DOI:
10.1039/C3CY00719G
(Communication)
Catal. Sci. Technol., 2014,
4, 38-42
Efficient and convenient oxidation of sulfides to sulfones using H2O2 catalyzed by V2O5 in ionic liquid [C12mim][HSO4]
Received
22nd September 2013
, Accepted 26th October 2013
First published on 11th November 2013
Abstract
A simple, efficient, and eco-friendly procedure for the oxidation of sulfides to sulfones using H2O2 catalyzed by V2O5 in ionic liquid [C12mim][HSO4] has been developed. This atom-economical protocol affords the target products in good to high yields. The products can be separated by a simple extraction with organic solvent, and the catalytic system can be recycled and reused without loss of catalytic activity.
Sulfones are an important class of chemicals that are used extensively for the preparation of a variety of fine or special chemicals such as drugs, natural products, and catalysts.1,2 In particular, sulfones are important building blocks for the synthesis of various biologically active molecules.2 Therefore, there has been tremendous interest in developing efficient methods for the synthesis of these molecules, and a well known method constitutes the oxidation of sulfides. Traditional methods for performing such a transformation generally involve the use of stoichiometric amounts of the strongest oxidizing reagents (i.e., HNO3, KMnO4, MnO2, etc.)3 and suffer from considerable drawbacks such as high cost, low yield, harsh or delicate reaction conditions, and a large amount of waste byproducts. Oxidation using potassium hydrogen persulfate,4 NaClO4,5 H5IO6,6 NaClO,7 UHP,8m-chloroperbenzoic acid,9 NaIO4,10 dimethyldioxirane,11 oxone12 and other reagents13 has also been developed for this conversion. However, these protocols are generally associated with one or more disadvantages, such as long reaction times, high temperatures, low yields, complex handling procedures, problematic side reactions, the use of expensive, toxic and moisture sensitive reagents, and environmental concerns associated with the use of poorly manageable catalysts. Hydrogen peroxide (H2O2) is an economical, environmentally friendly and atom-efficient oxidant which leads to only water after the reaction.14 This reagent has, however, high activation energy, making catalysis necessary,15 and a number of catalytic oxidation processes based on the combination of transition metals or organocatalysts and H2O2 have been developed for such a conversion.16 However, most of these methods still suffered from the use of expensive reagents, lower yields, long reaction times, environmental hazards, poor recovery of expensive metal catalysts and laborious workup procedures. Consequently, there is a great need to develop new and environment-benign procedures that address these drawbacks for such a conversion.
Ionic liquids (ILs) are a class of organic salts with unusually low melting temperatures that have attracted much attention from the scientific community in recent years. As a class of potential greener solvents, ILs exhibit many unique physicochemical properties, such as negligible volatility and nonflammability under ambient conditions, large liquid range, high thermal stability, wide electrochemical window, and strong ability to dissolve many chemicals.17,18 Therefore, ILs have found wide applications in chemical synthesis,19 biocatalytic transformations,20 electrochemistry,21 and analytical and separation science.22 In continuation of our interest in exploring green oxidation reactions in ionic liquids, we report herein a new, efficient and environmentally friendly protocol for the oxidation of sulfides to sulfones using H2O2 catalyzed by vanadium pentoxide (V2O5) in the ionic liquid 1-dodecyl-3-methylimidazolium hydrogensulfate ([C12mim][HSO4]) under mild conditions (Scheme 1). Furthermore, we demonstrate that the catalytic system can be recycled and reused without any significant loss of catalytic activity.
