DOI:
10.1039/C3AY41711E
(Communication)
Anal. Methods, 2014,
6, 67-72
One-step highly sensitive detection of melamine using gold nanoparticle-based dynamic light scattering
Received
1st October 2013
, Accepted 5th November 2013
First published on 5th November 2013
Abstract
In this paper, a dynamic light scattering (DLS)-based approach was developed for the real-time detection of melamine. Due to the strong interaction between melamine and AuNPs, the hydrodynamic diameter of AuNPs changed with increasing amounts of added melamine in the detection system, which can be directly observed by DLS. The sensitivity of this assay to detect melamine is about 0.05 ppm, and even in detection solutions with low pH values (2.5–3.5 or 4.0–5.0), this DLS-based assay can work well. All these results suggest that the DLS-based assay could be a good alternative method for one-step real-time detection of melamine, especially in complex systems (such as complex solutions with a low pH environment), without time-consuming procedures and costly instruments.
Introduction
Melamine is a nitrogen-abundant chemical compound (with a total nitrogen level of about 66% by mass).1 Therefore, it is illegally added to milk products to produce an incorrectly high reading in the measurement of protein content based on total nitrogen. Researchers have found that ingestion of melamine at levels above the safety limit (such as 1 ppm for milk) can be linked to the increased incidence of kidney stones,2 renal failure3 and other issues.4 Thus, due to the widespread illegal use of melamine, there is an increasing demand for sensitive and reliable methods that can detect it at trace levels. Analytical methods such as chromatography followed by mass spectroscopy,5,6 enzyme-linked immunosorbent assay ELISA,7 and spectroscopy8 have been introduced for detection of trace melamine in milk products. However, most of the above approaches require expensive and complicated instruments, as well as some time-consuming procedures, making on-site and real-time melamine detection difficult.
Recently, gold nanoparticles (AuNPs) have been extensively used as colorimetric probes for detecting melamine which is ascribed to the unique size-dependent and interparticle distance-dependent optical properties of AuNPs. The color of AuNP solution changed with addition of increased amounts of melamine. Ai et al. developed thiol-functionalized cyanuric acid derivative (MTT)-stabilized AuNPs for colorimetric detection of melamine via triple hydrogen-bonding recognition between melamine and AuNPs.9 Huang et al. developed a visual and light scattering spectrometric detection method for melamine through the formation of triple H-bonds between polythymine (polyTn) modified AuNPs and melamine.10
In addition to their absorption change, light scattering is another photophysical characteristic of AuNPs that is of great interest for development of analytical methods. Among them, dynamic light scattering (DLS) is a well-established noninvasive technique for measuring the size of particles ranging from 0.5 nm to 6 μm.11–14 DLS is a powerful tool and gives an absolute measurement through determining small changes in the size of particles in solution rather than monitoring the intensity change of scattered light.11–14 Recently, some researchers have shown that this technique coupled with noble metal nanoparticles can be used as an ultrasensitive assay for chemical and biological detection, in which nanoparticles are used as a light-scattering enhancer and DLS as a read-out system.11–14 Therefore, we developed a DLS based one-step assay for detecting melamine. This assay is easy to conduct and fast and the detection signal can directly reflect the average nanoparticle diameter. By switching from detecting the light intensity change to the particle size of AuNPs, this DLS based technique provides a fast and convenient platform for melamine analysis.
Materials and methods
Chemicals and apparatus
HAuCl4·4H2O was obtained from Sinopharm Chemical Reagent Co., Ltd., China. Phosphate buffer solutions (PBS, 0.2 M) of various pH values were prepared by mixing stock standard solutions of NaH2PO4 and Na2HPO4. Melamine (99% in purity) was purchased from Sigma-Aldrich (MO, USA). HCl (30 wt%) was purchased from Shanghai Chemical Factory and 0.1 M HCl solution was prepared as stock. Nanosep filters were purchased from Pall Nanosep (Ann Arbor, MI). All other reagents were analytical reagent grade. Double-distilled water was used throughout the experiments.
Transmission electron microscopy (TEM) images of the samples were taken with a JEOL model JEM2100 transmission electron microscope operating at 200 kV. A dynamic Light Scattering detection system NANO-ZS90 (England, Malvern) was used for DLS measurement. UV-vis absorption spectra were obtained on a nucleic Acid/protein analyzer (DU 730) using helium–neon laser light (632.8 nm) as the excitation source.
