DOI:
10.1039/C3AY41827H
(Paper)
Anal. Methods, 2014,
6, 229-234
Fragment-based hapten design and screening of a highly sensitive and specific monoclonal antibody for ractopamine
Received
18th October 2013
, Accepted 28th October 2013
First published on 29th October 2013
Abstract
Ractopamine (RCT) is a beta-adrenergic agonist and its use is forbidden in many countries. With the aim of more sensitive and specific detection of RCT, a RCT structure fragment-based hapten was synthesized by introducing a carboxyl group to octopamine through a reductive amination reaction with ethyl levulinate. Six mice were immunized with a hapten–KLH conjugate, and the best monoclonal antibody (Mab) with an IC50 value of 0.34 ng mL−1 in PBST buffer and 0.41 ng mL−1 in a 10-times diluted swine urine sample was obtained, which had no cross reactivity with clenbuterol and salbutamol. These results demonstrated that a fragment of RCT as a hapten can mimic the antigenicity and specificity of RCT very well, and the Mab has potential applications in the specific monitoring of the illegal use of RCT in real samples.
Introduction
Beta-adrenergic agonists are a group of synthetic compounds with muscle growth promoting effects in livestock.1–3 Ractopamine (RCT) is a beta-adrenergic agonist and is licensed for use in twenty-seven countries, including the United States. The maximum residue limits (MRLs) established by the FDA are 50 ng g−1 for pork and 30 ng g−1 for beef in the United States.4 However, the use of RCT is forbidden in China and European Union member countries. Earlier this year, after China and Russia made the decision that all American meat exports should be certified RCT-free, the demand for highly sensitive and highly selective analytical methods for the detection of RCT residues increased.5,6
Immunoassays for small molecule detection have been widely used as primary screening methods due to their specificity, simplicity, speed, cost-effectiveness and high-throughput. ELISA and lateral flow assay (LFA) are the most commonly used immunoassays and related commercial products are widely applied for food safety. In 1994, Haasnoot first reported an ELISA method for RCT detection, however, the antibody was raised against fenoterol–BSA, and was not specific to RCT, the detection limit for RCT was 2.6 ng mL−1.7 During the next ten years, only Elliott and Shelver developed immunoassays using a specific antibody to RCT,1,8–12 and the best IC50 value for RCT was 2.69 ng mL−1.10 In the last decade, only a few researchers developed RCT antibodies,13–17 and the polyclonal antibody developed by Li showed the best performance, with an IC50 value of 0.44 ng mL−1.16
Besides the classic immunoassays, ELISA and LFA, more sensitive methods based on antibodies have also been used to detect RCT, such as time-resolved fluoroimmunoassay,18 electrochemiluminescent immunosensors,19 and immunomagnetic-proximity ligation assay,20,21 with detection limits of 0.1 ng mL−1, 8.5 pg mL−1 and 0.01 ng mL−1, respectively. However, in all these immunoassays, the antibody is the most critical factor and fundamentally determines the final sensitivity. Thus, the production of a highly sensitive antibody is the basis for developing a highly sensitive immunoassay, and a suitable hapten design is the first step to success. To date, the haptens used to obtain a specific RCT antibody have all been directly derived from RCT itself by converting the hydroxyl group to a carboxyl group. However, there are three hydroxyl groups in the RCT structure, and moreover, two of them are on both sides of the benzene rings which results in similar reactivity, therefore, it is very difficult to obtain a single chemical compound as the derived hapten and produce a highly sensitive antibody. In this study, we designed and synthesized a new hapten structure containing a fragment of RCT, and generated a highly sensitive RCT antibody. The findings of this study may allow a better understanding of the role of hapten design and synthesis in the development of a highly sensitive RCT antibody.