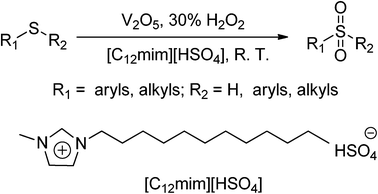 |
| Scheme 1 Catalytic oxidation of sulfides to sulfones. | |
The initial study was carried out using methyl(phenyl)sulfane as substrate to optimize the reaction conditions, and the results are summarized in Table 1. Initial reaction screening led to disappointing results. In the absence of catalyst and ionic liquid, the reaction proceeded very slowly, and the yield was only 20% after 24 h (Table 1, entry 1). The results mean that the oxidant H2O2 alone does not work effectively in the reaction. The effects of different ionic liquids, such as [C6mim][HSO4], [C10mim][HSO4], [C12mim][HSO4], [C12mim][PF6], [C12mim][OTf], [C12mim][BF4], and [C12mim]Br, were tested with V2O5 as catalyst in the reaction (Table 1, entries 2–8), and it was observed that [C12mim][HSO4] demonstrated the best performance (Table 1, entry 4). The different effects of ILs on the reaction may be attributed to their different abilities to stabilize and dissolve the oxidant H2O2 and the catalyst. Under the same conditions, the IL that stabilizes and dissolves them more easily will lead to a larger increase in the effective reactant concentration, which increases the encounter probabilities between the substrate and the reactive species; thus, a higher rate or yield of the reaction is observed. For a blank test (Table 1, entry 11), a lower yield of the product was obtained when the same reaction conditions were used in the absence of ionic liquid. In addition, the catalyst is crucial for this reaction, and a lack of it leads to a much lower yield (Table 1, entry 12).
Table 1 Optimization of the conditions for the oxidation of methyl(phenyl)sulfane using H2O2a
Entry |
Ionic liquid |
Catalyst |
Time/h |
Yieldb/(%) |
The reactions were carried out using methyl(phenyl)sulfane (10 mmol), catalyst (2 mmol), IL (10 mL), and H2O2 (30%, 25 mmol) at room temperature (25 °C).
Isolated yield.
The first run.
The second run.
|
1 |
— |
— |
24 |
20 |
2 |
[C6mim][HSO4] |
V2O5 |
6 |
75 |
3 |
[C10mim][HSO4] |
V2O5 |
4 |
87 |
4 |
[C12mim][HSO4] |
V2O5 |
4 |
90 |
5 |
[C12mim][PF6] |
V2O5 |
4 |
82 |
6 |
[C12mim][OTf] |
V2O5 |
4 |
79 |
7 |
[C12mim][BF4] |
V2O5 |
4 |
74 |
8 |
[C12mim]Br |
V2O5 |
6 |
70 |
9 |
[C12mim][HSO4] |
V2O5 |
4 |
90c |
10 |
[C12mim][HSO4] |
V2O5 |
4 |
89d |
11 |
— |
V2O5 |
8 |
59 |
12 |
[C12mim][HSO4] |
— |
8 |
68 |
13 |
[C12mim][HSO4] |
MnO2 |
4 |
76 |
14 |
[C12mim][HSO4] |
ZnO |
4 |
65 |
15 |
[C12mim][HSO4] |
Fe2O3 |
4 |
84 |
16 |
[C12mim][HSO4] |
CuO |
4 |
72 |
Besides V2O5, we also tried to use other types of catalysts in this model reaction (Table 1, entries 13–16), and the results showed that V2O5 demonstrated the best performance in terms of yield and reaction rate. Furthermore, the catalytic system could be typically recovered and reused with no appreciable decrease in yield and reaction rate (Table 1, entries 9 and 10). Therefore, the combination of H2O2, V2O5 and [C12mim][HSO4] was chosen as the optimal condition for further exploration.
With these results in hand, we subjected other sulfides to the oxidation reactions, and the results are listed in Table 2. It is clear that various types of aryl and alkyl sulfides can be successfully oxidized to the corresponding sulfones in good to high yields (Table 2). Various types of aryl-alkyl and alkyl sulfides can be successfully oxidized to the corresponding sulfones (Table 2, entries 1–6 and 10); because the aryl sulfides were less reactive, more drastic reaction conditions were needed to achieve good yields (Table 2, entries 7–9). It was also observed that the electronic nature of the substituents on the aromatic ring has some impact on the reaction rate. Aryl-alkyl sulfides, especially those with electron-donating substituents (Table 2, entries 3 and 4), were more reactive than those with electron-withdrawing substituents (Table 2, entries 5 and 6), providing excellent yields.
Table 2 Catalytic oxidation of sulfides to sulfonesa
Entry |
Substrate |
Product |
Time/h |
Yield/(%)b |
Unless otherwise noted, the reactions were carried out using sulfide (10 mmol), V2O5 (2 mmol), [C12mim][HSO4] (10 mL), and H2O2 (30%, 25 mmol) at room temperature.
