DOI:
10.1039/C4RA06695B
(Communication)
RSC Adv., 2014,
4, 51140-51145
Efficient and convenient oxidation of sulfides to sulfoxides with molecular oxygen catalyzed by Mn(OAc)2 in ionic liquid [C12mim][NO3]
Received
11th July 2014
, Accepted 1st September 2014
First published on 3rd September 2014
Abstract
A simple, efficient, and eco-friendly procedure for aerobic oxidation of sulfides catalyzed by Mn(OAc)2/[C12mim][NO3] has been developed. The reactions afford the target products in good to high yields and no over-oxidation was observed. The products can be easily separated, and the catalytic system can be recycled without loss of catalytic activity.
Sulfoxides are an important class of chemicals commonly used as synthetic intermediates for the construction of various chemically and biologically significant molecules, as well as for the preparation of natural products and drugs.1 Therefore, there has been tremendous interest in developing efficient methods for the synthesis of these molecules, and a well known method constitutes the oxidation of sulfides. Traditional methods for performing such a transformation generally involve the use of stoichiometric amount of the strongest oxidizing reagents (i.e., peracids, MeNO2/HNO3/H2SO4, HIO3, periodates, etc.).2 However, these protocols are generally associated with one or more disadvantages, such as environmental hazards, low yield, harsh or delicate reaction conditions, and a large amount of waste byproducts. Oxidation with ceric ammonium nitrate/NaBrO3,3 H5IO6/FeCl3,4 AgNO3/t-BuOOH,5 trichloroisocyanuric acid,6 H2O2,7 2-iodoxybenzoic acid,8 and other reagents9 have also been developed for this conversion. However, some of the procedures are invariably associated with one or more disadvantages such as long reaction times, high temperatures, difficulties in work up, the use of expensive, toxic and moisture sensitive reagents, and difficulties in recycling of the catalyst.
Molecular oxygen is a safe, economical and environmentally-benign reagent, molecular oxygen has recently been utilized as an attractive oxidant for the oxidation of sulfides for the production of these compounds due to cheap price and environmental friendly nature. Therefore, molecular oxygen-based protocols are powerful methods for oxidation of sulfides and attract a great attention, which make the reactions of choice in large and industrial scale applications. A variety of catalytic systems, such as ruthenium,10 palladium,11 iron,12 osmate,13 gold,14 and other systems,15 have been developed for this conversion. However, most of the procedures still suffered from the use of expensive reagents, difficulties in work up, long reaction times, environmental hazards, waste control, etc. Consequently, search for new and environmentally benign synthetic methodologies for oxidation of sulfides that address these drawbacks remains to be of value and interest.
Ionic liquids (ILs) have attracted considerable attention during the past few years due, in some cases, to their favourable properties such as very low vapor pressure, wide liquid temperature range, good ionic conductivity, excellent electrochemical properties, and strong ability to dissolve many chemicals.16,17 Therefore, ILs have recently gained recognition of scholars from various fields such as analytical and separation science,18 chemical synthesis,19 biocatalytic transformations,20 electrochemistry,21 and catalysis.22 In continuation of our interest in exploring green oxidation reactions in ionic liquids, we report herein a new, efficient and environmentally friendly protocol for the selective aerobic oxidation of sulfides to sulfoxides catalyzed by manganese acetate (Mn(OAc)2) in ionic liquid 1-dodecyl-3-methylimidazolium nitration ([C12mim][NO3]) under mild conditions (Scheme 1). Furthermore, we demonstrate that the catalytic system can be recycled and reused without any significant loss of catalytic activity.
