DOI:
10.1039/C4RA11726C
(Paper)
RSC Adv., 2015,
5, 1244-1261
New 1,4-anthracenedione derivatives with fused heterocyclic rings: synthesis and biological evaluation†
Received
3rd October 2014
, Accepted 21st November 2014
First published on 21st November 2014
Abstract
Several terpenylquinones derived from 1,4-anthracenedione (1,4-anthracenequinone, AQ) have been prepared by addition or substitution nucleophilic reactions and further transformed into extended polycyclic systems, which mainly kept the 1,4-quinone moiety fused to different nitrogen-heterocyclic rings (pyrrole, imidazole, pyrazine or quinoxaline) into the structure. The compounds synthesized were evaluated for their antineoplastic, antifungal and antiviral activities. GI50 antineoplastic values remained under μM levels for AQs, while the heterocyclic derivatives showed antifungal MIC values in the low μg mL−1 range against yeasts and filamentous fungi. Only few compounds displayed a discrete non-selective antiherpetic activity in the μg mL−1 range.
1. Introduction
The quinone/hydroquinone moiety is a common structural feature in a large number of natural and synthetic compounds with a wide range of biological activities such as anticancer,1–4 antiparasitic,5 antimicrobial,6 antifungal,7 antiviral,8 anti-oxidant,1b,9 etc., and it is possible to find agents, derived from benzoquinone (BQ), naphthoquinone (NQ) or anthracenequinone (AQ), used clinically as anticancer agents10 such as daunorubicin or mitomycin C (Fig. 1A). The mechanisms of the antineoplastic cytotoxicity of quinone derivatives have been widely studied, and it is known that they usually participate in important biological redox processes and can also act as intercalating or alkylating agents.11 The potential of quinones to generate reactive oxygen species (ROS) has been described in the literature,12 as well as the different targets that might be involved in its molecular mechanisms of action depending on the tumour cell type.13 For instance, the DNA cross-linking and monoalkylation by mitomycin C require the reduction of the quinone ring, while furonaphthoquinones are able to modify the mitochondrial permeability through ROS generation.12c In this sense, quinones can be considered as multi-target drugs.14 Several of those bioactive quinones bore a heterocyclic fragment fused to the quinone moiety, including five and six-membered heterocycles with nitrogen,15 oxygen16 and/or sulphur17 atoms. It has also been reported that the presence of an N-heterocyclic moiety in a polycyclic planar structure contributes to enhance their activity,18 being important too the number and position of the nitrogen atoms in the heterocyclic system.19
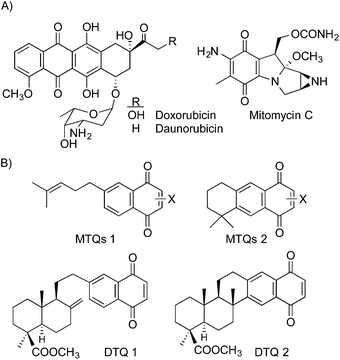 |
| Fig. 1 (A) Structure of clinically used anticancer quinones. (B) Structure of several monoterpene-derived (MTQ) and diterpene-derived (DTQ) antineoplastic quinones. | |
Among the bioactive quinones, 1,4-NQs and 9,10-AQs have been extensively studied. In contrast, AQs with the quinone carbonyls located at other positions are much less frequent and only the antitumor activity20,21 and the promising anti-trypanosomal profile22 of a limited number of 1,4-AQs has been described in the last few years. These facts prompted us to design and prepare new 1,4-AQ derivatives, including a number of heterocycle-fused quinones for evaluating them, not only for their expected antineoplastic cytotoxicity, but also for their antifungal activity, based on previous results reported by us for NQs and AQs23 and prospectively, against viruses, based on the assumption of their wide involvement in bio-redox processes, on their structural novelty and on the absence of previous evaluations in such field. Complementarily, it can be noted that antifungal and antiviral activities reported for quinones were often related to their reduction potential,6c,8b,11 and that recent mechanistic studies revealed that the antifungal activity of several NQs against various Candida spp. strains was related to the generation of ROS,24 in the same way as other antifungals as amphotericin B, miconazole or histatin 5 also do.25
In our search focused to the induction of antineoplastic and antimicrobial activities on natural inactive terpenoids, we prepared and evaluated a large number of 1,4-NQs, with the model structures shown in Fig. 1B, starting from natural terpenoids such as myrcene and myrcecommunic acid.26,27 They were synthesized through Diels–Alder cycloaddition between the natural terpenoids and p-benzoquinone. The preliminary biological results indicated that monoterpenylquinones (MTQs) and diterpenylquinones (DTQs) (Fig. 1B) were cytotoxic and good candidates to further structural optimization, becoming our lead compounds in order to improve their bioactivity profiles. Several structural modifications, dealing with size and oxidation degree of the quinone ring, were performed on those derivatives, including changes of substituents in the side chain or in the quinone ring.26,27
From those studies, we deduced that the presence of a terpenyl residue attached to the benzenoid ring of the NQ moiety improved the cytotoxicity of 1,4-NQ itself, and we attained to obtain some derivatives with GI50 values in the μM range against several tumour cell lines. Among them, we also prepared a number of 1,4-AQs by cyclization of the side chain in the MTQs 1 to get MTQs 2 (Fig. 1B), which also showed a very interesting cytotoxicity.21
In a prospective manner, several representative MTQs were evaluated against human pathogenic yeasts and filamentous fungi, with promising antifungal results found for several chlorinated derivatives.23
The incidence of opportunistic infections caused by microorganisms including fungi and viruses have increased significantly in recent decades, mainly in immunocompromised patients as those under anticancer treatment, who are susceptible to opportunistic infections. Among fungal pathogens, the species from Candida, Aspergillus and Cryptococcus genera are the most frequent in human infections.28 With the increase of these infections, has also increased the occurrence of secondary resistance by prolonged treatment with antifungals or by using them in prophylactic treatments.29 Also, infections caused by other emerging fungi as Fusarium spp. and Scedosporium spp. are increasing. Within these genera there are multiresistant strains or with variable susceptibility to antifungal drugs.30
Infections by Human Herpes virus type 1 (HHV-1) and Human Herpes virus type 2 (HHV-2) are also important due to morbidity that they can cause31 in immunocompromised patients. In this sense, the multitarget approach could be advantageous over drug combination, as it has been recently discussed,22a,32 and would reinforce the convenience of evaluating the new compounds for their antifungal and antiviral potential, in addition to the antineoplastic evaluation. Discovering entities that can be effective against two or more pathologies improves the chance of efficacy, and could prevent the development of the more and more frequent appearance of multi-drug resistant strains.33,34
Keeping in mind that quinone systems are involved in a wide range of biological activities and that the presence of nitrogen atoms can contribute to improve the bioactivity of quinone systems, together with our previous results with terpenylquinones, we planned to synthesize several new 1,4-AQs bearing fused nitrogen-heterocyclic rings very common in drug molecules, such as pyrrole, imidazole, pyrazine or quinoxaline, in order to analyze the influence of the enlargement of the heteropolycyclic planar system on their bioactivity. The synthesis of such new heterocycle-fused 1,4-AQ derivatives and the results of their evaluation in the three proposed pathogenic areas are reported and discussed here.
2. Results and discussion
2.1. Chemistry
The heterocyclic-fused quinones objective of this study included naphthoindole, anthraimidazole, anthraoxazole, naphthophenazine, naphthoquinoxaline and anthraimidazopyridine derivatives. We have used as starting compounds the AQs 1, 2 and 3 previously obtained by us through Diels–Alder cycloaddition between myrcene and p-benzoquinone derivatives as reported21,23 (Fig. 2).
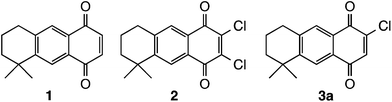 |
| Fig. 2 Anthracene-1,4-quinones used as starting materials. | |
The actual precursors of the heterocyclic systems were obtained through nucleophilic processes,35 either addition or substitution reactions, using N-nucleophiles as it is represented in Schemes 1–9. As the starting quinones are non-symmetrically substituted, the corresponding reaction products consisted of approximately 1
:
1 mixtures of the two expected regioisomers. However, in several cases they were separated by column chromatography in quantities enough to facilitate their independent spectroscopic characterization, and to perform further chemical transformations and their biological evaluation. NMR spectra of representative compounds and complete 1H and 13C NMR data assignments are included in the ESI.†
2.1.1. Pyrrole fused systems. Michael addition of aliphatic or aromatic amines to AQ 1 gave the monosubstituted AQs 4–8 as 1
:
1 mixtures of regioisomers, which were separated by CC to allow independent characterization and biological evaluation23 (Scheme 1). The regioisomers 4a and 4b, which were unequivocally identified in our previous work,23 were used to obtain the naphtho[2,3-f]indole-4,11-diones 9 and 10 by condensation with β-dicarbonyl compounds via a cerium(IV) mediated oxidative free radical reaction.36 The condensation reaction was carried out with each regioisomer independently. Thus, 4a was treated with 2,4-pentanedione or ethyl acetoacetate and CAN in methanol at room temperature to yield 9a and 10a respectively. When AQ 4b was used as the starting material, derivatives 9b and 10b were obtained in moderate yields. The formation of the pyrrole ring fused to the quinone system was deduced from the analysis of 1H NMR spectra, in which the signal at 5.6 ppm corresponding to the hydrogen H-3 in compounds 4, disappeared from the spectrum and new singlets at 2.4 and 2.7 (and 3.9) ppm were observed respectively for compounds 9 (and 10) (see ESI†). The correlations observed in two-dimensional NMR spectra obtained for 9a and 9b confirmed the structures assigned to both naphthoindolediones.
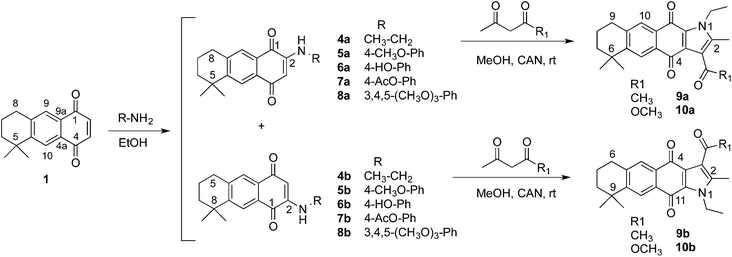 |
| Scheme 1 Preparation of aminoanthracenediones 4–8 and naphtho[2,3-f]indole-4,11-diones 9 and 10. | |
2.1.2. Imidazole-fused systems. The imidazole ring can be formed through the condensation of an acylamino and an alkylamino groups attached to the quinone fragment. Such groups would be previously introduced by substitution of the two chlorine atoms on the dichloro-AQ 2 as stated in the retrosynthetic Scheme 2.
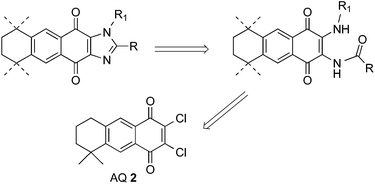 |
| Scheme 2 Retrosynthetic scheme for imidazole-fused AQs. | |
Dichloro-AQ 2 was obtained from 1,23 and both halogen atoms were subsequently substituted using N-nucleophiles. It is known that aliphatic amines and anilines smoothly substitute one chlorine atom, but such substitution induces electronic enrichment of the quinone system and makes difficult the replacement of the second chlorine atom.35,37 The second reaction step could be favoured if a substituent with electron-withdrawing effect is attached to the quinone ring. Due to that, we chose to acylate the monoaminoquinone, previously to the introduction of the alkylamino substituent. For such proposes, two different acyl groups, acetyl and benzoyl, were chosen.
Thus, treatment of 2 with sodium azide in acetic acid, followed by reduction with NaBH4 yielded the mixture of regioisomers 11 in low yield (data not shown). However, when ammonia was used as nucleophile, AQs 11 were obtained in 95% yield (Scheme 3). Reaction of 11 with acetic anhydride, in the presence of sulphuric acid at 0 °C, afforded the monoacylated AQs 12 in moderate yield. In order to improve the result, the acylation reaction was performed at room temperature and the imide derivatives 13 were obtained in good yield. When the monoacylated AQs 12 were treated in the same conditions, the same imide analogues 13 were obtained together with the oxazoles 14. The formation of the later can be easily explained through the intramolecular displacement of the halogen by the oxygen atom of the tautomerized amide function.
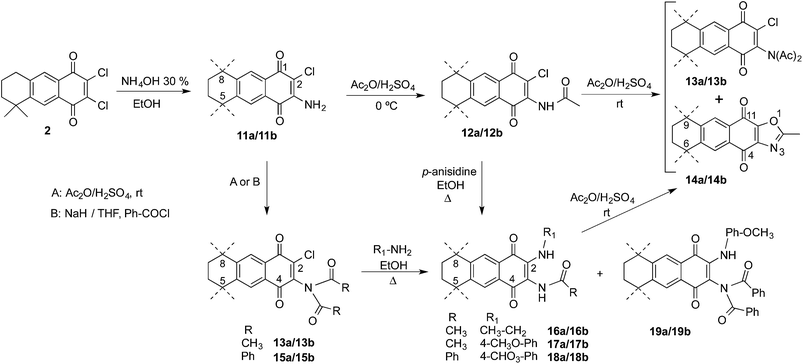 |
| Scheme 3 Preparation of intermediates and heterocycle-fused 1,4-anthraquinones. | |
When chloroquinones 11 were treated with benzoyl chloride in basic media, only the dibenzoylated AQs 15 were detected (Scheme 3). The next step was the substitution of the second chlorine atom using alkylamines and anilines. The corresponding monoacylated derivatives 16–18 were obtained when AQs 13 and 15 were submitted to the substitution reaction, in which one acyl group was transferred to the amine, used in excess in the reaction, as can be deduced from the acetyl-p-anisidine isolated during the chromatographic purification of the reaction products. In the case of 15, the imides 19 were also formed and the reaction of 12 with p-anisidine also gave the monoacylated analogues 17. Aiming to obtain the diacylated analogues from 16 and 17, both were treated with acetic anhydride in the presence of sulphuric acid but instead the expected products, the oxazoles 14 were formed again as the main products (up to 60% yield).
