DOI:
10.1039/C3RA45011B
(Paper)
RSC Adv., 2014,
4, 488-494
Enhanced efficiency of antiseptics with sustained release from clay nanotubes†
Received
11th September 2013
, Accepted 30th October 2013
First published on
1st November 2013
Abstract
Natural halloysite clay tubules were studied for their potential use as miniature biocompatible containers that can be loaded with antiseptics followed by their slow and controlled release. Brilliant green was loaded into 15 nm diameter halloysite lumen at 15 wt% and provided sustained release over six hours. Formation of a benzotriazole–copper coating on halloysite nanotubes allowed additional encapsulation providing for more sustained release from 50 to 200 hours. Antibacterial efficiency of the brilliant green in clay nanotubes was tested on Staphylococcus aureus cultures and antibacterial action extended up to 72 hours was demonstrated. Sustained release of amoxicillin and iodine from halloysite tubes was also demonstrated.
1. Introduction
Halloysite nanotubes are naturally occurring clay found in several deposits worldwide.1–3 For example, Applied Mineral's Dragonite mined from the deposit in Utah in the western United States, has an estimated millions of tons of halloysite making it economically viable for large scale production. Halloysite is a two-layered aluminosilicate, chemically similar to kaolin, and has a predominantly hollow tubular structure in the submicron range.2–4 Halloysite tubes typically display an inner diameter of 10–15 nm, an outer diameter 50–70 nm, and a length between 500 and 1500 nm.4,5
The halloysite lumen produces a large capillary force in polar liquids (e.g. for water capillary pressure is 200 atm.), which helps in loading chemical agents within the tubes. At pH above 2.5, halloysite has a negative SiO2 surface characterized by electrical zeta-potential of ca. −35 mV. This allows for its good dispersibility in water, alcohol, acetone, and polar polymers. Pristine halloysite has a large surface area of 60–70 m2 g−1. Control over loading and release has been achieved using several methods such as silanization of lumen for inner tube hydrophobicity for higher adsorption of low water soluble drugs,6,7 lumen enlargements by etching,8 and tube encapsulation by formation of polymeric coating and caps at the tube ends.9 Halloysite loading efficiency can be increased up to 30 wt% with lumen enlargement using acid etching of the internal alumina.8 These modified clay nanotubes can be loaded with drugs at 10–30 wt% and used for sustained release of chemical agents.1,3,5,8–11 Halloysite tubes are biocompatible as was demonstrated on different cell cultures and micro worms.2,12,13 Surface chemistry, tubule morphology, submicron size and biocompatibility make this nanomaterial an excellent candidate for diffusion nanopore-controlled release of antiseptics, drugs and proteins with time of delivery of 10–50 h.8–10
In this work, we studied halloysite as a nanocontainer for loading brilliant green to demonstrate slow release with extended antimicrobial activity of this antiseptic. Copper–benzotriazole coatings at the tube ends provided extended release reaching from hundred hours to months. The large increase in controlled release allowed for a significant increase of antimicrobial efficiency for the released antiseptics. These capsules may find applications in various fields of medicine like tissue engineering and bandages for wound healing.
2. Experimental
2.1. Materials
Halloysite has been obtained from Applied Minerals Inc. Brilliant green, benzotriazole, copper sulfate, tryptic soy broth (TSB), fluorescein diacetate (FDA), dimethyl sulfoxide (DMSO) have been obtained from Sigma Aldrich. Propidium iodide (PI) was obtained from Fluka. Staphylococcus aureus (ATCC 49774) bacteria were purchased at ATCC.
