DOI:
10.1039/C4RA03675A
(Paper)
RSC Adv., 2014,
4, 25795-25803
Novel green fatty acid-based bis-cyclic carbonates for the synthesis of isocyanate-free poly(hydroxyurethane amide)s†
Received
22nd April 2014
, Accepted 3rd June 2014
First published on 3rd June 2014
Abstract
Fatty acid-based bis-cyclic 5-membered carbonates containing amide linkages were prepared from methyl 10-undecenoate. The reaction in bulk of these bio-based carbonates with a series of di-amines led to poly(hydroxyurethane amide)s with molar masses up to 31
000 g mol−1. As expected, the so-formed bio-based thermoplastic poly(hydroxyurethane)s exhibit amorphous to semi-crystalline features with respect to the chemical structure of the monomers used.
Introduction
Despite the interest in developing renewable diols (or polyols),1–4 the use of toxic (poly-)isocyanates, manufactured from amine and phosgene, remains a matter to settle in the chemistry of polyurethanes.5–8 Therefore, the need to find alternative routes for the synthesis of PUs which avoid the use of isocyanate is of high importance. Several ways are considered to produce more sustainable non isocyanate polyurethanes (NIPU) from vegetable oil derivatives, such as: (i) the ring-opening of cyclic carbonates by amines,9–11 (ii) the transurethane process12–15 and (iii) the self-condensation method based on the Curtius rearrangement in which the AB-type monomer contains both hydroxyl and acyl azide groups.16,17 The reaction of cyclic carbonates with amines has emerged as the most promising non-isocyanate route leading to poly(hydroxyurethane)s (PHUs).5,7,8 The PHUs present specific properties, in comparison to those of classical PUs, notably due to the presence of the hydroxyl functions generated along with the polymerization.
The 5-membered cyclic carbonate can be generated efficiently through functionalization of the triglyceride double bonds. The epoxidation/carbonation strategy is a well-known and efficient procedure to prepare 5-membered cyclic carbonates from olefins. Research groups have intensely investigated poly(hydroxyurethane) networks from carbonated vegetable oils. Only one example of fatty acid-based thermoplastic poly(hydroxyurethane) has been reported so far in the literature.18 Besides, the vegetable oil-based cyclic carbonates are usually bearing ester groups due to the inherent structure of the triglycerides. However, the occurrence of amidation side reactions has been demonstrated in some cases.7,19–21 For instance, Javni et al. clearly demonstrated that during the curing of the poly(hydroxyurethane) networks, the amine groups can react with the ester functions to form amide linkages.21 Hence, cyclic carbonate compounds without ester linkages would be favored.
This paper is thus dedicated to the design of novel fatty acid-based bis-cyclic carbonates bearing mainly amide linkages in their structure with the idea to prepare non-isocyanate and non-phosgene thermoplastic poly(hydroxyurethane amide)s with high molar masses and glass transition temperatures. FTIR-ATR, NMR, SEC and DSC were performed to investigate the PHUs chemical structures, molar masses, thermal properties and thermal stabilities.
Monomer synthesis
To synthesize linear PHUs from fatty acid derivatives, five bis-cyclic carbonates were prepared starting from methyl undecenoate by (i) transesterification and/or amidation followed by (ii) epoxidation and (iii) carbonation reaction. The Fig. 1 illustrates the chemical structures of the synthesized bis-cyclic carbonates. The spacers of the cyclic carbonate dimers were of different nature so as to design PHUs with different thermal properties. One cyclic carbonate dimer presents two ester groups (UndPdE-b5CC) and the others have two amide linkages. Amide functions were introduced into the cyclic carbonates owing to their ability to form strong hydrogen bonds and also to replace ester functions, which can lead to side reactions during polymerization. Among the diamide bis-cyclic carbonates, UndBdA-b5CC is issued from butane-1,4-diamine, which allows the formation of hydrogen bonds with the CONH group. In order to obtain diamide bis-cyclic carbonates with lower melting point, piperazine (UndPipdA-b5CC), N,N′-dimethylpropane-1,3-diamine (UndPMedA-b5CC) and N,N′-dihexyldecane-1,10-diamine (UndDHexdA-b5CC) were used as spacers. As it has been reported in literature that internal cyclic carbonates were less reactive than terminal ones,18 methyl undecenoate was thus preferred as starting material. The chemical structure of the synthesized cyclic carbonates dimers and intermediates were evaluated by 1H and 13C NMR and FTIR-ATR spectroscopies.
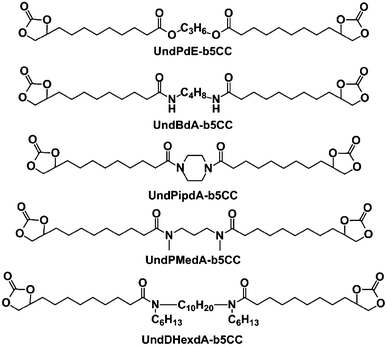 |
| Fig. 1 Chemical structures of the synthesized cyclic carbonates dimers. Abbreviations used are as follows: [Und = from methyl undecenoate]; [P = propyl, B = butyl, Pip = from piperazine, PMe = from N,N′-dimethylpropane-1,3-diamine, DHex = from N,N′-dihexyldecane-1,10-diamine]; [d = di-] and [E = ester, A = amide, b5CC = bis 5-membered cyclic carbonate]. | |
The Scheme 1 illustrates the synthesis of UndBdA-b5CC from methyl undecenoate and butane-1,4-diamine (see ESI† for the synthesis of UndPdE-b5CC). The syntheses of UndPipdA-b5CC, UndPMedA-b5CC and UndDHexdA-b5CC have been carried out in the same way with slight variations of the catalyst quantity, the solvent, the temperature and the pressure (see ESI†).
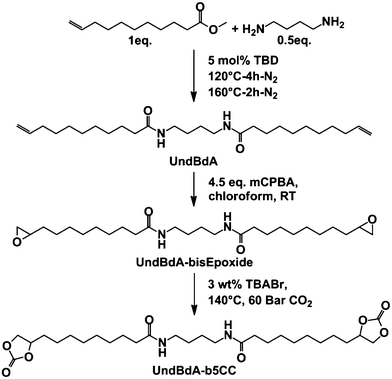 |
| Scheme 1 Synthetic strategy to UndBdA-b5CC from methyl undecenoate, butane-1,4-diamine and CO2. | |
In the specific case of UndDHexdA-b5CC, the N,N′-dihexyldecane-1,10-diamine (SebHex-diamine) was first prepared by the reduction of the corresponding diamide, itself obtained from sebacoyl chloride and hexylamine. Then, the reaction between SebHex-diamine and methyl undecenoate was investigated but no conversion was observed probably due to the lower reactivity of the SebHex-diamine. This observation led to the use of more reactive undecenyl chloride instead of methyl undecenoate.