The reactions performed until this point gave mixtures of the two regioisomers in a 1
:
1 ratio. In some cases they were separated by CC, but the exact position of the substituents in the AQ ring was undetermined, so that the same reactions were applied to the major regioisomer 3a, obtained previously at our laboratory.21b When 3a was treated with ammonia followed by acetic anhydride, the imide 13a was obtained in quantitative yield. The 1H NMR spectrum of 13a showed only two singlet signals over 6 ppm (δ 7.83 and 8.04 ppm) indicating that an addition reaction took place at the position C-3, instead of the substitution reaction of the chlorine atom. Further substitution with primary amines afforded 16a and 17a, which allowed us to identify regioisomer “a” as the one with the acyl group on the same side as the geminal methyl groups (Scheme 4).
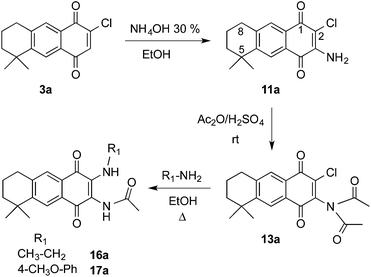 |
| Scheme 4 Synthesis of AQ regioisomers 16a and 17a. | |
Once in our hands the disubstituted AQs 16–18, the regioisomers 16a, 16b, 17a, 17b separately and the mixture 18a/18b were treated with NaOH to give the imidazole derivatives 20–22 in good yields (Scheme 5).
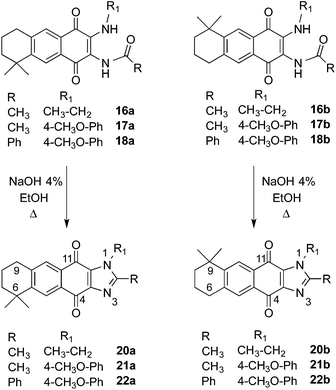 |
| Scheme 5 Preparation of anthra[2,3-d]imidazole-4,11-diones. | |
2.1.3. Other heterocycle-fused systems. In a previous work21b we found that 1,2-naphthoquinones (1,2-NQs) fused to a furan ring were more cytotoxic than the corresponding 1,4-NQs. Similar results were obtained with 1,2-naphthohydroquinone diacetates38 and after that, we explored the formation of 1,2-quinone analogues fused to nitrogenated rings. To get such type of compounds, we chose 2-aminopyridine as nucleophile to react with the dichloro-AQ 2. It was expected that the aromatic nitrogen promoted first the nucleophilic substitution of one chlorine atom, and then the reaction could evolve in two different ways, depending on if the amino group would produce the substitution of the second chlorine atom or the condensation with the close quinone carbonyl. In the later case, the remaining chlorine atom would be replaced by a hydroxyl group, which should favour the formation of the o-quinone.39 Both possible products are represented in Scheme 6.
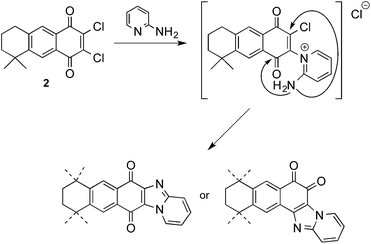 |
| Scheme 6 Possible reaction products of anthracenequinone 2 with 2-aminopyridine. | |
Thus, when AQ 2 was treated with 2-aminopyridine in methanol or ethanol, in the presence of K2CO3, complex reaction products were obtained from which the major products were the dimethoxy and diethoxy derivatives 23 and 24 respectively, resulting from the substitution of both chlorine atoms by the alcohol used as solvent, along with a small amount of the corresponding o-quinones 25 (Scheme 7). The formation of the alkoxy derivatives 23 and 24 should be due to the presence of base, so that the reaction was repeated without base to obtain in moderate yield only the o-quinone derivatives 25. Both regioisomers were separated by CC and their spectroscopic characterization was done on the basis of two-dimensional COSY and HMBC NMR experiments (see ESI†). Representative correlations observed between aromatic hydrogens and some carbonyl and quaternary carbons are shown in Fig. 3. These correlations served to unequivocally assign the structures of 25a and 25b. The presence of the 1,2-quinone system was also demonstrated through its reaction with 4,5-dimethyl-o-phenylenediamine to give compounds 26a and 26b. In order to achieve the formation of 1,2-quinones with six-membered fused-heterocyclic rings, we used aliphatic and aromatic 1,2-diamines in the addition and substitution reactions.
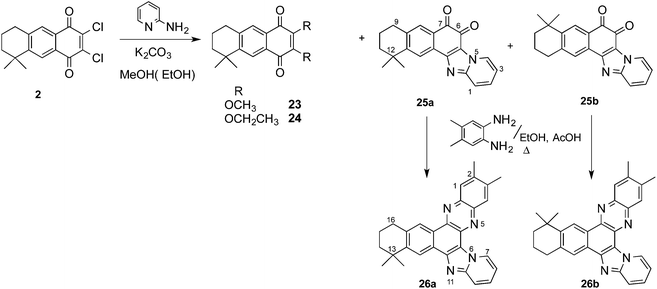 |
| Scheme 7 Preparation of 1,2-AQ derivatives 25a/b and extended condensation products. | |
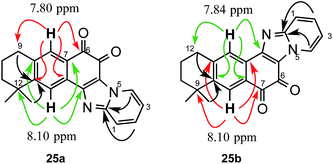 |
| Fig. 3 Selected long-range H/C connectivities for the heterofused ortho-quinones 25a and 25b. | |
When ethylenediamine was added to the AQ 1 in short times (10 min) only the addition products 27 were detected (Scheme 8); however with longer reaction times (30 min) or under microwave irradiation, mixtures of the addition products 27 and the further condensation/aromatization products 28 were obtained, giving rise to a polycyclic naphtho[2,3-a]quinoxaline system in low yield. The same quinoxaline arrangement was formed when dichloroquinone 2 was treated with ethylenediamines. In these cases, the compounds first formed were the substitution products of one chlorine atom, 30 or 31, followed by the condensation of the second amine group with one of the carbonyl groups of the quinone system, leading to compounds 32 and 33. The presence of the phenolic hydroxyl group was confirmed by acetylation to obtain the acetates 34 and 35. A similar result was attained when 4,5-dimethyl-o-phenylenediamine was used as the nucleophile, and only the naphthophenazines 36 were obtained after acetylation of the reaction product.
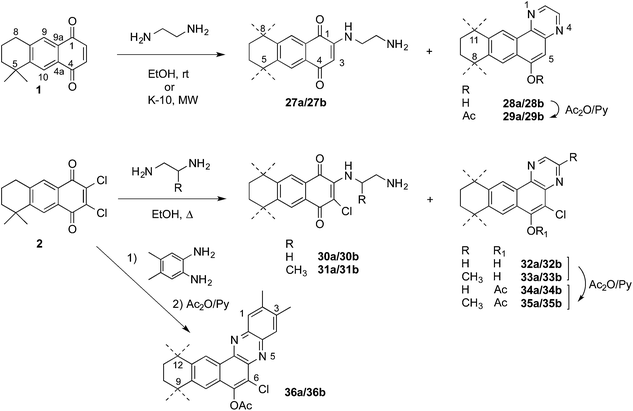 |
| Scheme 8 Preparation of angular naphthoquinoxaline and naphthophenazine derivatives. | |
Finally, linear naphthophenazines retaining the p-quinone system were prepared in two steps by reaction of the dichloroquinone 2 with arylamines to obtain the mononitrogenated quinones 37–39, in very good yields,21 from which further reaction with sodium azide afforded the expected naphthophenazinediones 40–43 in moderate to low yields (Scheme 9).
 |
| Scheme 9 Preparation of linear naphthophenazinequinones. | |
2.2. Antineoplastic cytotoxicity
Most of the compounds prepared were evaluated in vitro to establish their cytotoxicity against the following tumour cell lines: A-549 lung carcinoma, HT-29 colon adenocarcinoma and SK-BR3 breast carcinoma using a colorimetric cell growth inhibition assay.40 The results are shown in Table 1, expressed as GI50 values (drug concentration in μM causing 50% growth inhibition). The common anticancer quinone drug doxorubicin was included in the assays as reference. Most of the compounds tested were cytotoxic and from the results obtained, some general observations can be made for each class of heterocycles in comparison with the parent compounds 1 and 2.
Table 1 Cytotoxicity data (GI50 μM)a for AQ and AQ-heterofused derivatives
|
Compound |
A-549 |
HT-29 |
SK-BR3 |
GI50 values are expressed as the mean from triplicate determinations on A-549 lung carcinoma, HT-29 colon adenocarcinoma and SK-BR3 breast carcinoma. Values under 1 μM are bold-faced to facilitate comparisons. |
AQs without fused-heterocycle |
1 |
>12.5 |
9.16 |
9.95 |
2 |
— |
9.64 |
6.27 |
3a |
>10.9 |
>10.9 |
>10.9 |
4a |
6.28 |
1.64 |
9.88 |
4b |
6.81 |
2.07 |
>10.6 |
5a |
0.17 |
0.15 |
5.70 |
5b |
8.31 |
0.14 |
>8.30 |
6a/6b |
4.52 |
0.19 |
>8.64 |
7a/7b |
>7.7 |
1.09 |
0.38 |
8a/8b |
5.72 |
0.18 |
>7.12 |
12a |
2.98 |
3.92 |
2.47 |
12b |
4.82 |
5.42 |
4.22 |
13a |
4.55 |
7.22 |
3.74 |
15a/15b |
6.09 |
9.39 |
3.81 |
16a |
>29.4 |
28.2 |
>29.4 |
16b |
>29.4 |
29.1 |
13.2 |
17a |
15.3 |
11.5 |
10.5 |
19a/19b |
>17.1 |
>17.1 |
>17.1 |
30a/30b |
2.79 |
3.71 |
>30.1 |
37a/37b |
4.04 |
3.54 |
3.03 |
38a/38b |
10.1 |
19.7 |
9.39 |
39a/39b |
12.7 |
21.6 |
11.4 |
AQs with linear fused-heterocycle |
9a |
14.0 |
11.8 |
15.7 |
9b |
>27.2 |
12.1 |
17.1 |
10a |
>26.4 |
11.6 |
>26.4 |
10b |
>26.4 |
>26.4 |
17.1 |
14a |
1.79 |
5.76 |
1.93 |
14b |
1.93 |
6.09 |
1.93 |
20a |
8.68 |
11.5 |
52.7 |
20b |
12.1 |
13.0 |
14.9 |
21a |
9.24 |
10.2 |
9.74 |
21b |
23.0 |
>25 |
12.2 |
40a/40b |
5.10 |
4.83 |
4.83 |
42a/42b |
>27 |
>27 |
>27 |
43 |
>27 |
>27 |
15.7 |
AQs with angular fused-heterocycles |
25a |
1.06 |
12.4 |
19.4 |
25b |
8.17 |
>30.3 |
>30.3 |
26a |
>23.2 |
16.3 |
>23.2 |
26b |
>23.2 |
>23.2 |
>23.2 |
32a/32b |
13.1 |
13.1 |
13.1 |
34a/34b |
11.3 |
9.02 |
13.5 |
35a/35b |
>27.2 |
>27.2 |
>27.2 |
36a/36b |
>23.1 |
>23.1 |
>23.1 |
|
Doxorubicin |
0.07 |
0.10 |
0.09 |
If we considered the 1,4-AQ derivatives without any fused-heterocycle at the quinone ring (Table 1), the presence of an alkyl/aryl/acylamino groups at position C-2 with or without a chlorine atom at C-3, in general improved the cytotoxicity in comparison with the unsubstituted AQ 1 as we have already described for similar 1,4-NQs.27b
The better cytotoxicity results, on one or more tumour cell lines tested, were found for those derivatives bearing an arylamino group at C-2, AQs 5, 6, 7 and 8, which showed GI50 values under de μM level (bold-faced in Table 1), very close to those of doxorubicin. When the quinone ring was disubstituted at C-2 and C-3 with an alkyl/arylamino group and a chlorine atom, the cytotoxicity slightly decreased with respect to those monosubstituted (37 vs. 5), although they were more cytotoxic than the unsubstituted 1, keeping the GI50 values in the μM range. Additionally, the simultaneous presence of an alkyl/arylamino and an acylamino groups at neighbouring positions led to a decrease in cytotoxicity, as in AQs 16, 17 and 19.
Some of these derivatives were several times more potent on the HT-29 cell line than on the other cell lines tested, thus indicating a certain degree of selectivity, which is always an objective in the design of better drugs. When possible, the regioisomers were tested separately, but no significant differences were observed between them, except on the A-549 cell line for AQ 5, whose 5a isomer was nearly fifty times more potent than the 5b.
Those analogues with a heterocycle fused to the quinone ring at positions C-2/C-3 (linearly fused-heterocycle, Table 1) showed from moderate to poor cytotoxicity values, with a certain dependence on the ring size and on the number and type of heteroatoms present. Those with pyrrole- or imidazole-fused rings were cytotoxic in the same level than the parent compound 1, giving slightly better results with the imidazole-fused than with the pyrrole-fused rings. However, the presence of two different heteroatoms, as in compound 14, which bear an oxazole ring, led to a potency increase in comparison with the parent AQ 1.
Considering the six-membered fused rings, the nature of the substituent present in the additional aromatic rings seemed important for the bioactivity, and the presence of a methoxy group was much better than the presence of methyl groups (42, 43 vs. 40).
Heterocycles fused at C-1/C-2 positions of the quinone ring (angularly fused-heterocycle, Table 1) did not improve at all the cytotoxicity, and only compound 25a, which contains an o-quinone system, showed better cytotoxicity, though only against the A-549 cell line, as compared with the imidazole linearly-fused 1,4-quinones 20 and 21, being worth to notice the difference in potency observed for both regioisomers 25a and 25b. The other analogues in this group did not contain the 1,2-quinone system and this could explain their poor cytotoxicity. Again, the presence of methyl groups on the added heterocyclic system led to lesser potent compounds.