2.2. Instruments
Halloysite clay nanotubes were characterized with a Scanning Electron Microscope (Hitachi S4800 FE-SEM). The halloysite samples were coated with 1 nm gold by Cressington Sputter Coater (208HR) before the imaging. A Transmission Electron Microscope (TEM, Zeiss EM 912) was used at 120 kV for the imaging of internal tube lumens. Nitrogen adsorption–desorption isotherms were measured with NOVA 2200, Quanto Chrome Instruments at 77 K for the specific surface area estimations with the BET (Brunauer–Emmett–Teller) technique. UV-vis spectrophotometers Agilent 8453 and SmartSpec™ 3000, BioRad were used for monitoring of the antiseptic release and bacteria concentration estimation. Fluorescent plate readers FLx800 (BioTek) were used for checking bacterial cell viability. Cells were imaged with Nikon Eclipse TE 2000-U inverted fluorescent microscope equipped with CCD monochrome digital camera Cool Snap ES.
2.3. Loading procedures
Brilliant green (200 mg) was dissolved in 10 mL acetone. Then 500 mg of halloysite was added to the solution and thoroughly mixed followed by sonication for 30 minutes. The solution was placed in a vacuum chamber for 30 minutes. Air bubbles were popped-up from the halloysite pores allowing for antiseptic into lumens. After 30 minutes, the vacuum was stopped and air allowed to the chamber. This cycle was repeated three times followed by the sample washing with water to remove any unloaded antiseptic. Loaded halloysite was dried in an oven at 50 °C and milled to fine powder.
2.4. Benzotriazole–copper complexation
Halloysite was additionally encapsulated with benzotriazole–copper film to provide extended release (Scheme 1). Nanotubes loaded with antiseptics were washed for 10 s with benzotriazole solution and followed by aqueous CuSO4. A thin tube coating has been formed by chelation of benzotriazole with copper ions. Antiseptic release rate was decreased with this treatment. The complexation and release rate have been controlled by varying the concentrations of the benzotriazole and Cu2+.
 |
| Scheme 1 Procedure for loading brilliant green inside halloysite nanotubes and fabrication of a benzotriazole–copper film. | |
2.5. Release kinetics
Brilliant green release experiments were conducted by stirring 50 mg of loaded halloysite in 1 mL of deionized water. At each reading, the supernatant was removed by centrifugation at 7000 rpm (2 minutes) and fresh water was added. Collected supernatant was analyzed by UV-vis absorption at 625 nm absorption peak for the amount of brilliant green released. To get estimation of the full tube loading, halloysite was dispersed in 50 mL of water and sonicated for 30 minutes (until all brilliant green was released). Then supernatant was separated by centrifugation at 5000 rpm (10 minutes) and total amount of brilliant green was measured with UV absorption.8,14
2.6. Cultivation of Staphylococcus aureus
Bacteria were cultivated in tryptic soy broth (TSB, Sigma) at 37 °C according to the manufacturer protocol. 30 g of rehydrated media was dissolved in 1 L of deionized water and sterilized at 121 °C for 1 h. A day before the halloysite experiments, bacteria were inoculated into fresh broth and grown overnight. Concentration of cells was determined by UV spectrophotometry at 600 nm with conventional coefficient of 1 o.u. being equal to 108 CFU mL−1. Purity of culture was checked by standard Gram-staining technique.15
2.7. Kinetic viability assay
5 mL aliquot of cells at concentration of 104 CFU mL−1 was used for each experiment. Loaded halloysite was placed into bacteria tubes containing 104 CFU mL−1 and cultivated at 37 °C in shaker-incubator 24 to 72 hours. After every 24 hours 1 mL aliquots was collected for viability tests and media in the remaining sample was replaced by fresh nutrient media.
2.8. Live/dead assay
The ratio of live to dead cells was monitored by fluorescein diacetate/propidium iodide (FDA/PI) double staining. FDA is a non-fluorescent ester which is able to pass through cell membranes and become fluorescent after intracellular esterase cleavage. PI stains only membrane compromised cells. 0.5 mL of each sample was washed by 0.85% NaCl twice following with centrifugation at 7000 rpm. Then 2.5 μL of FDA was added to each sample from stock-solution of 10 mg mL−1 in acetone (final amount of FDA was 50 μg) and incubated for 20 min at room temperature. After that, 2.5 μL of PI stock-solution (1 mg mL−1 in water) was added to each tube and incubated for 10 min. Finally, 100 μL of each sample were dispensed in 96-well black plate in three replicates and fluorescence intensity was measured at 540 nm for FDA (exited at λ = 485 nm) and at 600 nm for PI (exited at λ = 548 nm). Live/dead cell ratio was represented as the relative ratio of green/red fluorescence units.
