Evidence supporting a 1,2-dioxetanone as an intermediate in the benzofuran-2(3H)-one chemiluminescence†
Received
3rd October 2013
, Accepted 14th October 2013
First published on 15th October 2013
Abstract
The mechanism of the chemiluminescent reaction of ethyl (5-fluoro-2-oxo-2,3-dihydrobenzofuran-3-yl) carbamate (a 2-coumaranone derivative) with a base and molecular oxygen was investigated. New evidence from the reaction kinetics and absorption/emission profiles was obtained, supporting the existence of a 1,2-dioxetanone as an intermediate: (i) its characteristic activation parameters (ΔH≠ = 7.2 ± 0.1 kcal mol−1; ΔS≠ = −45 ± 5 cal K−1 mol−1) indicating a high degree of thermal instability and (ii) its bimolecular decomposition rate constant for the reaction with perylene. The newly developed methodology has been shown to be suitable for determining the reactivity of such thermally unstable peroxides, which are very difficult to prepare and isolate, using this alternative approach of in situ generation of a 1,2-dioxetanone.
Introduction
The role of organic peroxides in chemiluminescence is crucial at the level of chemiexcitation, i.e. the formation of species at the excited electronic state by means of a chemical reaction. Within the large pool of chemiluminescent and bioluminescent reactions, one can name the presence of the model peroxides 1,2-dioxetanes (1), 1,2-dioxetanones (2) or 1,2-dioxetanedione (3) as almost obligatory.1
1,2-Dioxetanones
1,2-Dioxetanones are postulated intermediates in firefly bioluminescence2 and in chemiluminescence of acridinium esters,3 among many other chemiluminescent systems. The synthesis and isolation of those compounds are very difficult, due to their reactivity towards nucleophiles and electrophiles, and their thermal instability; it is thus an arduous task to work with such substances and study them from the chemical reactivity point of view.4
The CIEEL mechanism
In chemiluminescent systems, the peroxide is normally a high-energy species which is somehow able to transfer its energy to an acceptor; however, research made in this field in the 1970s showed that the acceptor is indeed an activator (ACT), since in many cases it is able to catalyse the decomposition of the peroxide. Schuster and coworkers proposed a theory able to explain this activation of peroxides by ACTs (in this case fluorescent aromatic polycyclic hydrocarbons with low oxidation potentials) in a series of papers where he proposed the occurrence of the intermolecular CIEEL (Chemically Initiated Electron Exchange Luminescence) mechanism.5 Many following papers have confirmed this proposition of Schuster's group, widening the scope of this mechanism, including systems where chemiexcitation may occur through a charge-transfer process;6 it seems clear by now that the CIEEL theory is well supported by experimental evidence.7,8
Decomposition of 1,2-dioxetanones
The decomposition of 1,2-dioxetanones, resulting in light emission, may take place via two main pathways (Scheme 1).9 The first one is by the thermal cleavage of such unstable compounds, a unimolecular process with a rate constant kD. The yields of singlet excited state production by this process are usually very low (ΦS < 0.01%); therefore, it is not a good model for firefly bioluminescence (Photinus pyralis), with a recently redetermined emission quantum yield of ΦBL = 41%.2
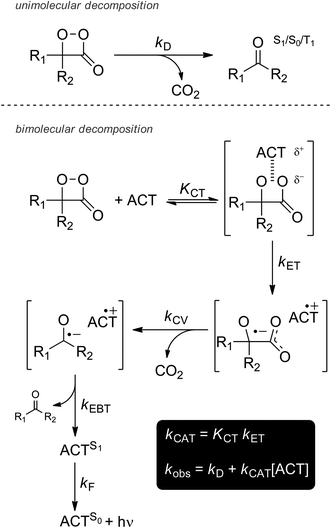 |
| Scheme 1 Possible interactions of a 1,2-dioxetanone producing excited states. The bimolecular decomposition is based on the intermolecular CIEEL mechanism proposition. | |
The second possible mechanism is by a bimolecular reaction with an ACT, an intermolecular version of the CIEEL mechanism.10 The yields of excited singlet states in this case are much higher, with ΦS ∼ 10%. The interaction between a peroxide like 2 and an ACT consists of five elementary steps: (i) interaction of the ACT and the peroxide inside a solvent cage, forming a charge-transfer type encounter complex, a process with an equilibrium constant KCT; (ii) electron transfer from the ACT to the peroxide, forming a pair of radical ions (kET), as the rate-determining step for the reaction; (iii) cleavage of the C–C bond eliminating CO2 in an irreversible process (kCV); (iv) electron back-transfer from the radical anion to the radical cation, forming the ACT at the excited singlet state (kEBT); (v) decay of the excited ACT to the ground state resulting in light emission (kF).