2.3. Antifungal activity
Recently we have described the antifungal activity of several 1,4-NQs and 1,4-AQs related to those reported here.23 Continuing this line of research, and in order to analyse the influence of the fused-heterocycles on the antifungal activity of 1,4-AQs, several representative compounds obtained in this work were also evaluated against Candida spp., Aspergillus spp., dermatophytes, and Fusarium oxysporum. The minimum inhibitory concentration 90 (MIC90), was determined following the standard microdilutions method AFST-EUCAST and CLSI M38-A,41,42 with some modifications, for yeast and filamentous fungi, respectively. Minimum inhibitory concentrations 90 were expressed as the Geometric mean (GM-MIC90). Only those derivatives that showed MIC values lower than 32.0 μg mL−1 (∼100 μM) against one or more strains were considered active. The selected results are shown in Table 2. Three standard drugs, itraconazole (ITZ), amphotericin B (AMB) and terbinafine (TRB), were used as reference drugs to cover the fungal spectrum. Complete evaluation data for all the compounds tested are given as ESI.† Also cytotoxicity was evaluated in Vero cells (African green monkey kidney-Cercopithecus aethiops ATCC: CCL 81 line) and the cytotoxic concentrations 50% (IC50) were calculated and included in Tables 2 and 3.
Table 2 In vitro susceptibilities of Candida spp. and filamentous fungi (GM-MIC90, μg mL−1) and Vero cell cytotoxicity (IC50, μg mL−1) for selected AQs and heterofused derivativesa
Comp |
Cp |
Ck |
Cl |
Ct1 |
Ct2 |
Ca1 |
Ca2 |
HSI (C. spp.) |
Afu |
Afl |
At |
An |
Fo |
HSI (A. spp.) |
Tm |
Tr |
HSI (T. spp.) |
Vero |
Minimum inhibitory concentrations 90 (MICs90) values are expressed as the geometric mean (GM). Cytotoxic Concentrations 50% (IC50. Vero cells) found in triplicate experiments for each compound. Cp: Candida parapsilosis; Ck: C. krusei; Cl: C. lusitaniae; Ct1 and Ct2: C. tropicalis; Ca1 and Ca2: C. albicans; Afu: Aspergillus fumigatus; Afl: A. flavus; At: A. terreus; An: A. niger; Fo: Fusarium oxysporum; Tm: Trycophyton mentagrophytes; Tr: T. rubrum. Vero cells (African green monkey kidney-Cercopithecus aethiops). na: not applicable. ITZ: itraconzole; AMB: amphotericin B; TRB: terbinafine. HSI: Highest Selectivity Index (IC50 Vero/lowest MIC90 found for each compound) rounded values. MIC values under 10 μg mL−1 and HSI over 8 are bold-faced to facilitate comparisons. Complete table with MIC values ± SD are given in ESI and for fungal strain characterizations, see experimental part. |
1 |
4.8 |
4.0 |
5.0 |
12.7 |
5.0 |
18.0 |
22.6 |
2.2 (Ck) |
>32 |
>32 |
>32 |
>32 |
>32 |
na |
1.3 |
4.0 |
6.6 (Tm) |
8.6 |
2 |
2.0 |
1.0 |
2.0 |
>32 |
5.0 |
>32 |
8.0 |
42.2 (Ck) |
8.0 |
4.8 |
>32 |
>32 |
>32 |
8.8 (Afl) |
6.1 |
8.0 |
6.9 (Tm) |
42.2 |
3a |
4.0 |
2.0 |
5.0 |
12.7 |
8.0 |
20.2 |
16.0 |
54.5 (Ck) |
16 |
>32 |
>32 |
>32 |
16 |
6.8 (Afu) |
3.2 |
5.0 |
34.1 (Tm) |
109 |
4b |
>32 |
>32 |
>32 |
>32 |
>32 |
>32 |
>32 |
na |
>32 |
>32 |
>32 |
>32 |
>32 |
na |
5.0 |
3.2 |
11.1 (Tr) |
35.6 |
12a |
8.0 |
2.8 |
2.2 |
6.4 |
4.0 |
4.0 |
8.0 |
10.1 (Cl) |
22.6 |
>32 |
>32 |
>32 |
32 |
0.9 (Afu) |
3.2 |
5.0 |
7.0 (Tm) |
22.3 |
14 |
6.7 |
19.0 |
20.2 |
>32 |
32 |
>32 |
>32 |
0.7 (Cp) |
16 |
>32 |
>32 |
>32 |
>32 |
0.3 (Afu) |
32 |
8.0 |
0.6 (Tr) |
4.6 |
38 |
>32 |
>32 |
>32 |
>32 |
>32 |
>32 |
>32 |
na |
>32 |
>32 |
>32 |
>32 |
>32 |
na |
25.4 |
25.4 |
2.0 |
50.5 |
ITZ |
0.25 |
0.5 |
|
|
|
|
|
>100 (Ck) |
|
|
|
|
|
|
|
|
|
>50 |
AMB |
|
|
|
|
|
|
|
|
2.0 |
4.0 |
|
|
|
7.1 (Afl) |
|
|
|
28.6 |
TRB |
|
|
|
|
|
|
|
|
|
|
|
|
|
|
0.03 |
0.08 |
386 (Tr) |
30.9 |
Table 3 Antiviral activity of selected AQ derivatives
Compound |
Vero ATCC CCL-81a |
Vero cells |
1 TCID50b HHV-1 |
10 TCID50c HHV-2 |
(Rf)d |
Antiviral activitye (μg mL−1) |
SIf |
(Rf)d |
Antiviral activitye (μg mL−1) |
SIf |
IC50 (μg mL−1) |
Vero Cercopithecus aethiops African green monkey kidney cell line ATCC CCL 81. 1TCID50: 1 cell culture infectious dose fifty percent. 10TCID50: 10 cell Culture infectious dose fifty percent. Rf: value obtained by dividing the viral titer in the absence of the compound on the titer obtained in presence of the compound. Maximum non-toxic concentration of compound which had the highest factor of reduction of viral titer. SI: selectivity index (IC50 Vero/TCID50); nd: not determined. |
1 |
100.5 |
6.25 |
1.37 |
nd |
>50 |
nd |
8.6 |
2 |
100.5 |
50 |
0.85 |
102 |
25 |
1.7 |
42.2 |
3a |
102 |
6.25 |
17.5 |
nd |
>50 |
nd |
109.3 |
10a |
nd |
>50 |
nd |
101 |
50 |
1.39 |
69.5 |
12a |
nd |
>50 |
nd |
101 |
12.5 |
1.78 |
22.3 |
14 |
nd |
>50 |
nd |
101 |
25 |
0.18 |
4.6 |
37a/b |
101 |
50 |
1.42 |
nd |
>50 |
nd |
71.0 |
38a/b |
101 |
50 |
1.01 |
nd |
>50 |
nd |
50.5 |
39a/b |
101 |
50 |
≤0.5 |
nd |
>50 |
nd |
≤25 |
43 |
nd |
>50 |
nd |
101 |
50 |
0.33 |
16.4 |
Dextran sulphate |
102 |
0.5 |
nd |
102 |
0.5 |
nd |
|
Acyclovir |
104 |
1.5 |
nd |
104 |
1.5 |
nd |
|
Seven of seventeen evaluated compounds were actives against Trichophyton spp. while five compounds showed activity against Candida spp. and three against A. fumigatus. The other Aspergillus spp. and F. oxysporum were less susceptible. Only compound 2 resulted active against A. flavus and 3a and 12a against F. oxysporum. These two later compounds showed fairly wide antifungal spectra. The most potent compounds against Candida spp. were 1, 2, 3a, 12a and 14. The AQ 12a was the best against both tested strains of C. albicans. This yeast is the most important in infections caused by fungi. AQ 12a was also active against other pathogenic yeasts like C. parapsilosis, C. krusei, C. lusitaniae and C. tropicalis with GM-MIC90 values from 2.2 to 8.0 μg mL−1.
Compound 2 showed important activity against C. parapsilosis, C. krusei and C. lusitaniae. These results are important considering the intrinsic resistance to fluconazole and echinocandin of C. krusei and C. parapsilosis, respectively and also because C. lusitaniae has the ability to rapidly develop resistance to AMB.34 This antifungal is the most important available agent for treating candidiasis and actually the only antifungal that has a broad spectrum of activity.
AQs 2, 3a and 12a and oxazole 14, showed moderate activity against Aspergillus fumigatus. The compound 2 was the most active against this filamentous fungus and also was active against A. flavus. None of the tested compounds were active against A. terreus and A. niger. A. terreus is intrinsically resistant to AMB and less susceptible to other antifungals43 for this reason it would be important to find new active molecules against this fungus.
The AQ 3a, in addition to be fairly active against Candida spp. and A. fumigatus, showed moderate activity against F. oxysporum. This result is relevant because, compared with other filamentous fungi, Fusarium species are intrinsically resistant to ITZ and echinocandins and the response to AMB and voriconazole is variable depending on the particular species.44
All compounds listed in Table 2 were active against both dermatophytes strains. AQs 3a, 4 and 12a, showed similar good activity against both Trichophyton species. AQ 1 was the most active against T. rubrum and T. mentagrophytes. This compound may be of interest as a lead compound for further studies in order to develop new molecules with activity against this group of fungi.
According to this data and the previous results with similar NQs,23 we can conclude that, in general, the precursor 1,4-AQs showed better antifungal activity than their heterofused derivatives. Only the oxazole-fused compound 14 was active against several strains, although its selectivity was poor. The MIC value for compound 14 against C. parapsilosis (MIC90 = 6.7 μg mL−1) was approximately three times lower than for the other Candida species. These data are important because C. parapsilosis exhibits a consistent trend of decreasing fluconazole susceptibility over the treatment time.45 AQ 14 also showed activity against A. fumigatus, but not against other Aspergillus spp. or F. oxysporum. However, it was also active against both Trycophyton species.
The viability of the Vero cell line in the presence of the seven 1,4-AQ derivatives selected was evaluated and the corresponding cytotoxicity calculated (Tables 2 and 3). All the tested compounds produced a dose-dependent inhibition on the Vero cell line, with linear regression coefficients higher than 0.7 (ESI†). According to the results obtained, the compounds reported here should be considered as low cytotoxic for normal monkey cells and the highest selectivity index (HSI = IC50 for Vero cells/lowest GM-MIC90 value of a compound against any pathogenic fungus) was calculated in order to quantify their respective selectivity and to facilitate comparisons.
According to literature, SI values higher than 8 are considered indicative of a potential therapeutic agent that merit further attention.46 To this respect, AQs 2, 3a and 12a were the most selective against Candida spp. (HSI = 10.1–54.5), AQ 2 was also selective against A. flavus and compounds 3a and 4b were selective against dermatophytes. At this point, it is important to note that HSI values should be higher than those calculated and included in Table 2 if we have in mind that MIC values refer to nearly total (90–100%) fungal growth inhibition and IC50 values refer to the inhibition of only 50% of Vero cells.
2.4. Antiviral activity
It has been reported that the most effective drugs against herpetic infections, acyclovir and its related prodrugs and analogues, have developed a number of drug-resistant strains after prolonged or inadequate use.33,47 Consequently, the search for new therapeutic agents, with non-related structures and different mechanisms of action, seems necessary to maintain efficacious treatments, and can justify the antiviral evaluation of some representative AQs synthesised in this work.
The antiviral activity was evaluated against the 50% Tissue-Culture Infective Dose, 1TCID50 and 10TCID50, of Human Herpes virus 1 (HHV-1 CDC Atlanta acyclovir-sensitive strain) and Human Herpes virus 2 (HHV-2 VR-734-G acyclovir-sensitive strain) respectively, by determining the reduction factor (Rf), which corresponds to the value obtained by dividing the viral titer in the absence of compound over the viral titer obtained in the presence of compound.48 Two positive controls, dextran sulfate and acyclovir, were included in this assay. Dextran sulfate was included because this sulfated polysaccharide can inhibit viral entry by a competitive effect with heparan sulfate receptors present on the cell surface. In addition, acyclovir was included because is effective in the inhibition of viral DNA polymerase. Compounds that showed reduction of viral titer respect to control (Rf) at concentrations lower or equal than 50 μg mL−1, were considered active and are collected in Table 3. Complete evaluation data for all the compounds tested are given as ESI.†
In this study, compounds that showed mild activity (Rf ≤ 1 × 101) against HHV-1 at a concentration of 50 μg mL−1 were 2, 37, 38 and 39, while AQ 1 attained the same Rf at a concentration of 6.25 μg mL−1. Notably, AQ 3a showed better anti HHV-1 activity (Rf = 1 × 102) at a concentration of 6.25 μg mL−1. The AQ 10a and the naphthophenazine 43 showed mild activity against HHV-2 at a concentration of 50 μg mL−1. Moreover, compounds 12a and 14 were active at a concentration of 12.5 and 25 μg mL−1, respectively. Only AQ 2 showed moderate anti HHV-2 activity (Rf = 1 × 102) at a concentration of 25 μg mL−1.
In our study, the parameter established to define antiviral selectivity was the selectivity index (SI) value. SI is defined as the relative effectiveness of a product to inhibiting viral replication compared to the capacity for inducing cell death. It is desirable to have a high therapeutic index (SI ≥ 10) giving maximum antiviral activity with minimal cell toxicity.48 The antiviral SI for each active compound was calculated and listed in Table 3. Our results showed that only compound 3a showed a high antiviral SI (17.5) against HHV-1 strain. This value could be considered indicative of a potential lead compound for further antiviral studies.49
3. Conclusions
As a global consideration, the best biological results were principally found for 1,4-AQs and their substituted derivatives rather than for their heterocycle-fused analogues. Among the 1,4-AQs, those bearing an arylamino group at C-2 were the most cytotoxic against the tumour cell lines tested, while the presence of a chlorine atom at the quinone moiety seemed to improve the antifungal and antiviral activities. Though the heterofused AQs were generally less potent than their corresponding AQ precursors and intermediates, the oxazole-fused compound 14 showed positive results on the three biological tests.
The relevance of the antifungal activity varies with the pathogenicity of the fungus. In general, filamentous fungi are more resistant than yeasts to antifungal agents. Then, the discovery of AQ 2, active against A. flavus and A. fumigatus is considered enough relevant. Furthermore, the finding of several molecules (AQs 1, 2, 3a, and 12a and the oxazole-fused AQ 14), active against yeasts as C. parapsilosis and C. krusei, with lower susceptibility to one or several standard antifungal drugs, could support further studies to establish their mechanism(s) of action.
