CAS.
- Re(VII) oxidative cyclisation to trans-THF rings:
(a) R. M. Kennedy and S. Tang, Tetrahedron Lett., 1992, 33, 3729–3732 CrossRef CAS;
(b) S. Tang and R. M. Kennedy, Tetrahedron Lett., 1992, 33, 5299–5302 CrossRef CAS;
(c) S. Tang and R. M. Kennedy, Tetrahedron Lett., 1992, 33, 5303–5306 CrossRef CAS;
(d) R. S. Boyce and R. M. Kennedy, Tetrahedron Lett., 1994, 35, 5133–5136 CrossRef CAS;
(e) F. E. McDonald and T. B. Towne, J. Org. Chem., 1995, 60, 5750–5751 CrossRef CAS;
(f) T. B. Towne and F. E. McDonald, J. Am. Chem. Soc., 1997, 119, 6022–6028 CrossRef CAS;
(g) Y. Morimoto and T. Iwai, J. Am. Chem. Soc., 1998, 120, 1633–1634 CrossRef CAS.
- S. Inoki and T. Mukaiyama, Co(II) oxidative cyclisation to trans-THF rings, Chem. Lett., 1990, 19, 67–70 CrossRef.
- Mn(VII) oxidative cyclisation to cis-THF rings:
(a) E. Klein and W. Rojahn, Tetrahedron, 1965, 21, 2353–2358 CrossRef CAS;
(b) J. E. Baldwin, M. J. Crossley and E. M. M. Lehtonen, J. Chem. Soc., Chem. Commun., 1979, 918–920 RSC;
(c) D. M. Walba, M. Wand and M. Wilkes, J. Am. Chem. Soc., 1979, 101, 4396–4397 CrossRef CAS;
(d) R. C. D. Brown and J. F. Keily, Angew. Chem., Int. Ed., 2001, 40, 4496–4498 CrossRef CAS.
- Os(VIII) oxidative cyclisation to cis-THF rings:
(a) M. de Champdoré, M. Lasalvia and V. Piccialli, Tetrahedron Lett., 1998, 39, 9781–9784 CrossRef;
(b) T. J. Donohoe, J. J. G. Winter, M. Helliwell and G. Stemp, Tetrahedron Lett., 2001, 42, 971–974 CrossRef CAS;
(c) T. J. Donohoe and S. Butterworth, Angew. Chem., Int. Ed., 2003, 42, 948–951 CrossRef CAS PubMed.
- Ru(VIII) oxidative cyclisation to cis-THF rings:
(a) P. H. J. Carlsen, T. Katsuki, V. S. Martin and K. B. Sharpless, J. Org. Chem., 1981, 46, 3937–3938 CrossRef;
(b) L. Albarella, D. Musumeci and D. Sica, Eur. J. Org. Chem., 2001, 997–1003 CrossRef CAS;
(c) V. Piccialli and N. Cavallo, Tetrahedron Lett., 2001, 42, 4695–4699 CrossRef CAS;
(d) V. Piccialli and T. Caserta, Tetrahedron Lett., 2004, 45, 303–308 CrossRef CAS PubMed;
(e) S. Roth, S. Göhler, H. Cheng and C. B. W. Stark, Eur. J. Org. Chem., 2005, 19, 4109–4118 CrossRef;
(f) S. Göhler, S. Roth, H. Cheng, H. Goksel, A. Rupp, L. O. Haustedt and C. B. W. Stark, Synthesis, 2007, 17, 2751–2754 Search PubMed;
(g) S. Göhler and C. B. W. Stark, Org. Biomol. Chem., 2007, 5, 1605–1614 RSC.
- Os(VI) oxidative cyclisation to cis-THF rings:
(a) T. J. Donohoe and S. Butterworth, Angew. Chem., Int. Ed., 2005, 44, 4766–4768 CrossRef CAS PubMed;
(b) T. J. Donohoe, P. C. M. Winship and D. S. J. Walter, J. Org. Chem., 2009, 74, 6394–6397 CrossRef CAS PubMed.
