DOI:
10.1039/C3BM60312A
(Paper)
Biomater. Sci., 2014,
2, 990-995
Chalcone coating on cotton cloth – an approach to reduce attachment of live microbes†
Received
10th December 2013
, Accepted 21st December 2013
First published on 26th February 2014
Abstract
Drug resistant bacteria are a major threat to humans, especially those which mediate nosocomial infections. In this paper, three different 4-sulfonylmethyl chalcones are coated onto cotton cloths with acacia as the binder using a padding mangle to make them antibacterial. A 99% reduction in the adhesion of three slime producing organisms, namely Staphylococcus aureus NCIM5021, Escherichia coli NCIM2931 and Pseudomonas aeruginosa NCIM2901, on these surfaces was observed. The coated surfaces are more hydrophobic than the original one. The attachment of the bacteria (CFU ml−1) to the cloth is directly proportional (correlation coefficient, R = 0.58) to the hydrophobicity of the surface of the microorganism. The extent of bacterial attachment on the cloths (CFU ml−1) is not correlated with the minimum inhibitory concentration (MIC) of the chalcones (R = −0.1), but on the other hand it is negatively correlated with the hydrophilicity of the coated cloth (R = −0.52). This indicates that hydrophilic surfaces prevent bacterial attachment and hydrophobic organisms have a greater propensity to attach to hydrophobic surfaces than hydrophilic ones. A simple multi-linear regression model with the surface hydrophobicity of the organism and the hydrophilicity of the cloth is able to predict the extent of bacterial attachment. This study suggests that the coated cloths could find applications in hospital environments.
Introduction
Health care personnel face the major threat of communicable diseases, mainly from bacteria, fungi and viruses. Blood and infectious body fluids upon contact with humans lead to bacterial and fungal infections.1,2 Beds, bed covers and curtains in hospitals that come into contact with patients also mediate the spread of pathogens. The incidence of hospital infections acquired by patients and visitors is very high. These infections very often spread through the transmission of bacteria asymptomatically or symptomatically from one patient to the other, or to a visitor, which may lead to the colonization of the strain thereby causing the infection.3 Preventive measures include hand washing and prophylactic consumption of antibiotics to terminate the colonization of the bacteria. Bacteria acquire resistance to antibiotics with time and to combat the resistance, the drug used in the treatment is applied in cycles or a mixture of antibacterial agents is applied.3
Pseudomonas aeruginosa is the most commonly isolated organism and it accounts for 10% of nosocomial infections. Staphylococcus aureus is another major cause of nosocomial infections and is related to surgical wounds and infections due to medical devices.4 MRSA (methicillin resistant Staphylococcus aureus) is also a major threat and the prevalence of MRSA colonization is found to be 52% for patients who stay for ten or more days in a hospital.5
Chalcones are very well known antibacterial agents in micro molar concentrations. Our previous research6 showed that they act upon the cell membrane leading to the lysis of the bacteria, so they are bactericidal in nature. Myxobacteria produce slime, which is composed of exopolysaccharides, glycoproteins and glycolipids.7 Colonization of bacteria on a cloth surface leads to infection and their adherence to it is directly proportional to the exopolysaccharide (slime) produced by them. The slimicidal activity exhibited by the chalcones can also prevent the colonization of bacteria on surfaces.
Researchers have used herbal extracts (including neem leaf extract) to coat cotton fabrics to develop efficient antimicrobial fabrics.8,9 Synthetic chemical entities including 5,5′-ethylenebis(5-methyl-3-(3-triethoxysilylpropyl)hydantoin) and 3-(2,3-dihydroxypropyl)-5,5-dimethylimidazolidine-2,4-dione are also used as antibacterial coatings.10,11 Commercial antibiotics including Miconazole nitrate is used as an antibacterial coating, using β-cyclodextrin as a grafting agent, on cotton surfaces.12 The cross-linking of polymers including poly(N-vinyl-2-pyrrolidone) to improve the activity of antibiotics on cotton fabrics have also been tested.13 Zinc oxide mixed with chalcone, when used as an adhesive coating on cotton fabric, shows effective antibacterial activity against S. aureus, P. aeruginosa and E. coli.14
Gum Arabic (acacia) is a complex and a variable mixture of arabinogalactan oligosaccharides, polysaccharides and glycoproteins, and it acts as a binder. It possesses a wide variety of therapeutic applications, which also include antibacterial activity.15 Wound healing dressings, including zinc paste bandages, contain gum Arabic as a binder.16 Using combinations of antibiotics (more than a single antibiotic) is a useful strategy to create synergy, which may lead to a reduction in the use of antibiotics as well as combat the resistance offered by the organism.17
In the present study, an antibacterial coating is developed for cotton cloth using three chalcones (compounds 1–3 are given in Fig. 1) along with acacia as the binder. The attachment of S. aureus, P. aeruginosa, and E. coli on the treated cloth is tested.