In all cases, the amidation reactions were monitored by means of FTIR-ATR and 1H NMR spectroscopies (see ESI†). IR spectroscopy of UndBdA showed two absorption bands at 1630 cm−1 and 1540 cm−1, whereas, as expected, UndPipdA, UndPMedA and UndDHexdA FTIR-ATR spectra presents only the amide carbonyl stretching vibration in the range 1650 cm−1 to 1640 cm−1. The ester carbonyl stretching (O
C–O) of the methyl undecenoate at 1720 cm−1 disappeared during all diamide syntheses. The UndBdA displayed also a band at 3295 cm−1 characteristic of N–H stretching vibrations (see ESI†). When necessary, the diamide was purified by flash chromatography to remove the unreacted methyl undecenoate and the monoamide formed.
As an example, the stacked 1H NMR spectra of the different steps for the synthesis of UndPipdA-b5CC are given in Fig. 2. The formation of the amide functions was confirmed in 1H NMR, by the appearance of a triplet at 2.32 ppm, corresponding to the protons nearby the (O
C)–NH group. Moreover, the singlet at 3.66 ppm, which is characteristic of the ester moiety of methyl undecenoate, has disappeared.
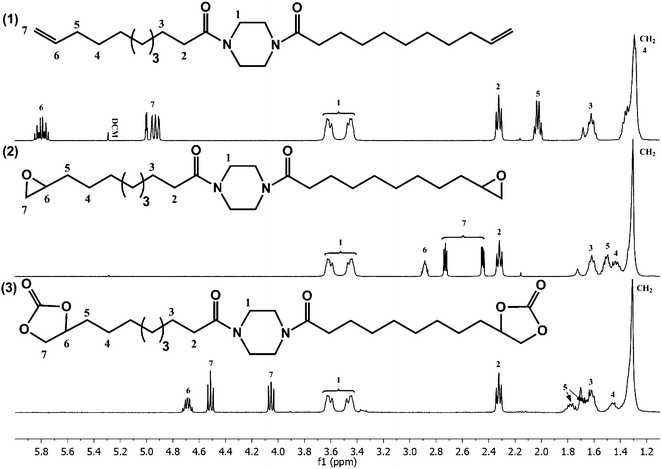 |
| Fig. 2 Stacked 1H NMR spectra of (1) UndPipdA, (2) UndPipdA-bisEpoxide and (3) UndPipdA-b5CC. (Analyses in CDCl3 at room temperature.) | |
The epoxidation reactions of the bis-unsaturated precursors were performed with m-CPBA (meta-chloroperoxybenzoic acid) according to the previous literature.18 The reaction progress was followed by the disappearance of olefinic protons by 1H NMR spectroscopy. The synthesis of the epoxide was attested by the formation of the epoxide characteristic peaks, e.g. multiplets at 2.88 ppm, 2.73 ppm and 2.45 ppm (protons H6 and H7). After completion of the epoxidation, the reaction mixture was then successively washed with aqueous sodium sulfite, aqueous sodium bicarbonate and water to remove excess of m-CPBA.
Various reaction conditions have been used for the carbonation of epoxide. After in situ FTIR investigations to monitor the kinetics of the carbonation reaction,22–24 (see ESI†) the following procedure has been chosen. After 24 hours, full conversion was attained for all fatty acid-based bis epoxides using the following reaction conditions: 80 °C/50 Bar for UndPdE-bisEpoxide, UndPMedA-bisEpoxide bis-epoxide and UndPipdA-b5CC clearly demonstrates the formation of the cyclic carbonate. (See protons H6 and UndDHexdA-bisEpoxide, 135 °C/50 Bar for UndPipdA-bisEpoxide and 140 °C/60 Bar for UndBdA-bisEpoxide.)
After carbonation reactions, a band in the range 1795 cm−1 to 1775 cm−1, corresponding to the carbonyl vibration of the cyclic carbonate was visible for all synthesized 5-membered cyclic carbonates (see ESI†). The formation of the cyclic carbonate was also confirmed by 1H NMR (see ESI†) For instance, the 1H NMR spectra of UndPipdA-b5CC is given in Fig. 2(3) (see H6 and H7).
The purity (when possible) and melting points of the monomers, as well as the HSQC (Heteronuclear single quantum coherence)-NMR analysis for UndPipdA-b5CC are given in ESI.† Amide-containing cyclic carbonates showed higher melting points than UndPdE-b5CC. While removing the possibility of H-bond formation and bringing flexibility thanks to pendent groups/chains to the spacer, lower melting points were observed. The global yields over the three steps were in accordance with green chemistry (see Table 1 in ESI†) and syntheses on several grams scale were achievable.
Poly(hydroxyurethane amide)s
To prepare a wide range of fatty acid-based isocyanate-free PHUs, the synthesized bis-cyclic carbonates were used in polyaddition processes with four diamines; butane-1,4-diamine (4DA), isophorone diamine (IPDA), the Priamine 1075 (a dimer of fatty acid from CRODA) and Jeffamine 400 (an amino-telechelic polyether with a molar mass of 400 g mol−1). The diamines IPDA, Priamine 1075 and Jeffamine 400 were used to introduce flexibility in the PHUs, by increasing the free volume between the polymer chains. The Scheme 2 illustrates the synthesis of PHUs. First, polymerization tests were carried out in solvent, but the reactions were really too slow. Polymerizations were then performed in bulk, thus avoiding further treatment to recover the solvent. Thus, polyadditions were carried out at a temperature depending on the melting point of the bis-cyclic carbonate used; 140 °C (for UndBdA-b5CC and UndPipdA-b5CC), 120 °C (for UndPMedA-b5CC and UndDHexdA-b5CC) and 70 °C or 110 °C/120 °C (for UndPdE-b5CC). The potential catalysis of the reaction was also investigated (see ESI†) and none of them show dramatic improvement as compared to a catalyst-free polymerization.