Because the charge-transfer reaction is the slowest, it is the rate-determining step of the whole process. So the observed rate constant (kobs) is defined by the sum of the rates of the unimolecular decomposition (kD) and the bimolecular decomposition kCAT [ACT].
2-Coumaranone chemiluminescence
The chemiluminescence of 2-coumaranone derivatives like 4 was first described by Lofthouse et al. in 1979;11 a simple mixture of the compounds with air-saturated DMF produced a bright emission which lasted for a couple of hours; the same reaction was also observed in other polar solvents, which require the addition of non-nucleophilic bases. However, the mechanism behind this emission has been only tentatively suggested, without being strongly backed by evidence; only recently, in an article by Schramm et al., a systematic approach for the mechanism of the reaction has been addressed.12
It is assumed that the chemiluminescent step involves the formation and decomposition of the 1,2-dioxetanone 5 (Scheme 2), formed after an unknown number of steps, probably by a radicalic mechanism. The intermediate 5 has never been isolated or characterized, and therefore its existence is just a suggestion at this point.
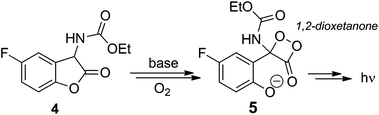 |
| Scheme 2 Reaction of the studied 2-coumaranone with a base and oxygen forming the intermediate 1,2-dioxetanone, which decays emitting light. | |
Experimental section
General materials
The ethyl (5-fluoro-2-oxo-2,3-dihydrobenzofuran-3-yl) carbamate 4 was prepared following the method of Schramm et al.12 Perylene (PER, Acros, 99+%) was used as received. Acetonitrile (ACN, Aldrich, anhydrous, 99.8%) was stored over molecular sieves (Aldrich, 3 Å, beads 8–12 mesh). 1,8-Diazabicyclo-[5.4.0]undec-7-ene (DBU, Aldrich, 98%) was used as received.
The quartz cuvettes used (Hellma, QS suprasil) had a volume of 3.0 mL and an optical path of 10.00 mm, with two polished sides for absorption assays and four sides for fluorescence assays.
Kinetic assays
All kinetic assays were carried out in fluorescence or absorption quartz cuvettes. The standard assay was done by filling the cuvette with 3.0 mL of ACN or the solution of PER in ACN of the desired concentration. This cuvette was left for 5 minutes in the cell holder thermostatized at 25.0 °C, and 20 μL of a 15 mM solution of 4 in ACN was injected, the resulting concentration in the cuvette being [4] = 0.1 mM. The reaction was started with the injection of 5 μL of DBU, and data acquisition was started after a quick shake made by hand (∼3 s). The kinetics was followed for at least five half-lives, and the obtained kobs values are the means of at least three experiments.
Results
The solvent chosen to conduct all assays was acetonitrile (ACN); even if it was shown that dimethylformamide (DMF) and dimethyl sulfoxide (DMSO) led to higher emission quantum yields, in ACN the decomposition time-profile of the high-energy intermediate shows a clean first-order decay. The high solubility of oxygen (1.9 mM at 0.213 bar O2) in ACN13 is also another advantage, since using 0.1 mM of 4 we set a pseudo-first order condition throughout the experiments.14
UV-Vis absorption studies
A solution of 4 in ACN is stable throughout a standard experiment procedure, as the spectra acquired within one hour showed no difference. Compound 4 absorbs in the UV region (λmax = 280 nm; ε = 2.07 × 103 M−1 cm−1 in ACN), which are somehow characteristic parameters for this class of substances. However, upon addition of DBU (which absorbs in wavelengths lower than 270 nm), a new transient absorption band is formed with fairly complex spectroscopic features, with λmax = 290 and 332 nm; the compound responsible for this absorption, hereafter called A, decays forming another compound, B, which this time absorbs at even higher wavelengths (λmax = 378 nm); this last one is stable throughout the experiment duration. An important point to be noted from the UV spectra is the presence of an isosbestic point at λ = 356 nm; also, as the absorption of those three species is well spaced, they can be followed separately (Fig. 1).