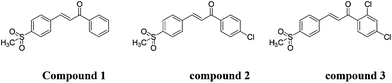 |
| Fig. 1 Structure of chalcones 1–3. | |
Materials and methods
All the chemicals were purchased from Sigma-Aldrich (St. Louis, USA) and SRL (Mumbai, India). The bacterial strains (Staphylococcus aureus NCIM5021, Escherichia coli NCIM2931 and Pseudomonas aeruginosa NCIM2901) were purchased from the National Chemical Laboratory, Pune, India.
Chalcone synthesis
The procedure for the synthesis of 4-methylthio chalcone is adopted from Lin et al.18 The conversion of 4-methylthio chalcone to 4-methylsulfonyl chalcone is performed based on the method suggested by Davis et al.19 The synthesised 4-methylsulfonyl chalcones are characterized by FT-IR (Perkin Elmer Spectrum one), NMR (JEOL GSX 400NB), and mass spectrometer (Finnigan MAT 8230 MS) and these results are reported in our previous communications.6,20
Coating procedure
A 100% cotton woven fabric of medium weight (the fabric particulars are: plain weave, 140 grams per square meter, 20 ends per cm; 16 picks per cm) was used for the experiments. The cotton fabric, cut to a size of 10 × 10 sq. cm was immersed for 5 min in a solution containing chalcone and acacia (50 and 125 mg, respectively) and then was passed through a padding mangle (Electronic and Engineering Company, Bombay, India), which was running at a speed of 15 m min−1 and a pressure of 25 kg cm−2 to remove excess solution. The material to liquor ratio was kept at 1
:
20. A 100% wet pick-up was maintained for all the treatments. After padding, the fabric was dried in a hot air oven at 40 °C.
Hydrophilicity of the cloths
The static immersion test reported in the ATCC Technical Manual 2001 was followed to assess the hydrophilicity of the treated and untreated cloth samples.9 The samples were weighed and immersed in a 250 ml beaker containing distilled water to a depth of 10 cm. The cloth was removed after 20 min, tapped ten times to remove excess water and then weighed once again. The absorption percentage was estimated from the amount of water taken up by the cloth.9
Absorption percentage = (mass of water absorbed/original mass) × 100 |
Surface analysis
The presence of chalcone on the cloth was determined with a FEI Quanta 200 environmental scanning electron microscope (ESEM) with an energy dispersive X-ray spectroscope (EDAX). The various functional groups present in chalcone, acacia, and the cotton coated and uncoated cloths were identified with a Perkin Elmer Spectrum 1 FT-IR. The spectra were collected in the range of 450–4000 cm−1 at a sensitivity of 4 cm−1. The minimum inhibitory concentration (MIC) of the three chalcones against S. aureus, P. aeruginosa and E. coli were determined according to the reported literature.6
Estimation of bacterial adhesion
Adhesion of S. aureus, P. aeruginosa and E. coli on the compound coated and uncoated cloths was estimated in triplicate, as reported by Zhao et al. with slight modifications.21 A single colony of the bacteria from a plate was inoculated into 20 ml of tryptic soy broth (TSB) and cultured at 37 °C for 16 h at 180 rpm in a shaker. The culture was centrifuged for 10 min at 8000 rpm at a temperature of 4 °C. The pellets were suspended in 0.9% saline and adjusted to an optical density of 0.1 at 660 nm (which was approximately 1 × 107 cells per ml). The chalcone coated and uncoated cloths were immersed in 25 ml of the above made bacterial suspension and incubated in static conditions for 2 h at 37 °C. Then, the samples were transferred into 25 ml of fresh tryptic soy broth and incubated for 24 h at 37 °C at an agitation speed of 120 rpm. After 24 h, the samples were removed using sterile forceps and washed twice in sterile water to remove non-adhered bacteria. Those that were strongly adhered were removed from the cloth surface by water-bath ultrasonication (total of 10 min with 1 min intervals) and the viable cells were counted (colony forming units; CFU ml−1) in tryptic soy agar (TSA). The percentage reduction in the bacterial adhesion is estimated as given below:
100[CFU ml−1 on uncoated − CFU ml−1 on coated]/[CFU ml−1 on uncoated cloth] |
Morphology of the biofilm