Preparation of AuNPs
20 nm gold nanoparticles were synthesized by a modified citrate reduction method according to a procedure reported previously.15 Briefly, 100 mL of 0.01% HAuCl4 solution was heated to boiling, and then 2 mL of 1% sodium citrate solution was added rapidly with vigorous stirring and the solution was refluxed for an additional 15 min. During this procedure, the solution color changed from pale yellow to claret-red. The solution was cooled to room temperature, followed by stirring for a further 10 min. Then it was filtered through a 0.8 μm Gelman membrane filter. The synthesized colloidal gold nanoparticles were stored at 4 °C before use.16,17
Detection of melamine at different concentrations
The gold nanoparticle solution was used as a stock liquid for detecting melamine. Melamine, at different concentrations, was added to the AuNP solution at room temperature. Then, a Malvern Zetasizer Nano ZS DLS system was used to perform all DLS measurements. The sample was held at 25 °C by a temperature controlled sample holder. All DLS data were collected and analyzed by Malvern Zetasizer 6.34 software. Particle hydrodynamic diameters were calculated from intensity based particle size distributions.
For the colorimetric detection, 0.5 mL of melamine solution with different concentration was added to 0.5 mL of AuNP solution, and then the mixture was allowed to react for 5 min at 40 °C. The color change was observed either by the naked eye or from the absorbance values of the solution.
Effect of different pH on the detection of melamine
The prerequisite for AuNPs as an analytical probe is their colloidal stability. As a major factor influencing the stability of AuNPs, the pH value of the solution needs to be considered. Hydrochloric acid was added to the PBS buffer to change the pH value of the test system from nearly neutral to acidity (pH value: 2.5–3.5, 4.0–5.0). Then, DLS measurement was done to analyze the size changes.
Preparation and detection of extracted milk sample
0.2 mL milk was transferred to a micro-centrifugal tube followed by addition of 0.4 mL 0.1 M HCl solution. The mixed solution in the tube was sonicated for about 5 min, and then centrifuged at 10
000 rpm for 10 min. Finally, 0.4 mL of the supernatant liquid was loaded into a Nanosep filter (Ann Arbor 10K) and centrifuged at 12
000 rpm for 30 min. The obtained filtered liquid was used for analysis.
100 mL of diluted HCl solution was added to 2 mL of AuNP colloidal suspension to afford detection solution with pH 3–4. 100 mL of different extracted milk solutions containing different concentrations of melamine were added to the above AuNP solutions at room temperature.18 Finally, DLS measurement was done to analyze the size changes.
Results and discussion
DLS detection of melamine
Our detection method is based on the binding between melamine and AuNPs (Fig. 1).19–23 We proposed that melamine can bind with AuNPs, which gives rise to the aggregation of AuNPs, thereby resulting in quantitative diameter changes. Then the changes of hydrodynamic diameters were readout by the DLS system.
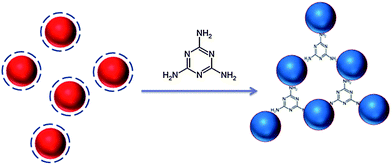 |
| Fig. 1 Schematic illustration of the proposed aggregation mechanism of AuNPs after addition of melamine. | |
The principle of this detection is based on the fact that AuNPs undergo aggregation in the presence of melamine, which is due to the formation of melamine and AuNP complexes. DLS signals increase tremendously owing to the increment in the particle size. Because DLS can distinguish a dimer from a monomer,11–13,24,25 and is a powerful method to determine small changes in the size of the particles,11–13,26 AuNP-based DLS assay should be able to show the response at a very low concentration of melamine. First, we performed the DLS assay in the AuNP solution, into which varying concentrations of melamine were added. As shown in Fig. 2A, the average size of AuNPs without melamine was around 24.36 nm. In a sample solution containing 0.1 ppm melamine, the average nanoparticle size increased to 30.67 nm (Fig. 2B), which was ascribed to the formation of nanoparticle oligomers through the interaction between melamine and AuNPs. With increased concentration of melamine, the average nanoparticle hydrodynamic diameter increased accordingly. Fig. 3 is a plot that shows different particle sizes over the melamine concentration range from 0 ppm to 0.5 ppm.