Isolated yield.
The reaction was carried out at 45 °C.
|
1 |
|
|
4 |
90 |
2 |
|
|
4 |
94 |
3 |
|
|
4 |
93 |
4 |
|
|
4 |
95 |
5 |
|
|
6 |
83 |
6 |
|
|
6 |
85 |
7 |
|
|
8 |
84c |
8 |
|
|
8 |
87c |
9 |
|
|
8 |
82c |
10 |
|
|
4 |
93 |
According to the literature23 and the observations in our reactions, taking the oxidation of methyl(phenyl)sulfane with H2O2 as an example, a possible mechanism is proposed (Scheme 2). In the reaction, the catalyst V2O5 provides a source of VO3− and VO2+ (1), and 1 reacts with the oxidant H2O2 to form the red oxoperoxo VO(O2)+ (2) and the VO(O2)OOH species (3). Then, 3 reacts with the substrate to form the transition state 4. Then, 4 eliminates the oxoperoxo (2) and water to yield the intermediate substrate 5, which reacts with 3 to form the transition state 6. 6 then very rapidly affords 2 and water to yield the desired product. 2 is then re-oxidized to 3 by H2O2 to complete the catalytic cycle. It appears that the formations of 4 and 6 are the rate-determining steps.
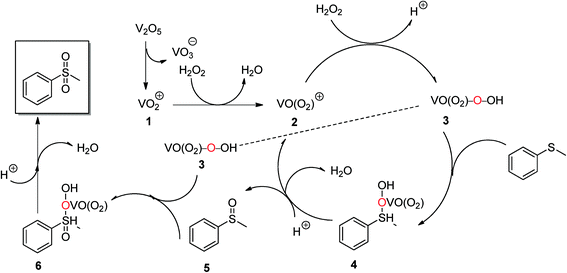 |
| Scheme 2 Possible mechanism for the oxidation of methyl(phenyl)sulfane using H2O2. | |
In conclusion, we have demonstrated an efficient and convenient oxidation of sulfides to sulfones using the H2O2–V2O5 catalytic system in the ionic liquid [C12mim][HSO4]. Compared to the synthetic methods reported in the literature,3–13,16 not only were the yields of products enhanced or greatly improved but the operating units were also easily worked up. Mild reaction conditions, simplicity of operation, high yields, stability, easy isolation of products, and excellent recyclability of the catalytic system are the attractive features of this methodology. The scope, definition of the mechanism and synthetic application of this reaction are currently under study in our laboratory.
Experimental
All the chemicals were obtained from commercial sources without any pretreatment. All reagents were of analytical grade. The ionic liquids were synthesized according to the procedure in the literature.181H NMR spectra were recorded using a Bruker 500 MHz spectrometer with CDCl3 as solvent and tetramethylsilane (TMS) as internal standard. High performance liquid chromatography (HPLC) experiments were performed using a liquid chromatograph (Dionex Softron GmbH, America) consisting of a pump (P680) and an ultraviolet–visible light detector (UVD) system (170U). The experiments were performed on a Discovery C18 column (ø 4.6 × 250 mm). Elemental analysis was performed using a Vario EL III instrument (Elementar Analysensysteme GmbH, Germany).
General procedure for oxidation reactions.
For a typical experiment, to 10 mL of [C12mim][HSO4] were sequentially added sulfide (10 mmol), V2O5 (2 mmol), and an aqueous solution of H2O2 (30%, 25 mmol). The obtained mixture was stirred vigorously at room temperature for an appropriate time (Table 2), and the reaction progress was monitored by HPLC. Upon completion of the reaction, ethyl acetate was used to extract the organic compounds (3 × 20 mL). The ethyl acetate solution was washed with water (3 × 20 mL) and then dried over anhydrous Na2SO4. The solvent was removed, and the residue was distilled under vacuum to give the desired pure product. The rest of the ionic liquid and the catalyst were recovered by decantation of water produced in the reaction and concentration under vacuum. Fresh substrates were then recharged to the recovered catalytic system and then recycled under identical reaction conditions. The target substrates were characterized by 1H NMR and elemental analysis or compared with their authentic samples. Spectroscopic data for selected products are as follows.