2.9. Fluorescent microscopy
Nikon Eclipse TE 2000-U inverted fluorescent microscope equipped with Cool Snap ES Photometric digital camera was used to visualize treated cells using FDA/PI double staining of S. aureus cells. Before microscopic observation cells of 108 CFU mL−1 concentration, they were incubated for 3 hours in TSB nutrient media supplemented with free brilliant green, brilliant green loaded halloysites or loaded halloysite capped with copper–benzotriazole. Weights of these additives were adjusted so that each sample contains 663 μM of brilliant green (its loading efficiency was 3 wt% and 15 wt% correspondingly). After this, cells were washed twice with sterile 0.85% NaCl and centrifuged at 7000 rpm for 2 min. The precipitated cells were re-suspended in 0.5 mL 0.85% NaCl solution. To this solution 0.75 μL of PI (stock solution in DMSO 20 mM) and 0.5 μL of FDA (stock solution in acetone 10 mg mL−1) were added, vortexed and incubated for 20 min at room temperature. Samples were analyzed using fluoresce microscope using broadband blue filter (λex = 480 nm, λem = 535 nm) for viable cells and green filter (λex = 540 nm, λem = 620 nm) for dead cells. Pictures were colored using MetaMorph software. As a control sample we used S. aureus cells at concentration 108 CFU mL−1 incubated at room temperature for 3 hours without addition halloysite and brilliant green.
3. Results and discussion
3.1. Halloysite nanotubes
Morphology of halloysite tubes was analyzed with SEM and TEM imaging. A tubular clay structure with the external diameters of 50 ± 10 nm is evident (Fig. 1A and B). The length of tubes covers 0.5–1.5 μm and lumen diameter is 10–20 nm. Halloysite lumen inner surface is composed of aluminol (Al–OH) and external tube surface is siloxane (Si–O–Si). Halloysite walls are made of 10–15 rolled aluminosilicate sheets with packing periodicity of 0.70 ± 0.02 nm as determined by X-ray diffraction.14 Elemental composition of nanotubes was determined using SEM-EDX analysis as follows (atomic %): Al, 18.5; Si, 19.1; O, 62.2. Brunauer–Emmett–Teller (BET) surface area of the used halloysite sample was 62 ± 5 m2 g−1.
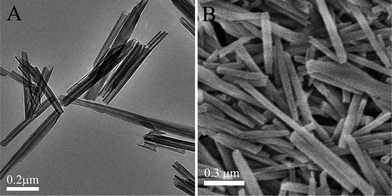 |
| Fig. 1 Images of halloysite clay: TEM – tubes dispersed in water and dried on a copper grid (a) and SEM – dry powder (b). | |
3.2. Release of brilliant green
A commonly used antiseptic agent, brilliant green (BG), was studied for extended release delivery in three different formulations: (1) free microcrystals, (2) loaded within halloysite lumen, and (3) halloysite loaded with brilliant green followed by coating with benzotriazole–copper (BTA–Cu) complexation. The antiseptic release rate slowed down significantly with nanotube encapsulation and was becoming even more extended after an additional coating on the tubes (Fig. 2A). Crystals of brilliant green completely dissolved within 15 minutes; however, release time from halloysite nanotubes was prolonged to five hours (70% release in 3 hours). BTA–Cu encapsulation allowed for 5 wt% of brilliant green release within 3 hours, and total release time reached 200 hours, drastically extending supply of this antiseptic. Fig. 2B demonstrates TEM image of BTA–Cu coating on halloysite tube.