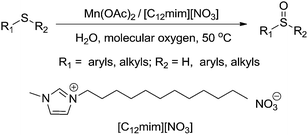 |
| Scheme 1 Aerobic oxidation of sulfides to sulfoxides. | |
To optimize the reaction conditions, methyl(phenyl)sulfane was firstly chosen as the model substrate (Table 1). Eight type of ionic liquids, [C8mim][NO3], [C10mim][NO3], [C12mim][NO3], [C12mim][NO2], [C12mim][HSO4], [C12mim][PF6], [C12mim][BF4], and [C12mim]Br, were tested with Mn(OAc)2 as catalyst in the reaction (Table 1, entries 2–9), it was observed that [C12mim][NO3] demonstrated the best performance (Table 1, entry 4). The different catalytic effects of ILs may be attributed to their different abilities of stabilizing and dissolving O2, the catalyst and the substrate. Under reaction conditions, O2 may be more soluble in [C12mim][NO3], leading to higher effective concentration of the oxidant, which increases the encounter probabilities between the substrate and reactive species, and so the higher rate and yield of the reaction is observed. As expected, no product was detected in the absence of ionic liquid and catalyst (Table 1, entry 1). Furthermore, the catalyst Mn(OAc)2, the ionic liquid [C12mim][NO3] and molecular oxygen are crucial for the oxidation, and the lack of any component can lead to a much lower yield (Table 1, entries 10–12). Besides Mn(OAc)2, other type of catalysts were also tested in this model reaction (Table 1, entries 13–20), and the results showed that Mn(OAc)2 demonstrated the best performance. In addition, no over-oxidized product ((methylsulfonyl)benzene) was detected by 1H NMR analysis of the crude reaction mixtures in all the cases. Therefore, the combination of [C12mim][NO3] and Mn(OAc)2 was chosen as the optimal conditions for further exploration.
Table 1 Optimization of the conditions for oxidation of methyl(phenyl)sulfane with molecular oxygena
Entry |
Ionic liquid |
Catalyst |
Time/h |
Yieldb/(%) |
The reactions were carried out with methyl(phenyl)sulfane (0.1 mol), catalyst (0.2 mol), IL (20 mL), and H2O (10 mL) in the presence of O2 gas (50 mL min−1) at 50 °C. Isolated yield. The reaction was carried out in the absence of O2 gas. |
1 |
— |
— |
12 |
0 |
2 |
[C8mim][NO3] |
Mn(OAc)2 |
2 |
86 |
3 |
[C10mim][NO3] |
Mn(OAc)2 |
2 |
95 |
4 |
[C12mim][NO3] |
Mn(OAc)2 |
2 |
97 |
5 |
[C12mim][NO2] |
Mn(OAc)2 |
2 |
91 |
6 |
[C12mim][HSO4] |
Mn(OAc)2 |
2 |
58 |
7 |
[C12mim][PF6] |
Mn(OAc)2 |
2 |
69 |
8 |
[C12mim][BF4] |
Mn(OAc)2 |
6 |
37 |
9 |
[C12mim]Br |
Mn(OAc)2 |
2 |
82 |
10 |
— |
Mn(OAc)2 |
8 |
46 |
11 |
[C12mim][NO3] |
— |
8 |
52 |
12 |
[C12mim][NO3] |
Mn(OAc)2 |
12 |
76c |
13 |
[C12mim][NO3] |
Cu(OAc)2 |
2 |
67 |
14 |
[C12mim][NO3] |
Co(OAc)2 |
2 |
74 |
15 |
[C12mim][NO3] |
Fe(OAc)2 |
4 |
58 |
16 |
[C12mim][NO3] |
Fe(OAc)3 |
2 |
77 |
17 |
[C12mim][NO3] |
FeSO4 |
2 |
43 |
18 |
[C12mim][NO3] |
FeCl3 |
4 |
76 |
19 |
[C12mim][NO3] |
MnSO4 |
2 |
61 |
20 |
[C12mim][NO3] |
Pd(OAc)2 |
5 |
78 |
In addition, the catalytic system could be typically recovered and reused for subsequent reactions with no appreciable decrease in yields and reaction rates (Fig. 1). The recycling process involved the removal of the product from the catalytic system, by a simple extraction with organic solvent. Fresh substrates were then recharged to the aqueous layer of catalytic system and the mixture was heated to react once again.