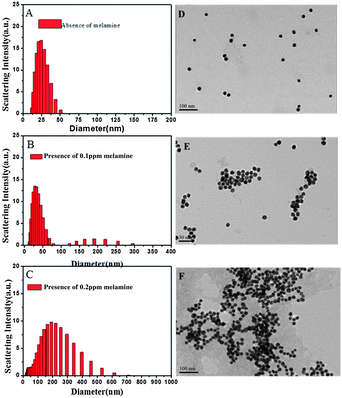 |
| Fig. 2 Size distribution of AuNPs measured by DLS in solution with pH 6.5–7.5. (A) In the absence of melamine, (B) in the presence of 0.1 ppm melamine, and (C) in the presence of 0.2 ppm melamine. TEM images showing the changes of AuNP aggregate sizes with addition of melamine at different concentrations to the solution with pH 6.5–7.5, (D) in the absence of melamine, (E) in the presence of 0.1 ppm melamine and (F) in the presence of 0.2 ppm melamine. | |
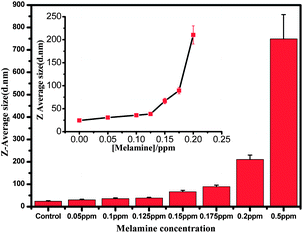 |
| Fig. 3 The average diameters of AuNPs determined from DLS measurement plotted against the melamine concentration. | |
As shown in Fig. 3, the detection limit is found to be around 0.05 ppm. The melamine stimulated aggregation of AuNPs were also identified by TEM images that displayed monodisperse nanoparticles in the absence of melamine and significant aggregation of nanoparticles in the presence of a solution containing 0.1 ppm and 0.2 ppm melamine, respectively (Fig. 2D–F).
Colorimetric detection of melamine
To further identify the accuracy and efficiency of this DLS-based method, colorimetric detection combined with UV-vis spectroscopic measurements were also performed in the AuNP solution to which different amounts of melamine had been added. The results are presented in Fig. 4A.
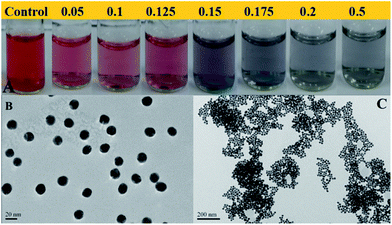 |
| Fig. 4 (A) Colorimetric changes of AuNPs in the presence of different concentrations of melamine (from 0.05 ppm to 0.5 ppm). (B) TEM image of AuNPs in the absence of melamine and (C) TEM image of AuNPs in the presence of 0.15 ppm melamine (pH of the detection system was 6.5–7.5). | |
On increasing the concentration of melamine added, the color of AuNPs gradually changed initially from claret-red to bluish violet, and finally a violet blue solution was observed within 15 min, which was consistent with the changes in UV-vis spectra (Fig. 5). The original absorbance centered at 524 nm was gradually reduced with the increasing concentration of melamine.
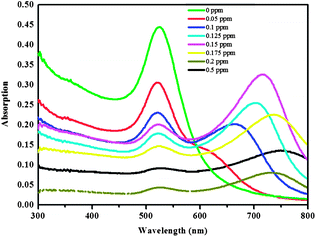 |
| Fig. 5 UV-vis absorbance spectra of AuNP colloidal solution with the melamine concentration ranging from 0 to 0.5 ppm at pH 6.5–7.5. | |
A new absorbance band centered at 675 nm was observed in the presence of 0.1 ppm melamine which then gradually shifted from 705 to 730 and finally to 760 nm with an increase in the concentration of melamine from 0.1 ppm to 0.5 ppm. All these results were consistent with results from DLS detection. In particular, as shown in Fig. 4A, when melamine at lower concentrations (0.05 and 0.1 ppm) was added to solution of the AuNPs, respectively, colorimetric change cannot be observed by naked eyes, while this small aggregation of AuNPs can be detected by DLS. By comparing the results of the simple colorimetric method with DLS data, we found that color shifts occurred at the melamine concentration between 0.125 ppm and 0.15 ppm. Accordingly, the hydrodynamic diameter of AuNPs increased to 66.73 nm at this concentration range. Previously reported results have indicated that the surface plasmon resonance based colorimetric change is dependent on aggregate size and the inter-particle distances.27–29 The particles aggregate as the inter-particle distance becoming greater, which will enlarge the average hydrodynamic diameters, and the color will also change.27–30 However, subtle changes in inter-particle distance are reflected in average hydrodynamic diameters, while no remarkable color changes can be observed. Thus, compared to colorimetric detection, the detection range of DLS based approach is much wider.