The surfaces of the coated and uncoated cloths were observed under a scanning electron microscope (SEM) before and after the adhesion experiments. After adhesion, the cloth was washed with distilled water and then fixed for an hour using 3% glutaraldehyde solution (in 0.1% phosphate buffer at pH 7.2). Later it was washed twice with phosphate buffer and once with distilled water, and dehydrated with alcohol of various concentrations (20, 50, 70 and 90%) for 10 min. They were dried overnight in a dessicator, coated with platinum at 30 mA for 1 min and were viewed under a scanning electron microscope (HITACHI-S3400N) at a maximum magnification of ×2000.
Hydrophobicity of the organisms
The hydrophobic nature of the three bacteria are estimated using a BATH assay using hexadecane.22 The propensity of the organism to partition to the organic phase from the aqueous media is an indication of its hydrophobicity.
Fluorescence microscopic analysis
The live and dead cells present in the biofilm formed on the cloth surface, after 24 h of incubation, were isolated and observed using a mixture of two nucleic acid staining dyes, namely SYTO9 and propidium iodide (PI) (Baclight®, Invitrogen, USA).
Statistical analysis
The data reported here are expressed as the mean ± standard error (SE) of three samples in each experiment. Two way analysis of variance (ANOVA) and two sample t-tests were performed using MiniTab Version 14.0 (MiniTab, Inc., State College, PA, USA). A p-value of <0.05 is considered statistically significant.
Results and discussion
Characterization of coated cloths
Fig. S1 (a–c), ESI † show the comparative FT-IR spectra of the compound 1–3 coated and uncoated cloth samples. The pure cotton cloth shows characteristic peaks arising from C–O–C asymmetric stretching at 1155 cm−1 and C–H stretching at 2908 cm−1. The peak observed at 3283 cm−1, which corresponds to –OH stretching, is also observed by other researchers.8 All three of the coated cotton FTIR spectra show a characteristic peak in the range of 1655–1625 cm−1, which is due to the presence of chalcone (α,β-unsaturated carbonyl system). This peak is also observed in the FTIR spectra of the individual chalcones. Chalcones when coated alone, show a strong characteristic peak at 1655–1625. The concentration of chalcone on the cloth decreases resulting in less intense IR peaks when it is mixed with acacia and coated. The less intense IR peaks are due to the presence of acacia, which masks the chalcone. Out of the 50 mg of chalcone coated onto the 10 × 10 cm cloth, 2 mg of chalcone leached out during the first wash, and there was no leaching out of chalcone after further repeated washing, which shows that 48 mg of chalcone was coated onto every 10 × 10 cm cloth.
Fig. 2A shows the SEM micrograph of the uncoated cloth, and Fig. 2B–D show the compound 1, 2 and 3 coated cloths, respectively. The uncoated cotton fabric appears smoother (Fig. 2A) when compared to the coated ones. Chalcone, which is bound to the surface, could be seen on the fibers in Fig. 2B–D. The weight percentage of various elements on the surface of these materials as determined by EDAX, as shown in ESI.† The uncoated cloth shows only C and O (42.6 and 30.6%, respectively) (ESI, Table S1†). Au is also observed since it is used as a coating material for improving the conductivity of the cloth (ESI, Fig. S2†). The EDAX of the coated cloth samples show contributions of elemental sulphur, since the chalcones contain a thio group. Elemental chlorine on the compound 2 and 3 coated cloths are observed, since these two chalcones are chlorine derivatives of 4-methylthio chalcones. No significant differences are observed in the percentage of Cl atoms on the cloths treated with compounds 2 and 3, probably because of the concentration variation arising due to the inhomogeneity of the coating process and diffusion of the compound into the cotton. Also EDAX focuses on a single point of the cotton fabric and there could be a possibility of variation in concentration from one location to another. The chalcones are bound to the cotton with acacia, so the elemental composition (as determined by EDAX) will correspond to chalcone as well as acacia, and many not truly indicate the amount of chlorine present in a molecule of compound 2 or 3.