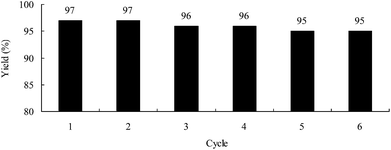 |
| Fig. 1 Repeating reactions using recovered catalytic system. The reactions were carried out with methyl(phenyl)sulfane (0.1 mol), recovered catalytic system ([C12mim][NO3], Mn(OAc)2 and H2O), in the presence of O2 gas (50 mL min−1) at 50 °C for 2 h. | |
According to the literature23 and the observations in our reactions, taking the oxidation of methyl(phenyl)sulfane with O2 as an example, a possible mechanism is proposed (Scheme 2). In the reaction, the catalyst Mn(OAc)2 provides a source of Mn(II), which reacts with the oxidant O2 in the ionic liquid [C12mim][NO3] to form O
Mn(IV)
O (2). Then, 2 reacts with the substrate to form transition state 3. 3 then very rapidly affords Mn(II) to yield the desired product. The two divalent manganese ions are then re-oxidized to O
Mn(IV)
O by O2 in [C12mim][NO3] to complete the catalytic cycle. It looks like that the formation of 3 from 2 and the substrate is the rate-determining step.
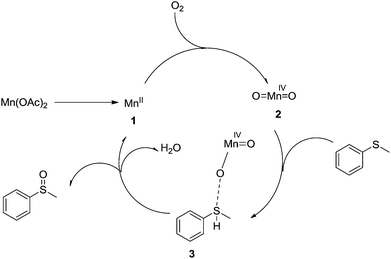 |
| Scheme 2 Possible mechanism for the oxidation of methyl(phenyl)sulfane. | |
With these results in hand, we subjected other sulfides to the oxidation reactions, and the results are listed in Table 2. It is clear that various types of aryl and alkyl sulfides, can be successfully oxidized to the corresponding sulfoxides in good to high yields (Table 2). Various types of alkyl sulfides can be successfully oxidized to the corresponding sulfoxides (Table 2, entries 9 and 10), whereas the aryl sulfides were less reactive, longer reaction time were needed to reach good yields (Table 2, entries 1–8). It was also observed that the electronic nature of the substituents on the aromatic ring have some impact on the reaction rate. Aryl sulfides, especially those with electron-donating substituents (Table 2, entries 4 and 5), were more reactive than those with or without electron-withdrawing substituents (Table 2, entries 1 and 6–8), excellent yields of the expected sulfoxides were obtained.
Table 2 Catalytic oxidation of sulfides to sulfoxidesa
Entry |
Substrate |
Product |
Time/h |
Yieldb/(%) |
Unless otherwise noted, the reactions were carried out with sulfide (0.1 mol), Mn(OAc)2 (0.2 mol), [C12mim][NO3] (20 mL), and H2O (10 mL) in the presence of O2 gas (50 mL min−1) at 50 °C. Isolated yield. |
1 |
 |
 |
2 |
97 |
2 |
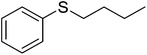 |
 |
2 |
98 |
3 |
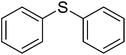 |
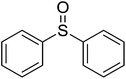 |
2 |
94 |
4 |
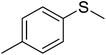 |
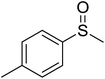 |
1.5 |
97 |
5 |
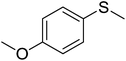 |
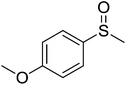 |
1.5 |
99 |
6 |
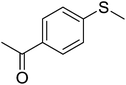 |
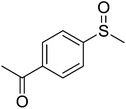 |
4 |
89 |
7 |
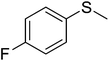 |
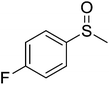 |
4 |
85 |
8 |
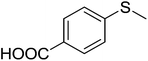 |
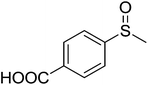 |
4 |
86 |
9 |
 |
 |
1 |
97 |
10 |
 |
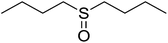 |
1 |
98 |
In conclusion, a new and highly efficient oxidation reaction system using [C12mim][NO3] in combination with Mn(OAc)2 has been developed, which is capable of converting sulfides into the corresponding sulfoxides in good to excellent isolated yield. Mild reaction conditions, simplicity of operation, high yields, stability, easy isolation of products, and excellent recyclability of the catalytic system are the attractive features of this methodology. The scope, definition of the mechanism and synthetic application of this reaction are currently under study in our laboratory.