1-Methyl-4-(methylsulfonyl)benzene (Table 2, entry 3).
1H NMR (500 MHz, CDCl3): δ (ppm) = 2.46 (s, 3H, CH3), 3.57 (s, 3H, CH3), 7.37–7.42 (m, 2H, Ar–H), 7.86–7.91 (m, 2H, Ar–H); elemental analysis % calc. (% found): C 56.42 (56.44), H 5.90 (5.92), O 18.76 (18.80), S 18.81 (18.84).
1-Methoxy-4-(methylsulfonyl)benzene (Table 2, entry 4).
1H NMR (500 MHz, CDCl3): δ (ppm) = 3.61 (s, 3H, CH3), 3.87 (s, 3H, CH3), 7.21–7.25 (m, 2H, Ar–H), 7.83–7.87 (m, 2H, Ar–H); elemental analysis % calc. (% found): C 51.55 (51.60), H 5.39 (5.41), O 25.74 (25.77), S 17.18 (17.22).
2,3-Dihydrobenzo[b]thiophene 1,1-dioxide (Table 2, entry 8).
1H NMR (500 MHz, CDCl3): δ (ppm) = 3.25 (t, 2H, CH2), 3.83 (t, 2H, CH2), 7.45–7.78 (m, 4H, Ar–H); elemental analysis % calc. (% found): C 57.09 (57.12), H 4.77 (4.79), O 18.98 (19.02), S 19.04 (19.06).
Dibenzo[b,d]thiophene 5,5-dioxide (Table 2, entry 9).
1H NMR (500 MHz, CDCl3): δ (ppm) = 7.56–7.63 (m, 4H, Ar–H), 7.92–8.05 (m, 4H, Ar–H); elemental analysis % calc. (% found): C 66.61 (66.65), H 3.68 (3.73), O 14.79 (14.80), S 14.77 (14.83).
Acknowledgements
We thank the Natural Science Foundation of Jiangsu Province and the Science and Technology Program of Jiangxi Provincial Education Bureau (no. GJJ13535) for supporting this research.
References
- E. N. Prilezhaeva, Russ. Chem. Rev., 2000, 69, 367 CrossRef CAS PubMed;
S. Patai and Z. Rappoport, Synthesis of Sulfones, Sulfoxides and Cyclic Sulfides, John Wiley, Chichester, 1994 RSC; R. L. Atienza, H. S. Roth and K. A. Scheidt, Chem. Sci., 2011, 2, 1772 RSC; R. Chawla, R. Kapoor, A. K. Singh and L. D. S. Yadav, Green Chem., 2012, 14, 1308 RSC; F. Chemla, J. Chem. Soc., Perkin Trans. 1, 2002, 275 RSC; P. H. Bos, B. Maciá, M. Á. Fernández-Ibáñez, A. J. Minnaard and B. L. Feringa, Org. Biomol. Chem., 2010, 8, 47 Search PubMed; B. L. Yin, Y. X. Zhang and L. W. Xu, Synthesis, 2010, 3583 CrossRef PubMed; A. N. R. Alba, X. Companyó and R. Rios, Chem. Soc. Rev., 2010, 39, 2018 RSC.
- K. Fujiki, S. Akieda, H. Yasuda and Y. Sasaki, Synthesis, 2001, 1035 CrossRef CAS PubMed; N. S. Zefirov, N. V. Zyk, E. K. Beloglazkina and A. G. Kutateladze, Sulfur Rep., 1993, 14, 223 CrossRef; C. M. Rayner, Contemp. Org. Synth., 1994, 1, 191 RSC; E. N. Prilezhaeva, Russ. Chem. Rev., 2001, 70, 897 CrossRef PubMed.
- T. Durst, J. Am. Chem. Soc., 1969, 91, 1034 CrossRef CAS; D. Edwards and J. B. Stenlake, J. Chem. Soc., 1954, 3272 RSC; G. W. Gokel, H. M. Gerdes and D. M. Dishong, J. Org. Chem., 1980, 45, 3634 CrossRef; A. Shaabania, P. Mirzaeia, S. Naderia and D. G. Leeb, Tetrahedron, 2004, 60, 11415 CrossRef PubMed; G. Agnihotri and A. K. Misra, Carbohydr. Res., 2006, 341, 275 CrossRef PubMed.