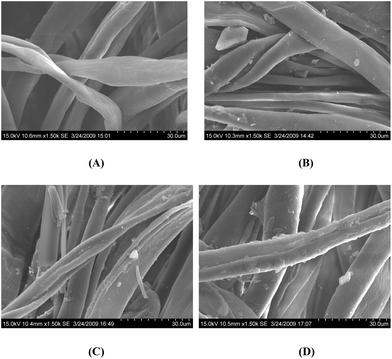 |
| Fig. 2 SEM photomicrographs of (A) the uncoated cloth and (B–D) the compound 1–3 coated cloths, respectively. | |
The SEM figures shows that the compound is not absorbed fully by the fiber, but has formed a layer of coating around each fiber (chalcones are hydrophobic and the cotton cloth is hydrophilic). Although the SEM images shows uneven coating at the μm level, during chalcone estimation, it was found that each 1 × 1 cm cloth piece contained approximately 4.78 mg of chalcone. Also, there was no leaching of the compound after five repeated washes, which shows the stability of the compound coated cloths. SEM–EDAX of the compound 1–3 coated cloths are given in Table S2 and Fig. S3,†, and show very minute differences in the weight and atom% of the compounds before and after immersing in water.
The −log(MIC) values of the three chalcones against these three organisms are plotted in Fig. 3. As expected, the dichloro chalcone exhibits the highest activity against all three organisms, followed by the monochloro chalcone against E. coli and P. aeruginosa. The BATH assay indicates that S. aureus is the most hydrophilic amongst these organisms (70.4% hydrophobicity). E. coli is marginally more hydrophobic (95.4%) than P. aeruginosa (94.1%).
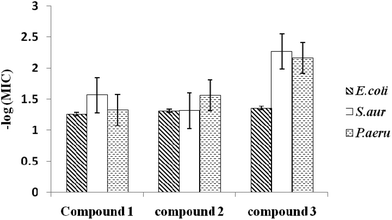 |
| Fig. 3 MIC of the three chalcones against E. coli, S. aureus and P. aeruginosa. | |
Biological evaluation
The numbers of colonies (CFU ml−1) of the three bacteria on the uncoated and the coated cloth samples are shown in Fig. 4.
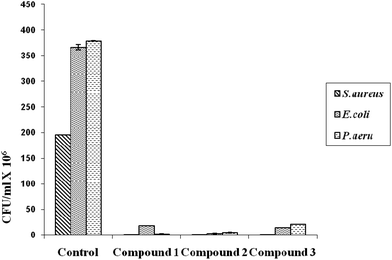 |
| Fig. 4 CFU of E. coli, S. aureus and P. aeruginosa on the three coated cloths. | |
The coated cotton cloths show a remarkable reduction in the adhesion of all three bacteria when compared to the uncoated cotton (by 94–99%). Other researchers also found similar results using neem extract (active component as azadirachtin) with 18 hours of radio frequency oxygen plasma treatment on cotton fabrics.8,9 Researchers who used citric acid as a cross-linking agent observed that the cloth turned yellow, which is not desirable. Thus, the natural product acacia reported here has advantages as a binder against other reported ones. Also it increases the tensile strength of the fibers.