Experimental
Chemicals and reagents
Ractopamine hydrochloride, octopamine (OA), triethylamine (TEA), ethyl levulinate (EL), sodium triacetoxyborohydride (NaBH(OAc)3), keyhole limpet hemocyanin (KLH), ovalbumin (OVA), Freund's complete and incomplete adjuvants, N-(3-dimethylaminopropyl)-N′-ethylcarbodiimide hydrochloride (EDC), N-hydroxysuccinimide (NHS), Tween 20, TMB (3,3′,5,5′-tetramethylbenzidine), and polyethylene glycol solution (PEG, Hybri-Max, 50% (w/v)) were purchased from Sigma Chemical Co. (Shanghai, China). HAT supplement, HT supplement, 1640 cell culture and fetal calf serum were purchased from Life Technologies Corporation (Shanghai, China). The Sp2/0-Ag14 murine myeloma cell line was purchased from the Cell Bank of Chinese Academy of Sciences (Shanghai, China). HRP-goat anti-mouse IgG conjugate was purchased from Jackson ImmunoResearch Laboratories (PA, USA). Ultrapure water was produced in our laboratory using a Milli-Q Integral system (Millipore, MA, USA). All other solvents and chemicals were analytical reagents and used without further purification.
Preparation of OA–levulinic acid
Synthesis of the RCT hapten was initiated by the reductive amination reaction of octopamine as shown in Fig. 1. First, OA (500 mg, 2.64 mmol) was dissolved in 20 mL of methanol using a magnetic stirrer at room temperature. While stirring, 0.8 mL of triethylamine, 0.4 mL of ethyl levulinate and 557 mg of sodium triacetoxyborohydride were added. After 12 h, 6 mL of 1 mol L−1 NaOH was added, and stirring was continued for 4 h. The reaction solution was evaporated using a rotary evaporator, and the solid residue was dissolved in 10 mL water. The pH of the solution was adjusted to approximately 3.0 using 6 mol L−1 HCl, and a cloudy white precipitate appeared. The solid (OA–levulinic acid) was filtered and washed using diethyl ether, then dried in vacuo at 37 °C for 2 h. Mass spectral analysis of unhydrolyzed OA–EL ester and OA–levulinic acid (hapten) indicated masses of m/z 281 and 253 compatible with the structure proposed in Fig. 1. The OA–levulinic acid obtained in this step was used as the hapten for RCT.
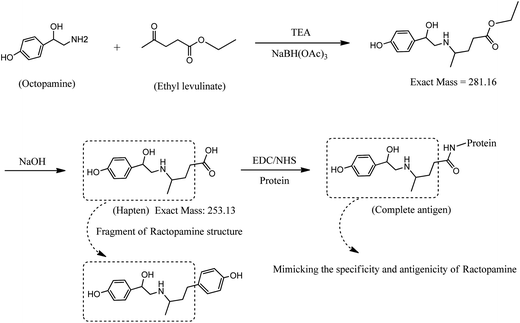 |
| Fig. 1 Synthesis of a fragment-based hapten for ractopamine and coupling of hapten to protein. | |
Conjugation of OA–levulinic acid with carrier proteins
The conjugation of this new hapten for RCT was similar to the procedure reported for the synthesis of melamine by Sun et al.22 Briefly, OA–levulinic acid (16 mg, 0.063 mmol) was dissolved in 1 mL of DMF, and then 1 mL of 0.1 M pH 5.5 2-[N-morpholino]ethane sulfonic acid (MES) was added. While the solution was stirred, 60 mg of EDC and 30 mg of NHS were added and stirring was continued for 4 h. The solution was then divided into two aliquots. One portion was added to 25 mg of ovalbumin (OVA) dissolved in 2 mL of 0.1 M pH 9.6 carbonate–bicarbonate buffer and the other portion was added to 25 mg of keyhole limpet hemocyanin (KLH) dissolved in 2 mL of 0.1 M pH 9.6 carbonate–bicarbonate buffer. Each solution was reacted at room temperature for 12 h and then dialyzed with PBS (0.01 M pH 7.4). The protein concentrations in the conjugates (OA–LA–OVA and OA–LA–KLH) were measured using the Bradford method. After the UV-Vis spectra of conjugates were characterized, the conjugates were aliquoted and stored at −20 °C until use.