- B. M. Trost and D. P. Curran, Tetrahedron Lett., 1981, 22, 1287 CrossRef CAS.
- J. M. Khurana, A. K. Panda, A. Ray and A. Gogia, Org. Prep. Proced. Int., 1996, 28, 234 CrossRef CAS.
- L. Xu, J. Cheng and M. L. Trudell, J. Org. Chem., 2003, 68, 5388 CrossRef CAS PubMed; D. H. R. Barton, W. Li and J. A. Smith, Tetrahedron Lett., 1998, 39, 7055 CrossRef.
- N. Fukuda and T. Ikemoto, J. Org. Chem., 2010, 75, 4629 CrossRef CAS PubMed.
- R. S. Varma and K. P. Naicker, Org. Lett., 1999, 1, 189 CrossRef CAS; H. Q. N. Gunaratne, M. A. McKervey, S. Feutren, J. Finlay and J. Boyd, Tetrahedron Lett., 1998, 39, 5655 CrossRef; R. Balicki, J. Prakt. Chem., 1999, 341, 184 CrossRef; R. Balicki, Synth. Commun., 1999, 29, 2235 CrossRef.
- N. K. Jana and J. G. Verkade, Org. Lett., 2003, 5, 3787 CrossRef CAS PubMed; R. J. Gruffin, A. Henderson, N. J. Curtin, A. Echalier, J. A. Endicott, I. R. Hardcastle, D. R. Newell, M. E. M. Noble, L. Z. Wang and B. T. Golding, J. Am. Chem. Soc., 2006, 128, 6012 CrossRef PubMed; A. Dishington, S. Fillery and M. R. V. Finlay, Tetrahedron Lett., 2010, 51, 4211 CrossRef PubMed.
- R. S. Varma, R. K. Saini and H. M. Meshram, Tetrahedron Lett., 1997, 38, 6525 CrossRef CAS.
- P. Hanson, R. A. A. J. Hendrickx and J. R. L. Smith, New J. Chem., 2010, 34, 65 RSC; P. Hanson, R. A. A. J. Hendrickx and J. R. L. Smith, Org. Biomol. Chem., 2008, 6, 745 Search PubMed.
- B. Yu, A. H. Liu, L. N. He, B. Li, Z. F. Diao and Y. N. Li, Green Chem., 2012, 14, 957 RSC.
- M. Hashmat Ali and G. J. Bohnert, Synth. Commun., 1998, 28, 2983 CrossRef CAS; M. Hashmat Ali and G. J. Bohnert, Synthesis, 1998, 1238 Search PubMed; P. J. Kropp, G. W. Breton, J. D. Fields, J. C. Jung and B. R. Loomis, J. Am. Chem. Soc., 2000, 122, 4280 CrossRef; S. X. Ying and W. J. Fa, J. Mol. Catal. A: Chem., 2008, 280, 142 CrossRef PubMed; N. Iranpoor, D. Mohajer and A. R. Rezaeifard, Tetrahedron Lett., 2004, 45, 3811 CrossRef PubMed; B. M. Choudary, C. R. V. Reddy, B. V. Prakash, M. L. Kantam and B. Sreedhar, Chem. Commun., 2003, 754 RSC; A. Ghaemi, S. Rayati, S. Zakavi and N. Safari, Appl. Catal., A, 2009, 353, 154 CrossRef PubMed; M. V. Gómez, R. Caballero, E. Vázquez, A. Moreno, A. de la Hoz and Á. Díaz-Ortiz, Green Chem., 2007, 9, 331 RSC; A. de León, J. García-Antón, J. Ros, G. Guirado, I. Gallardo and J. Pons, New J. Chem., 2013, 37, 1889 RSC; M. M. Savant, A. M. Pansuriya, C. V. Bhuva, N. Kapuriya, A. S. Patel, V. B. Audichya, P. V. Pipaliya and Y. T. Naliapar, J. Comb. Chem., 2010, 12, 176 CrossRef PubMed; S. R. Livingston and C. C. Landry, J. Am. Chem. Soc., 2008, 130, 13214 CrossRef PubMed; S. P. Das, J. J. Boruah, H. Chetry and N. S. Islam, Tetrahedron Lett., 2012, 53, 1163 CrossRef PubMed.