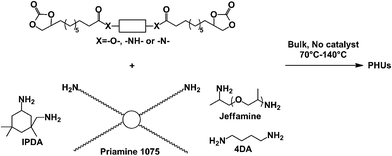 |
| Scheme 2 Synthesis of fatty acid-based poly(hydroxyurethane)s. | |
Even at high temperature, the blends were not fully homogeneous while using UndBdA-b5CC or IPDA, due to the hydrogen bonds and cyclo-aliphatic structure of the monomers used. The polymerizations were monitored with FTIR-ATR. PHUs were obtained as brown to yellow viscous to solid compounds. Table 1 gives the experimental details along with the abbreviations used for the PHUs, as well as the polymerizations results.
Table 1 Molar masses and dispersities of the PHUs from 5-membered cyclic carbonate dimers and diamines polymerized in bulka
Sample |
Used b5CC |
Diamine |
Temperature (°C) |
Time |
Conversiona (%) |
nb (g mol−1) |
Đb |
Calculated by FTIR-ATR using the equation: , where x, t, HCC and HAd are the conversion, the time, the height of the peaks corresponding to the cyclic carbonate and amide (or ester) carbonyls respectively. SEC in DMF with 1 wt% LiBr – calibration PS standards. The given data correspond to the SEC analysis at different reaction times of the full sample. Highly multi modal molar masses. ns = not soluble and nd = not determined. |
PHU-dE-1 |
UndPdE-b5CC |
4DA |
70 |
1 day |
95.1 |
25 400 |
1.6 |
3 day |
95.6 |
29 800 |
1.8 |
7 day |
96.4 |
30 400 |
2.5 |
PHU-BdA-1 |
UndBdA-b5CC |
IPDA |
140 |
5 h |
64.1 |
15 300 |
1.3 |
13 day |
97.6 |
18 900 |
2.4 |
PHU-BdA-2 |
Priamine |
140 |
5 h |
97.6 |
14 900 |
1.5 |
PHU-BdA-3 |
Jeffamine |
140 |
5 h |
84.3 |
14 600 |
1.3 |
6 day |
98.9 |
20 900 |
2.6 |
PHU-PipdA-1 |
UndPipdA-b5CC |
IPDA |
140 |
5 h |
76.3 |
16 500 |
1.5 |
1 day |
87.0 |
18 200 |
1.5 |
PHU-PipdA-2 |
Priamine |
140 |
5 h |
91.9 |
19 300 |
1.7 |
1 day |
93.2 |
19 200 |
1.7 |
PHU-PipdA-3 |
Jeffamine |
140 |
5 h |
46.7 |
11 200 |
1.2 |
3 day |
91.5 |
23 300 |
2.9 |
PHU-PMedA-1 |
UndPMedA-b5CC |
IPDA |
120 |
5 h |
53.5 |
11 000 |
1.2 |
12 day |
91.5 |
31 100 |
2.2 |
PHU-PMedA-2 |
Priamine |
120 |
5 h |
87.6 |
mmc |
mmc |
6 day |
94.4 |
28 700 |
1.7 |
PHU-PMedA-3 |
Jeffamine |
120 |
5 h |
12.4 |
mmc |
mmc |
6 day |
84.1 |
20 700 |
1.4 |
PHU-DHexdA-1 |
UndDHexdA-b5CC |
IPDA |
120 |
1 day |
ns |
ns |
ns |
PHU-DHexdA-2 |
Priamine |
120 |
1 day |
nd |
nd |
nd |
PHU-DHexdA-3 |
Jeffamine |
120 |
1 day |
nd |
nd |
nd |
PHU-DHexdA-4 |
4DA |
120 |
1 day |
nd |
nd |
nd |
The polymer chemical structures were assessed by FTIR-ATR and 1H NMR spectroscopies. The appearance of bands around 1700 cm−1 and 1540 cm−1, corresponding to the vibrations of (O
C)–NH and C–N respectively, attested to the formation of urethane linkage. Besides, a large band attributed to the NH and OH vibrations were observed in the region 3660 cm−1 to 3120 cm−1. The ester and amide moieties of the bis-cyclic carbonate precursor were well preserved even at high temperature. The FTIR-ATR spectra of PHU-BdA-1, PHU-PipdA-1, PHU-PMedA-1 and PHU-DHexdA-1 are given in ESI.† As illustrated in Fig. 3, the synthesis of PHU was assessed by 1H NMR by the formation of a clearly visible peak at 3.15 ppm. Besides, the peaks corresponding to the cyclic carbonates decreased with conversion. The signals corresponding to the hydroxyl urethanes could be attributed that revealed the balanced formation (50
:
50) of primary and secondary alcohols. As an example, for PHU-PipdA-2, the ratio between the formation of primary and secondary alcohols was 43.8
:
56.2 (see Fig. 3). Concerning the potential side reaction between the amine and the ester or amide functions, 1H NMR of PHU-PipdA-2 testified that no transamidification took place during the polymerization. It can be noticed from Table 1 that conversions were relatively fast and reached values of 60–95% after 5 hours. The polymerizations with Priamine 1075 were much faster than with IPDA and Jeffamine, probably due to the unhindered character of the amine.
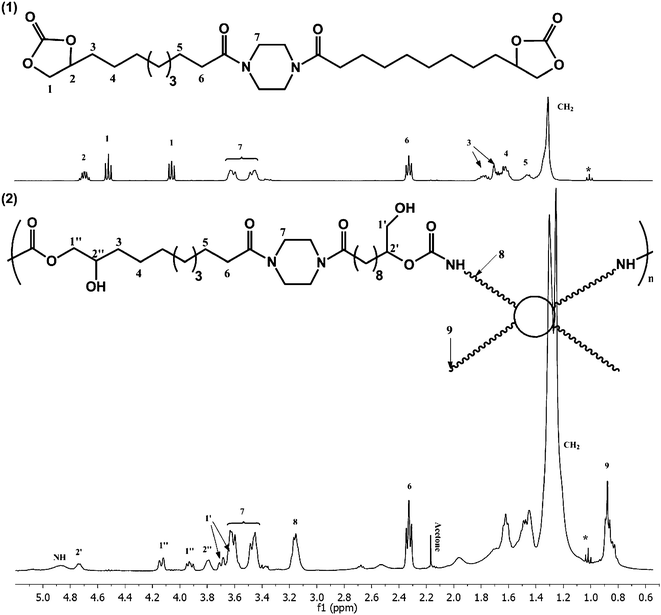 |
| Fig. 3 Stacked 1H NMR spectra of PHU-PipdA-2. (*) TBABr. (Analyses in CDCl3.) | |
SEC data, which are exposed in Table 1, indicate the formation of PHUs with molar masses in the range 11
000 to 31
000 g mol−1. However, the molar mass values provided by SEC should not be taken as absolute values as the SEC calibration was carried out in DMF using PS standards (see ESI† for SEC analysis of PHU-PMedA-2). The molar mass dispersities were in the range of 1.2 to 2.9. The molar masses data given in Table 1 correspond to the main peak observable in SEC. However, in almost all analyses, a smaller peak around 4000 g mol−1 can be detected and could be attributed to the presence of cycles.