Fig. 5 shows the SEM photomicrographs of the adhered bacteria on the uncoated and coated cloth samples. The reduction in bacterial adhesion is seen in all three of the coated cloths (B–D) when compared to the uncoated cloth (A). The mechanism of action of chalcone is already reported in several of our earlier research communications and it possibly acts by damaging the bacterial cell membrane.6
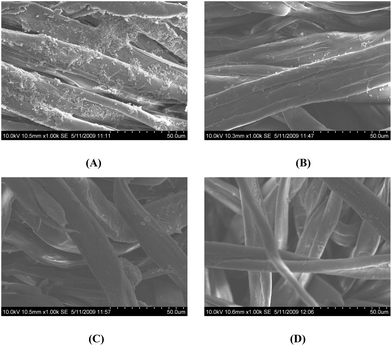 |
| Fig. 5 Comparative SEM photomicrographs of the adhesion of S. aureus to (A) uncoated cloth and (B–D) compound 1–3 coated cloths, respectively. | |
Surface hydrophilicity
The hydrophilicity of the coated and uncoated cloths was measured using static immersion tests and the results are shown in Fig. 6. The uncoated cloth has the highest water absorption capacity (170%) when compared to the three coated cloths (125–140%), which means that the former is the most hydrophilic when compared to the latter ones. Chalcones are known to be hydrophobic23 and hence they decrease the water absorption capacity. The third cloth is relatively the most hydrophobic (lowest water absorption capacity) since chalcone 3 is the most hydrophobic in this set.
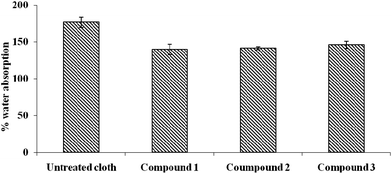 |
| Fig. 6 Absorption of water by the coated cloths measured using static immersion tests. | |
Mechanism of adhesion
Bacterial adhesion on a surface is a complex process to explain and it has several parameters as determinants. Its adhesion to the surface is determined by three important parameters, namely the properties of the bacterial cell surface, the liquid environment and the properties of the material surface (the presence of functional groups on the polymer, surface roughness, charge, attributes present and flexibility). The interaction between the coating and the material surface also plays an important role in determining the performance of the latter. Bacterial adhesion is determined by adhesive structures present on the surface of the bacteria including pilli, fimbriae etc. E. coli and P. aeruginosa possess fimbriae. Studies have shown that there is a correlation between cell surface hydrophobicity and fimbriae.24–26 Fimbriae are a non-flagellar component of the bacteria and they play a role in its hydrophobicity. They facilitate the attachment of the bacteria by overcoming the electrostatic repulsion barrier between a cell and the substratum.27 Fimbriae are found to contain a number of hydrophobic amino acid residues.28 Due to hydrophobic components on the bacterial cell surface, the bacterial adhesion onto hydrophobic surfaces is facilitated while it is reduced on hydrophilic surfaces. Fresh water and marine water bacteria show more adhesion towards hydrophobic (lesser charged) surfaces when compared to hydrophilic (more charged) surfaces. Surface medications by coating with proteins including bovine serum albumin (BSA), bovine glycoprotein and fatty acid free BSA are capable of reducing the surface hydrophobicity leading to a reduction in bacterial adherence.29 However, a few researchers have found that in in vitro environments, hydrophobic surfaces showed less bacterial adhesion when compared to the hydrophilic surfaces in the human body.30,31 Hence in our case, even though the moisture absorbing capacity (related to hydrophilicity) of the cloths has decreased after chalcone coating, they have shown remarkable reduction in bacterial adhesion. This is due to the fact that the compounds possess bactericidal and slimicidal activity, thereby preventing the colonization of bacteria.
The number of live colonies (CFU ml−1) on the surface of the three cloths are positively correlated (correlation coefficient = 0.58) with the hydrophobicity of the organism (based on BATH assays), indicating that hydrophobic organisms attach more easily than hydrophilic ones (reasons are given above). Also, the number of colonies on these three cloths are negatively correlated (correlation coefficient = −0.52) with the water absorbing capacity of the cloth (which is an indication of the hydrophilicity of the cloth), indicating that hydrophilic surfaces prevent bacterial attachment better than hydrophobic ones. Using these two variables, a simple linear regression model can be described, as shown below:
[CFU ml−1] = 43.97 + 0.34[organism hydrophobicity] − 0.50[cloth hydrophilicity] |
R2 = 0.61, F = 5.1, p < 0.05 |
So, the surface properties of the organism and the surface properties of the cloth affect the CFU on the material. Interestingly, the correlation between the MIC and CFU is poor (−0.1), indicating that when a compound is coated on the surface, its antibacterial effectiveness may change depending on the surface properties, the interaction between the compound and the material, and the coating method adopted etc. Chalcones are hydrophobic and hence they decrease the hydrophilicity of the cloth, so one should strike a balance between its antibacterial activity and the extent to which it decreases the hydrophilicity of the coated surface.