Experimental
All the chemicals were from commercial sources without any pretreatment. All reagents were of analytical grade. The ionic liquids were synthesized according to the literature procedure.17 1H NMR spectra were recorded on a Bruker 500 MHz spectrometer using CDCl3 as the solvent with tetramethylsilane (TMS) as an internal standard. High performance liquid chromatography (HPLC) experiments were performed on a liquid chromatograph (Dionex Softron GmbH, America), consisting of a pump (P680) and ultraviolet-visible light detector (UVD) system (170U). The experiments were performed on Diacovery C18 column, ø 4.6 × 250 mm. Elemental analysis were performed on a Vario EL III instrument (Elementar Analysensyteme GmbH, Germany).
General procedure for oxidation reactions
All the three-necked flasks were loaded with sulfide (0.1 mol), Mn(OAc)2 (0.2 mol), [C12mim][NO3] (20 mL), and H2O (10 mL). O2 gas was bubbled into the flask at the flow rate of 50 mL min−1. The reaction mixture was stirred at 50 °C for an appropriate time (Table 2), the reaction progress was monitored by HPLC. The mixture was then cooled to room temperature and the organic phase was extracted with dichloromethane (3 × 20 mL). The dichloromethane solution was washed with 5% NaHCO3 (2 × 20 mL) and water (3 × 20 mL), and then dried over anhydrous Na2SO4. The solvent was removed and the residue was distilled under vacuum to give the desired pure product. The rest of the ionic liquid and the catalyst were recovered. Fresh substrates were then recharged to the recovered catalytic system and then recycled under identical reaction conditions. The target substrates were characterized by elemental analysis, 1H NMR or compared with their authentic samples. Spectroscopic data for selected products is as follows.
(Methylsulfinyl)benzene (Table 2, entry 1)
1H NMR (500 MHz, CDCl3): δ = 2.71 (s, 3H, CH3), 7.53–7.57 (m, 4H, Ar-H), 7.73 (m, 1H, Ar-H); Anal. calcd for C7H8OS: C, 59.95; H, 5.73; O, 11.42; S, 22.84. Found: C, 59.97; H, 5.75; O, 11.41; S, 22.87.
(Butylsulfinyl)benzene (Table 2, entry 2)
1H NMR (500 MHz, CDCl3): δ = 1.07 (t, 3H, CH3), 1.47–1.58 (m, 4H, CH2CH2), 2.91 (m, 2H, CH2), 7.54–7.79 (m, 5H, Ar-H); Anal. calcd for C10H14OS: C, 65.84; H, 7.71; O, 8.76; S, 17.55. Found: C, 65.89; H, 7.74; O, 8.78; S, 17.59.
Sulfinyldibenzene (Table 2, entry 3)
1H NMR (500 MHz, CDCl3): δ = 7.54–7.59 (m, 8H, Ar-H), 7.72 (m, 2H, Ar-H); Anal. calcd for C12H10OS: C, 71.23; H, 4.96; O, 7.89; S, 15.83. Found: C, 71.25; H, 4.98; O, 7.91; S, 15.85.
1-Methyl-4-(methylsulfinyl)benzene (Table 2, entry 4)
1H NMR (500 MHz, CDCl3): δ = 2.39 (s, 3H, CH3), 2.73 (s, 3H, CH3), 7.42 (m, 2H, Ar-H), 7.59 (m, 2H, Ar-H); Anal. calcd for C8H10OS: C, 62.27; H, 6.51; O, 10.34; S, 20.75. Found: C, 62.30; H, 6.54; O, 10.37; S, 20.79.