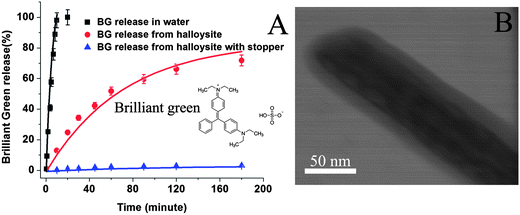 |
| Fig. 2 Brilliant green (BG) release curves in DI water and room temperature (A), halloysite nanotubes and from halloysite with BTA–Cu stopper. TEM image of BTA–Cu coating on halloysite tube (B). | |
3.3. Cu–BTA complexation
Organic triazole forms a two-dimensional film upon interaction with transition metal ions: copper, iron and nickel. This has been utilized to make halloysite coating with end-stoppers to slow release of brilliant green from the tube lumens.16,17 Factors affecting BTA–Cu complexation and coating formation, i.e. concentrations of the benzotriazole and Cu(II), were studied for optimization of the antiseptic release kinetics. Concentrations of benzotriazole and CuSO4 for the complexation were varied at 40–170 mM and 4–80 mM ranges, respectively. Fig. 3 presents brilliant green release curves treated at different complexation conditions. A release rate gradually decreases at fixed Cu(II) concentration of 4 mM (Fig. 3B) upon decrease of benzotriazole concentration. An encapsulating film formed at higher BTA concentration lead to more porous coating and its decrease to 41 mM retarded release rates. Surprisingly, opposite phenomenon was observed at high Cu(II) concentration of 80 mM: brilliant green release rate was highest at 41 mM BTA concentration and gradually decreases with increasing concentration (Fig. 3A).
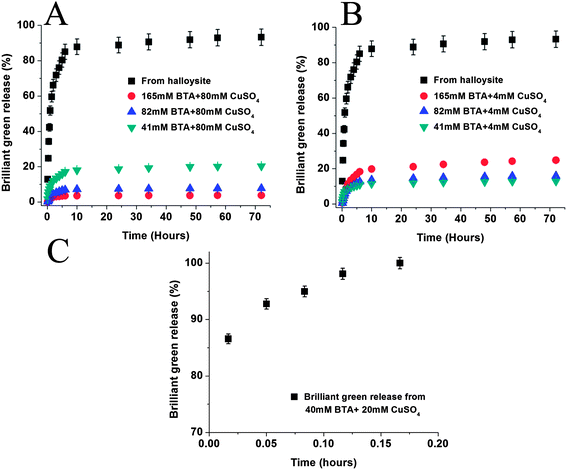 |
| Fig. 3 Brilliant green release with different tube encapsulations: halloysite with BTA–Cu stopper which has various concentrations of BTA and 80 mM CuSO4 (A); halloysites with BTA–Cu stoppers which have various concentrations of BTA and 4 mM CuSO4 (B). Brilliant green release from microcrystals BTA–Cu encapsulated without halloysite tubes (much faster release) (C). | |
As expected faster film formation occurs upon using higher concentrations of benzotriazole, which results in smaller amounts of leaking brilliant green during coating formation (it leaks from halloysite pores during encapsulation as tubes are not sealed yet, causing to reduce loading efficiency). Higher loading efficiency causes higher concentration gradient between inner pores and external solution, which is the major driving force for drug release. Indeed, loading efficiencies of the samples coated with 41, 82, and 165 mM benzotriazole and 80 mM CuSO4 increased from 6.5 to 15.8 wt% as compared with pristine halloysite of 3 wt% loading. For comparison, in Fig. 3C we demonstrate fast 10 min dissolution curve for brilliant green microcrystals encapsulated with benzotriazole–copper complexation only, which determined the necessity of clay nanotube usage to achieve a sustained long-time release.