The fatty acid-based cyclic carbonate dimers demonstrate low reactivity, even at high temperatures. Indeed, cyclic carbonates are known to be stabilized by the +I inductive effect of the alkyl chain.25,26 Thus, no full conversions were achieved even after long polymerization times. Moreover, after long times at high temperature, side reactions could occur. In FTIR-ATR, those side reactions could be attributed to ester or dialkyl carbonate formation (see ESI† for PHU-PMedA-2). The appearance of side reactions is in good agreement with the slight decrease of the molar masses and the glass transition temperatures (see ESI†).
Thermal properties
The thermal properties of the synthesized PHUs are well correlated with their chemical structure. Table 2 summarized the glass transition and melting temperatures of the PHUs. Amorphous PHUs were obtained with UndPipdA-b5CC, UndPMedA-b5CC and UndDHexdA-b5CC. The cyclic structure of the spacer of UndPipdA-b5CC led to higher Tg. Moreover, the longer the alkyl dangling group (or chain), the lower the glass transition (see PHU-PipdA-1, PHU-PMedA-1 and PHU-DHexdA-1 in Fig. 4) The use of a cyclic aliphatic amine induced the formation of a PHU with a glass transition temperature of 39.9 °C. However, while using Priamine, a lower glass transition temperature (2.4 °C) was obtained. Indeed, the resulting dangling chains in the polymer backbone, plasticizes the so-formed PHU. The PHU-BdA-3 with Jeffamine (400 g mol−1) as a co-monomer reached an even lower glass transition of −21 °C, which can be explained by the polyether nature of the Jeffamine.
Table 2 Thermal properties of the synthesized PHUsa
Sample |
Time |
Tga (°C) |
Tma (°C) |
Determined by DSC at 10 °C min−1. With crystallization upon heating. |
PHU-dE-1 |
1 day |
−17 |
— |
PHU-BdA-1 |
13 day |
40 |
115b |
PHU-BdA-2 |
6 day |
2 |
115 |
PHU-BdA-3 |
6 day |
−21 |
109 |
PHU-PipdA-1 |
6 day |
55 |
— |
PHU-PipdA-2 |
1 day |
−2 |
— |
PHU-PipdA-3 |
6 day |
−15 |
— |
PHU-PMedA-1 |
6 day |
32 |
— |
PHU-PMedA-2 |
1 day |
−4 |
— |
PHU-PMedA-3 |
6 day |
−17 |
— |
PHU-DHexdA-1 |
6 day |
3 |
— |
PHU-DHexdA-2 |
1 day |
−18 |
— |
PHU-DHexdA-3 |
6 day |
−29 |
— |
PHU-DHexdA-4 |
1 day |
−13 |
— |
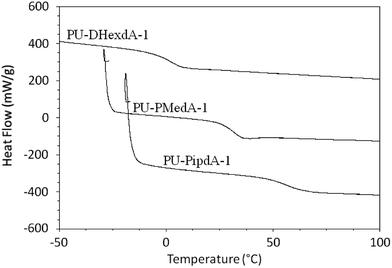 |
| Fig. 4 DSC traces of amorphous PHU-PipdA-1, PHU-PMedA-1 and PHU-DHexdA-1. | |
With UndBdA-b5CC as a co-monomer, the PHUs obtained were semi-crystalline in nature. The presence of hydrogen bonds from the amide linkages of UndBdA-b5CC favored interactions between polymer chains and thus the crystallization of the resulting PHUs. The effect of the chemical structure of the diamine can be observed in Fig. 5 with UndBdA-b5CC. Fig. 5 shows the DSC traces of the semi-crystalline PHUs based on UndBdA-b5CC with various diamines.
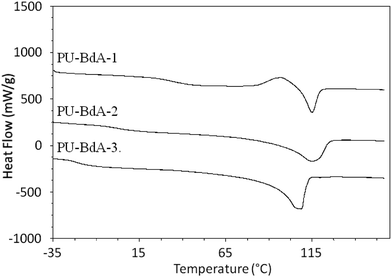 |
| Fig. 5 DSC thermograms after second heating of semi-crystalline PHU-BdA-1, PHU-BdA-2 and PHU-BdA-3. | |
Experimental methods
Synthesis and characterizations of cyclic carbonates and their polyhydroxyurethane
1 Transesterification and amidation reactions.
Transesterification step. Methyl undecenoate (20 g, 101 mmol), propanediol (3.8 g, 50 mmol), TBD (702 mg, 5 mmol) (1
:
0.5
:
0.05) were stirred under nitrogen flow at 120 °C (4 h), at 160 °C (2 h) then under vacuum at 160 °C (30 min). The reaction mixture was dissolved in ethyl acetate (200 mL) and washed with water (3 × 50 mL). The organic layer was dried over anhydrous sodium sulfate, filtered and solvent was removed on rotary evaporator. Yield = 73%. UndPdE: 1H NMR (CDCl3, 25 °C, 400 MHz) δ (ppm): 5.78 (m, 2H), 4.93 (m, 4H), 4.12 (t, 4H), 2.27 (t, 4H), 2.02 (m, 4H), 1.93 (m, 2H), 1.59 (m, 4H), 1.34 (m, 4H), 1.26 (m, 16H). IR (cm−1): 2924, 2853, 1735, 1161, 906.