Finally, among the compounds tested as antiviral, the chlorinated 1,4-AQ 3a, as an active compound against HHV-1 with the highest selectivity index, could be considered as a lead compound for further research.
At the current level of our research, we cannot formulate consistent proposals for possible mechanisms or targets to the new type of compounds, with novel structural arrangements directly attached to the quinone ring. Further experimental research will be planned with such objectives.
4. Experimental section
4.1. Chemistry
NMR spectra were recorded on a Bruker AC 200 at 200 MHz for 1H and 50.3 MHz for 13C in deuterochloroform with TMS as internal standard. Chemical shift (δ) values are expressed in ppm followed by multiplicity and coupling constants (J) in Hz. IR spectra were obtained on a Nicolet Impact 410 spectrophotometer in NaCl film, and wavenumbers are given in cm−1. UV spectra were determined on a Hitachi 100-60 spectrophotometer in CHCl3 solution, and λmax are given in nm. HRMS were run in a VG-TS-250 spectrometer working at 70 eV. Column chromatography (CC) was performed on silica gel (Merck no. 9385) and TLC was carried out on silica gel 60 F245 (Merck, 0.25 mm thick). Solvents and reagents were purified by standard procedures as necessary.
1H NMR data are given for the new compounds described here and complete 1H and 13C NMR assignments are included in the ESI.†
Anthracene-1,4-diones 1–8, 12, 24, and 37–39 were obtained by means of previously described procedures.21,23 Other compounds were prepared as follows.
4.1.1. 3-Acetyl-1-ethyl-2,6,6-trimethyl-1H-6,7,8,9-tetrahydronaphtho[2,3-f]indole-4,11-dione 9a. To a solution of 4a (22 mg, 0.08 mmol) and 2,4-pentanedione (32 μL, 0.29 mmol) in MeOH (1 mL) and dichloromethane (0.2 mL) stirred at rt was added CAN (154 mg, 0.28 mmol) in four portions at 10 min intervals. The reaction mixture was stirred for another 30 min and then diluted with EtOAc, washed with sat aq sodium bicarbonate and brine, dried over Na2SO4 and vacuum-evaporated to yield a product, which was purified by column chromatography (eluent: Hex/EtOAc 8
:
2) affording 9a (12 mg, 43%): mp 128–132 °C (from CH2Cl2); UV λmax(log
ε): 270 (4.6), 297 (4.2), 388 (3.9); IR νmax (film): 1657, 1596, 1495, 1463, 1265, 1026 cm−1; 1H NMR (CDCl3) δ: 1.34 (6H, s), 1.39 (3H, t, J = 7.1), 1.70–1.82 (4H, m), 2.42 (3H, s), 2.72 (3H, s), 2.88 (2H, t, J = 6.3), 4.52 (2H, m), 7.78 (1H, s), 8.09 (1H, s); HRMS (ES, M + Na) calcd: 386.1726, found: 386.1736.
4.1.2. 3-Acetyl-1-ethyl-2,9,9-trimethyl-1H-6,7,8,9-tetrahydronaphtho[2,3-f]indole-4,11-dione 9b. Following the above procedure, treatment of 4b and 2,4-pentanedione with CAN, gave 9b (47%): mp 137–140 °C (from CH2Cl2); IR νmax (film): 1660, 1596, 1495, 1465, 1265, 1017 cm−1; 1H NMR (CDCl3) δ: 1.35 (6H, s), 1.41 (3H, t, J = 7.1), 1.71–1.83 (4H, m), 2.43 (3H, s), 2.70 (3H, s), 2.88 (2H, t, J = 6.3), 4.52 (2H, m), 7.79 (1H, s), 8.09 (1H, s); HRMS (ES, M + Na) calcd: 386.1726, found: 386.1734.
4.1.3. Methyl 1-ethyl-2,6,6-trimethyl-4,11-dioxo-1H-6,7,8,9-tetrahydronaphtho[2,3-f]indole-3-carboxylate 10a. Following the above procedure, treatment of 4a and methyl acetylacetate with CAN, gave 10a (40%): mp 160–163 °C (from CH2Cl2); IR νmax (film): 1711, 1660, 1597, 1464, 1263, 1018 cm−1; 1H NMR (CDCl3) δ: 1.32 (6H, s), 1.40 (3H, t, J = 7.1), 1.69–1.81 (4H, m), 2.48 (3H, s), 2.86 (2H, t, J = 6.2), 3.95 (3H, s), 4.52 (2H, m), 7.78 (1H, s), 8.07 (1H, s); HRMS (ES, M + Na) calcd: 402.1675, found: 402.1691.
4.1.4. Methyl 1-ethyl-2,9,9-trimethyl-4,11-dioxo-1H-6,7,8,9-tetrahydronaphtho[2,3-f]indole-3-carboxylate 10b. Following the above procedure, treatment of 4b and methyl acetylacetate with CAN, gave 10b (22%): mp 150–154 °C (from CH2Cl2); IR νmax (film): 1710, 1668, 1597, 1465, 1270, 1108 cm−1; 1H NMR (CDCl3) δ: 1.33 (6H, s), 1.39 (3H, t, J = 7.1), 1.72–1.82 (4H, m), 2.48 (3H, s), 2.87 (2H, t, J = 6.0), 3.97 (3H, s), 4.52 (2H, m), 7.77 (1H, s), 8.11 (1H, s).
4.1.5. 3-Amino-2-chloro-5,5(8,8)-dimethyl-5,6,7,8-tetrahydroanthracene-1,4-diones 11a(11b). To a solution of AQ 2 (1.82 mmol) in EtOH (30 mL), 30% NH4OH (17 mL) was added dropwise and kept stirring at room temperature for 1 h. Then, the solvent was vacuum-evaporated and the residue was redissolved in ethyl acetate. The organic layer was washed with 2 N HCl and brine, dried over Na2SO4, filtered and evaporated till dryness, affording a 1
:
1 mixture of 11a/11b, that was used in the next steps without purification.Treatment of 3a (0.71 mmol) with 30% NH4OH (7 mL), following the above procedure, afforded 11a.
4.1.6. 3-Acetamido-2-chloro-5,5(8,8)-dimethyl-5,6,7,8-tetrahydroanthracene-1,4-diones 12a(12b). AQ 11a/11b (0.36 mmol) were acetylated at 0 °C with Ac2O–H2SO4 (6 mL taken from a previously solution prepared with 50 mL of Ac2O and 0.15 mL of H2SO4) for 30 min. The reaction was quenched with ice and extracted with EtOAc. The organic layer was washed with satd aq NaHCO3, and brine, dried over anhydrous Na2SO4 and vacuum-evaporated yielding a crude product, which was purified by column chromatography (eluent: Hex/EtOAc 8
:
2) to afford 12a/12b.23
4.1.7. 2-Chloro-3-diacetylamino-5,5(8,8)-dimethyl-5,6,7,8-tetrahydroanthracene-1,4-diones 13a(13b). AQ 11a/11b (1.73 mmol) were acetylated at rt with Ac2O–H2SO4 (31 mL taken from a solution previously prepared with 50 mL of Ac2O and 0.15 mL of H2SO4) for 1 h. The reaction was worked up as described above to afford 13a/13b (80%): IR νmax (film): 1670, 1599, 1493, 1288, 1253, 1048 cm−1; 1H NMR (CDCl3) δ: 1.34 (6H, s), 1.71–1.86 (4H, m), 2.28/2.36 (6H, s), 2.90/2.91 (2H, t, J = 6.2), 7.83/8.17 (1H, s), 8.04/7.82 (1H, s); HRMS (ES, M + Na) calcd: 396.0973, found: 396.0986.Treatment of 11a with Ac2O–H2SO4, in the same way described above yielded 13a (96%).
4.1.8. 2-Chloro-3-dibenzoylamino-5,5(8,8)-dimethyl-5,6,7,8-tetrahydroanthracene-1,4-diones 15a(15b). AQ 11a/11b (0.83 mmol) and NaH (1.8 mmol) were stirred at room temperature until compound 12 disappeared by TLC control. Then, benzoyl chloride (1.23 mmol) was added and stirred for another 5 min. The reaction mixture was quenched with water and extracted with EtOAc. The organic layer was washed with satd aq NaHCO3, and brine, dried over anhydrous Na2SO4 and vacuum-evaporated yielding a crude product, which was purified by column chromatography (eluent: Hex/EtOAc 8
:
2) to afford AQs 15a/15b (30%): 1H NMR (CDCl3) δ: 1.30/1.33 (6H, s), 1.71–1.81 (4H, m), 2.83–2.93 (2H, m), 7.27–7.47 (6H, m), 7.73–7.77 (4H, m), 7.84/8.12 (1H, s), 8.07/7.78 (1H, s); HRMS (ES, M + Na) calcd: 520.1286, found: 520.1277.
4.1.9. General procedure for the nucleophilic substitution to obtain compounds 16–19. AQ 12, 13 or 15 and the corresponding amine in EtOH were stirred at reflux for a specify time. Then, the solvent was vacuum-evaporated and the residue was redissolved in ethyl acetate. The organic layer was washed with 2 N HCl and brine, dried over Na2SO4, filtered and evaporated till dryness, affording a crude product, which was purified through column chromatography over silica gel.
4.1.10. 3-Acetamido-2-ethylamino-5,5(8,8)-dimethyl-5,6,7,8-tetrahydroanthracene-1,4-diones 16a(16b). Following the above procedure, treatment of 13a/13b (0.33 mmol) with ethylamine (0.8 mmol) for 45 min, gave out, after preparative TLC (eluent EtOAc): (a) 15 mg (13%) of 16a: mp 160–165 °C (from MeOH); IR νmax (film): 3300, 1674, 1594, 1558, 1505, 1341, 1257, 1013, 809 cm−1; 1H NMR (CDCl3) δ: 1.24 (2H, t, J = 7.2), 1.31 (6H, s), 1.69–1.80 (4H, m), 2.23 (3H, s), 2.83 (2H, t, J = 6.1), 3.45 (2H, m), 7.68 (1H, s), 7.99 (1H, s); HRMS (ES, M + Na) calcd: 363.1679, found: 363.1689. (b) 9 mg (8%) of 16a/16b. (c) 16 mg (14%) of 16b: mp 154–159 °C (from MeOH); IR νmax (film): 3283, 1673, 1600, 1595, 1515, 1341, 1300, 1005, 919 cm−1; 1H NMR (CDCl3) δ: 1.24 (2H, t, J = 7.0), 1.31 (6H, s), 1.66–1.80 (4H, m), 2.22 (3H, s), 2.85 (2H, t, J = 6.1), 3.45 (2H, m), 7.69 (1H, s), 7.97 (1H, s); HRMS (ES, M + Na) calcd: 363.1679, found: 363.1666.Following the same procedure, treatment of 13a (0.7 mmol) with ethylamine (0.7 mmol) afforded 16a (48%) after CC.
4.1.11. 3-Acetamido-2-(p-methoxyanilino)-5,5(8,8)-dimethyl-5,6,7,8-tetrahydroanthracene-1,4-diones 17a(17b). Following the above procedure, treatment of 13a/13b (0.2 mmol) with p-anisidine (0.5 mmol) for 20 h at rt, gave out, after two consecutive preparative TLC (eluents Hex/EtOAc 3
:
7 and benzene/methanol 9
:
1): (a) 3 mg (4%) of 17a: 1H NMR (CDCl3) δ: 1.33 (6H, s), 1.62 (3H, s), 1.67–1.85 (4H, m), 2.87 (2H, t, J = 6.4), 3.80 (3H, s), 6.81 (2H, d, J = 9.1), 6.89 (2H, d, J = 9.1), 7.75 (1H, s), 8.02 (1H, s). (b) 30 mg of 17a/17b impurified with N-acetylanisidine. (c) 7 mg (9%) of 17b: 1H NMR (CDCl3) δ: 1.34 (6H, s), 1.61 (3H, s), 1.67–1.85 (4H, m), 2.88 (2H, t, J = 6.4), 3.80 (3H, s), 6.80 (2H, d, J = 9.0), 6.90 (2H, d, J = 9.0), 7.76 (1H, s), 8.04 (1H, s).Treatment of 12a/12b (0.08 mmol) with p-anisidine (0.11 mmol) following the general procedure for 1.5 h, afforded the mixture 17a/17b in quantitative yield.
Treatment of 13a (0.3 mmol) with p-anisidine (0.7 mmol) following the same procedure, afforded 17a impurified with N-acetylanisidine.
4.1.12. 3-Benzamido-2-(p-methoxyanilino)-5,5(8,8)-dimethyl-5,6,7,8-tetrahydroanthracene-1,4-diones 18a(18b) and 3-dibenzoylamino-2-(p-methoxyanilino)-5,5(8,8)-dimethyl-5,6,7,8-tetrahydro anthracene-1,4-diones 19a(19b). Following the above procedure, treatment of 15a/15b (0.26 mmol) with p-anisidine (0.56 mmol) for 1 h, gave out, after column chromatography (eluent: toluene/EtOAc 8
:
2): (a) 29 mg of 19a/19b with N-acetylanisidine, which were submitted to PTLC (eluent: benzene/EtOAc 8
:
2) to yield 14 mg (11%) of 19a/19b: IR νmax (film): 3346, 1664, 1599, 1508, 1474, 1334, 1249, 1029 cm−1; 1H NMR (CDCl3) δ: 1.30/1.33 (6H, s), 1.67–1.82 (4H, m), 2.86 (2H, m), 3.66 (3H, s), 6.56 (2H, d, J = 8.8), 6.89 (2H, d, J = 8.8), 7.21–7.76 (10H, m), 7.76 (1H, s), 8.03/8.04 (1H, s); HRMS (ES, M + Na) calcd: 607.2203, found: 607.2200. (b) 19 mg of 18a/18b (19%): IR νmax (film): 3300, 1667, 1596, 1515, 1472, 1343, 1284, 1031 cm−1; 1H NMR (CDCl3) δ: 1.30/1.33 (6H, s), 1.68–1.83 (4H, m), 2.88 (2H, t, J = 6.2), 3.59 (3H, s), 6.64 (2H, d, J = 8.7), 6.91 (2H, d, J = 8.7), 7.30–7.80 (5H, m), 7.75/7.77 (1H, s), 8.05/8.07 (1H, s); HRMS (ES, M + Na) calcd: 503.1941, found: 503.1924.