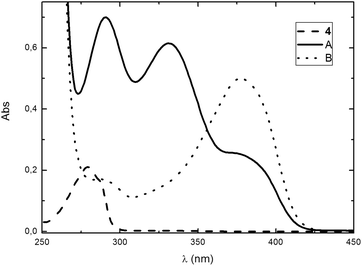 |
| Fig. 1 Absorption spectra of the 2-coumaranone 4, intermediate A and the final product B. | |
A study of the kinetics for the formation of A and B was carried out, and matching rate constants were found for the decomposition of A and the formation of B. Those constants (kobs = 0.01 s−1 and −0.01 s−1 at 25 °C) were shown to be independent from the base concentration. From the determination of the rate constant for the formation of B in different temperatures, an Eyring plot was obtained which led to the determination of the activation parameters for this process, which are: ΔH≠ = 7.85 ± 0.05 kcal mol−1; ΔS≠ = −41 ± 1 cal K−1 mol−1; ΔG≠ = 20.1 ± 0.3 kcal mol−1 at 25 °C.
Emission studies
The addition of DBU to a solution of 4 in ACN showed a bright blue chemiluminescence emission that could be readily observed even in daylight. The rate constant for this emission has been shown to be independent of the concentration of the base (kobs ∼ 0.01 s−1 at 25 °C); through an Eyring plot the following activation parameters were obtained: ΔH≠ = 7.2 ± 0.1 kcal mol−1; ΔS≠ = −45 ± 5 cal K−1 mol−1; ΔG≠ = 20 ± 2 kcal mol−1 at 25 °C.
To confirm the proposed mechanism operating at the chemiexcitation process and also to get clues about the structure of the high-energy intermediate, this chemiluminescent reaction of 4 triggered by the addition of DBU was also studied in different concentrations of perylene (PER) as an activator.
First, emission spectra of the chemiluminescence of 4 in the absence and presence of PER were acquired (data not shown). In the absence of PER, the chemiluminescent emission spectrum has a broad-shaped profile, with a maximum centred at ca. 440 nm; this profile of chemiluminescence matches exactly the fluorescence of the spent reaction mixture. With the addition of PER, the chemiluminescent emission spectrum changes radically, turning into a fine structured emission which, this time, matches the fluorescence of PER.
A linear correlation was indeed observed between the light emission rate constant and the concentration of PER, and a bimolecular rate of kbim = 3.05 ± 0.15 M−1 s−1 (R = 0.996) could be obtained (Table 1), together with the values for the relative quantum yield determined by integration of the chemiluminescent emission (Fig. 2).
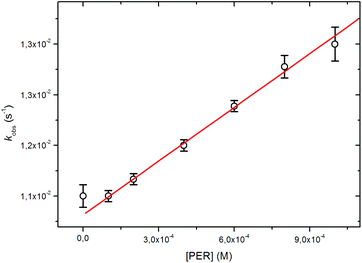 |
| Fig. 2 Linear dependence of the observed rate constant (kobs) with the perylene concentration. | |
Table 1 Observed rate constants (kobs) and relative quantum yields (ΦCL) for the chemiluminescent emission of 4 triggered by DBU in different concentrations of perylene
[PER] (mM) |
k
obs (s−1) |
Φ
CL a (%) |
Conditions: Acetonitrile, 25 °C, [4] = 0.1 mM, [DBU] = 10 mM. Standard deviation of all measurements is <2%. |
0 |
0.0108 ± 0.0002 |
91 |
0.1 |
0.0108 ± 0.0001 |
100 |
0.2 |
0.0111 ± 0.0001 |
99 |
0.4 |
0.0117 ± 0.0001 |
96 |
0.6 |
0.0124 ± 0.0001 |
91 |
0.8 |
0.0131 ± 0.0002 |
86 |
1.0 |
0.0135 ± 0.0003 |
83 |
Discussion
It seems to be certain from experiments in the absence of perylene that kobs for light emission, i.e. the decay rate of the emission, is the rate constant for the decomposition of the intermediate 5, being the rate-determining step.