Amidation step. The diamides were prepared with different method according to the chemical structure of the diamine.Methyl undecenoate (20 g, 101 mmol), butane-1,4-diamine (4.4 g, 50 mmol) and TBD (702 mg, 5 mmol) (1
:
0.5
:
0.05) were stirred under nitrogen flow at 120 °C (4 h) then at 160 °C (2 h). The reaction flask was cooled down at 90 °C and NMP (60 mL) was added to end up with an homogeneous phase. The required UndBdA was slowly precipitated by reaching room temperature. A filtration and washes with methanol were performed. Yield = 83%. UndBdA: 1H NMR (CDCl3, 50 °C, 400 MHz) δ (ppm): 5.79 (m, 2H), 4.98 (m, 4H), 3.26 (m, 4H), 2.15 (t, 4H), 1.99 (m, 4H), 1.65 (m, 4H), 1.53 (m, 4H), 1.40 (m, 4H), 1.32 (m, 16H). IR (cm−1): 3295, 2918, 2847, 1630, 1537.
For UndPipdA and UndPMedA diamides, the amidation reactions were as followed. Methyl undecenoate, diamine and TBD (1
:
0.5
:
0.05) were stirred in a round-bottom flask equipped with a bubbling system under inert atmosphere at 100 °C (2 h), then under nitrogen flow at 120 °C (4 h) and at 160 °C (2 h). The diamides were purified by column chromatography and obtained as a yellow viscous liquid.
UndPipdA.
Methyl undecenoate (20 g, 101 mmol), piperazine (4.3 g, 50 mmol) and TBD (702 mg, 5 mmol). UndPipdA was purified by column chromatography to eliminate completely the monoamide (eluent: cyclohexane–ethyl acetate with increasing percentage of ethyl acetate from 20% to 60%). Yield = 68.2%. 1H NMR (CDCl3, 25 °C, 400 MHz) δ (ppm): 5.79 (m, 2H), 4.94 (m, 4H), 3.62 (m, 4H), 3.44 (m, 4H), 2.32 (t, 4H), 2.03 (m, 4H), 1.63 (m, 4H), 1.35–1.29 (m, 20H). IR (cm−1): 2918, 2847, 1650, 906.
UndPMedA.
Methyl undecenoate (20 g, 101 mmol), N,N′-dimethylpropane-1,3-diamine (5.2 g, 50 mmol) and TBD (702 mg, 5 mmol). UndPMedA was purified by column chromatography to eliminate completely the monoamide (eluent: heptane–ethyl acetate with increasing percentage of ethyl acetate from 20% to 60%). Yield = 79.3%. 1H NMR (CDCl3, 25 °C, 400 MHz) δ (ppm): 5.80 (m, 2H), 4.95 (m, 4H), 3.35–3.25 (m, 4H), 2.98–2.89 (s, 6H), 2.26 (m, 4H), 2.01 (m, 4H), 1.75 (m, 2H), 1.59 (m, 4H), 1.34–1.28 (m, 20H). IR (cm−1): 2924, 2850, 1639, 906.
Concerning the preparation of UndDHexdA, the diamine (SebHex-diamine) used as central block was synthesized in a first step, and then the amidation was performed. The SebHex-diamine was obtained by the reduction of the corresponding diamide issued from sebacoyl chloride and hexylamine. Hexylamine (9 g, 86.4 mmol, 2.05 eq.), triethylamine (20.7 mL, 143.3 mmol, 3.41 eq.), then chloroform (125 mL) were introduced in a round-bottom flash. Afterwards, the sebacoyl chloride (10 g, 42 mmol, 1 eq.) was added dropwise. The formation of a white precipitate due to the generation of triethylamine hydrochloride salt revealed the progress of the reaction. After filtration and washes with hot water, the organic phase was dried over anhydrous sodium sulfate, filtered and the chloroform was removed on rotary evaporator. After drying, the diamide was reduced by LiAlH4 (3 eq. per function) in dried THF under reflux overnight. Then, an aqueous solution of potassium sodium tartrate at 1 mol L−1 (200 mL) was added to the reaction mixture placed into an ice bath. The SebHex-diamine was recovered after filtration followed by extraction of the filtrate with ethyl acetate. SebHex-diamine was purified by column chromatography (eluent: ethyl ether–methanol with increasing percentage of methanol from 0% to 31%). Yield = 84.6%. SebHex-diamine: 1H NMR (CDCl3, 25 °C, 400 MHz) δ (ppm): 2.57 (1, 8H), 1.46 (m, 8H), 1.27 (m, 24H), 0.81 (t, 6H).
To SebHex-diamine (8.4 g, 25 mmol), dried THF (100 mL) and triethylamine (5.5 g, 55 mmol, 1.1 eq.) were added. Then undecenyl chloride (10 g, 49 mmol) was added dropwise. The reaction mixture was then stirred at room temperature for 2 hours. UndDHexdA was purified by filtration of the formed salt, followed by column chromatography to eliminate completely the monoamide (eluent: heptane–ethyl acetate (95/5)). Yield = 91.3%. UndDHexdA: 1H NMR (CDCl3, 50 °C, 400 MHz) δ (ppm): 5.80 (m, 2H), 4.94 (m, 4H), 3.27 (m, 4H), 3.19 (m, 4H), 2.27 (t, 4H), 2.02 (m, 4H), 1.62 (m, 4H), 1.51 (m, 8H), 1.36–1.28 (m, 40H), 0.88 (m, 6H). IR (cm−1): 2924, 2851, 1642, 906.
2 Epoxidation reaction. The diester or diamide and m-CPBA (3 eq. and 4.5 eq. for UndBdA) were stirred at room temperature in DCM (20 mL g−1 of product) or chloroform (for UndBdA). After 1 day, the conversion of the double bonds, monitored by 1H NMR spectroscopy, were in the range 84% to 100%. The reaction mixture was then thoroughly washed with aqueous Na2SO3 (3 × 50 mL), aqueous NaHCO3 (4 × 50 mL) and water (4 × 50 mL) until neutral pH. The organic layer was dried over anhydrous sodium sulfate filtered and solvent was removed on rotary evaporator to obtain the bis epoxides.
UndPdE-bisEpoxide. UndPdE (17 g, 42 mmol) and m-CPBA (21.5 g, 125 mmol, 3 eq.). The purity of UndPdE-bisEpoxide (92.8%) was determined by GC-FID. Yield = 95.9%. 1H NMR (CDCl3, 25 °C, 400 MHz) δ (ppm): 4.12 (t, 4H), 2.90 (m, 2H), 2.74 (t, 2H), 2.45 (m, 2H), 2.29 (t, 4H), 1.95 (m, 2H), 1.61 (m, 4H), 1.51 (m, 4H), 1.44 (m, 4H), 1.30 (m, 16H). IR (cm−1): 2927, 2856, 1732, 1161.