We conclude that two main factors affect the rate of brilliant green release: the loading efficiency and Cu–BTA coating porosity. Both factors are influenced by the concentration of Cu(II) and BTA. At high concentrations of Cu and BTA, both factors are modified in such a way that is beneficial to sustained release. Loading efficiency is increased due to faster coating formation preventing loss of material already loaded. Higher concentrations also facilitate smaller pore sized further reducing the rate of release. This provides a good opportunity for making long lasting release of antiseptics loaded within halloysite lumen and can be generalized to other bioactive agent loading and release. For bacterial tests we chose loaded pristine halloysite and halloysite encapsulated with 165 mM BTA/4 mM CuSO4, which provided stable 20% release in 50 h with extrapolated 90% total release within 30 days.
Release curves in Fig. 2 and 3 were described with first order exponential kinetic approximation: R = M∞(1 − e−kt), where M∞ is the amount of active agent released at infinite time (i.e. amount loaded within lumen) and k is the release rate constant.14–18 Release profiles are composed of two parts: fast release in the initial 6 hours followed by slower release. Different constants of the reaction rate were used to describe these two parts of the release profiles (Table 1). We assume that the initial release is associated with brilliant green that is deposited at the tube endings or in external pockets of the tubes (Fig. 1). These molecules are loosely attached to the halloysite surface and release quickly. In the second stage, molecules from inner tube lumen are released.
Table 1 First order release parameters M∞ and k of halloysite/brilliant green samples
Sample |
M
∞ (6 hours) |
K (6 hours) |
M∞ (remain) |
K
2
(remain) |
LEa(wt%) |
Loading efficiency.
|
BG release in water |
122 |
10.4 |
— |
— |
— |
BG release from halloysite tubes |
83 |
0.84 |
93 |
0.37 |
2.8 |
BG release from halloysite with 165 mM BTA + 80 mM Cu2+ |
3.5 |
0.65 |
3.8 |
0.40 |
15.8 |
BG release from halloysite with 82 mM BTA + 80 mM Cu2+ |
7.0 |
0.59 |
7.8 |
0.39 |
11.5 |
BG release from halloysite with 41 mM BTA + 80 mM Cu2+ |
17 |
0.53 |
20.2 |
0.27 |
6.5 |
BG release from halloysite with 165 mM BTA + 4 mM Cu2+ |
18 |
0.55 |
24 |
0.21 |
10.8 |
BG release from halloysite with 82 mM BTA + 4 mM Cu2+ |
12.5 |
0.55 |
15.5 |
0.27 |
8.6 |
BG release from halloysite with 41 mM BTA + 4 mM Cu2+ |
11 |
0.65 |
12.7 |
0.33 |
7.8 |
3.4. Antibacterial effect of brilliant green loaded halloysite
Fig. 4 shows live/dead ratio of Staphylococcus aureus cells with different brilliant green loaded halloysite (pristine and with coating) and free not-encapsulated brilliant green. We used fluorescein diacetate and propidium iodide double staining to assess live/dead cell ratio using plate reader assay. Kinetic experiments included centrifugation and discarding of old media steps, which leads to an unavoidable loss of cells creating a decrease in the relative fluorescence intensity of living cells. According to the results of fluorescent plate reader assay both brilliant green loaded halloysite with and without stopper suppress growth of S. aureus as compared with untreated cells. Free brilliant green only suppress growth of S. aureus within approximately 5 to 10 hours; after that bacteria grow similar to the untreated sample. Kinetic viability test data has shown that coated brilliant green loaded tubes (BTA + Cu) significantly inhibit bacteria growth as compared as both untreated control and uncoated halloysite samples. Cu–BTA complex releases less than 10−4 M of copper and benzotriazole, which themself did not show antibacterial toxicity.17 Bacteria growing in nutrient media supplemented by equal amount of free brilliant green continue growing after 1st media replacement. Moreover, it has been noticed that S. aureus decolorize free brilliant green for 5 hours whereas media containing loaded halloysite tubes remains green within 72 hours. Previous studies have shown that inhibitory effect of brilliant green is connected with colored form of the dye.19