Immunization and antibody generation
Animal welfare and experimental procedures were carried out in strict accordance with the recommendations in the Guide for the Care and Use of Laboratory Animals (23a) of the National Institutes of Health. The protocols were approved by the Institutional Animal Care and Use Committee (IACUC) of Jiangnan University. All efforts were made to minimize animal suffering and to reduce the number of animals used. Six female BALB/c mice, 6 weeks old, were initially immunized by intradermal injection at 4 sites on the back with 100 μg of OA–LA–KLH mixed with complete Freund's adjuvant. Four booster immunizations were administered at 3-week intervals, consisting of 50 μg antigen emulsified with incomplete Freund's adjuvant. Seven days after the third and the last booster immunization, tail blood was collected and tested for antibody titers and affinity to RCT by indirect competitive ELISA using OA–LA–OVA as the coating antigen. After the 4th booster, the mice which showed the best competition to RCT were used in the fusion experiments. Three days prior to splenocyte harvest, the mice received an intraperitoneal injection of 20 μg of antigen. SP2/0 myeloma cells were fused with the splenocytes from the best mice at a ratio of 1
:
5 in the presence of 50% PEG 1500 and were then cultured in RPMI 1640 with 20% FCS (v/v) and HAT in 96-well culture plates (200 μL per well) for 1 week. The hybridoma cells were selected by indirect competitive ELISA using OA–LA–OVA (0.3 μg mL−1) as the coating antigen. The holes with a highly positive and good inhibition effect were subcloned three times by limiting dilution assay. One week after each subclone, hybridoma cells were selected by indirect competitive ELISA. After the last selection, three hybridomas with the best inhibition effect were chosen for ascites generation in accordance with the method of Jackson et al.23 Ascites were collected 1 week after injection of the cloned cells, purified using the caprylic acid–ammonium method24 and frozen at −20 °C until use.
Antibody specificity determination
The optimum amount of coating antigen OA–LA–OVA (0.05–1 μg mL−1) and primary antibody (0.05–1 μg mL−1) for the indirect competition ELISA was determined using the checkerboard method. After optimization, the indirect competitive ELISA procedure was used to determine the 50% inhibition concentration (IC50) for RCT and structurally related compounds. The indirect competitive ELISA procedure followed the method of Hoffmann et al.25 with some modifications. Briefly, 96-well flat-bottom ELISA plates were coated with 100 μL per well antigen in 50 mM pH 9.6 carbonate–bicarbonate buffer and incubated at 4 °C for 12 h, after which the plates were washed 3 times with PBST (10 mM pH 7.4 PBS containing 0.05% Tween 20) and blocked by incubation with 2% gelatin in 50 mM pH 9.6 carbonate–bicarbonate buffer for 2 h at 37 °C. After removal of the blocking buffer, the plates were washed 3 times. Fifty microliters of competitors at different concentrations and 50 μL primary antibody were added to each well sequentially and incubated for 30 min at 37 °C. After the plates were washed 3 times, 100 μL of HRP-goat anti-mouse IgG conjugate diluted 1
:
3000 was added to each well and incubated for 30 min at 37 °C. After the final wash, the plates were developed with 100 μL per well of TMB substrate solution (0.1 mL of 10 mg mL−1 TMB–glycol mixed with 10 mL of citrate–phosphate buffer and 2 μL of 30% hydrogen peroxide) for 15 min at 37 °C. The color development was stopped by adding 50 μL per well 2 M sulfuric acid. The optical density (OD) of the plates was read at 450 nm and the data were analyzed by a four-parameter logistic equation.
Matrix effects and standard curve generation
Determination of the matrix effects followed the method of Shelver et al.10 with some modifications. Swine urine samples were collected from an organic swine farm (Shandong, China) where the swine had not received beta-adrenergic agonist treatment. The urine samples were spiked with RCT and diluted to a series of concentrations using blank urine or blank urine diluted 1
:
2, 1
:
5, and 1
:
10 with PBST. The indirect competitive ELISA in the matrices was performed according to the procedure described above, and the standard curve was plotted by fitting to a four-parameter logistic equation. The measured B0 value and calculated IC50 were compared with the values generated from the PBST standard curve.