UndBdA-bisEpoxide. UndBdA (12.7 g, 30 mmol) and m-CPBA (23.4 g, 136 mmol, 4.5 eq.). The purity of UndBdA-bisEpoxide (80.4%) was determined by GC-FID. Yield = 97%. 1H NMR (CDCl3, 25 °C, 400 MHz) δ (ppm): 5.79 (s, 2NH), 3.26 (m, 4H), 2.89 (m, 2H), 2.74 (t, 2H), 2.46 (m, 2H), 2.16 (t, 4H), 1.71 (m, 4H), 1.61–1.53 (m, 12H), 1.31 (m, 16H). IR (cm−1): 3292, 2912, 2851, 1631, 1537.
UndPipdA-bisEpoxide. UndPipdA (13.2 g, 31 mmol) and m-CPBA (16.3 g, 95 mmol, 3 eq.). The purity of UndPipdA-bisEpoxide (97.9%) was determined by GC-FID. Yield = 84.5%. 1H NMR (CDCl3, 25 °C, 400 MHz) δ (ppm): 3.61 (m, 4H), 3.45 (m, 4H), 2.88 (m, 2H), 2.73 (t, 2H), 2.45 (m, 2H), 2.32 (t, 4H), 1.61 (m, 4H), 1.49–1.44 (m, 8H), 1.34–1.30 (m, 16H). IR (cm−1): 2913, 2848, 1651.
UndPMedA-bisEpoxide. UndPMedA (20 g, 46 mmol) and m-CPBA (23.8 g, 138 mmol, 3 eq.). The purity of UndPMedA-bisEpoxide (93.9%) was determined by GC-FID. Yield = 83.9%. 1H NMR (CDCl3, 25 °C, 400 MHz) δ (ppm): 3.37 (m, 4H), 3.00–2.92 (s, 6H), 2.90 (m, 2H), 2.75 (t, 2H), 2.46 (m, 2H), 2.31 (m, 4H), 1.79 (m, 2H), 1.61 (m, 4H), 1.51 (m, 4H), 1.42 (m, 4H), 1.29 (m, 16H). IR (cm−1): 2924, 2854, 1639.
UndDHexdA-bisEpoxide. UndDHexdA (10 g, 15 mmol) and m-CPBA (7.7 g, 44 mmol, 3 eq.). Yield = 54.4%. 1H NMR (CDCl3, 25 °C, 400 MHz) δ (ppm): 3.28 (m, 4H), 3.19 (m, 4H), 2.89 (m, 2H), 2.73 (t, 2H), 2.46 (m, 2H), 2.27 (t, 4H), 1.62 (m, 4H), 1.43 (m, 16H), 1.31 (m, 40H), 0.88 (m, 6H). IR (cm−1): 2924, 2853, 1637.
3 Synthesis of 5-membered cyclic carbonates. The bis-epoxide was first pre-mixed with TBABr (3 wt%). Then the mixture was placed in a reactor and heated up at the selected temperature (80 °C for UndPdE-bisEpoxide, UndPMedA-bisEpoxide and UndDHexdA-bisEpoxide, 135 °C for UndPipdA-bisEpoxide and 140 °C for UndBdA-bisEpoxide). Once the temperature got stabilized, CO2 was slowly introduced into the reactor until 50 Bar or 60 Bar in the case of UndBdA-bisEpoxide. After 24 hours, the reactor was cooled down to room temperature and slowly depressurized to the atmospheric pressure. All the 1H NMR of all products revealed quantitative conversion by the disappearance of the protons of the epoxide.
UndPdE-CC. UndPdE-bisEpoxide (3 g, 6.8 mmol) and TBABr (0.09 g, 0.28 mmol, 3 wt%). The purity of UndPdE-bisEpoxide (99.1%) was determined by SEC. Yield = 97%. 1H NMR (CDCl3, 25 °C, 400 MHz) δ (ppm): 4.68 (m, 2H), 4.50 (t, 2H), 4.11 (t, 4H), 4.04 (t, 2H), 2.27 (t, 4H), 1.94 (m, 2H), 1.76 (m, 2H), 1.66 (m, 2H), 1.58 (m, 4H), 1.45 (m, 4H), 1.28 (m, 16H). 13C NMR (CDCl3, 25 °C, 100 MHz) δ (ppm): 173.81 (COO), 155.20 (OCOO), 77.21 (CH–OCOO), 69.51 (CH2–OCOO), 60.93 (CH2–OCO), 34.28 (CH2–COO), 33.95 (CH2–CH–OCOO), 29.21–29.13 (CH2), 28.10 (CH2–CH2–OCO), 24.94 (CH2–CH2–COO), 24.44 (CH2–CH2–CH–OCOO). IR (cm−1): 2927, 2856, 1792, 1727, 1163.
UndBdA-b5CC. UndBdA-bisEpoxide (3 g, 6.6 mmol) and TBABr (0.09 g, 0.28 mmol, 4.5 wt%). Yield = 95%. 1H NMR (CDCl3,, 25 °C, 400 MHz) δ (ppm): 5.83 (s, 2NH), 4.70 (m, 2H), 4.53 (t, 2H), 4.06 (t, 2H), 3.26 (m, 4H), 2.16 (t, 4H), 1.78 (m, 2H), 1.62 (m, 6H), 1.53 (m, 4H), 1.47 (m, 4H), 1.30 (m, 16H). 13C NMR (CDCl3, 25 °C, 100 MHz) δ (ppm): 173.81 (CONH), 155.27 (OCOO), 77.22 (CH–OCOO), 69.54 (CH2–OCOO), 39.20 (CH2–NHCO), 36.65 (CH2–CONH), 33.94 (CH2–CH–OCOO), 29.17 (CH2), 26.91 (CH2–CH2–NHCO), 25.84 (CH2–CH2–CONH), 24.43 (CH2–CH2–CH–OCOO). IR (cm−1): 3309, 2918, 2850, 1778, 1637, 1535.