The antibacterial action of chalcone is through cell membrane damage, as evidenced by Baclight® assays, where dead cells are shown red in colour and live cells are shown green in colour. Propidium iodide enters damaged cells and binds to the nucleus emitting a red colour. Our previous studies have indicated that chalcones exhibit antibacterial activity by damaging the cell walls.22 Once the compound is immobilized on a surface, in addition to its antibacterial efficiency, other physico-chemical properties of the surface would also affect the overall behavior of this material. This may be similar to the way an enzyme behaves when it is in solution or in the immobilized state. It is well known that the physico-chemical properties of the solid support would affect the behavior of the enzyme. Chalcones are hydrophobic, so they alter the hydrophobicity of the cotton cloth. Hydrophobic organisms favorably attach to hydrophobic surfaces. The antibacterial nature of the chalcone starts acting on the organisms, which are attached to the surface. Fluorescence microscopic images of microbes isolated from biofilms formed on the coated and uncoated cloths are shown in Fig. 7.
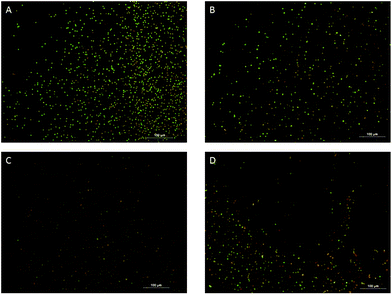 |
| Fig. 7 Fluorescence microscopic images of microbes isolated from biofilms formed on the uncoated cloth (A) and the compound 1–3 coated cloths (B–D) are shown below. | |
Conclusions
There was not any significant correlation between the MICs and the antimicrobial activity of the coated cloths. This is because the antibacterial activity is due to several factors including the amount of acacia and chalcones present on the surface, the properties of the cloth and the interaction between the two. Hence, differences in the number of chlorine atoms alone did not influence the overall performance of the cloth. Although the hydrophobicity of the surface plays a major role in bacterial adhesion, in this paper we have demonstrated that the adhesion is not only a function of this property, but it is also related to other properties, which are also observed by other researchers too. The number of live colonies (CFU ml−1) on the surface of the three cloths is positively correlated with the hydrophobicity of the organism and negatively correlated with the water absorbing capacity of the cloth, indicating that hydrophobic organisms attach more easily on surfaces than hydrophilic ones and hydrophilic surfaces prevent bacterial attachment better than hydrophobic ones. Since acacia and chalcones are antibacterial agents, one can achieve a synergy in performance by combining them both. Such a strategy can be useful in the treatment of drug resistant bacteria including MRSA. Moreover these chalcones have a broad spectrum of activity (they are active against Gram +ve and Gram −ve bacteria). Thus the coated cloth has potential applications in hospital environments and can be used as bed covers, curtains, linens and dressings. The long term stability and washability of these coated cloths needs to be assessed in field conditions.
Acknowledgements
The authors thank SAIF and the Department of Chemistry, IIT Madras for providing analytical support.
References
- C. P. Oplustil, O. H. M. Leite, M. S. Oliveira, S. I. Sinto, D. E. Uip, M. Boulos and C. F. Mendes, Braz. J. Infect. Dis., 2001, 5, 252–259 CrossRef CAS PubMed.
- J. S. Cho and C. Cho, Text. Res. J., 1997, 67, 875–880 CAS.
- M. Lipsitch, C. T. Bergstrom and B. R. Levin, Proc. Natl. Acad. Sci. U. S. A., 2000, 97, 1938–1943 CrossRef CAS.
- H. Boucher, L. G. Miller and R. R. Razonable, Clin. Infect. Dis., 2010, 51, S183–S197 CrossRef CAS PubMed.
- M. R. Law and O. N. Gill, Epidemiol. Infect., 1988, 101, 623–629 CrossRef CAS.
- P. M. Sivakumar, S. Priya and M. Doble, Chem. Biol. Drug Des., 2009, 73, 403–415 CAS.
- P. M. Sivakumar, G. Sheshayan and M. Doble, Chem. Biol. Drug Des., 2008, 72, 303–313 CAS.