Results and discussion
Hapten design and antigen conjugation
The conventional hapten of RCT is obtained by converting the hydroxyl group to a carboxyl group, without consideration of the fact that there are three hydroxyl groups in the RCT molecule. Using this method, most of the hapten obtained is a mixture not a single chemical compound, which means there are at least three types of antigen epitopes. However, the monoclonal antibody can just recognize one epitope of a small molecule. Therefore, it is very difficult to screen highly sensitive antibodies using an impure hapten. In the present study, a carboxyl group was introduced to octopamine following a reductive amination reaction with ethyl levulinate. This reaction avoided the problem of the multiple group reaction. The obtained OA–levulinic acid was similar to a fragment of the RCT structure and retained most of the characteristic features of the RCT structure. The introduced carboxyl group allowed the hapten to conjugate with proteins by forming amide bonds using EDC and NHS. KLH was chosen as the immunizing carrier due to its strong immune response, and OVA was chosen as the coating antigen carrier as it is heterologous to KLH. The UV spectrum absorbance of the hapten, protein, and conjugates was recorded from 200 to 400 nm (Fig. 2). The maximum characteristic absorbance of the hapten–protein conjugates had a blue shift away from that of the carrier proteins. Due to the contribution of the hapten, the absorbance values of the conjugates between 250 and 280 nm were higher than those of the carriers. These UV spectra demonstrated that the conjugation of hapten and carrier protein was successful.
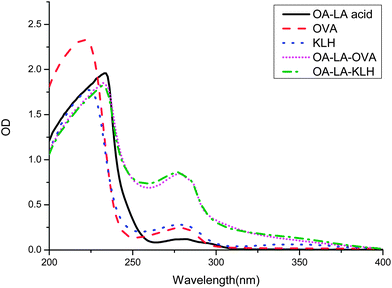 |
| Fig. 2 UV-Vis spectrum of OA–LA acid, OVA, KLH, and their conjugates. | |
Antibody generation
Each mouse was immunized with 100 μg of OA–LA–KLH conjugate to ensure high immunogenicity, then a booster immunization dose of 50 μg was administered to avoid immunologic tolerance. The antibody in mice sera and hybridoma supernatants evaluated the ability of recognizing RCT by indirect competitive ELISA. OA–LA–OVA conjugates chosen as the coating antigen avoided the undesired nonspecific reaction caused by protein homology. Four mice with high titers (>1
:
27
000) after the 3rd booster were used in next boost. After the final booster immunization, one of the mice showed the best reactivity against RCT (IC50 = 5 ng mL−1). As the best IC50 was obtained using 0.3 μg mL−1 OA–LA–OVA, the ELISA plates for hybridoma cell selection were also coated with this antigen concentration. After three rounds of limited dilution of the candidate hybridoma clones, three cell clones (D8, D11, F7) with highly positive and the best recognition of RCT were obtained. The inhibition ratios of these cell supernatants to 0.5 ng mL−1 RCT were all greater than 50%, which showed stable and sensitive antibody production against RCT. The sensitivities of the purified antibodies were compared (Table 1). The clones D8 and D11 had a similar IC50, but the B0 and B0/IC50 values of D11 were much greater than those of D8. Thus, D11 was chosen as the best antibody and used for further evaluation.