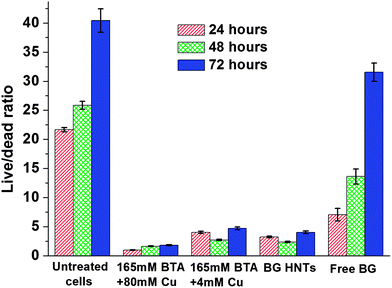 |
| Fig. 4 Kinetics of live/dead S. Aureus cells ratio treated with brilliant green loaded halloysite with BTA + CuSO4, halloysite without coating (HNT) and free brilliant green (BG). Data are represented as average over three experiments. Control – untreated cells growth. | |
Samples were visualized using fluorescence microscope with broadband blue filter for live cells and green filter for dead cells. In Fig. 5, green dots represent live cells while red dots indicate dead ones. According to fluorescence microscopy data one can see that the samples treated with loaded halloysite (capped and uncoated) and free brilliant green contain dead membrane compromised cells as compared with the control sample. It was counted that control contains 3 ± 1% dead cells whereas free brilliant green treated sample, halloysite treated and loaded capped halloysite give 80 ± 5, 65 ± 3 and 68 ± 4% dead cells, respectively. Larger amount of dead cells in the sample treated with free brilliant green can be explained by the immediate action of the antiseptic, but according to the kinetic viability assay bactericide action of brilliant green lasts less than 24 hours and eliminated after 1st media replacement. It was also noticed that some cells have double green/red emission. This phenomenon can be explained that brilliant green partially damage cell membrane and enable PI to penetrate through the formed gaps and stain nucleic acids inside cells. A longer time antiseptic release provided by encapsulating halloysite allowed for efficient bacteria suppression for over 72 hours.
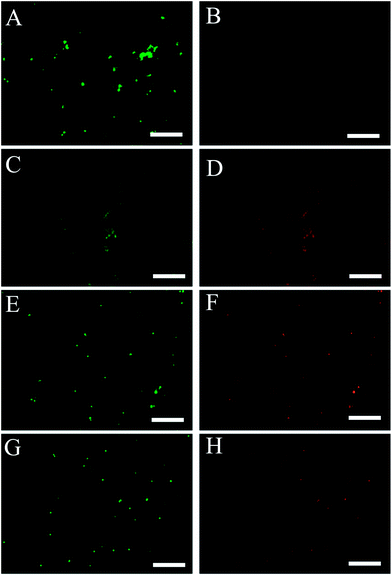 |
| Fig. 5 Fluorescent micrographs of alive/dead (green/red) S. aureus cells after 72 hours: control-untreated sample (A and B); treated with 663 μM of free brilliant green (C and D); treated with loaded halloysite without coating (E and F), and loaded halloysite with coating (G and H). Scale bar – 10 μm. | |
Halloysite loading/release experiments were also performed with the common antiseptics (amoxicillin and iodine) and 2–5 hours sustained release profile was achieved (ESI Materials, Fig. S1 and S2†).
Halloysite is biocompatible material: for many biological cells and tissues it is safe up to concentration of 0.2 mg per mL of culture which is very high safe concentration for inorganic inclusions. Halloysite was tested non-toxic for 48 hours incubation with fibroblast and human breast cells. To investigate the effect of halloysite on in vitro model of the large intestine, Caco-2/HT29-MTX cells were exposed to nanotubes for toxicity proteomic analysis: halloysite exhibits a high degree of biocompatibility characterized by an absence of cytotoxicity, despite elevated pro-inflammatory cytokine release.2 Bioinformatics analysis of differentially expressed protein profiles suggest that halloysite stimulates processes related to cell growth and proliferation, subtle responses to cell infection, irritation and injury, enhanced antioxidant capability, and an overall adaptive response to exposure.20 Our preliminary experiments with micro-warms and feeding mice and rats also support halloysite's safety for bio-organisms. All these properties suggest using halloysite as natural nanocontainers for loading and sustained release of biomedical agents (drugs, proteins, DNA, and antibacterials).1,2,5,9–13,20–23 Commonly used antibacterials, such as tetracycline, gentamicin,5 and hydroxyquinoline,14 were loaded into halloysite nanotubes at 5–6 wt% and have shown 6–10 hours release time without any additional encapsulation.