- K. Vaideki, S. Jayakumar, R. Rajendran and G. Thilagavathi, Appl. Surf. Sci., 2008, 254, 2472–2478 CrossRef CAS PubMed.
- K. Vaideki, S. Jayakumar, G. Thilagavathi and R. Rajendran, Appl. Surf. Sci., 2007, 253, 7323–7329 CrossRef CAS PubMed.
- K. Barnes, J. Liang, R. Wu, S. D. Worley, J. Lee, R. M. Broughton and T. S. Huang, Biomaterials, 2006, 27, 4825–4830 CrossRef CAS PubMed.
- X. Ren, H. B. Kocer, S. D. Worley, R. M. Broughton and T. S. Huang, Carbohydr. Polym., 2009, 75, 683–687 CrossRef CAS PubMed.
- J. Wang and Z. Cai, Carbohydr. Polym., 2008, 72, 695–700 CrossRef CAS PubMed.
- H. M. Fahmy, M. H. Abo-Shosha and N. A. Ibrahim, Carbohydr. Polym., 2009, 77, 845–850 CrossRef CAS PubMed.
- P. M. Sivakumar, S. Balaji, V. Prabhawathi, R. Neelakandan, P. T. Manoharan and M. Doble, Carbohydr. Polym., 2010, 79, 717–723 CrossRef CAS PubMed.
- M. L. Saini, R. Saini, S. Roy and A. Kumar, J. Med. Plants Res., 2008, 2, 378–386 Search PubMed.
-
P. Hanns and H. Volker, U. S. Patent, 4486488, 1984 Search PubMed.
- R. G. Petersdorf, West J. Med., 1975, 123, 135–136 CAS.
- J. Lin, D. E. Rivett and J. F. K. Wilshire, Aust. J. Chem., 1977, 30, 629–637 CrossRef CAS.
- F. A. Davis and P. Zhou, Tetrahedron Lett., 1994, 35, 7525–7528 CrossRef CAS.
- P. M. Sivakumar, T. M. Kumar and M. Doble, Chem. Biol. Drug Des., 2009, 74, 68–79 CAS.
- F. Zhao, H. Nozawa, A. Daikonnya, K. Kondo and S. Kitanaka, Biol. Pharm. Bull., 2003, 26, 61–65 CAS.
- P. M. Sivakumar, V. Prabhawathi and M. Doble, Colloids Surf., B, 2010, 81, 439–446 CrossRef CAS PubMed.
- F. Bois, A. Boumendjel, A. M. Mariotte, G. Conseil and A. Di Petro, Bioorg. Med. Chem., 1999, 7, 2691–2695 CrossRef CAS.
- S. Faris, M. Lindahl and T. Wadstrom, Curr. Microbiol., 1982, 7, 357–362 CrossRef.
- S. J. Vesper, Appl. Environ. Microbiol., 1987, 53, 1397–1405 CAS.
- D. Pavithra and M. Doble, Biomed. Mater., 2008, 3, 034003 CrossRef CAS PubMed.
-
W. A. Corpe, in Adsorption of microorganisms to surfaces, ed. G. Bitton and K. C. Marshall, John Wiley & Sons, New York, 1980, vol. 105 Search PubMed.
- M. Rosenberg and S. Kjelleberg, Adv. Microb. Ecol., 1986, 9, 353–393 CAS.
- M. Fletcher and K. C. Marshall, Appl. Environ. Microbiol., 1982, 44, 184–192 CAS.
- M. Quirynen, M. Marechal, H. J. Busscher, A. H. Weerkamp, J. Arends, P. L. Darius and D. Van Steenberghe, J. Dent. Res., 1989, 68, 796–799 CrossRef CAS PubMed.
- E. P. Everaert, H. F. Mahieu, R. P. Wong Chung, G. J. Verkerke, H. C. Van der Mei and H. J. Busscher, Eur. Arch. Otorhinolaryngol., 1997, 254, 261–263 CrossRef CAS.
Footnotes |
† Electronic supplementary information (ESI) available. See DOI: 10.1039/c3bm60312a |
‡ Both the authors contributed equally. |
|
This journal is © The Royal Society of Chemistry 2014 |