Since the rate constants for the emission decay and the formation of B or decomposition of A, measured respectively in a fluorescence- and a UV-Vis spectrometer, are essentially the same; we can address A as intermediate 5, while B is the decomposition product.
The activation parameters (Table 2) were obtained by following the kinetics of emission and absorption in different temperatures; matching values for the activation enthalpy and entropy, as well as for the Gibbs free energy, were indeed obtained.
Table 2 Activation parameters for the decomposition of intermediate 5 followed by absorption or light emissiona
|
ΔH≠ (kcal mol−1) |
ΔS≠ (cal mol−1 K−1) |
ΔG≠ at 25 °C (kcal mol−1) |
Conditions: Acetonitrile, 25 °C, [4] = 0.1 mM, [DBU] = 10 mM.
|
Absorption |
7.85 ± 0.05 |
−41 ± 1 |
20.1 ± 0.3 |
Emission |
7.2 ± 0.1 |
−45 ± 5 |
20 ± 2 |
A relatively low value for the activation enthalpy was observed, which is as expected since the peroxidic intermediate 5, a 1,2-dioxetanone, should be very thermal labile. However, a surprisingly very high value for the activation entropy was obtained, indicating that the mechanism of such decomposition is entropically controlled.
A value of ca. −40 cal mol−1 K−1 for the activation entropy could indicate a bimolecular process in which there is an encounter of two different species at the transition state; however, it does not seem to be the case, since a unimolecular decomposition mechanism was postulated for the process of light emission from the decomposition of 1,2-dioxetanones.15 Another possibility is the occurrence of a major structural rearrangement of the compound just before the rate-determining step. This would occur at the molecular geometry level, without prominent cleavage of chemical bonds.
Highly negative activation entropies for the unimolecular decomposition of 1,2-dioxetanones have already been observed, and there is not any accepted explanation for this phenomenon. Baader and de Oliveira16 proposed the existence of a dark decomposition pathway, which is catalyzed by impurities (mainly metal cations), which may be the reason for those negative values of entropy, since the process would have a multimolecular rate-determining step. No formal proposal of this idea has been published until now, although it is backed by experimental evidence; therefore, it remains just a proposition.
The fact that some peroxides may undergo an intramolecular type CIEEL mechanism, producing light emission in high yields, seems to be not the present case. Noting that 5 is a phenolate, able to transfer its charge to the peroxidic ring triggering a chemiluminescent reaction cascade, the ΔS≠ value is far too low when compared to similar systems.17 In addition, when the phenolate is located at the ortho position relative to the peroxide, the quantum yields are then very low,18 and both the situations seem not to be the case here.
The suggestion of a 1,2-dioxetanone as an intermediate in the chemiluminescence of 2-coumaranones should be seriously considered, since the intermediate does interact with an added activator, as other 1,2-dioxetanones do with reasonable rates. The reactivity of 5 with perylene has the value of the bimolecular rate constant kCAT = 3.05 ± 0.15 M−1 s−1. This constant has also been determined by other authors for the 1,2-dioxetanones 6 (3,3-dimethyl-1,2-dioxetanone), 7 (spiro-cyclopentyl-1,2-dioxetanone) and 8 (spiro-adamantyl-1,2-dioxetanone) under slightly different conditions (Table 3).
Table 3 First order decomposition rates (kD) and second-order rates (kCAT) with perylene for some 1,2-dioxetanones
Entry |
Structure |
k
D (10−3 s−1) |
k
CAT (M−1 s−1) |
Determined in this work: ACN, 25 °C.
Ref. 9, determined in CH2Cl2 at 25 °C.
Ref. 19, determined in CH2Cl2 at 27 °C.
Ref. 20, determined in toluene at 25 °C.
Ref. 16, determined in toluene at 50 °C.
|
5
|
|
10.8 ± 0.01a |
3.05 ± 0.15a |
6
|
|
2 ± 1b |
0.247 ± 0.030c |
7
|
|
1.0 ± 0.1d |
1.26 ± 0.06d |
8
|
|
0.25e |
1.81 ± 0.09e |
The decrease of the emission quantum yields with an increase of PER concentration (Table 1) could be explained by the occurrence of its bimolecular interaction with 5. As the PER concentration increases, the proportion of intermolecular CIEEL increases too; however, this type of interaction has markedly low emission quantum yields,9 additionally supporting the proposed general mechanism. A complete determination of the emission (ΦCL) and singlet quantum yields (ΦS) would contribute to confirming the suggestions made in this paper.