-
C. W. Jones and J. H. Clark, Applications of hydrogen peroxide and derivatives, Royal Society of Chemistry, UK, 1999 Search PubMed.
- L. Marinescu, M. Molbach, C. Rousseau and M. Bols, J. Am. Chem. Soc., 2005, 127, 17578 CrossRef CAS PubMed.
- M. Kirihara, A. Itou, T. Noguchi and J. Yamamoto, Synlett, 2010, 1557 CrossRef CAS PubMed; K. Bahrami, M. M. Khodaei and M. S. Arabi, J. Org. Chem., 2010, 75, 6208 CrossRef PubMed; B. Karimi, M. Ghoreishi-Nezhad and J. H. Clark, Org. Lett., 2005, 7, 625 CrossRef PubMed; F. Gregori, I. Nobili, F. Bigi, R. Maggi, G. Predieri and G. Sartori, J. Mol. Catal. A: Chem., 2008, 286, 124 CrossRef PubMed; A. Rostami and J. Akradi, Tetrahedron Lett., 2010, 51, 3501 CrossRef PubMed; X. L. Xue, W. Zhao, B. C. Ma and Y. Ding, Catal. Commun., 2012, 29, 73 CrossRef PubMed; X. Y. Shi, X. Y. Han, W. J. Ma, J. F. Wei, J. Li, Q. Zhang and Z. G. Chen, J. Mol. Catal. A: Chem., 2011, 341, 57 CrossRef PubMed; S. P. Das, J. J. Boruah, N. Sharma and N. S. Islam, J. Mol. Catal. A: Chem., 2012, 356, 36 CrossRef PubMed; W. Al-Maksoud, S. Daniele and A. B. Sorokin, Green Chem., 2008, 10, 447 RSC; S. M. G. Pires, M. M. Q. Simões, I. C. M. S. Santos, S. L. H. Rebelo, M. M. Pereira, M. G. P. M.
S. Neves and J. A. S. Cavaleiro, Appl. Catal., A, 2012, 439–440, 51 CrossRef PubMed; S. K. Karmee, L. Greiner, A. Kraynov, T. E. Müller, B. Niemeijer and W. Leitner, Chem. Commun., 2010, 6705 RSC.
- M. Saravanababu and J. L. Robert, Curr. Org. Synth., 2005, 2, 437 CrossRef CAS; M. Petkovic, K. R. Seddon, L. P. N. Rebelo and C. S. Pereira, Chem. Soc. Rev., 2011, 40, 1383 RSC; F. Dommert, K. Wendler, R. Berger, L. D. Site and C. Holm, ChemPhysChem, 2012, 13, 1625 CrossRef PubMed.
-
S. J. Zhang and X. M. Lu, Ionic liquids: from fundamental research to industrial applications, Science Press, Beijing, 2006 Search PubMed.
- N. V. Plechkova and K. R. Seddon, Chem. Soc. Rev., 2008, 37, 123 RSC;
P. Wasserschein and T. Welton, Ionic liquids in synthesis, Wiley-VCH, Weinheim, 2008 Search PubMed; J. M. Andanson, S. Marx and A. Baiker, Catal. Sci. Technol., 2012, 2, 1403 Search PubMed; A. Rouch, T. Castellan, I. Fabing, N. Saffon, J. Rodriguez, T. Constantieux, J. C. Plaquevent and Y. Génisson, RSC Adv., 2013, 3, 413 RSC; B. Zhang, M. D. Zhou, M. Cokoja, J. Mink, S. L. Zang and F. E. Kühn, RSC Adv., 2012, 2, 8416 RSC; M. A. P. Martins, C. P. Frizzo, D. N. Moreira, N. Zanatta and H. G. Bonacorso, Chem. Rev., 2008, 108, 2015 CrossRef CAS PubMed; N. Ferlin, M. Courty, S. Gatard, M. Spulak, B. Quilty, I. Beadham, M. Ghavre, A. Haiß, K. Kümmerer, N. Gathergood and S. Bouquillon, Tetrahedron, 2013, 69, 6150 CrossRef PubMed; M. Vafaeezadeh and M. M. Hashemi, Chem. Eng. J., 2013, 221, 254 CrossRef PubMed; S. Saravanamurugan and A. Riisager, Catal. Today, 2013, 200, 94 CrossRef PubMed; Q. Zhang, S. Zhang and Y. Deng, Green Chem., 2011, 13, 2619 RSC.