pH effect on the detection of melamine by DLS
Finally, in order to further study the pH effect on the detection of melamine, AuNPs were exposed to melamine solutions with different pH values. As melamine is a weak base with a pKa of 5.05,31 pH also affects the charge state of melamine. Therefore, the pH value of media solution plays a key role in the interaction between AuNPs and melamine. As shown in Fig. 6A and B, a new absorbance band centered at 684 nm was observed in the presence of 0.075 ppm melamine at the pH 4.0–5.0. And this new absorbance band was also observed in the presence of 0.05 ppm melamine at the pH of 2.5–3.5. However, by increasing the concentration of melamine (above 0.075 ppm) added into the solution, the absorbance band at 684 nm disappeared. These results revealed that even in the presence of low concentrations of melamine, the AuNPs easily aggregated at lower pH. This may be mainly attributed to the protonation of melamine in the presence of higher concentrations of H+ (proton) and thereby the electrostatic interaction between AuNPs and melamine could be enhanced, resulting in large-scale aggregation. Similar results can also be obtained from colorimetric detection. As shown in Fig. 6C and D, the color changed quickly after addition of 0.05 ppm melamine into AuNP solution either at pH of 4.0–5.0 or 2.5–3.5. Then with increased amounts of melamine added into AuNP solution, no significant color changes were observed.
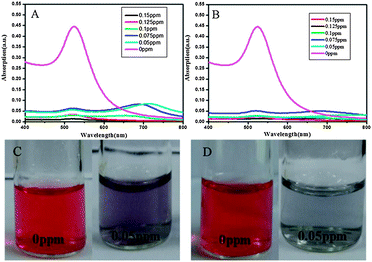 |
| Fig. 6 Absorption spectra of AuNPs and colorimetric changes at different pH values. (A and C) pH value is 4.0–5.0; (B and D) pH value is 2.5–3.5. | |
These phenomena were not observed at pH of 6.5–7.5 (Fig. 4A and 5). Therefore, in the microenvironment with lower pH values (such as 2.5–3.5 or 4.0–5.0), the colorimetric approach combined with UV-vis detection can not work well after the concentration of melamine increased to 0.075 ppm. In contrast, even when the melamine concentration was increased to 0.15 ppm, the changes in the hydrodynamic diameter could be clearly determined by the DLS based method in the solution with lower pH values (Fig. 7).
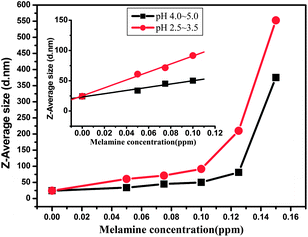 |
| Fig. 7 The average diameters of AuNPs as determined from DLS measurements and plotted against the melamine concentration in solutions with different pH values. Inset graph: linear relationship between average diameters of AuNPs and different concentrations of melamine. Black line: Y = 23.25 + 267.6X, R2 = 0.969; red line: Y = 25.05 + 657.9X, R2 = 0.992. | |
Meanwhile, by increasing the concentration of melamine from 0 to 0.01 ppm, a linear relationship between size changes of AuNPs and melamine concentration could be realized (Fig. 7, inset graph). Thus even lower pH values (such as 2.5–3.5 or 4.0–5.0) do not interfere with the detection of melamine through this DLS based approach and the changes in melamine concentration can be detected by capturing hydrodynamic changes of AuNPs. These results also indicate that the detection range of the DLS based approach is much wider than that of the colorimetric method in the acid system.
Interference from other substances
In order to evaluate the selectivity of this DLS-based method, the interference from other analytes was also investigated. Fig. 8 shows the changes in average diameter of the AuNPs in the presence of melamine and other potential interferents, respectively. It was clearly observed that only melamine induced the aggregation of AuNPs whereas other analytes did not. These results indicate that the DLS-based approach can work well for selective detection of melamine.