- Cr(VI) oxidative cyclisation to cis-THF rings:
(a) B. D. Hammock, S. S. Gill and J. E. Casida, J. Agric. Food Chem., 1974, 22, 379–385 CrossRef CAS;
(b) D. M. Walba and G. S. Stoudt, Tetrahedron Lett., 1982, 23, 727–730 CrossRef CAS.
- H. Chang and C. B. W. Stark, Ru(VIII) oxidative cyclisation to cis-THF rings, Angew. Chem., Int. Ed., 2010, 49, 1587–1590 CrossRef PubMed.
- T. J. Donohoe, K. M. P. Wheelhouse, P. J. Lindsay-Scott, G. H. Churchill, M. J. Connolly and P. A. Glossop, Asian J. Chem., 2009, 4, 1237–1247 CrossRef CAS PubMed.
-
(a) D. G. Lee and J. R. Brownridge, J. Am. Chem. Soc., 1973, 95, 3033–3034 CrossRef CAS;
(b) S. Wolfe, C. F. Ingold and R. U. Lemieux, J. Am. Chem. Soc., 1981, 103, 938–939 CrossRef CAS;
(c) K. N. Houk and T. Strassner, J. Org. Chem., 1999, 64, 800–802 CrossRef CAS PubMed;
(d) A. Poethig and T. Strassner, Collect. Czech. Chem. Commun., 2007, 72, 715–727 CrossRef CAS.
- T. J. Donohoe, R. M. Harris, O. Williams, G. C. Hargaden, J. Burrows and J. Parker, J. Am. Chem. Soc., 2009, 131, 12854–12861 CrossRef CAS PubMed.
-
(a) N. S. Sheikh, C. J. Bataille, T. J. Luker and R. C. D. Brown, Org. Lett., 2010, 12, 2468–2471 CrossRef CAS PubMed;
(b) A. M. Al Hazmi, N. S. Sheikh, C. J. R. Bataille, A. A. M. Al-Hadedi, S. V. Watkin, T. J. Luker, N. P. Camp and R. C. D. Brown, Org. Lett., 2014, 16, 5104–5107 CrossRef CAS PubMed.
-
(a) B. M. Trost, D. M. T. Chan and T. N. Nanninga, Org. Synth., 1984, 62, 58–66 CrossRef CAS;
(b) M. R. Masjedizadeh, D.-D. Ingeborg and R. D. Little, J. Org. Chem., 1990, 55, 2742–2752 CrossRef CAS;
(c) D. F. Taber and J. P. Louey, Tetrahedron, 1995, 51, 4495–4506 CrossRef CAS.
-
(a) E. J. Corey and G. H. Posner, J. Am. Chem. Soc., 1968, 90, 5616–5617 CrossRef CAS;
(b) F. M. Hauser, P. Hewawsam and D. Mal, J. Am. Chem. Soc., 1988, 110, 2919–2924 CrossRef CAS.
- X. Chen, T. Li, F. Nan, S. Shao and Y. Li, Tetrahedron, 1993, 49, 3075–3080 CrossRef CAS.
- For the synthesis of (2R)-10,2-camphorsultam, see:
(a) P. D. Bartlett and L. H. Knox, Org. Synth., 1965, 45, 14–16 CrossRef CAS;
(b) J. C. Towson, M. C. Weismiller, G. S. Lal, A. C. Sheppard and F. A. Davis, Org. Synth., 1990, 69, 158–168 CrossRef CAS;
(c) W. Oppolzer, C. Chapuis and G. Bernardinelli, Helv. Chim. Acta, 1984, 67, 1397–1401 CrossRef CAS;
(d) W. Oppolzer, D. Dupuis, G. Poli, T. M. Raynham and G. Bernardinelli, Tetrahedron Lett., 1988, 29, 5885–5888 CrossRef CAS.
- For selected recent examples illustrating the use of (2R)-10,2-camphorsultam as a chiral auxiliary, see:
(a) R. Ramesh, P. S. Swaroop, R. G. Gonnade, C. Thirupathi, R. A. Waterworth, J. G. Millar and D. S. Reddy, J. Org. Chem., 2013, 78, 6281–6284 CrossRef CAS PubMed;
(b) E. Kumazaki and H. Nagano, Tetrahedron, 2013, 69, 3486–3494 CrossRef CAS PubMed;
(c) K.-I. Takao, S. Sakamoto, M. A. Touati, Y. Kusakawa and K.-I. Tadano, Molecules, 2012, 17, 13330–13344 CrossRef CAS PubMed.