4.1.13. 2,6,6(2,9,9)-Trimethyl-6,7,8,9-tetrahydroanthra[2,3-d]oxazole-4,11-dione 14a(14b). Treatment of 16a/16b (0.06 mmol) with Ac2O–H2SO4 as described above for 12a/12b, yielded a reaction product that was chromatographed on PTLC (eluent Hex/EtOAc 1
:
1) to give: (a) 4 mg (24%) of 14a: mp 154–156 °C (from CH2Cl2); IR νmax (film): 1674, 1588, 1453, 1381, 1240, 1089, 1031, 803 cm−1; 1H NMR (CDCl3) δ: 1.35 (6H, s), 1.71–1.83 (4H, m), 2.63 (3H, s), 2.82 (2H, d, J = 6.2), 7.57 (1H, s), 7.81 (1H, s); HRMS (ES, M + Na) calcd: 318.1100, found: 318.1041. (b) 6 mg (36%) of 14b: mp 135–138 °C (from CH2Cl2); IR νmax (film): 1681, 1592, 1462, 1420, 1264, 1091, 1032, 736 cm−1; 1H NMR (CDCl3) δ: 1.31 (6H, s), 1.67–1.86 (4H, m), 2.61 (3H, s), 2.82 (2H, m), 7.32 (1H, s), 8.10 (1H, s); HRMS (ES, M + Na) calcd: 318.1100, found: 318.1096.Treatment of 17a/17b (0.09 mmol) with Ac2O–H2SO4 as described above, yielded 14a/14b (40%).
Treatment of 12a/12b (0.09 mmol) with Ac2O–H2SO4 as described above, yielded a complex reaction product containing 13a/13b and 14a/14b.
4.1.14. General procedure for the preparation of compounds 20–22. The corresponding AQ was dissolved in EtOH (1–3 mL) and 4% NaOH (3–6 mL) was added dropwise and kept under reflux for 30 min. Then, the organic solvent was evaporated off, the residue was redissolved in EtOAc, washed with 2 N HCl and brine, and dried over Na2SO4 to give the corresponding imidazole.
4.1.15. 1-Ethyl-2,6,6-trimehyl-6,7,8,9-tetrahydro-1H-anthra[2,3-d]imidazole-4,11-dione 20a. Following the above general procedure, treatment of 16a (0.71 mmol) with 4% NaOH (3 mL) yielded 20a (77%): mp 104–108 °C (from CH2Cl2); UV λmax(log
ε): 259 (4.5), 267 (4.6), 299 (4.1), 347 (3.4); IR νmax (film): 1665, 1594, 1517, 1473, 1436, 1291, 1243, 1172, 1091, 1012, 922 cm−1; 1H NMR (CDCl3) δ: 1.34 (6H, s), 1.43 (3H, t, J = 7.2), 1.70–1.82 (4H, m), 2.57 (3H, s), 2.88 (2H, t, J = 6.3), 4.43 (2H, m), 7.77 (1H, s), 8.17 (1H, s); HRMS (ES, M + Na) calcd: 345.1578, found: 345.1593.
4.1.16. 1-Ethyl-2,9,9-trimehyl-6,7,8,9-tetrahydro-1H-anthra[2,3-d]imidazole-4,11-dione 20b. Following the above general procedure, treatment of 16b (0.04 mmol) with 4% NaOH (3 mL) yielded 20b (63%): mp 158–161 °C (CH2Cl2); IR νmax (film): 1662, 1594, 1517, 1473, 1436, 1289, 1244, 1172, 1088, 921 cm−1; 1H NMR (CDCl3) δ: 1.34 (6H, s), 1.45 (3H, t, J = 7.1), 1.70–1.82 (4H, m), 2.56 (3H, s), 2.87 (2H, t, J = 6.3), 4.43 (2H, m), 7.89 (1H, s), 8.08 (1H, s); HRMS (ES, M + Na) calcd: 345.1578, found: 345.1562.
4.1.17. 1-(p-Methoxyphenyl)-2,6,6-trimehyl-6,7,8,9-tetrahydro-1H-anthra[2,3-d]imidazole-4,11-dione 21a. Following the above general procedure, treatment of 17a (0.03 mmol) with 4% NaOH (3 mL) yielded 21a (86%): mp 98–102 °C (from CH2Cl2); IR νmax (film): 1670, 1597, 1515, 1454, 1251, 1171, 1027, 802 cm−1; 1H NMR (CDCl3) δ: 1.34 (6H, s), 1.65–1.79 (4H, m), 2.38 (3H, s), 2.82 (2H, t, J = 6.2), 3.89 (3H, s), 7.06 (2H, d, J = 8.8), 7.24 (2H, d, J = 8.8), 7.65 (1H, s), 8.18 (1H, s); HRMS (ES, M + 1) calcd: 401.1859, found: 401.1879.
4.1.18. 1-(p-Methoxyphenyl)-2,9,9-trimethyl-6,7,8,9-tetrahydro-1H-anthra[2,3-d]imidazole-4,11-dione 21b. Following the above general procedure, treatment of 17b (0.03 mmol) with 4% NaOH (3 mL) yielded 21b (97%): mp 130–133 °C (from CH2Cl2); IR νmax (film): 1671, 1596, 1515, 1456, 1253, 1171, 1025, 800 cm−1; 1H NMR (CDCl3) δ: 1.27 (6H, s), 1.61–1.84 (4H, m), 2.39 (3H, s), 2.87 (2H, t, J = 6.3), 3.90 (3H, s), 7.07 (2H, d, J = 6.8), 7.25 (2H, d, J = 6.8), 7.91 (1H, s), 7.99 (1H, s); HRMS (ES, M + 1) calcd: 401.1859, found: 401.1869.
4.1.19. 1-(p-Methoxyphenyl)-2-phenyl-6,6(9,9)-dimethyl-6,7,8,9-tetrahydro-1H-anthra[2,3-d]imidazole-4,11-dione 22a(22b). Following the above general procedure, treatment of 18a/18b (0.04 mmol) with 4% NaOH (3 mL) yielded 22a/22b (93%): IR νmax (film): 1670, 1596, 1514, 1429, 1246, 1174, 1030, 840 cm−1; 1H NMR (CDCl3) δ: 1.28/1.36 (6H, s), 1.67–1.84 (4H, m), 2.86 (2H, m), 3.88 (3H, s), 6.96–7.59 (9H, m), 7.69/8.03 (1H, s), 8.23/7.95 (1H, s); HRMS (ES, M + Na) calcd: 485.1835, found: 485.1832.
4.1.20. 12,12(9,9)-Dimethyl-9,10,11,12-tetrahydroanthra[1′,2′:4,5]imidazo[1,2-a]pyridine-6,7-dione 25a(25b). A mixture of AQ 2 (0.65 mmol) and 2-aminopyridine (1.3 mmol) in EtOH (8 mL) was stirred under reflux for 72 h. The solvent was vacuum evaporated and the residue was redissolved in EtOAc, washed with 2 N HCl and brine, dried over Na2SO4, filtered and evaporated till dryness yielding a reaction product that was purified by column chromatography (eluent Hex/EtOAc 1
:
1) to afford: (a) 22 mg (10%) of 25a: mp 270–275 °C (from CH2Cl2): UV λmax(log
ε): 283 (4.4), 351 (3.7), 402 (3.7): IR νmax (film): 1644, 1455, 1428, 1391, 1249, 1039, 913 cm−1: 1H NMR (CDCl3) δ: 1.39 (6H, s), 1.70–1.85 (4H, m), 2.82 (2H, t, J = 6.4), 7.16 (1H, dd, J = 6.2 and 7.6), 7.60 (1H, dd, J = 7.6 and 8.8), 7.79 (1H, s), 7.80 (1H, d, J = 8.9), 8.10 (1H, s), 9.29 (1H, d, J = 6.2): HRMS (ES, M + Na) calcd: 353.1260, found: 353.1260. (b) 26 mg (12%) of 25a/25b. (c) 7 mg (3%) of 25b: mp 279–282 °C (from CH2Cl2): IR νmax (film): 1641, 1457, 1427, 1388, 1250, 1111, 1041, 769 cm−1: 1H NMR (CDCl3) δ: 1.34 (6H, s), 1.70–1.85 (4H, m), 2.89 (2H, t, J = 6.4), 7.17 (1H, dd, J = 6.3 and 7.4), 7.61 (1H, dd, J = 7.4 and 8.7), 7.78 (1H, d, J = 8.7), 7.84 (1H, s), 8.10 (1H, s), 9.29 (1H, d, J = 6.3); HRMS (ES, M + Na) calcd: 353.1260, found: 353.1275.A mixture of AQ 2 (0.43 mmol), 2-aminopyridine (0.95 mmol) and K2CO3 (64 mg) in MeOH (2 mL) was stirred under reflux for 19 h. Following the procedure described above and after column chromatography (eluent Hex/EtOAc 8
:
2 and 1
:
1) of the complex reaction product, 18 mg (14%) of 23 were obtained: IR νmax (film): 1665, 1602, 1455, 1338, 1296, 1041, 917, 734 cm−1; 1H NMR (CDCl3) δ: 1.32 (6H, s), 1.71–1.80 (4H, m), 2.86 (2H, t, J = 6.2), 4.08 (6H, s), 7.71 (1H, s), 8.00 (1H, s).
A mixture of AQ 2 (0.65 mmol), 2-aminopyridine (1.3 mmol) and K2CO3 (200 mg) in EtOH (5 mL) was stirred under reflux for 14 h. Following the procedure described above and after column chromatography (eluent Hex/EtOAc 8
:
2 and 1
:
1) of the reaction product, the following compounds were obtained: 44 mg (20%) of 24 (ref. 23) and 22 mg (10%) of 25a/25b.
4.1.21. 2,3,13,13-Tetramethyl-13,14,15,16-tetrahydronaphtho[2,3-a]pyrido[1′,2′:1,2]imidazo[4,5-c]phenazine 26a. A mixture of 25a (0.07 mmol) and glacial acetic acid (400 μL) in EtOH (1.5 mL) was stirred at rt for 30 min. Then, 4,5-dimethyl-1,2-phenylenediamine (0.07 mmol) was added and heated under reflux for 6 h. The reaction mixture was diluted with water and extracted with EtOAc. The organic phase was washed with brine, dried over Na2SO4, filtered and evaporated off to yield a reaction product, which was purified by column chromatography (eluent Hex/EtOAc 1
:
1) affording 26a (50%): mp 240–245 °C (from CH2Cl2); IR νmax (film): 1450, 1334, 1264, 1114, 1028, 735 cm−1; 1H NMR (CDCl3) δ: 1.48 (6H, s), 1.84–1.97 (4H, m), 2.46 (3H, s), 2.49 (3H, s), 3.14 (2H, t, J = 6.2), 7.10 (1H, dd, J = 6.7 and 7.0), 7.51 (1H, dd, J = 7.0 and 8.2), 7.80 (1H, s), 7.90 (1H, s), 7.94 (1H, d, J = 8.2), 8.70 (1H, s), 8.94 (1H, s), 10.12 (1H, d, J = 6.7); HRMS (ES, M + H) calcd: 431.2230, found: 431.2260.
4.1.22. 2,3,16,16-Tetramethyl-13,14,15,16-tetrahydronaphtho[2,3-a]pyrido[1′,2′:1,2]imidazo[4,5-c]phenazine 26b. A mixture of 25b (0.05 mmol) and glacial acetic acid (300 μL) in EtOH (1.5 mL) was stirred at rt for 30 min. Then, 4,5-dimethyl-1,2-phenylenediamine (0.06 mmol) was added and heated under reflux for 2 h. The reaction mixture was diluted with water and extracted with EtOAc. The organic phase was washed with brine, dried over Na2SO4, filtered and evaporated off to yield a reaction product, which was purified by column chromatography (eluent Hex/EtOAc 1
:
1) affording 26b (32%): mp 205–210 °C (from CH2Cl2); IR νmax (film): 1454, 1336, 1261, 1116, 1043, 735 cm−1; 1H NMR (CDCl3) δ: 1.57 (6H, s), 1.75–1.95 (4H, m), 2.49 (3H, s), 2.52 (3H, s), 3.11 (2H, t, J = 6.2), 7.08 (1H, dd, J = 6.7 and 7.0), 7.48 (1H, m), 7.85 (1H, d, J = 8.4), 7.85 (1H, s), 7.98 (1H, s), 8.39 (1H, s), 9.31 (1H, s), 10.13 (1H, d, J = 6.7); HRMS (ES, M + H) calcd: 431.2230, found: 431.2225.
4.1.23. General procedure for the preparation of compounds 27–36.
Method A. The AQs 1 or 2 and the corresponding 1,2-diamine were dissolved in EtOH and kept stirring for the specified time. Then, the organic solvent was evaporated off and the residue was chromatographed over silica-gel to give the corresponding derivative.
Method B. The AQs 1 or 2 and the corresponding 1,2-diamine were dissolved in dichloromethane and mortmorillonite K10, as solid support (3 times the reactants weight), was added and well mixed. Then, the organic solvent was evaporated under vacuum and the dried material was subjected to microwave irradiation in a domestic microwave oven for a specified time. The solid mixture was extracted with EtOAc and MeOH and after evaporation of the organic solvent, the reaction product was obtained and purified by column chromatography.