Table 1 Antibody specificities of three cell lines to ractopamine
Clones |
D8 |
D11 |
F7 |
Coating antigen conc. (μg mL−1) |
0.3 |
0.3 |
0.3 |
Antibody conc. (μg mL−1) |
0.1 |
0.05 |
0.1 |
B
0
|
1.174 |
1.571 |
1.728 |
IC50 (ng mL−1) |
0.30 |
0.34 |
0.52 |
B
0/IC50 |
3.88 |
4.91 |
3.34 |
Antibody specificity and matrix effects
The selectivity of the antibody produced by hybridoma D11 is shown in Table 2. The antibody was the most selective for OA–LA acid with a cross-reactivity of 118% relative to RCT. This may be due to the benzene ring on the other side of RCT having some steric hindrance which affected antibody recognition of the antibody produced using the OA–LA–protein conjugate. Fenoterol and octopamine had little cross-reactivity with the antibody. This weak binding effect may be caused by their similar structure to RCT. The most commonly used illegal beta-agonists, clenbuterol and salbutamol, did not cross-react with the D11 antibody. These results demonstrated that the D11 antibody recognized the same structure of OA–LA acid and RCT. This means that designing a fragment of RCT as a hapten can mimic the antigenicity and specificity of RCT very well, and the production of a highly sensitive antibody for RCT detection using this fragment-based hapten was successful. In China, beta-agonists were most illegally added to swine feed, therefore swine urine was chosen and spiked with RCT to simulate the real-world situation. Fig. 3 shows the standard curve for PBST and for swine urine with 0, 2, 5 and 10 times dilution. The B0 values for PBST and urine dilutions at 0, 1
:
2, 1
:
5, and 1
:
10 were 1.671, 0.755, 0.889, 1.235 and 1.512, respectively. The IC50 values were 0.34, 0.24, 0.24, 0.38 and 0.41 ng mL−1, respectively. The corresponding B0/IC50 values were 4.91, 3.14, 3.7, 3.25 and 3.68, respectively. These results showed that swine urine has strong matrix effects on antibody recognition. At 0, 1
:
2 and 1
:
5 urine dilutions, the IC50 values were fine, but their B0 values were too low, thus, these dilutions had little practical value. The B0 value was close to the value in PBST only after the urine was diluted 10 times. The IC50 value was poorer than that in PBST, nevertheless the ELISA IC50 values of RCT in urine samples were much better than those in other published reports, therefore this method using the D11 antibody can be used for the detection of RCT in urine samples.
Table 2 IC50 and percentage cross-reactivities towards ractopamine and other analogs
Analogs |
IC50 (ng mL−1) |
Cross-reactivity (%) |
Ractopamine |
0.34 |
— |
Clenbuterol |
— |
<0.1 |
Salbutamol |
— |
<0.1 |
Fenoterol |
18.7 |
1.8 |
Octopamine |
236 |
0.14 |
OA–LA acid |
0.29 |
118 |
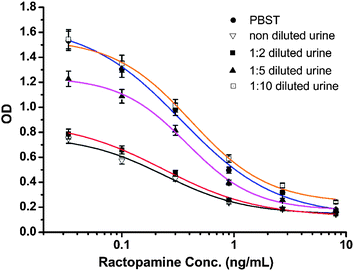 |
| Fig. 3 Standard curve for PBST and for swine urine with non-, 2, 5 and 10 dilution times. | |
Conclusions
We designed and synthesized a new hapten for highly sensitive ractopamine monoclonal antibody production. This hapten is only a fragment of the ractopamine structure, but can still mimic the antigenicity and specificity of ractopamine successfully. And the antibody screened from this hapten exhibited good sensitivity and specificity to ractopamine. The IC50 is 0.41 ng mL−1 in a 10-times diluted swine urine sample showing attractive potential applications in real samples.
Acknowledgements
This work is financially supported by the National Natural Science Foundation of China (21101079, 21175034, 21371081, 21301073), the Key Programs from MOST (2012BAC01B07, 2012AA06A303, 2012BAD29B04, 2012 BAK11B01, 2011BAK10B07, 2011BAK10B01, 2010AA06Z302, 2010DFB3047, 2013ZX08012-001, 2012BAK17B10, 2012BAK08B01, 2012YQ090194, 2012BAD29B05, 2013AA065501), and grants from Jiangsu Province, MOF and MOE (NCET-12-0879, BE2011626, BE2013613, BE2013611, 201310128, 201210127, 201210036, 201310135, 311002, JUSRP51308A, CXLX12-0738).