1-Methoxy-4-(methylsulfinyl)benzene (Table 2, entry 5)
1H NMR (500 MHz, CDCl3): δ = 2.71 (s, 3H, CH3), 3.87 (s, 3H, OCH3), 7.21 (m, 2H, Ar-H), 7.59 (m, 2H, Ar-H); Anal. calcd for C8H10O2S: C, 56.39; H, 5.87; O, 18.78; S, 18.83. Found: C, 56.44; H, 5.92; O, 18.80; S, 18.84.
1-(4-(Methylsulfinyl)phenyl)ethanone (Table 2, entry 6)
1H NMR (500 MHz, CDCl3): δ = 2.57 (s, 3H, CH3), 2.73 (s, 3H, CH3), 7.76 (m, 2H, Ar-H), 8.05 (m, 2H, Ar-H); Anal. calcd for C9H10O2S: C, 59.28; H, 5.51; O, 17.55; S, 17.56. Found: C, 59.32; H, 5.53; O, 17.56; S, 17.59.
1-Fluoro-4-(methylsulfinyl)benzene (Table 2, entry 7)
1H NMR (500 MHz, CDCl3): δ = 2.71 (s, 3H, CH3), 7.42 (m, 2H, Ar-H), 7.71 (m, 2H, Ar-H); Anal. calcd for C7H7FOS: C, 53.13; H, 4.45; F, 12.03; O, 10.09; S, 20.26. Found: C, 53.15; H, 4.46; F, 12.01; O, 10.11; S, 20.27.
4-(Methylsulfinyl)benzoic acid (Table 2, entry 8)
1H NMR (500 MHz, CDCl3): δ = 2.75 (s, 3H, CH3), 7.85 (m, 2H, Ar-H), 8.16 (m, 2H, Ar-H), 12.97 (br, 1H, COOH); Anal. calcd for C8H8O3S: C, 52.11; H, 4.35; O, 26.03; S, 17.38. Found: C, 52.16; H, 4.38; O, 26.06; S, 17.41.
(Ethylsulfinyl)ethane (Table 2, entry 9)
1H NMR (500 MHz, CDCl3): δ = 1.13 (t, 3H, CH3), 2.62 (dd, 2H, CH2); Anal. calcd for C4H10OS: C, 45.21; H, 9.47; O, 15.03; S, 30.18. Found: C, 45.24; H, 9.49; O, 15.07; S, 30.20.
1-(Butylsulfinyl)butane (Table 2, entry 10)
1H NMR (500 MHz, CDCl3): δ = 0.93 (t, 3H, CH3), 1.35–1.42 (m, 4H, CH2CH2), 2.55–2.63 (m, 2H, CH2), 2.67–2.72 (m, 2H, CH2); Anal. calcd for C8H18OS: C, 59.19; H, 11.19; O, 9.83; S, 19.74. Found: C, 59.21; H, 11.18; O, 9.86; S, 19.76.
Acknowledgements
We thank the Natural Science Foundation of Jiangsu Province (no. BK20140460) and the University Science Research Project of Jiangsu Province for support of this research.
References
- G. Solladie, Heteroat. Chem., 2002, 13, 443 CrossRef CAS; I. Fernández and N. Khiar, Chem. Rev., 2003, 103, 3651 CrossRef PubMed; I. Forristal, J. Sulfur Chem., 2005, 26, 163 CrossRef; R. Bentley, Chem. Soc. Rev., 2005, 34, 609 RSC; X. C. Zhao, Q. Zhang, C. Chen, B. S. Zhang, S. Reiche, A. Wang, T. Zhang, R. Schlögl and D. S. Su, Nano Energy, 2012, 1, 624 CrossRef PubMed.