These data and the data reported above, suggest that for a number of antiseptics and antibiotics one could drastically increase their antibacterial efficiency from tens to hundreds of hours supply in the treated area. This is especially important for hospitals-acquired infections (e.g., the well-known nosocomial infections) that drive even further use of antimicrobials in medical applications.
4. Conclusion
Release study and antibacterial effect of common antiseptic agent – brilliant green have been studied using tubule halloysite clay as nanocontainers for loading and sustained release of this chemical. Complete dissolution of free brilliant green powder takes only 10 minutes while 70% of this antiseptic was slowly released from halloysite lumen within 3 hours allowing extending its antibacterial efficiency. Even longer controlled release within 10–200 hours was achieved by benzotriazole–copper tube coating. The factors affecting release rate are concentrations of benzotriazole and copper used for the tube coating: release rate reduces with increased benzotriazole concentration at lower copper concentrations, which is associated with formation of tighter coating with less porosity. Similar results of sustained release of amoxicillin and iodine from halloysite tubes were also demonstrated. S. aureus viability essay revealed that antiseptics loaded within halloysite provided much longer antibacterial activity as compared to the usage of free brilliant green.
Acknowledgements
The authors acknowledge support by NSF-1029147 and NSF EPS-1003897 grants. Any opinions, findings, and conclusions or recommendations expressed in this report are those of authors and do not necessarily reflect the view of National Science Foundation. R.M. also acknowledges the Russian President Research fellowship #539 (2012) for work at Louisiana Tech. This study was supported by RFBR 12-03-93939-G8.
References
- Y. Lvov, D. Shchukin, H. Moehwald and R. Price, Halloysite clay nanotubes for controlled release of protective agents, ACS Nano, 2008, 2, 814–820 CrossRef CAS PubMed.
- V. Vergaro, E. Abdullayev, Y. Lvov, A. Zeitoun, R. Cingolani, R. Rinaldi and S. Leporatti, Cytocompatibility and uptake of halloysite clay nanotubes, Biomacromolecules, 2010, 11, 820–826 CrossRef CAS PubMed.
- D. Shchukin, R. Price, G. Sukhorukov and Y. Lvov, Biomimetic synthesis of vaterite in the interior of clay nanotubules, Small, 2005, 5, 510–513 CrossRef PubMed.
- E. Joussein, S. Petit, J. Churchman, B. Theng, D. Righi and B. Delvaux, Halloysite clay minerals – a review, Clay Miner., 2005, 40, 383–426 CrossRef CAS PubMed.
- R. Price, B. Gaber and Y. Lvov,
In-vitro release characteristics of tetracycline, khellin, and nicotinamide adenine dinucleotide from halloysite: a cylindrical mineral for delivery of biologically active agents, J. Microencapsulation, 2001, 18, 713–723 CrossRef CAS PubMed.
- W. Yah, A. Takahara and Y. Lvov, Selective modification of halloysite lumen with octadecyl phosphonic acid: new inorganic tubular micelle, J. Am. Chem. Soc., 2012, 134, 1853–1859 CrossRef CAS PubMed.
- G. Cavallaro, G. Lazzara and S. Milioto, Exploiting the colloidal stability and solubilization ability of clay nanotubes/ionic surfactant hybrid nanomaterials, J. Phys. Chem., 2012, 116, 21932–21938 CAS.
- E. Abdullayev, A. Joshi, W. Wei, Y. Zhao and Y. Lvov, Enlargement of halloysite clay nanotube lumen by selective etching of aluminum oxide, ACS Nano, 2012, 6, 7216–7226 CrossRef CAS PubMed.