- A. R. Hajipour, L. Khazdooz and A. E. Ruoho, Catal. Commun., 2008, 9, 89 CrossRef CAS PubMed; F. van Rantwijk, R. M. Lau and R. A. Sheldon, Trends Biotechnol., 2003, 21, 131 CrossRef; N. Wood, J. L. Ferguson, H. Q. N. Gunaratne, K. R. Seddon, R. Goodacre and G. M. Stephens, Green Chem., 2011, 13, 1843 RSC.
- P. Hapiot and C. Lagrost, Chem. Rev., 2008, 108, 2238 CrossRef CAS PubMed; S. Fu, S. Gong, C. Liu, L. Zheng, W. Feng, J. Nie and Z. Zhou, Electrochim. Acta, 2013, 94, 229 CrossRef PubMed; J. Reiter, E. Paillard, L. Grande, M. Winter and S. Passerini, Electrochim. Acta, 2013, 91, 101 CrossRef PubMed.
- H. N. Abdelhamid, J. Gopal and H. F. Wu, Anal. Chim. Acta, 2013, 767, 104 CrossRef CAS PubMed; M. D. Joshi and J. L. Anderson, RSC Adv., 2012, 2, 5470 RSC; P. Sun and D. W. Armstrong, Anal. Chim. Acta, 2010, 661, 1 CrossRef PubMed; S. Stefan and S. Piotr, Curr. Org. Chem., 2011, 15, 1871 CrossRef; Y. Tang, A. Sun, R. Liu and Y. Zhang, Anal. Chim. Acta, 2013, 767, 148 CrossRef PubMed.
- A. Butler, M. J. Clague and G. E. Meister, Chem. Rev., 1994, 94, 625 CrossRef CAS; R. Z. Khaliullin, A. T. Bell and M. Head-Gordon, J. Phys. Chem. B, 2005, 109, 17984 CrossRef PubMed; G. B. Shul'pin, Y. N. Kozlov, G. V. Nizova, G. Süss-Fink, S. Stanislas, A. Kitaygorodskiy and V. S. Kulikova, J. Chem. Soc., Perkin Trans. 2, 2001, 1351 RSC; G. B. Shul'pin, D. Attanasio and L. Suber, J. Catal., 1993, 142, 147 CrossRef; A. M. Kirillov and G. B. Shul'pin, Coord. Chem. Rev., 2013, 257, 732 CrossRef PubMed; M. V. Kirillova, M. L. Kuznetsov, V. B. Romakh, L. S. Shul'pina, J. J. R. F. da Silva, A. J. L. Pombeiro and G. B. Shul'pin, J. Catal., 2009, 267, 140 CrossRef PubMed; Y. N. Kozlov, V. B. Romakh, A. Kitaygorodskiy, P. Buglyó, G. Süss-Fink and G. B. Shul'pin, J. Phys. Chem. A, 2007, 111, 7736 CrossRef PubMed; C. Bolm and D. C. F. Bienewald, Angew. Chem., Int. Ed. Engl., 1996, 34, 2640 CrossRef; A. Coletti, P. Galloni, A. Sartorel, V. Conte and B. Floris, Catal. Today, 2012, 192, 44 CrossRef PubMed; Q. Zeng, H. Wang, T. Wang, Y. Cai, W. Weng and Y. Zhao, Adv. Synth. Catal., 2005, 347, 1933 CrossRef; V. Conte and B. Floris, Inorg. Chim. Acta, 2010, 363, 1935 CrossRef PubMed.
|
This journal is © The Royal Society of Chemistry 2014 |
Click here to see how this site uses Cookies. View our privacy policy here.