- G. Pattenden and L. Roberts, Tetrahedron Lett., 1996, 37, 4191 CrossRef CAS; R. Mello, A. Olmos, A. Alcalde-Aragonés, A. Díaz-Rodríguez, M. E. G. Núñez and G. Asensio, Eur. J. Org. Chem., 2010, 6200 CrossRef; F. Gasparrini, M. Giovannoli, L. Maresca, G. Natile and G. Palmieri, Synth. Commun., 1984, 14, 1111 CrossRef; M. M. Lakouraj, M. Tajbakhsh, F. Shirini and M. V. A. Tamami, Synth. Commun., 2005, 35, 775 CrossRef PubMed; H. Tohma, S. Takizawa, H. Watanabe, Y. Fukuoka, T. Maegawa and Y. Kita, J. Org. Chem., 1999, 64, 3519 CrossRef PubMed; H. Tohma, T. Maegawa and Y. Kita, ARKIVOC, 2003, vi, 62 CrossRef; F. Ruff, A. Fábián, Ö. Farkas and Á. Kucsman, Eur. J. Org. Chem., 2009, 2102 CrossRef; V. G. Shukla, P. D. Salgaonkar and K. G. Akamanchi, J. Org. Chem., 2003, 68, 5422 CrossRef PubMed.
- M. H. Ali, D. Kriedelbaugh and T. Wencewicz, Synthesis, 2007, 3507 CrossRef CAS PubMed.
- S. S. Kim, K. Nehru, S. S. Kim, D. W. Kim and H. C. Jung, Synthesis, 2002, 2484 CrossRef CAS.
- R. Das and D. Chakraborty, Synthesis, 2011, 277 CAS.
- Z. X. Xiong, N. P. Huang and P. Zhong, Synth. Commun., 2001, 31, 245 CrossRef CAS PubMed.
- K. Bahrami, M. M. Khodaei and M. S. Arabi, J. Org. Chem., 2010, 75, 6208 CrossRef CAS PubMed; X. F. Wu, Tetrahedron Lett., 2012, 53, 4328 CrossRef PubMed; M. Kirihara, A. Itou, T. Noguchi and J. Yamamoto, Synlett, 2010, 1557 CrossRef PubMed; M. M. Khodaei, K. Bahrami and A. Karimi, Synthesis, 2008, 1682 CrossRef PubMed; S. Hussain, D. Talukdar, S. K. Bharadwaj and M. K. Chaudhuri, Tetrahedron Lett., 2012, 53, 6512 CrossRef PubMed; M. Mba, L. J. Prins and G. Licini, Org. Lett., 2007, 9, 21 CrossRef PubMed; S. Liao, I. Čorić, Q. Wang and B. List, J. Am. Chem. Soc., 2012, 134, 10765 CrossRef PubMed; M. Rahimizadeh, G. Rajabzadeh, S. M. Khatami, H. Eshghi and A. Shiri, J. Mol. Catal. A: Chem., 2010, 323, 59 CrossRef PubMed; R. Maggi, S. Chitsaz, S. Loebbecke, C. G. Piscopo, G. Sartori and M. Schwarzer, Green Chem., 2011, 13, 1121 RSC; C. Drago, L. Caggiano and R. F. W. Jackson, Angew. Chem., Int. Ed., 2005, 44, 7221 CrossRef PubMed; A. Rostami, F. Hassanian, A. Ghorbani-Choghamarani and S. Saadati, Phosphorus, Sulfur Silicon Relat. Elem., 2013, 188, 833 CrossRef; H. Egami and T. Katsuki, J. Am. Chem. Soc., 2007, 129, 8940 CrossRef PubMed; T. Yamaura, K. Kamata, K. Yamaguchi and N. Mizuno, Catal. Today, 2013, 203, 76 CrossRef PubMed.
- J. N. Moorthy, N. Singhal and K. Senapati, Tetrahedron Lett., 2008, 49, 80 CrossRef CAS PubMed; A. Y. Koposov and V. V. Zhdankin, Synthesis, 2005, 22 Search PubMed; V. Satam, A. Harad, R. Rajule and H. Pati, Tetrahedron, 2010, 66, 7659 CrossRef PubMed.