UndPipdA-b5CC. UndPipdA-bisEpoxide (5 g, 11.1 mmol) and TBABr (0.15 g, 0.46 mmol, 3 wt%). Yield = 98%. 1H NMR (CDCl3, 25 °C, 400 MHz) δ (ppm): 4.69 (m, 2H), 4.51 (t, 2H), 4.06 (t, 2H), 3.62 (m, 4H), 3.45 (m, 4H), 2.32 (t, 4H), 1.76 (m, 2H), 1.70–1.63 (m, 8H), 1.47 (m, 2H), 1.31 (m, 16H). 13C NMR (CDCl3, 25 °C, 100 MHz) δ (ppm): 172.00 (C
O–N), 155.15 (OCOO), 77.12 (CH–OCOO), 69.47 (CH2–OCOO), 45.41 and 41.61 ((CH2)2–N–C
O), 33.93 (CH2–CH–OCOO), 33.29 (CH2–C
O–N), 29.25 (CH2), 25.20 (CH2–CH2–C
O–N), 24.42 (CH2–CH2–CH–OCOO). IR (cm−1): 2915, 2847, 1775, 1628.
UndPMedA-b5CC. UndPMedA-bisEpoxide (5 g, 10.7 mmol) and TBABr (0.15 g, 0.46 mmol, 3 wt%). The purity of UndPMedA-bisEpoxide (88.6%) was determined by GC-FID. Yield = 96%. 1H NMR (CDCl3, 25 °C, 400 MHz) δ (ppm): 4.69 (m, 2H), 4.51 (t, 2H), 4.05 (t, 2H), 3.34–3.27 (m, 4H), 2.98–2.89 (s, 6H), 2.27 (m, 4H), 1.79 (m, 6H), 1.61 (m, 6H), 1.47 (m, 2H), 1.30 (m, 16H). 13C NMR (CDCl3, 25 °C, 100 MHz) δ (ppm): 173.12 (CONCH3), 155.20 (OCOO), 77.16 (CH–OCOO), 69.51 (CH2–OCOO), 47.76–45.50 (CH2–NCH3CO), 35.51–33.08 (NCH3CO), 33.92 (CH2–CH–OCOO), 33.65–33.38 (CH2–CONCH3), 29.52–24.46 (CH2), 26.53 (CH2–CH2–NCH3CO). IR (cm−1): 2913, 2847, 1787, 1631.
UndDHexdA-b5CC. UndDHexdA-bisEpoxide (3 g, 4.2 mmol) and TBABr (0.09 g, 0.28 mmol, 3 wt%). Yield = 88.7%. 1H NMR (CDCl3, 25 °C, 400 MHz) δ (ppm): 4.67 (m, 2H), 4.50 (t, 2H), 4.04 (t, 2H), 3.25 (m, 4H), 3.17 (m, 4H), 2.24 (t, 4H), 1.75 (m, 2H), 1.65–1.60 (m, 6H), 1.45 (m, 12H), 1.29 (m, 40H), 0.87 (m, 6H). 13C NMR (CDCl3, 25 °C, 100 MHz) δ (ppm): 173.10 (CONCH3), 155.14 (OCOO), 76.97 (CH–OCOO), 69.27 (CH2–OCOO), 47.73–45.56 (CH2–NCO), 33.59 (CH2–CH–OCOO), 32.77 (CH2–CON), 31.27–22.30 (CH2), 13.78 (CH3). IR (cm−1): 2924, 2854, 1795, 1634.
Polymer synthesis and characterizations
The bis 5-membered cyclic carbonates (UndPdE-b5CC, UndBdA-b5CC, UndPipdA-b5CC, UndPMedA-b5CC and UndDHexdA-CC) and the diamines (butane-1,4-diamine (4DA), isophorone diamine (IPDA), Jeffamine 400 g mol−1, CRODA diamine, Priamine 1075) were weighted in a test tube. The polymerization reactions were conducted in bulk under static nitrogen. The mixture was stirred at the selected temperature: 140 °C (for UndBdA-b5CC and UndPipdA-b5CC), 120 °C (for UndPMedA-b5CC and UndDHexdA-b5CC) and 70 °C or 110 °C/120 °C for UndPdE-b5CC. No catalysts were added for the polymerization reactions.
PHU-dE-1 [UndPdE-b5CC + 4DA]: 3655–3127, 2924, 2854, 1714–1691, 1528.
PHU-BdA-1 [UndBdA-b5CC + IPDA]: 3536–3132, 2922, 2852, 1700, 1642, 1539.
PHU-DHexdA-1 [UndDHexdA-b5CC + IPDA]: 3595–3128, 2922, 2852, 1713, 1626, 1535.
Kinetic experiments monitored by FTIR-ATR of the cyclic carbonate–amine reaction
The kinetic experiments were performed in bulk at 25 °C with a ratio 1
:
1 between the propylene carbonate and hexylamine, using 5 mol% of catalyst. For some experiments, higher temperature (80 °C) was also investigated. For instance; propylene carbonate (5CC) (0.25 g, 2.4 mmol) and MTBD (18.7 mg, 0.12 mmol, 5 mol%) were weighted in a test tube. Then, hexylamine (0.25 g, 2.4 mmol) was added to the reaction mixture. The reaction was monitored with FTIR-ATR spectroscopy with the disappearance of the carbonyl band of the cyclic carbonate. Two isomers are obtained; one with a primary alcohol (isomer OH-I) and one with a secondary alcohol (isomer OH-II).
PCC + hexylamine-isomer OH-II. 1H NMR (CDCl3, 25 °C, 400 MHz) δ (ppm): 4.97 (NH), 4.05 (m, 1H), 3.98 (m, 1H), 3.91 (m, 1H), 3.14 (m, 2H), 1.47 (m, 2H), 1.27 (m, 6H), 1.15 (d, 3H), 0.86 (t, 3H). 13C NMR (CDCl3, 25 °C, 100 MHz) δ (ppm): 156.98 (OCONH), 72.69 (CH–OCONH), 66.52 (CH2–OH), 41.17 (CH2–NHCOO), 29.94 (CH2–CH2–NHCOO), 31.54–22.64 (CH2), 16.70 (CH3–CH–OCONH), 14.08 (CH2–CH3). IR (cm−1): 3321, 2929, 2856, 1687, 1533.