Conclusions
In this work we were able to obtain kinetic data for the chemiluminescent reaction of ethyl (5-fluoro-2-oxo-2,3-dihydrobenzofuran-3-yl) carbamate with a base and oxygen. They point to the existence of a peroxidic intermediate, which is probably a 1,2-dioxetanone with low thermal stability. The data obtained also confirmed the viability of generating 1,2-dioxetanones and studying their reactivity towards reducing compounds in situ without the need of isolation and purification.
Acknowledgements
The authors thank the Fundação de Amparo à Pesquisa do Estado de São Paulo (FAPESP; LFMLC 2012/02428-5 and FHB 2012/13807-7) for financial support.
Notes and references
-
W. J. Baader, C. V. Stevani and E. L. Bastos, in The Chemistry of peroxides, ed. Z. Rappoport, Wiley & Sons, Chichester, 2006, vol. 2, ch. 16, p. 1211 Search PubMed
.
- J.-Y. Koo, S. P. Schmidt and G. B. Schuster, Bioluminescence of the firefly: Key steps in the formation of the electronically excited state for model systems, Proc. Natl. Acad. Sci. U. S. A., 1978, 75, 30 CrossRef CAS
; Y. Ando, K. Niwa, N. Yamada, T. Enomoto, T. Irie, H. Kubota, Y. Ohmiya and H. Akiyama, Firefly bioluminescence quantum yield and colour change by pH-sensitive green emission, Nat. Photonics, 2008, 2, 44 CrossRef
.
- M. Adamczyk, Y.-Y. Chen, D. D. Johnson, P. G. Mattingly, J. A. Moore, Y. Pan and R. E. Reddy, Chemiluminescent acridinium-9-carboxamide boronic acid probes: Application to a homogeneous glycated hemoglobin assay, Bioorg. Med. Chem. Lett., 2006, 16, 1324 CrossRef CAS PubMed
.
- F. H. Bartoloni, M. A. de Oliveira, F. A. Augusto, L. F. M. L. Ciscato, E. L. Bastos and W. J. Baader, Synthesis of Unstable Cyclic Peroxides for Chemiluminescence Studies, J. Braz. Chem. Soc., 2012, 23, 2093 CAS
.
- G. B. Schuster and S. P. Schmidt, Chemiluminescence of Organic Compounds, Adv. Phys. Org. Chem., 1982, 18, 187 CrossRef CAS
; G. B. Schuster, Chemiluminescence of Organic Peroxides. Conversion of Ground-State Reactants to Excited-State Products by the Chemically Initiated Electron-Exchange Luminescence Mechanism, Acc. Chem. Res., 1979, 12, 366 CrossRef
; J.-Y. Koo and G. B. Schuster, Chemiluminescence of Diphenoyl Peroxide. Chemically Initiated Electron Exchange Luminescence. A New General Mechanism for Chemical Production of Electronically Excited States, J. Am. Chem. Soc., 1978, 100, 4496 CrossRef
; J.-Y. Koo and G. B. Schuster, Dioxetane Chemiluminescence. The Effect of Deuterium Substitution on the Thermal Decomposition of trans-3,4-Diphenyl-1,2-dioxetane, J. Am. Chem. Soc., 1977, 99, 5403 CrossRef
.
- L. Yue, Y. J. Liu and W. H. Fang, Mechanistic Insight into the Chemiluminescent Decomposition of Firefly Dioxetanone, J. Am. Chem. Soc., 2012, 134, 11632 CrossRef CAS PubMed
; H. Isobe, Y. Takano, M. Okumura, S. Kuramitsu and K. Yamaguchi, Mechanistica Insights in Charge-Transfer-Induced Luminescence of 1,2-Dioxetanones with a Substituent of Low Oxidation Potential, J. Am. Chem. Soc., 2005, 127, 8667 CrossRef PubMed
; L. P. da Silva and J. C. G. E. da Silva, Interstate Crossing-Induced Chemiexcitation as the reason for the Chemiluminescence of Dioxetanones, ChemPhysChem, 2013, 14, 1071 CrossRef PubMed
.