- A. Bottoni, M. Calvaresi, A. Ciogli, B. Cosimelli, G. Mazzeo, L. Pisani, E. Severi, D. Spinelli and S. Superchid, Adv. Synth. Catal., 2013, 355, 191 CrossRef CAS; W. Qian and L. Pei, Synlett, 2006, 709 CrossRef PubMed; R. S. Varma and K. P. Naicker, Org. Lett., 1999, 1, 189 CrossRef; A. Ghorbani-Choghamarani and S. Rezaei, J. Chin. Chem. Soc., 2009, 56, 251 Search PubMed; A. Ghorbani-Choghamarani and S. Sardari, Chin. J. Catal., 2010, 31, 1347 CrossRef; C. V. Reddy and J. G. Verkade, J. Mol. Catal. A: Chem., 2007, 272, 233 CrossRef PubMed; F. Bigi, H. Q. N. Gunaratne, C. Quarantelli and K. R. Seddon, C. R. Chim., 2011, 14, 685 CrossRef PubMed; P. Zhao, M. Zhang, Y. Wu and J. Wang, Ind. Eng. Chem. Res., 2012, 51, 6641 CrossRef.
- X. T. Zhou, H. B. Ji, Z. Cheng, J. C. Xu, L. X. Pei and L. F. Wang, Bioorg. Med. Chem. Lett., 2007, 17, 4650 CrossRef CAS PubMed; H. B. Ji, T. T. Wang, L. F. Wang and Y. X. Fang, React. Kinet. Catal. Lett., 2007, 90, 259 CrossRef; H. Tanaka, H. Nishikawa, T. Uchida and T. Katsuki, J. Am. Chem. Soc., 2010, 132, 12034 CrossRef PubMed.
- R. Aldea and H. Alper, J. Org. Chem., 1995, 60, 8365 CrossRef CAS; W. P. To, Y. Liu, T. C. Lau and C. M. Che, Chem.–Eur. J., 2013, 19, 5654 CrossRef PubMed.
- S. E. Martin and L. I. Rossi, Tetrahedron Lett., 2001, 42, 7147 CrossRef CAS; N. M. Okun, J. C. Tarr, D. A. Hilleshiem, L. Zhang, K. I. Hardcastle and C. L. Hill, J. Mol. Catal. A: Chem., 2006, 246, 11 CrossRef PubMed; B. Li, A. H. Liu, L. N. He, Z. Z. Yang, J. Gao and K. H. Chen, Green Chem., 2012, 14, 130 RSC.
- B. M. Choudary, C. R. V. Reddy, B. V. Prakash, M. L. Kantam and B. Sreedhar, Chem. Commun., 2003, 754 RSC.
- E. Boring, Y. V. Geletii and C. L. Hill, J. Am. Chem. Soc., 2001, 123, 1625 CrossRef CAS PubMed.
- S. Colonna, N. Gaggero, F. Montanari, G. Pozzi and S. Quici, Eur. J. Org. Chem., 2001, 181 CrossRef CAS; Y. Imada, H. Iida, S. Ono, Y. Masui and S. I. Murahash, Chem.–Asian J., 2006, 1, 136 CrossRef PubMed; Y. Ishii, S. Sakaguchi and T. Iwahama, Adv. Synth. Catal., 2001, 343, 393 CrossRef; A. H. Sun, Z. G. Xiong and Y. M. Xu, J. Mol. Catal. A: Chem., 2006, 259, 1 CrossRef PubMed; Y. Imada, H. Iida, S. Ono and S. I. Murahashi, J. Am. Chem. Soc., 2003, 125, 2868 CrossRef PubMed; H. Claudia, T. Marion and J. Manuela, Chem. Geol., 2013, 342, 29 CrossRef PubMed.