4.1.24. 2-(2-Aminoethylamino)-5,5(8,8)-dimethyl-5,6,7,8-tetrahydroanthracene-1,4-dione 27a(27b) and 8,8(11,11)-dimethyl-8,9,10,11-tetrahydronaphtho[2,3-f]quinoxalin-6-yl acetate 29a(29b). Following the above method A, a mixture of 1 (1.19 mmol) and 1,2-ethylenediamine (1.20 mmol) dissolved in EtOH, was stirred at rt for 10 min. Column chromatography of the reaction product (eluent Hex/EtOAc 1
:
1) afforded a 1
:
1 mixture of 27a/27b (30%): IR νmax (film): 3296, 3246, 1609, 1572, 1543, 1458, 1335, 1297, 1104, 732 cm−1; 1H NMR (CDCl3) δ: 1.30 (6H, s), 1.72–1.80 (4H, m), 2.81 (2H, t, J = 6.1), 3.28 (2H, t, J = 6.2), 4.02 (2H, t, J = 6.2), 5.63 (1H, s), 7.73 (1H, s), 7.99 (1H, s); HRMS (ES, M + Na − H2O) calcd: 303.1473, found: 303.1483.Following the method A, a mixture of 1 (1.21 mmol) and 1,2-ethylenediamine (1.73 mmol) dissolved in EtOH, was stirred at rt for 30 min. Column chromatography of the reaction product (eluent Hex/EtOAc 7
:
3) afforded: (a) a 1
:
1 mixture of 28a/28b (8%). (b) A 1
:
1 mixture of 27a/27b (6%). Acetylation of 28a/28b with acetic anhydride and pyridine yielded the acetates 29a/29b (61%): IR νmax (film): 1766, 1605, 1441, 1371, 1299, 1194, 1093, 733 cm−1; 1H NMR (CDCl3) δ: 1.42/1.44 (6H, s), 1.78–1.95 (4H, m), 2.54/2.53 (3H, s), 3.05/3.12 (2H, t, J = 6.3), 7.64/7.63 (1H, s), 7.90/7.62 (1H, s), 8.84 (1H, s), 8.86 (1H, s), 8.89/9.22 (1H, s); HRMS (ES, M + H) calcd: 321.1603, found: 321.1616.
Following the method B, a mixture of 1 (0.96 mmol) and 1,2-ethylenediamine (1.76 mmol) adsorbed on mortmorillonite K10 was irradiated at 700 W for 3 min at 1 min intervals. Column chromatography of the reaction product (eluent Hex/EtOAc 8
:
2 and 7
:
3) afforded: (a) a 1
:
1 mixture of 28a/28b (13%). (b) A 1
:
1 mixture of 27a/27b (4%).
4.1.25. 2-(2-Aminoethylamino)-3-chloro-5,5(8,8)-dimethyl-5,6,7,8-tetrahydroanthracene-1,4-dione 30a(30b), 5-chloro-8,8(11,11)-dimethyl-8,9,10,11-tetrahydronaphtho[2,3-f]quinoxalin-6-ol 32a(32b) and 5-chloro-8,8(11,11)-dimethyl-8,9,10,11-tetrahydro-naphtho[2,3-f]quinoxalin-6-yl acetate 34a(34b). Following the above method A, a mixture of 2 (0.45 mmol) and 1,2-ethylenediamine (0.48 mmol) dissolved in EtOH, was stirred at reflux for 16 h. Column chromatography of the reaction product (eluent Hex/EtOAc 7
:
3) afforded a 1
:
1 mixture of 30a/30b (30%): IR νmax (film): 3290, 1605, 1567, 1554, 1433, 1337, 1287, 1110, 734 cm−1; 1H NMR (CDCl3) δ: 1.33/1.34 (6H, s), 1.60–1.82 (4H, m), 2.85 (2H, m), 3.45 (2H, m), 4.14 (2H, m), 7.80/8.10 (1H, s), 8.12/7.81 (1H, s); HRMS (ES, M − H2O + H) calcd: 315.1258, found: 315.1272.Following the method A, a mixture of 2 (0.65 mmol) and 1,2-ethylenediamine (0.68 mmol) dissolved in EtOH, was stirred at reflux for 96 h. Column chromatography of the reaction product (eluent Hex/EtOAc 8
:
2) afforded a 1
:
1 mixture of 32a/32b (10%): IR νmax (film): 3306, 1582, 1434, 1375, 1331, 1151, 1118, 1082, 844, 735 cm−1; 1H NMR (CDCl3) δ: 1.44/1.46 (6H, s), 1.75–1.93 (4H, m), 3.06 (2H, m), 8.28/7.99 (1H, s), 8.77/9.11 (1H, s), 8.84 (1H, d, J = 2.2), 8.74 (1H, d, J = 2.2); HRMS (ES, M + H) calcd: 313.1108, found: 313.1148. Acetylation of 32a/32b with acetic anhydride and pyridine yielded the acetates 34a/34b: IR νmax (film): 1779, 1594, 1447, 1369, 1174, 1098, 1010, 871, 736 cm−1; 1H NMR (CDCl3) δ: 1.46/1.40 (6H, s), 1.76–1.92 (4H, m), 2.59/2.57 (3H, s), 3.03/3.08 (2H, t, J = 6.3), 7.83/7.55 (1H, s), 8.83/9.17 (1H, s), 8.91/8.88 (1H, d, J = 2.1), 8.95 (1H, d, J = 2.1); HRMS (ES, M + H) calcd: 355.1207, found: 355.1216.
Following the method B, a mixture of 2 (0.3 mmol) and 1,2-ethylenediamine (0.47 mmol) adsorbed on mortmorillonite K10 was irradiated at 500 W for 1 min and at 750 W for 2 min (1 min intervals). Column chromatography of the reaction product (eluent Hex/EtOAc 8
:
2) afforded: (a) 2 (11%). (b) A 1
:
1 mixture of 32a/32b (24%). (c) A 1
:
1 mixture of 30a/30b (20%).
4.1.26. 2-((2-Amino-1-methyl)ethylamino)-3-chloro-5,5(8,8)-dimethyl-5,6,7,8-tetrahydro-anthracene-1,4-dione 31a(31b), 5-chloro-3,8,8(3,11,11)-trimethyl-8,9,10,11-tetrahydro-naphtho[2,3-f]quinoxalin-6-ol 33a(33b) and 5-chloro-3,8,8(3,11,11)-trimethyl-8,9,10,11-tetrahydronaphtho[2,3-f]quinoxalin-6-yl acetate 35a(35b). Following the method A, a mixture of 2 (0.45 mmol) and 1,2-propanediamine (0.48 mmol) dissolved in EtOH, was stirred at reflux for 7 days. Column chromatography of the reaction product (eluent Hex/EtOAc 8
:
2) afforded: (a) a 1
:
1 mixture of 33a/33b (11%): 1H NMR (CDCl3) δ: 1.45/1.49 (6H, s), 1.76–1.94 (4H, m), 2.80/2.82 (3H, s), 3.08 (2H, m), 8.28/7.99 (1H, s), 8.82/9.14 (1H, s), 8.73 (1H, s). (b) A 1
:
1 mixture of 31a/31b (20%): IR νmax (film): 3311, 1641, 1605, 1570, 1436, 1272, 1175, 1093, 734 cm−1; 1H NMR (CDCl3) δ: 1.32/1.34 (6H, s), 1.65–1.93 (4H, m), 1.66/1.69 (3H, d, J = 3.3) 2.85 (2H, t, J = 6.0), 3.13 (2H, m), 3.51 (1H, m) 7.80/8.10 (1H, s), 8.14/7.83 (1H, s); HRMS (ES, M − H2O + H) calcd: 329.1421, found: 329.1427. Acetylation of 33a/33b with acetic anhydride and pyridine yielded the acetates 35a/35b: IR νmax (film): 1779, 1595, 1457, 1362, 1190, 1096, 966, 869, 735 cm−1; 1H NMR (CDCl3) δ: 1.41/1.48 (6H, s), 1.77–1.94 (4H, m), 2.59/2.58 (3H, s), 2.85/2.87 (3H, s), 3.04/3.10 (2H, t, J = 6.3), 7.81/7.54 (1H, s), 8.88/9.20 (1H, s), 8.83/8.82 (1H, s); HRMS (ES, M + H) calcd: 369.1364, found: 369.1373.
4.1.27. 6-Chloro-2,3,9,9(2,3,12,12)-tetramethyl-9,10,11,12-tetrahydronaphtho[2,3-a]phenazin-7-yl acetate 36a(36b). Following the method B, a mixture of 2 (0.3 mmol) and 4,5-dimethyl-1,2-phenylenediamine (0.47 mmol) adsorbed on mortmorillonite K10 was irradiated at 650 W for 0.5 min and an additional 1 min. The reaction product was acetylated and column chromatography of the acetylation product (eluent Hex/EtOAc 8
:
2) afforded a 1
:
1 mixture of 36a/36b (22%): IR νmax (film): 1778, 1492, 1357, 1179, 1097, 1015, 921, 869 cm−1; 1H NMR (CDCl3) δ: 1.42/1.52 (6H, s), 1.78–1.94 (4H, m), 2.56/2.58 (3H, s), 2.59 (6H, s), 3.04/3.13 (2H, t, J = 6.2), 7.52/7.81 (1H, s), 8.07 (1H, s), 8.13 (1H, s), 9.34/9.01 (1H, s); HRMS (ES, M + H) calcd: 433.1683, found: 433.1687.
4.1.28. General procedure for the preparation of compounds 40–43. To a solution of the corresponding arylamino-AQ 37–39 (ref. 23) in DMF, sodium azide, suspended in a little amount of water, was added and heated under reflux overnight. The reaction mixture was diluted with EtOAc and the organic phase was washed with brine, dried over Na2SO4 and the solvent evaporated off, affording a crude product that was purified by column chromatography to yield the corresponding phenazines.
4.1.29. 2-Methoxy-11,11(8,8)-dimethyl-8,9,10,11-tetrahydronaphtho[2,3-b]phenazine-6,13-dione 40a(40b). From 37a/37b (0.53 mmol) in DMF (5 mL) and NaN3 (1.07 mmol) and following the general procedure, a 1
:
1 mixture of 40a/40b (21%) was obtained: IR νmax (film): 1685, 1616, 1487, 1417, 1334, 1248, 1127, 1097, 1020, 841, 731 cm−1; 1H NMR (CDCl3) δ: 1.41 (6H, s), 1.76–1.87 (4H, m), 2.97 (2H, t, J = 6.5), 4.03 (3H, s), 7.62/7.60 (1H, d, J = 9.4/9.1), 7.69/7.71 (1H, s), 8.33/8.31 (1H, d, J = 9.1/9.4), 8.13/8.41 (1H, s) 8.41/8.13 (1H, s); HRMS (ES, M + Na) calcd: 395.1366, found: 395.1363.
4.1.30. 1,2-Dimethoxy-11,11(8,8)-dimethyl-8,9,10,11-tetrahydronaphtho[2,3-b]phenazine-6,13-dione 41a(41b). From 38a/38b (0.35 mmol) in DMF (4 mL) and NaN3 (0.77 mmol) and following the general procedure, a 1
:
1 mixture of 41a/41b (13%) was obtained: IR νmax (film): 1686, 1599, 1494, 1465, 1384, 1261, 1071, 1018, 732 cm−1; 1H NMR (CDCl3) δ: 1.41/1.42 (6H, s), 1.41–1.80 (4H, m), 2.99 (2H, t, J = 6.2), 4.14 (3H, s), 4.32/4.33 (3H, s), 7.83 (1H, d, J = 9.3), 8.13/8.42 (1H, s), 8.25/8.26 (1H, d, J = 9.3), 8.42/8.15 (1H, s); HRMS (ES, M + H) calcd: 403.1652, found: 403.1670.
4.1.31. 1,2,11,11(1,2,8,8)-Tetramethyl-8,9,10,11-tetrahydronaphtho[2,3-b]phenazine-6,13-dione 42a(42b) and 2,3,8,8-tetramethyl-8,9,10,11-tetrahydro-naphtho[2,3-b]phenazine-6,13-dione 43. From 39a/39b (0.60 mmol) in DMF (6 mL) and NaN3 (1.29 mmol), following the general procedure, and after column chromatography, the following compounds were separated: (a) a 1
:
1 mixture of 42a/42b (13%): IR νmax (film): 1686, 1597, 1458, 1334, 1252, 1018, 753 cm−1; 1H NMR (CDCl3) δ: 1.42 (6H, s), 1.64–1.77 (4H, m), 2.60 (3H, s), 2.91 (3H, s), 2.99 (2H, m), 7.79 (1H, d, J = 8.6), 8.20/8.21 (1H, d, J = 8.6), 8.12/8.41 (1H, s), 8.42/8.14 (1H, s); HRMS (ES, M + H) calcd: 371.1754, found: 371.1759. (b) Compound 43 (8%): mp 104–107 °C (from CH2Cl2); UV λmax(log
ε): 312 (4.6), 351 (4.0); IR νmax (film): 1688, 1596, 1481, 1363, 1334, 1311, 1248, 1022, 873, 734 cm−1; 1H NMR (CDCl3) δ: 1.41 (6H, s), 1.73–1.90 (4H, m), 2.56 (6H, s), 2.98 (2H, t, J = 6.3), 8.13 (1H, s), 8.18 (1H, s), 8.20 (1H, s), 8.41 (1H, s); HRMS (ES, M + H) calcd: 371.1754, found: 371.1766.
4.2. Cytotoxicity studies on tumour cell lines
A colourimetric assay using sulforhodamine B (SRB) was adapted for a quantitative measurement of cell growth and viability, following a previously described method.40 Cells were seeded in 96-well microtitre plates, at 5 × 103 cells per well in aliquots of 195 μL of RPMI medium, and they were allowed to attach to the plate surface by growing in a drug-free medium for 18 h. Afterwards, samples were added in aliquots of 5 μL (dissolved in DMSO/H2O, 3
:
7). After 72 h exposure, the antitumor effect was measured by the SRB methodology: cells were fixed by adding 50 μL of cold 50% (wt/vol) trichloroacetic acid (TCA) and incubating for 60 min at 4 °C. Plates were washed with deionised water and dried; 100 μL of SBR solution (0.4% wt/vol in 1% acetic acid) was added to each microtitre well and incubated for 10 min at room temperature. Unbound SRB was removed by washing with 1% acetic acid. Plates were air-dried and bound stain was solubilized with Tris buffer. Optical densities (OD) were read on an automated spectrophotometer plate reader at single wavelength of 490 nm. Data analyses were generated automatically by the LIMS implementation. Using control OD values (C), test OD values (T) and time zero OD values (To), the drug concentration that caused a 50% growth inhibition (GI50 value) was calculated from the equation: 100 × [(T – To)/(C – To)] = 50. Each value represents the mean from triplicate determinations.