- L. F. M. L. Ciscato, F. H. Bartoloni, D. Weiss, R. Beckert and W. J. Baader, Experimental Evidence of the Occurrence of Intramolecular Electron Transfer in Catalyzed 1,2-Dioxetane Decomposition, J. Org. Chem., 2010, 75, 6574 CrossRef CAS PubMed
.
- E. L. Bastos, S. M. da Silva and W. J. Baader, Solvent Cage Effects: Basis of a General Mechanism for Efficient Chemiluminescence, J. Org. Chem., 2013, 78, 4432 CrossRef CAS PubMed
.
- M. A. de Oliveira, F. H. Bartoloni, F. A. Augusto, L. F. M. L. Ciscato, E. L. Bastos and W. J. Baader, Revision of Singlet Quantum Yields in the Catalyzed Decomposition of Cyclic Peroxides, J. Org. Chem., 2012, 77, 10537 CrossRef PubMed
.
- L. F. M. L. Ciscato, F. H. Bartoloni, E. L. Bastos and W. J. Baader, Experimental Evidence of the Occurrence of Intramolecular Electron Transfer in Catalyzed 1,2-Dioxetane Decomposition, J. Org. Chem., 2009, 74, 8974 CrossRef CAS PubMed
.
- G. J. Lofthouse, H. Suschitzky, B. J. Wakefield and R. A. Whittaker, Synthesis and Chemiluminescent Reactions of Some 3-Alkoxycarbamoyl-benzo[b]furan-2(3H)-ones, J. Chem. Soc., Perkin Trans. 1, 1979, 1634 RSC
.
- S. Schramm, D. Weiss, I. Navizet, D. Roca-Sanjuán, H. Brandl, R. Beckert and H. Görls, Investigations on the synthesis and chemiluminescence of novel 2-coumaranones, ARKIVOC, 2013, iii, 174 CrossRef
.
-
S. L. Murov, I. Carmichael and G. L. Hug, in Handbook of Photochemistry, Marcel Dekker, New York, 1993, 2nd edn, p. 293 Search PubMed
.
- L. F. M. L. Ciscato, D. Weiss, R. Beckert, E. L. Bastos, F. H. Bartoloni and W. J. Baader, Chemiluminescence-based uphill energy conversion, New J. Chem., 2011, 35, 773 RSC
.
-
W. Adam, in Chemical and Biological Generation of Excited States, ed. W. Adam and G. Cilento, Academic Press, New York, 1982, ch. 4, pp. 115–152 Search PubMed
.
-
M. A. de Oliveira, Ph.D. Thesis, University of São Paulo, 2007 Search PubMed
.
- L. F. M. L. Ciscato, D. Weiss, R. Beckert and W. J. Baader, Fenchyl substituted 1,2-dioxetanes as an alternative to adamantyl derivatives for bioanalytical applications, J. Photochem. Photobiol., A, 2011, 218, 41 CrossRef CAS PubMed
.
- W. Adam and A. V. Trofimov, The Effect of meta versus para Substitution on the Efficiency of Chemiexcitation in the Chemically Triggered Electron-Transfer-Initiated Decomposition of Spiroadamantyl Dioxetanes, J. Org. Chem., 2000, 65, 6474 CrossRef CAS
.
- W. Adam and O. Cueto, Fluorescer-Enhanced Chemiluminescence of α-Peroxylactones via Electron Exchange, J. Am. Chem. Soc., 1979, 101, 6511 CrossRef CAS
.
-
F. H. Bartoloni, M. A. de Oliveira, L. F. M. L. Ciscato, F. A. Augusto, E. L. Bastos and W. J. Baader, Experimental evidence of the occurrence of an intermolecular electron transfer in the catalysed decomposition of spiro-alkyl-1,2-dioxetanones, manuscript in preparation.
Footnote |
† Electronic supplementary information (ESI) available: Kinetic data in all conditions used. See DOI: 10.1039/c3pp50345c |
|
This journal is © The Royal Society of Chemistry and Owner Societies 2014 |
Click here to see how this site uses Cookies. View our privacy policy here.