PCC + hexylamine-isomer OH-I. 1H NMR (CDCl3, 25 °C, 400 MHz) δ (ppm): 4.97 (NH), 4.85 (m, 1H), 3.63–3.55 (m, 2H), 3.14 (m, 2H), 1.47 (m, 2H), 1.27 (m, 6H), 1.17 (d, 3H), 0.86 (t, 3H). 13C NMR (CDCl3, 25 °C, 100 MHz) δ (ppm): 156.98 (OCONH), 70.21 (CH2–OCONH), 66.61 (CH–OH), 41.17 (CH2–NHCOO), 29.94 (CH2–CH2–NHCOO), 31.54–22.64 (CH2), 19.17 (CH3–CH–OH), 14.08 (CH2–CH3). IR (cm−1): 3321, 2929, 2856, 1687, 1533.
Conclusions
Various fatty acid-based 5-membered cyclic carbonate dimers bearing ester or amide linkages have been synthesized following a three-step procedure. The first stage was the transesterification or the amidation of methyl undecenoate. Then, the cyclic carbonates were introduced by epoxidation of the double bonds and subsequent carbonation with CO2. Amorphous to semi-crystalline poly(hydroxyurethane)s with reasonable molar masses (30
000 g mol−1) have been achieved. The tailor-made bio-based thermoplastic poly(hydroxyurethane)s properties are those expected when using cyclic carbonate dimers and diamines structures. Indeed, the presence of ester or amide functions, of hydrogen bonds through amide linkages and of cyclic structures or pendant groups or alkyl chains were found to drastically modify the PHUs properties.
Acknowledgements
The authors thank University of Bordeaux, ANR (Polygreen project), IPB, CNRS and Aquitaine Council for the financial support of this research. Authors also acknowledge Anne-Laure Wirotius for her help during variable temperature NMR studies.
References
- L. Maisonneuve, T. Lebarbe, E. Grau and H. Cramail, Polym. Chem., 2013, 4, 5472–5517 RSC.
- M. Desroches, M. Escouvois, R. Auvergne, S. Caillol and B. Boutevin, Polym. Rev., 2012, 52, 38–79 CrossRef CAS.
- D. P. Pfister, Y. Xia and R. C. Larock, ChemSusChem, 2011, 4, 703–717 CrossRef CAS PubMed.
- G. Lligadas, J. C. Ronda, M. Galiá and V. Cádiz, Biomacromolecules, 2010, 11, 2825–2835 CrossRef CAS PubMed.
- B. Nohra, L. Candy, J.-F. Blanco, C. Guerin, Y. Raoul and Z. Mouloungui, Macromolecules, 2013, 46, 3771–3792 CrossRef CAS.
- O. Kreye, H. Mutlu and M. A. R. Meier, Green Chem., 2013, 15, 1431–1455 RSC.
- M. S. Kathalewar, P. B. Joshi, A. S. Sabnis and V. C. Malshe, RSC Adv., 2013, 3, 4110–4129 RSC.
- J. Guan, Y. Song, Y. Lin, X. Yin, M. Zuo, Y. Zhao, X. Tao and Q. Zheng, Ind. Eng. Chem. Res., 2011, 50, 6517–6527 CrossRef CAS.
- B. Lebedev, V. Veridusova, H. Höcker and H. Keul, Macromol. Chem. Phys., 2002, 203, 1114–1125 CrossRef CAS.
- J. Kušan, H. Keul and H. Höcker, Macromolecules, 2001, 34, 389–395 CrossRef.
- S. Neffgen, J. Kušan, T. Fey, H. Keul and H. Höcker, Macromol. Chem. Phys., 2000, 201, 2108–2114 CrossRef CAS.
- P. Deepa and M. Jayakannan, J. Polym. Sci., Part A: Polym. Chem., 2008, 46, 2445–2458 CrossRef CAS.
- B. Ochiai and T. Utsuno, J. Polym. Sci., Part A: Polym. Chem., 2012, 51, 525–533 CrossRef.
- E. Hablot, D. Graiver and R. Narayan, in PU Magazine International, 2012, pp. 255–257 Search PubMed.
- M. Unverferth, O. Kreye, A. Prohammer and M. A. R. Meier, Macromol. Rapid Commun., 2013, 34, 1569–1574 CrossRef CAS PubMed.
- D. V. Palaskar, A. Boyer, E. Cloutet, C. Alfos and H. Cramail, Biomacromolecules, 2010, 11, 1202–1211 CrossRef CAS PubMed.
- A. S. More, B. Gadenne, C. Alfos and H. Cramail, Polym. Chem., 2012, 3, 1594–1605 RSC.
- A. Boyer, E. Cloutet, T. Tassaing, B. Gadenne, C. Alfos and H. Cramail, Green Chem., 2010, 12, 2205–2213 RSC.
- M. Bahr and R. Mulhaupt, Green Chem., 2012, 14, 483–489 RSC.
- I. Javni, D. P. Hong and Z. S. Petrović, J. Appl. Polym. Sci., 2012, 128, 566–571 CrossRef.
- I. Javni, P. H. Doo and Z. S. Petrovic, J. Appl. Polym. Sci., 2008, 108, 3867–3875 CrossRef CAS.
- S. Foltran, J. Alsarraf, F. Robert, Y. Landais, E. Cloutet, H. Cramail and T. Tassaing, Catal. Sci. Technol., 2013, 3, 1046–1055 CAS.
- S. Foltran, L. Maisonneuve, E. Cloutet, B. Gadenne, C. Alfos, T. Tassaing and H. Cramail, Polym. Chem., 2012, 3, 525–532 RSC.
- S. Foltran, R. Mereau and T. Tassaing, Phys. Chem. Chem. Phys., 2011, 13, 9209–9215 RSC.
- H. Tomita, F. Sanda and T. Endo, J. Polym. Sci., Part A: Polym. Chem., 2001, 39, 3678–3685 CrossRef CAS.
- R. M. Garipov, V. A. Sysoev, V. V. Mikheev, A. I. Zagidullin, R. Y. Deberdeev, V. I. Irzhak and A. A. Berlin, Dokl. Phys. Chem., 2003, 393, 289–292 CrossRef CAS.
Footnote |
† Electronic supplementary information (ESI) available: Experimental methods and graphical data. See DOI: 10.1039/c4ra03675a |
|
This journal is © The Royal Society of Chemistry 2014 |
Click here to see how this site uses Cookies. View our privacy policy here.