- W. Oppolzer and A. J. Kingma, Helv. Chim. Acta, 1989, 72, 1337–1345 CrossRef CAS.
- For some additional examples of permanganate-mediated oxidative cyclisation of 1,5-dienes, see:
(a) A. R. L. Cecil and R. C. D. Brown, Org. Lett., 2002, 4, 3715–3718 CrossRef CAS PubMed;
(b) A. R. L. Cecil, Y. Hu, M. J. Vicent, R. Duncan and R. C. D. Brown, J. Org. Chem., 2004, 69, 3368–3374 CrossRef CAS PubMed;
(c) R. C. D. Brown, C. J. Battaille, R. M. Hughes, A. Kenney and T. J. Luker, J. Org. Chem., 2002, 67, 8079–8085 CrossRef CAS PubMed;
(d) R. C. D. Brown, R. M. Hughes, J. Keily and A. Kenney, Chem. Commun., 2000, 1735–1736 RSC;
(e) G. D. Head, W. G. Whittingham and R. C. D. Brown, Synlett, 2004, 8, 1437–1439 Search PubMed;
(f) Y. Hu and R. C. D. Brown, Chem. Commun., 2005, 5636–5637 RSC;
(g) L. J. Brown, I. B. Spur, S. C. Kemp, N. P. Camp, K. R. Gibson and R. C. D. Brown, Org. Lett., 2008, 10, 2489–2492 CrossRef CAS PubMed;
(h) R. A. Bhunnoo, L. D. I. Hobbs, M. E. Light and R. C. D. Brown, Org. Biomol. Chem., 2009, 7, 1017–1024 RSC;
(i) C. L. Morris, Y. Hu, G. D. Head, L. J. Brown, W. G. Whittingham and R. C. D. Brown, J. Org. Chem., 2009, 74, 981–988 CrossRef CAS PubMed;
(j) S. B. A. Ghani, J. M. Chapman, B. Figadère, J. M. Herniman, G. J. Langley, S. Niemann and R. C. D. Brown, J. Org. Chem., 2009, 74, 6924–6928 CrossRef PubMed;
(k) S. B. A. Ghani, L. J. Brown, B. Figadère and R. C. D. Brown, Org. Biomol. Chem., 2010, 8, 4543–4545 RSC.
- F. Dumas, B. Mezrhab, J. d’Angelo, C. Riche and A. Chiaroni, J. Org. Chem., 1996, 61, 2293–2304 CrossRef CAS.
- O. Reiser, in Organic Synthesis, Highlights IV, ed. H.-G. Schmalz, Wiley-VCH, Weinheim, 2000, pp. 11–16 Search PubMed.
- J. S. Foot, H. Kanno, G. M. P. Giblin and R. J. K. Taylor, Synthesis, 2003, 1055–1064 CAS.
- For selected recent examples illustrating the use of (S)-4-benzyloxazolidin-2-one as a chiral auxiliary, see:
(a) Y. Suga, H. Fuwa and M. Sasaki, J. Org. Chem., 2014, 79, 1656–1682 CrossRef CAS PubMed;
(b) D. Poth, P. S. Peram, M. Vences and S. Schulz, J. Nat. Prod., 2013, 76, 1548–1558 CrossRef CAS PubMed;
(c) K. Kempf, A. Raja, F. Sasse and R. Schobert, J. Org. Chem., 2013, 78, 2455–2461 CrossRef CAS PubMed;
(d) G. Sabitha, K. Shankaraaiah, M. N. Prasad and J. S. Yadav, Synthesis, 2013, 45, 251–259 CrossRef CAS PubMed;
(e) Y. Minko, M. Pasco, L. Lercher, M. Botoshansky and I. Marek, Nature, 2012, 490, 522–526 CrossRef CAS PubMed;
(f) C. Cook, F. Liron, X. Guinchard and E. Roulland, J. Org. Chem., 2012, 77, 6728–6742 CrossRef CAS PubMed.