References
- C. Elliott, T. Thompson, C. J. Arts, J. Crooks, M. J. Baak, E. R. Verheij and R. Baxter, Screening and confirmatory determination of ractopamine residues in calves treated with growth promoting doses of the beta-agonist, Analyst, 1998, 123, 1103–1107 RSC.
- J. K. Apple, P. J. Rincker, F. K. McKeith, S. N. Carr, T. A. Armstrong and P. D. Matzat, Meta-Analysis of the Ractopamine Response in Finishing Swine, The Professional Animal Scientist, 2007, 23, 179–196 Search PubMed.
- C. S. Thompson, S. A. Haughey, I. M. Traynor, T. L. Fodey, C. T. Elliott, J.-P. Antignac, B. Le Bizec and S. R. H. Crooks, Effective monitoring for ractopamine residues in samples of animal origin by SPR biosensor and mass spectrometry, Anal. Chim. Acta, 2008, 608, 217–225 CrossRef CAS PubMed.
- L. M. Rocha, A. M. Bridi, A. Foury, P. Mormede, A. V. Weschenfelder, N. Devillers, W. Bertoloni and L. Faucitano, Effects of ractopamine administration and castration method on the response to preslaughter stress and carcass and meat quality in pigs of two Pietrain genotypes, J. Anim. Sci., 2013, 91, 3965–3977 CrossRef CAS PubMed.
- T. J. Burnett, J. M. Rodewald, S. L. Brunelle, M. Neeley, M. Wallace, P. Connolly and M. R. Coleman, Determination and Confirmation of Parent and Total Ractopamine in Bovine, Swine, and Turkey Tissues by Liquid Chromatography with Tandem Mass Spectrometry, J. AOAC Int., 2012, 95, 1235–1255 CrossRef CAS.
- J. Pleadin, A. Vulic, N. Persi, S. Terzic, M. Andrisic, I. Zarkovic, K. Sandor and E. Perak, Comparison of ractopamine residue depletion from internal tissues, Immunopharmacol. Immunotoxicol., 2013, 35, 88–92 CrossRef CAS PubMed.
- W. Haasnoot, P. Stouten, A. Lommen, G. Cazemier, D. Hooijerink and R. Schilt, Determination of fenoterol and ractopamine in urine by enzyme immunoassay, Analyst, 1994, 119, 2675–2680 RSC.
- C. T. Elliott, A. Baxter, W. Haasnoot, A. Lommen and W. J. McCaughey, Development of a dual label time-resolved fluoroimmunoassay for the detection of beta-agonists in cattle urine, Food Agric. Immunol., 1996, 8, 219–227 CrossRef CAS.
- W. L. Shelver and D. J. Smith, Development of an Immunoassay for the β-Adrenergic Agonist Ractopamine, J. Immunoassay, 2000, 21, 1–23 CrossRef CAS PubMed.
- W. L. Shelver, D. J. Smith and E. S. Berry, Production and Characterization of a Monoclonal Antibody Against the β-Adrenergic Agonist Ractopamine, J. Agric. Food Chem., 2000, 48, 4020–4026 CrossRef CAS PubMed.
- W. L. Shelver and D. J. Smith, Application of a Monoclonal Antibody based Enzyme-Linked Immunosorbent Assay for the Determination of Ractopamine in Incurred Samples from Food Animals, J. Agric. Food Chem., 2002, 50, 2742–2747 CrossRef PubMed.
- W. L. Shelver and D. J. Smith, Determination of Ractopamine in Cattle and Sheep Urine Samples Using an Optical Biosensor Analysis: Comparative Study with HPLC and ELISA, J. Agric. Food Chem., 2003, 51, 3715–3721 CrossRef CAS PubMed.
- J. P. Wang, S. X. Zhang and J. Z. Shen, Technical Note: A monoclonal antibody-based immunoassay for determination of ractopamine in swine feeds, J. Anim. Sci., 2006, 84, 1248–1251 CAS.