4.3. Cytotoxicity studies on normal Vero cells
Vero cells (African green monkey kidney-Cercopithecus aethiops, ATCC: CCL 81 line) were maintained in Dulbecco's Modified Eagle Medium (DMEM) supplemented with 5% inactivated Fetal Bovine Serum (FBS), 100 units per mL of penicillin, 100 μg mL−1 of streptomycin, 100 μg mL−1 of L-glutamine, 0.14% NaHCO3, and 1% of each non-essential amino acids and minimum essential medium vitamin solution (choline chloride, D-calcium pantothenate, folic Acid, nicotinamide, pyridoxal hydrochloride, riboflavin, thiamine hydrochloride and i-Inositol). Cells were incubated at 37 °C in humidified 5% CO2 atmosphere.
The cytotoxicity on Vero cells, was examined in vitro using an MTT (dimethylthiazol-2-yl]-2,5-diphenyl tetrazolium bromide) (Sigma, New Jersey, USA) assay as described by Betancur-Galvis et al.50 Briefly, Vero (African green monkey kidney, ATCC: CCL 81) cell line was seeded into 96-well plates at 2.0 × 103 cells per well and incubated for 24 h at 37 °C. After incubation, each diluted compound was added to the appropriate well and the plates were incubated for 48 h at 37 °C in a humidified 5% CO2 atmosphere. Subsequently, the culture medium was removed and the MTT solution (24 μL, 2 mg mL−1) was added to each well. The minimal dilution of compound that induced 50% growth inhibition of the cells was expressed as Inhibitory Concentration 50% (IC50). The IC50 values for each compound were obtained by linear regression analysis of the dose–response curves generated from the absorbance data with the statistical package R (Development Core Team, Vienna, Austria, 2008). IC50 values were expressed as the mean ± standard deviation (M ± SD) of two independent experiments done in quadruplicate.
4.4. Antifungal assay
The antifungal activity were evaluated following the standard method of the Antifungal Susceptibility Testing Subcommittee of the European Committee on Antibiotic Susceptibility Testing for yeasts (AFST-EUCAST)41 and the Clinical and Laboratory Standards Institute (CLSI) M38-A protocol42 for filamentous fungi with some modifications. The yeast Candida parapsilosis (ATCC 22019), C. krusei (ATCC 6258), C. tropicalis (Ct1, CECT 11901), C. tropicalis (Ct2, ATCC 200956), C. albicans (Ca1, ATCC 10231), C. albicans (Ca2, ATCC 90028), C. lusitaniae (ATCC 200951), and filamentous fungi Aspergillus fumigatus (ATCC 204305), A. flavus (ATCC 204304), A. terreus (CDC 317), A. niger (ATCC 16404), Fusarium oxysporum (ATCC 48112), and the dermatophytes Trichophyton rubrum (ATCC 28188) and T. mentagrophytes (ATCC 24198) were used to evaluate antifungal activity.
Briefly, 100 μL of five serial dilutions of the compounds were dispensed into 96-well microtitration plates (Becton Dickinson, New Jersey, USA) in duplicate at final concentrations between 2–32 μg mL−1. The same volume of inoculum of each strain was added to the dilutions of the compounds to achieve a final inoculum size of 0.5–2.5 × 105 and 0.4–5 × 104 CFU mL−1 for yeast and filamentous fungi, respectively. Aspergillus fumigatus and A. flavus were tested with amphotericin B (AmB, Sigma Chemical Co, MO, USA), C. krusei ATCC and C. parapsilosis ATCC with itraconazole (ITZ, Sigma Chemical Co, MO, USA) and T. mentagrophytes and T. rubrum with terbinafine (TRB, Recalcine Laboratories, Santiago de Chile, Chile) as positive control. The range of antifungal was 0.031 to 16.0 μg mL−1. For the AFST-EUCAST method, the Minimal Inhibitory Concentrations (MICs) were determined after 24 h of incubation at 35 °C by spectrophotometric reading at 405 nm and it was defined as the lowest concentration that resulted in a 90% reduction of growth. For filamentous fungi MICs were determined after 48 h of incubation at 35 °C for Aspergillus spp. and at 28 °C to F. oxysporum. The MIC values for T. rubrum and T. mentagrophytes were defined after 6 days of incubation at 28 °C. MICs were the lowest drug concentrations that produced growth inhibition in a 90% of inhibition. Each compound was tested in duplicate on three different assays and MIC results were expressed as their geometric mean (GM).
4.5. Antiviral assay
Both Human Herpes virus 1 (HHV-1 CDC Atlanta acyclovir-sensitive strain) and Human Herpes virus 2 (HHV-2 VR-734-G acyclovir-sensitive strain) were from the Center for Disease Control (CDC, Atlanta, GA) and they were amplified in Vero cells. Virus stocks were titrated in Vero cells by plaque assay and expressed as plaque forming units (PFU mL−1).
The antiviral activity evaluation of 1,4-AQ derivatives on Vero cells (Cercopithecus aethiops African green monkey kidney cell line ATCC CCL 81) against 1TCID50 of HHV-1 and 10TCID50 of HHV-2, were carried out using the end-point titration technique (EPTT).47 Vero cells were harvested in 96-well plates at a density of 2.0 × 104 cells per well at 37 °C in a humidified 5% CO2 atmosphere until constituted ≥80% of the cell monolayer. Then TCID50 viral suspensions of HHV-1 or HHV-2 with the compounds at concentrations of 50 to 6.25 μg mL−1 were performed in Minimum Essential Medium eagle (MEM) 2% FBS supplemented containing carboxymethylcellulose 1% and 0.5% for HHV-1 and HHV-2 respectively and incubated for 15 minutes at room temperature before being added to cell monolayer. Virus/compound mixture was added later to the confluent monolayer of Vero cells. After 48 h of a humidified 5% CO2 atmosphere at 37 °C, the cytopathic effect was examined, and then the microplates cells were stained with a solution of 3.5% formaldehyde with 0.2% of crystal violet. The activity was evaluated by determining the reduction factor (Rf), which corresponds to the value obtained by dividing the viral titer in the absence of compound over the viral titer obtained in the presence of compound.47 Two independent experiments by quadruplicate were carried out for each viral serotype and for each compound. Controls were included untreated cells, cells treated with compounds and cells infected with HHV-1 or HHV-2. Two positive controls were included in this assay: dextran sulfate and acyclovir. According to the parameters established by Vlietinck et al.,51 the relevant or moderate antiviral activity of a purified natural product, is one whose Rf of viral titer is respectively of ≥1 × 103 or 1 × 102. In this study, the mild or moderate antiviral activity is one whose Rf of viral titer of ≥1 × 101 or 1 × 102, respectively. The antiviral SI was determinate and defined as the ratio between the inhibitory concentration 50 (IC50) in Vero cells and the concentration that inhibits 50% of the cytopathic effect caused by the virus on cells (EC50). A compound with a SI ≥ 10 was considered selective.
Acknowledgements
Financial support came from: Spanish Junta de Castilla y León, co-funded by FSE (BIO/SA74/13 and SA 221U13) and Universidad de Salamanca (18KAQD and KAC9); AMG thanks the JCyL for a pre-doctoral fellowship; Universidad de Antioquia and COLCIENCIAS (Colombia, Patrimonio Autónomo del Fondo Nacional de Financiamiento para la Ciencia, la Tecnología y la Innovación, Francisco José de Caldas) (Grant RC 366-2011). Collaborative work performed under the auspices of “Programa Iberoamericano de Ciencia y Tecnología para el Desarrollo (CYTED), Área de Salud. Red RIBECÁNCER”.
References
-
(a) V. K. Tandon and S. Kumar, Expert Opin. Ther. Pat., 2013, 23, 1087–1108 CrossRef CAS PubMed;
(b) S. N. Sunassee and M. T. Davies-Coleman, Nat. Prod. Rep., 2012, 29, 513–535 RSC.
- M. A. Colucci, C. J. Moody and G. D. Couch, Org. Biomol. Chem., 2008, 6, 637–656 CAS.
- C. Asche, Mini-Rev. Med. Chem., 2005, 5, 449–467 CrossRef CAS PubMed.
-
(a) R. H. Thomson, Naturally Occurring Quinones. IV. Recent Advances, Blackie Academic & Professional, London, 4th edn, 1997 Search PubMed;
(b) P. Babula, V. Adam, L. Havel and R. Kizek, Curr. Pharm. Anal., 2009, 5, 47–68 CrossRef CAS.
-
(a) K. Kaur, M. Jain, T. Kaur and R. Jain, Bioorg. Med. Chem., 2009, 17, 3229–3256 CrossRef CAS PubMed;
(b) M. L. Bolognesi, F. Lizzi, R. Perozzo, R. Brun and A. Cavalli, Bioorg. Med. Chem. Lett., 2008, 18, 2272–2276 CrossRef CAS PubMed;
(c) A. V. Pinto and S. L. de Castro, Molecules, 2009, 14, 4570–4590 CrossRef PubMed;
(d) B. S. Samant and C. Chakaingesu, Bioorg. Med. Chem. Lett., 2013, 23, 1420–1423 CrossRef CAS PubMed;
(e) P. M. García-Barrantes, G. V. Lamoureux, A. L. Pérez, R. N. García-Sánchez, A. R. Martínez and A. San Feliciano, Eur. J. Med. Chem., 2013, 70, 548–557 CrossRef PubMed.
-
(a) V. K. Tandon, D. B. Yadav, R. V. Singh, A. K. Chaturvedi and P. K. Shukla, Bioorg. Med. Chem. Lett., 2005, 15, 5324–5328 CrossRef CAS PubMed;
(b) J. Koyama, I. Morita, N. Kobayashi, T. Osakai, Y. Usuki and M. Taniguchi, Bioorg. Med. Chem. Lett., 2005, 15, 1079–1082 CrossRef CAS PubMed;
(c) V. K. Tandon, D. B. Yadav, H. K. Maurya, A. K. Chaturvedi and P. K. Shukla, Bioorg. Med. Chem., 2006, 14, 6120–6126 CrossRef CAS PubMed.
-
(a) K. Sasaki, H. Abe and F. Yoshizaki, Biol. Pharm. Bull., 2002, 25, 669–670 CrossRef CAS PubMed;
(b) V. K. Tandon, R. B. Chhor, R. V. Singh, S. Rai and D. B. Yadav, Bioorg. Med. Chem. Lett., 2004, 14, 1079–1083 CrossRef CAS PubMed;
(c) C. K. Ryu, J. Y. Shim, M. J. Chae, I. H. Choi, J. Y. Han, O. J. Jung, J. Y. Lee and S. H. Jeong, Eur. J. Med. Chem., 2005, 40, 438–444 CrossRef CAS PubMed.
-
(a) V. K. Tandon, D. B. Yadav, R. V. Singh, M. Vaish, A. K. Chaturvedi and P. Shukla, Bioorg. Med. Chem. Lett., 2005, 15, 3463–3466 CrossRef CAS PubMed;
(b) J. Koyama, Y. Nisino, I. Morita, N. Kobayashi, T. Osakai and H. Tokuda, Bioorg. Med. Chem. Lett., 2008, 18, 4106–4109 CrossRef CAS PubMed;
(c) I. T. Crosby, D. G. Bourke, E. D. Jones, P. J. de Bruyn, D. Rhodes, N. Vandegraaff, S. Cox, J. A. V. Coates and A. D. Robertson, Bioorg. Med. Chem., 2010, 18, 6442–6450 CrossRef CAS PubMed.
- R. L. Nyland II, M. Luo, M. R. Kelley and R. F. Borch, J. Med. Chem., 2010, 53, 1200–1210 CrossRef PubMed.
-
(a) W. O. Foye, Cancer chemotherapeutic agents, ACS, Washington, DC, 1995 Search PubMed;
(b) E. A. Sausville, J. I. Johnson and G. M. Cragg, S. Decker, in Anticancer agents. Frontiers in cancer chemotherapy, ed. I. Ojima, G. D. Vite and K. H. Altmann, ACS, Washington, DC, 2001, pp. 1–15 Search PubMed;
(c) P. R. Dandawate, A. C. Vyas, S. B. Padhye, M. W. Singh and J. B. Baruah, Mini-Rev. Med. Chem., 2010, 10, 436–454 CrossRef CAS PubMed.
-
(a) J. Koyama, I. Morita and T. Yamori, Molecules, 2010, 15, 6559–6569 CrossRef CAS PubMed;
(b) T. J. Monks, R. P. Hanzlik, G. M. Cohen, D. Ross and D. G. Graham, Toxicol. Appl. Pharmacol., 1992, 112, 2–16 CrossRef CAS PubMed;
(c) P. J. O'Brien, Chem.-Biol. Interact., 1991, 80, 1–41 (Chem.-Biol. Interact., 1992, 81, 219) CrossRef , erratum;
(d) T. J. Monks and D. C. Jones, Curr. Drug Metab., 2002, 3, 425–438 CrossRef CAS PubMed;
(e) Y. Kumagai, Y. Shinkai, T. Miura and A. K. Cho, Annu. Rev. Pharmacol. Toxicol., 2012, 52, 221–247 CrossRef CAS PubMed.
-
(a) B. Cavalcanti, I. O. Cabral, F. A. R. Rodrigues, F. W. A. Barros, D. D. Rocha, H. I. F. Magalhaes, D. J. Maura, J. Saffi, J. A. P. Henriques, T. S. C. Carvalho, M. O. Moraes, C. Pessoa, I. M. M. de Melo and E. N. da Silva-Junior, J. Braz. Chem. Soc., 2013, 24, 145–163 CrossRef CAS;
(b) E. A. Hillard, F. C. de Abreu, D. C. M. Ferreira, G. Jaouen, M. O. F. Goulart and A. Amatore, Chem. Commun., 2008, 2612–2628 RSC;
(c) E. Simamura, H. Shimada, Y. Ishigaki, T. Hatta, N. Higashi and K. I. Hirai, Anat. Sci. Int., 2008, 83, 261–266 CrossRef PubMed.