- S. Levis and P. Deasy, Use of coated microtubular halloysite for the sustained release of diltiazem hydrochloride and propranolol hydrochloride, Int. J. Pharm., 2003, 253, 145–157 CrossRef CAS.
- W. Wei, E. Abdullayev, A. Hollister, D. Mills and Y. Lvov, Clay nanotube/poly(methyl methacrylate) bone cement composites with sustained antibiotic release, Macromol. Mater. Eng., 2012, 297, 645–653 CrossRef CAS.
- Y. Suh, D. Kil, K. Chung, E. Abdullayev, Y. Lvov and D. Mongayt, Natural nanocontainer for the controlled delivery of glycerol as a moisturizing agent, J. Nanosci. Nanotechnol., 2011, 11, 661–665 CrossRef CAS PubMed.
- D. Kommireddy, S. Sriram, Y. Lvov and D. Mills, Layer-by-layer assembled nanoparticle thin films - a new surface modification approach for stem cell attachment, Biomaterials, 2006, 27, 4296–4303 CrossRef CAS PubMed.
- S. Konnova, I. Sharipova, T. Demina, Y. Osin, D. Yarullina, P. Zelenikhin, D. Ishmuchametova, O. Ilinskaya, Y. Lvov and R. Fakhrullin, Cell-mediated three-dimensional assembly of halloysite nanotubes, Chem. Commun., 2013, 49, 4208–4212 RSC.
- E. Abdullayev, R. Price, D. Shchukin and Y. Lvov, Halloysite tubes as nanocontainers for anticorrosion coating with benzotriazole, ACS Appl. Mater. Interfaces, 2009, 7, 1437–1443 Search PubMed.
-
K. Chapin and P. Murray, Strains, in Manual of clinical microbiology, ed. P. R. Murray, E. J. Baron, M. A. Pfaller, F. C. Tenover and R. H. Yolken, American Society for Microbiology, Washington, D.C, 1999, pp. 1674–1686 Search PubMed.
- E. Abdullayev and Y. Lvov, Halloysite clay nanotubes for controlled release of protective agents, J. Nanosci. Nanotechnol., 2011, 11, 10007–10026 CrossRef CAS PubMed.
- E. Abdullayev and Y. Lvov, Clay nanotubes for corrosion inhibitor encapsulation: release control with end stoppers, J. Mater. Chem., 2010, 20, 6681–6687 RSC.
- A. Ferretti and G. Joyce, Kinetic properties of an RNA enzyme that undergoes self-sustained exponential amplification, Biochemistry, 2013, 52, 1227–1235 CrossRef CAS PubMed.
- W. Moats, J. Kinner and S. Maddox, Effect of heat on the antimicrobial activity of brilliant green dye, Appl. Microbiol., 1974, 27, 844–847 CAS.
- X. Lai, M. Agarwal, Y. Lvov, C. Pachpande, K. Varahramyan and F. Witzmann, Proteomic profiling of halloysite clay nanotube exposure in intestinal cell co-culture, J. Appl. Toxicol., 2014, 34 DOI:10.1002/jat.2858.
- Y. Lee, G.-E. Jung, S. Cho, K. Geckeler and H. Fuchs, Cellular interactions of doxorubicin-loaded DNA-modified halloysite nanotubes, Nanoscale, 2013, 5, 8577–8585 RSC.
- E. Abdullayev and Y. Lvov, Halloysite clay nanotubes as a ceramic “skeleton” for functional biopolymer composites with sustained drug release, J. Mater. Chem. B, 2013, 1, 2894–2903 RSC.
- Y. Lvov and E. Abdullayev, Functional polymer–clay nanotube composites with sustained release of chemical agents, Prog. Polym. Sci., 2013, 38, 1690–1719 CrossRef CAS PubMed.
Footnote |
† Electronic supplementary information (ESI) available. See DOI: 10.1039/c3ra45011b |
|
This journal is © The Royal Society of Chemistry 2014 |
Click here to see how this site uses Cookies. View our privacy policy here.