- For selected recent examples illustrating the use of (−)-8-phenylmenthol as a chiral auxiliary, see:
(a) X. García-Mera, M. J. Alves, A. Goth, M. L. do Vale and J. E. Rodríguez-Borges, Tetrahedron, 2013, 69, 2909–2919 CrossRef PubMed;
(b) S. G. Davies, J. A. Lee, P. M. Roberts, J. E. Thomson and J. Yin, Tetrahedron, 2011, 67, 6382–6403 CrossRef CAS PubMed;
(c) S. Ikeda, M. Shibuya, N. Kanoh and Y. Iwabuchi, Org. Lett., 2009, 11, 1833–1836 CrossRef CAS PubMed;
(d) S. Fustero, M. Sánchez-Roselló, J. L. Aceña, B. Fernández, A. Asensio, J. F. Sanz-Cervera and C. del Pozo, J. Org. Chem., 2009, 74, 3414–3423 CrossRef CAS PubMed;
(e) M. Pettersson, D. Knueppel and S. F. Martin, Org. Lett., 2007, 9, 4623–4626 CrossRef CAS PubMed;
(f) E. Tayama and H. Kimura, Angew. Chem., Int. Ed., 2007, 46, 8869–8871 CrossRef CAS PubMed;
(g) E. Bergin, C. T. O'Connor, S. B. Robinson, E. M. McGarrigle, C. P. O'Mahony and D. G. Gilheany, J. Am. Chem. Soc., 2007, 129, 9566–9567 CrossRef CAS PubMed.
- S. Yashwant, Y. S. Kulkarni, M. Niwa, E. Ron and B. B. Snider, J. Org. Chem., 1987, 52, 1568–1576 CrossRef.
- Major diastereoisomer was tentatively assigned by analogy with cis-THF diols 11 and 26, which is in accordance with the proposed conformational analysis.
- Assignment of major diastereoisomer obtained from (1R,2S,5R)-5-methyl-2-(2-phenylpropan-2-yl)cyclohexanol ((−)-24) was assigned by analogy with (1S,2R)-trans-2-tritylcyclohexanol (ref. 19b), which was confirmed by stereoselective total synthesis of trans-(2S,5S)-linalool oxide.
-
(a) R. E. Ireland, R. S. Meissner and M. A. Rizzacasa, J. Am. Chem. Soc., 1993, 115, 7166–7172 CrossRef CAS;
(b) D. A. Evans, H. A. Rajapakse and D. Stenkamp, Angew. Chem., Int. Ed., 2002, 41, 4569–4573 CrossRef CAS.
- S. A. Ramachandran, R. K. Kharul, S. Marque, P. Soucy, F. Jacques, R. Chenevert and P. Deslongchamps, J. Org. Chem., 2006, 71, 6149–6156 CrossRef CAS PubMed.
- J. U. Rhee, B. I. Bliss and T. V. RajanBabu, J. Am. Chem. Soc., 2003, 125, 1492–1493 CrossRef CAS PubMed.
- H. S. Park, H. Y. Lee and Y. H. Kim, Org. Lett., 2005, 7, 3187–3190 CrossRef CAS PubMed.
-
(a) D. H. R. Barton and S. W. McCombie, J. Chem. Soc., Perkin Trans. 1, 1975, 1574–1585 RSC;
(b) D. A. Evans, H. A. Rajapakse and D. Stenkamp, Angew. Chem., Int. Ed., 2002, 41, 4569–4573 CrossRef CAS.
-
(a) C. Chatgilialoglu, J. Org. Chem., 1988, 53, 3641–3642 CrossRef CAS;
(b) C. Chatgilialoglu, Acc. Chem. Res., 1992, 25, 188–194 CrossRef CAS.
- W. L. F. Armarego and D. D. Perrin, Purification of Laboratory Chemicals, Butterworth-Heinemann Ltd, Oxford, UK, 4th edn, 1997 Search PubMed.
Footnote |
† Electronic supplementary information (ESI) available. See DOI: 10.1039/c4ra13258k |
|
This journal is © The Royal Society of Chemistry 2015 |
Click here to see how this site uses Cookies. View our privacy policy here.