- E. Pérez-Sacau, R. G. Díaz-Peñate, A. Estévez-Braun, A. G. Ravelo, J. M. García-Castellano, L. Pardo and M. Campillo, J. Med. Chem., 2007, 50, 696–706 CrossRef PubMed.
- M. L. Bolognesi, Curr. Med. Chem., 2013, 20, 1639–1645 CrossRef CAS PubMed.
-
(a) A. A. Aly, E. A. Ishak, M. A. Alsharari, N. S. Al-Muaikel and T. M. I. Bedair, J. Heterocycl. Chem., 2012, 49, 9–20 CrossRef CAS;
(b) A. Bargiotti, L. Musso, S. Dallavalle, L. Merlini, G. Gallo, A. Ciacci, G. Giannini, W. Cabri, S. Penco, L. Vesci, M. Castorina, F. M. Milazzo, M. L. Cervoni, M. Barbarino, C. Pisano, C. Giommarelli, V. Zuco, M. De Cesare and F. Zunino, Eur. J. Med. Chem., 2012, 53, 64–75 CrossRef CAS PubMed.
- J. Sperry, P. Bachu and M. A. Brimble, Nat. Prod. Rep., 2008, 25, 376–400 RSC.
-
(a) C. Ibis, A. F. Tuyun, Z. Ozsoy-Gunes, H. Bahar, M. V. Stasevych, R. Y. Musyanovych, O. Komarovska-Porokhnuavets and V. Novikov, Eur. J. Med. Chem., 2011, 46, 5861–5867 CrossRef CAS PubMed;
(b) L. S. Konstantinova, K. A. Lysov, L. I. Souvorova and O. A. Rakitin, Beilstein J. Org. Chem., 2013, 9, 577–584 CrossRef CAS PubMed.
-
(a) M. H. Moore, W. N. Hunter, B. L. D'Estaintot and O. Kennard, J. Mol. Biol., 1989, 206, 693–705 CrossRef CAS PubMed;
(b) R. Martínez and L. Chacón-Garcia, Curr. Med. Chem., 2005, 12, 127–151 CrossRef;
(c) J. Jacobs, K. A. Tehrani and N. de Kimpe, Tetrahedron, 2011, 67, 9459–9471 CrossRef CAS.
- J. S. Kim, H. J. Lee, M. E. Suh, H. Y. P. Choo, S. K. Lee, H. J. Park, C. Kim, S. W. Park and C. O. Lee, Bioorg. Med. Chem., 2004, 12, 3683–3686 CrossRef CAS PubMed.
-
(a) D. Mal and S. Ray, Eur. J. Org. Chem., 2008, 3014–3020 CrossRef CAS;
(b) E. M. Perchellet, Y. Wang, K. Lou, H. Zhao, S. K. Battina, D. H. Hua and J. P. H. Perchellet, Int. J. Oncol., 2007, 31, 1231–1241 CAS.
-
(a) M. A. Castro, J. M. Miguel del Corral, M. Gordaliza, P. A. García, A. M. Gamito, S. A. Gualberto, R. Batista and A. San Feliciano, Synthesis, 2005, 3202–3208 CrossRef CAS;
(b) J. M. Miguel del Corral, M. A. Castro, A. B. Oliveira, S. A. Gualberto, C. Cuevas and A. San Feliciano, Bioorg. Med. Chem., 2006, 14, 7231–7240 CrossRef CAS PubMed.
-
(a) F. Prati, E. Uliassi and M. L. Bolognesi, MedChemComm, 2014, 5, 853–861 RSC;
(b) S. Pieretti, J. R. Haanstra, M. Mazet, R. Perozzo, C. Bergamini, F. Prati, R. Fato, G. Lenaz, G. Capranico and R. Brun, PLoS Neglected Trop. Dis., 2013, 7, e2012 CAS.
- M. A. Castro, A. M. Gamito, V. Tangarife-Castaño, B. Zapata, J. M. Miguel del Corral, A. C. Mesa-Arango, L. Betancur-Galvis and A. San Feliciano, Eur. J. Med. Chem., 2013, 67, 19–27 CrossRef PubMed.
-
(a) J. B. A. Neto, C. R. da Silva, M. A. S. Neta, R. S. Campos, J. T. Siebra, R. A. C. Silva, D. M. Gaspar, H. I. F. Magalhaes, M. O. de Moraes, M. D. P. Lobo, T. B. Grangeiro, T. S. C. Carvalho, E. B. T. Diogo, E. N. da Silva-Junior, F. A. R. Rodrigues, B. C. Cavalcanti and H. V. N. Junior, PLoS One, 2014, 9, e93698 Search PubMed;
(b) C. P. V. Freire, S. B. Ferreira, N. S. M. de Oliveira, A. B. J. Matsuura, I. L. Gama, F. C. da Silva, M. C. B. C. de Souza, E. S. Lima and V. F. Ferreira, MedChemComm, 2010, 1, 229–232 RSC.
-
(a) A. C. Mesa-Arango, N. Trevijano-Contador, E. Román, R. Sánchez-Fresneda, C. Casas, E. Herrero, J. C. Argüelles, J. Pla, M. Cuenca-Estrella and O. Zaragoza, Antimicrob. Agents Chemother., 2014, 58, 6627–6638 CrossRef PubMed;
(b) P. Belenky, D. Camacho and J. J. Collins, Cell Rep., 2013, 21, 350–358 CrossRef PubMed;
(c) E. J. Helmerhorst, R. F. Troxler and F. G. Oppenheim, Proc. Natl. Acad. Sci. U. S. A., 2001, 98, 14637–14642 CrossRef CAS PubMed.
-
(a) J. M. Miguel del Corral, M. Gordaliza, M. A. Castro, M. M. Mahiques, A. San Feliciano and M. D. García-Grávalos, Bioorg. Med. Chem., 1998, 6, 31–41 CrossRef CAS PubMed;
(b) J. M. Miguel del Corral, M. Gordaliza, M. A. Castro, M. M. Mahiques, P. Chamorro, A. Molinari, M. D. García-Grávalos, H. B. Broughton and A. San Feliciano, J. Med. Chem., 2001, 44, 1257–1267 CrossRef CAS PubMed.
-
(a) J. M. Miguel del Corral, M. A. Castro, M. Gordaliza, M. L. Martín, S. A. Gualberto, A. M. Gamito, C. Cuevas and A. San Feliciano, Bioorg. Med. Chem., 2005, 13, 631–644 CrossRef CAS PubMed;
(b) J. M. Miguel del Corral, M. A. Castro, M. Gordaliza, M. L. Martín, A. M. Gamito, C. Cuevas and A. San Feliciano, Bioorg. Med. Chem., 2006, 14, 2816–2827 CrossRef CAS PubMed;
(c) J. M. Miguel del Corral, M. A. Castro, M. L. Rodríguez, P. Chamorro, C. Cuevas and A. San Feliciano, Bioorg. Med. Chem., 2007, 15, 5760–5774 CrossRef CAS PubMed;
(d) A. Molinari, A. Oliva, C. Ojeda, J. M. Miguel del Corral, M. A. Castro, C. Cuevas and A. San Feliciano, Arch. Pharm., 2009, 342, 591–599 CrossRef CAS PubMed;
(e) M. A. Castro, J. M. Miguel del Corral, M. L. Rodríguez and A. San Feliciano, Tetrahedron Lett., 2012, 53, 519–521 CrossRef CAS.
-
(a) M. A. Pfaller and D. J. Diekema, Crit. Rev. Microbiol., 2010, 36, 1–53 CrossRef PubMed;
(b) J. Pemán and M. Salavert, Enferm. Infecc. Microbiol. Clin., 2012, 30, 90–98 CrossRef PubMed.
- M. A. Pfaller, Am. J. Med., 2012, 125(suppl. 1), S3–S13 CrossRef CAS PubMed.
- M. Nucci, A. G. Varon, M. Garnica, T. Akiti, G. Barreiros, B. M. Trope and S. A. Nouér, Emerging Infect. Dis., 2013, 19, 1567–1572 CrossRef PubMed.
-
(a) M. D. Guerrero and K. K. Swenson, Oncol. Nurs. Forum., 2014, 41, 327–330 CrossRef PubMed;
(b) D. Toomey, A. Dhadda, L. Sanni, J. Cooke and J. Hartley, Ann. R. Coll. Surg. Engl., 2014, 96, 12–14 CrossRef PubMed.
- F. Belluti, E. Uliassi, G. Veronesi, C. Bergamini, M. Kaiser, R. Brun, A. Viola, R. Fato, P. A. M. Michels, R. L. Krauth-Siegel, A. Cavalli and M. L. Bolognesi, ChemMedChem, 2014, 9, 371–382 CrossRef CAS PubMed.
-
(a) T. H. Bacon, M. J. Levin, J. J. Leary, R. T. Sarisky and D. Sutton, Clin. Microbiol. Rev., 2003, 16, 114–128 CrossRef CAS PubMed;
(b) C. Gilbert, J. Bestman-Smith and G. Boivin, Drug Resist. Updates, 2002, 5, 88–114 CrossRef CAS.
- C. Spampinato and D. Leonardi, BioMed Res. Int., 2013, 2013, 204237 Search PubMed.
- A. A. Kutyrev, Tetrahedron, 1991, 47, 8043–8065 CrossRef CAS.
- C. C. Tseng, Y. L. Wu and C. P. Chuang, Tetrahedron, 2002, 58, 7625–7633 CrossRef CAS.
- S. Yerushalmi, N. G. Lemcoff and S. Bittner, Synthesis, 2007, 239–242 CAS.
-
(a) A. Molinari, A. Oliva, C. Ojeda, J. M. Miguel del Corral, M. A. Castro, C. Cuevas and A. San Feliciano, Bioorg. Med. Chem., 2005, 13, 6645–6650 CrossRef CAS PubMed;
(b) A. Molinari, A. Oliva, C. Ojeda, J. M. Miguel del Corral, M. A. Castro, C. Cuevas and A. San Feliciano, Arch. Pharm., 2008, 341, 301–306 CrossRef CAS PubMed.
- Y. S. Kim, S. Y. Park, M. E. Suh, H. J. Lee and D. Schollmeyer, Bioorg. Med. Chem., 2003, 11, 1829–1833 CrossRef CAS PubMed.
-
(a) G. T. Faircloth, D. Stewart and J. J. Clement, J. Tissue Cult. Methods, 1988, 11, 201–205 CrossRef;
(b) P. Skehan, R. Storeng, D. Scudiero, A. Monks, J. McMahon, D. Vistica, J. T. Waren, H. Bokesch, S. Kenney and M. R. Boyd, J. Natl. Cancer Inst., 1990, 82, 1107–1112 CrossRef CAS PubMed.
- M. Cuenca-Estrella, C. B. Moore, F. Barchiesi, J. Bille, E. Chryssanthou, D. W. Denning, J. P. Donnelly, F. Dromer, B. Dupont, J. H. Rex, M. D. Richardson, B. Sancak, P. E. Verweij and J. L. Rodríguez-Tudela, AFST Subcommittee of the European Committee on Antimicrobial Susceptibility Testing. Multicenter evaluation of the reproducibility of the proposed antifungal susceptibility testing method for fermentative yeasts of the Antifungal Susceptibility Testing Subcommittee of the European Committee on Antimicrobial Susceptibility Testing (AFST-EUCAST), Clin. Microbiol. Infect., 2003, 9, 467–474 CrossRef CAS PubMed.
- National Committee for Clinical Laboratory Standards, Reference Method for Broth Dilution Antifungal Susceptibility Testing of Filamentous Fungi; Approved Standard, Document M38-A. Wayne, National Committee for Clinical Laboratory Standards, USA, 2002 Search PubMed.
- C. Lass-Flörl, K. Griff, A. Mayr, A. Petzer, G. Gastl, H. Bonatti, M. Freund, G. Kropshofer, M. P. Dierich and D. Nachbaur, Br. J. Haematol., 2005, 131, 201–207 CrossRef PubMed.
-
(a) A. K. Esnakula, I. Summers and T. J. Naab, Case Rep. Infect. Dis., 2013, 379320 Search PubMed;
(b) M. Muhammed, J. J. Coleman, H. A. Carneiro and E. Mylonakis, Virulence, 2011, 2, 91–96 CrossRef PubMed.
- K. C. Hazen, E. J. Baron, A. L. Colombo, C. Girmenia, A. Sanchez-Sousa, A. del Palacio, C. de Bedout and D. L. Gibbs, J. Clin. Microbiol., 2003, 41, 5623–5632 CrossRef CAS PubMed.
-
(a) S. Kumar, N. Shakya, S. Gupta, J. Sarkar and D. P. Sahu, Bioorg. Med. Chem. Lett., 2009, 19, 2542–2545 CrossRef CAS PubMed;
(b) P. Prayong, S. Barusrux and N. Weerapreeyakul, Fitoterapia, 2008, 79, 598–601 CrossRef CAS PubMed.
- L. S. Agudelo-Gómez, G. A. Gómez Ríos, D. C. Durán García, E. Stashenko and L. Betancur-Galvis, Salud UIS, 2010, 42, 234–243 Search PubMed.
- Food and Drug Administration, Center for Drug Evaluation and Research. Guidance for Industry, Antiviral Product Development-Conducting and Submitting Virology Studies to the Agency, U.S. Department of Health and Human Services, 2006 Search PubMed.
- L. M. Bush, K. Talledo-Thais, A. Casal-Fernandez and M. T. Perez, Lab. Med., 2011, 42, 452–457 CrossRef.
- L. A. Betancur-Galvis, G. E. Morales, J. E. Forero and J. Roldan, Mem. Inst. Oswaldo Cruz, 2002, 97, 541–546 CAS.
- A. J. Vlietinck, L. Van Hoof, J. Totté, A. Lasure, D. Vanden Berghe, P. C. Rwangabo and J. Mvukiyumwami, J. Ethnopharmacol., 1995, 46, 31–47 CrossRef CAS PubMed.
Footnote |
† Electronic supplementary information (ESI) available: 13C NMR data and complete assignments for the 1H and 13C NMR signals are included together with HMQC and HMBC spectra for several separated regioisomers. Complementary antifungal, antiviral and Vero cytotoxicity data for all the compounds tested. See DOI: 10.1039/c4ra11726c |
|
This journal is © The Royal Society of Chemistry 2015 |