- F. Dommert, K. Wendler, R. Berger, L. D. Site and C. Holm, ChemPhysChem, 2012, 13, 1625 CrossRef CAS PubMed; O. Andreussi and N. Marzari, J. Chem. Phys., 2012, 137, 044508 CrossRef PubMed; M. Petkovic, K. R. Seddon, L. P. N. Rebelo and C. S. Pereira, Chem. Soc. Rev., 2011, 40, 1383 RSC.
- S. J. Zhang and X. M. Lu, Ionic liquids: from fundamental research to industrial applications, Science Press, Beijing, 2006 Search PubMed.
- M. D. Joshi and J. L. Anderson, RSC Adv., 2012, 2, 5470 RSC; P. Sun and D. W. Armstrong, Anal. Chim. Acta, 2010, 661, 1 CrossRef CAS PubMed; S. Stefan and S. Piotr, Curr. Org. Chem., 2011, 15, 1871 CrossRef.
- N. V. Plechkova and K. R. Seddon, Chem. Soc. Rev., 2008, 37, 123 RSC; P. Wasserschein and T. Welton, Ionic liquids in synthesis, Wiley-VCH, Weinheim, 2008 Search PubMed; J. M. Andanson, S. Marx and A. Baiker, Catal. Sci. Technol., 2012, 2, 1403 Search PubMed; A. Rouch, T. Castellan, I. Fabing, N. Saffon, J. Rodriguez, T. Constantieux, J. C. Plaquevent and Y. Génisson, RSC Adv., 2013, 3, 413 RSC.
- F. van Rantwijk, R. M. Lau and R. A. Sheldon, Trends Biotechnol., 2003, 21, 131 CrossRef CAS; N. Wood, J. L. Ferguson, H. Q. N. Gunaratne, K. R. Seddon, R. Goodacre and G. M. Stephens, Green Chem., 2011, 13, 1843 RSC.
- A. Balducci, W. A. Henderson, M. Mastragostino, S. Passerini, P. Simon and F. Soavi, Electrochim. Acta, 2005, 50, 2233 CrossRef CAS PubMed; P. Hapiot and C. Lagrost, Chem. Rev., 2008, 108, 2238 CrossRef PubMed.
- S. Saravanamurugan and A. Riisager, Catal. Today, 2013, 200, 94 CrossRef CAS PubMed; V. I. Parvulescu and C. Hardacre, Chem. Rev., 2007, 107, 2615 CrossRef PubMed; H. Olivier-Bourbigou, L. Magna and D. Morvan, Appl. Catal., A, 2010, 373, 1 CrossRef PubMed; Q. Zhang, S. Zhang and Y. Deng, Green Chem., 2011, 13, 2619 RSC; M. Bagherzadeh and S. Ghazali-Esfahani, Tetrahedron Lett., 2013, 54, 3765 CrossRef PubMed.
- C. C. Cosner, P. J. Cabrera, K. M. Byrd, A. M. Thomas and P. Helquist, Org. Lett., 2011, 13, 2071 CrossRef CAS PubMed; J. A. Gonçalves and E. V. Gusevskaya, Appl. Catal., A, 2004, 258, 93 CrossRef PubMed; M. Moghadam, S. Tangestaninejad, V. Mirkhani, I. Mohammadpoor-Baltork and A. A. Abbasi-Larki, Appl. Catal., A, 2008, 349, 177 CrossRef PubMed; A. M. Kirillov and G. B. Shul'pin, Coord. Chem. Rev., 2013, 257, 732 CrossRef PubMed; S. Lentini, P. Galloni, I. Garcia-Bosch, M. Costas and V. Conte, Inorg. Chim. Acta, 2014, 410, 60 CrossRef PubMed; M. Mondal and U. Bora, RSC Adv., 2013, 3, 18716 RSC; M. M. Najafpour, B. Pashaei and S. Nayeri, Dalton Trans., 2012, 41, 4799 RSC.
|
This journal is © The Royal Society of Chemistry 2014 |