- M. Li, Z. Chen and Q. Zhu, Development and Identification of Monoclonal Antibodies Against Ractopamine, Hybridoma, 2007, 26, 148–154 CrossRef CAS PubMed.
- X. Li, G. Zhang, R. Deng, Y. Yang, Q. Liu, Z. Xiao, J. Yang, G. Xing, D. Zhao and S. Cai, Development of rapid immunoassays for the detection of ractopamine in swine urine, Food Addit. Contam., Part A, 2010, 27, 1096–1103 CrossRef CAS PubMed.
- C. Y. Li and J. Q. Jiang, Preparation of a polyclonal antibody based heterologous indirect competitive ELISA for detecting ractopamine residue, J. Food, Agric. Environ., 2012, 10, 1349–1352 CAS.
- J.-X. Dong, Z.-F. Li, H.-T. Lei, Y.-M. Sun, F. Ducancel, Z.-L. Xu, J.-C. Boulain, J.-Y. Yang, Y.-D. Shen and H. Wang, Development of a single-chain variable fragment-alkaline phosphatase fusion protein and a sensitive direct competitive chemiluminescent enzyme immunoassay for detection of ractopamine in pork, Anal. Chim. Acta, 2012, 736, 85–91 CrossRef CAS PubMed.
- J. Shen, Z. Zhang, Y. Yao, W. Shi, Y. Liu and S. Zhang, Time-resolved fluoroimmunoassay for ractopamine in swine tissue, Anal. Bioanal. Chem., 2007, 387, 1561–1564 CrossRef CAS PubMed.
- Z. Li, Y. Wang, W. Kong, C. Li, Z. Wang and Z. Fu, Highly sensitive near-simultaneous assay of multiple “lean meat agent” residues in swine urine using a disposable electrochemiluminescent immunosensors array, Biosens. Bioelectron., 2013, 39, 311–314 CrossRef CAS PubMed.
- S. Cheng, F. Shi, X. Jiang, L. Wang, W. Chen and C. Zhu, Sensitive Detection of Small Molecules by Competitive Immunomagnetic-Proximity Ligation Assay, Anal. Chem., 2012, 84, 2129–2132 CrossRef CAS PubMed.
- X. Jiang, Z. Zhu, Z. Sun, L. Wang, L. Zhou, H. Miao, Z. Zhang, F. Shi and C. Zhu, The development of an indirect competitive immunomagnetic-proximity ligation assay for small-molecule detection, Analyst, 2013, 138, 438–442 RSC.
- F. Sun, L. Liu, H. Kuang and C. Xu, Development of ELISA for melamine detection in milk powder, Food Agric. Immunol., 2011, 24, 79–86 CrossRef.
- L. R. Jackson, L. J. Trudel, J. G. Fox and N. S. Lipman, Monoclonal antibody production in murine ascites. I. Clinical and pathologic features, Lab. Anim. Sci., 1999, 49, 70–80 CAS.
- M. Temponi, T. Kageshita, F. Perosa, R. Ono, H. Okada and S. Ferrone, Purification of Murine IgG Monoclonal Antibodies by Precipitation with Caprylic Acid: Comparison with Other Methods of Purification, Hybridoma, 1989, 8, 85–95 CrossRef CAS.
- P. Hoffmann, M. Jimenez-Diaz, M. Loleit, W. Tröger, K.-H. Wiesmüller, J. Metzger, G. Jung, I. Kaiser, S. Stöcklin, S. Lenzner, J. H. Peters, R. Grimm, E. Schäfer and W. G. Bessler, Preparation of human and murine monoclonal antibodies: antigens combined with or conjugated to lipopeptides constitute potent immunogens for in vitro and in vivo immunizations, Hum. Antibodies, 1990, 1, 137–144 CAS.
|
This journal is © The Royal Society of Chemistry 2014 |
Click here to see how this site uses Cookies. View our privacy policy here.