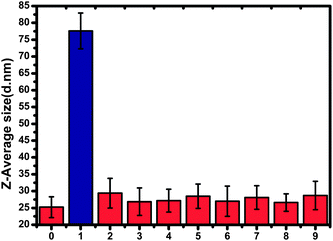 |
| Fig. 8 The average size of AuNPs in the presence of melamine or other analytes. (0) Control, (1) melamine, (2) aminoacetic acid, (3) L-alanine, (4) L-valine, (5) L-serine, (6) L-threonine, (7) L-isoleucine, (8) triethylamine and (9) ethanediamine. The concentrations of melamine and ethanediamine are 0.15 ppm, and that of other analytes is 15 ppm. | |
DLS detection of melamine in milk
To show whether DLS can be applied for the direct detection of melamine in milk samples, the samples were doped with melamine at different concentrations, and then the real samples were extracted by the method aforementioned in the experimental section. As shown in Fig. 9, the average hydrodynamic diameter increased by increasing the concentration of melamine added, from 0.05 ppm to 0.125 ppm. The lower detection limit was found to be 0.05 ppm in the real milk sample. Although the sensitivity of this DLS-based assay was lower than that of liquid chromatography-tandem mass spectrometry and extractive electrospray, this method still demonstrates highly reproducible and rapid detection in a simple way.
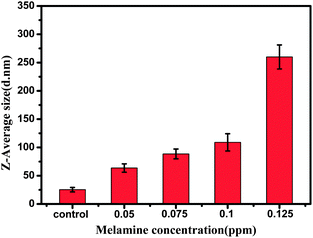 |
| Fig. 9 The average diameters of AuNPs at different concentrations of melamine in milk as determined from DLS measurement. | |
Conclusion
In summary, a DLS based approach was developed for monitoring melamine. We take advantage of the hydrodynamic diameter change of AuNPs induced by the strong interaction between melamine and AuNPs to detect melamine. Specifically, even in solutions with low pH values (of 2.5–3.5 or 4.0–5.0), this DLS method can work well for tracing different concentrations of melamine. In addition, the interaction between melamine and AuNPs can be completed within 20 min. And the results of this interaction can be directly traced by the DLS technique through the changes in AuNP size, which makes the DLS approach a potential tool for real-time detection of melamine in milk products.
Acknowledgements
This work was supported by Shanghai Sci. & Tech. Committee (12JC1407200), National 973 Project (2010CB933901), Program for Changjiang Scholars and Innovative Research Team in University (IRT1269).
Notes and references
- D. R. Kimbrough and A. C. Jensen, J. Chem. Educ., 2010, 87, 496–499 CrossRef CAS.
- H.-F. Ji and X. Xu, Langmuir, 2010, 26, 4620–4622 CrossRef CAS PubMed.
- C. A. Brown, K.-S. Jeong, R. H. Poppenga, B. Puschner, D. M. Miller, A. E. Ellis, K.-I. Kang, S. Sum, A. M. Cistola and S. A. Brown, J. Vet. Diagn. Invest., 2007, 19, 525–531 CrossRef PubMed.
- L. Zhu, G. Gamez, H. Chen, K. Chingin and R. Zenobi, Chem. Commun., 2009, 559–561 RSC.
- R. A. Yokley, L. C. Mayer, R. Rezaaiyan, M. E. Manuli and M. W. Cheung, J. Agric. Food Chem., 2000, 48, 3352–3358 CrossRef CAS PubMed.
- X. Zhu, S. Wang, Q. Liu, Q. Xu, S. Xu and H. Chen, J. Agric. Food Chem., 2009, 57, 11075–11080 CrossRef CAS PubMed.
- E. A. Garber, J. Food Prot., 2008, 71, 590–594 CAS.
- C. Lu, B. Xiang, G. Hao, J. Xu, Z. Wang and C. Chen, J. Near Infrared Spectrosc., 2009, 17, 59–67 CrossRef CAS.
- K. Ai, Y. Liu and L. Lu, J. Am. Chem. Soc., 2009, 131, 9496–9497 CrossRef CAS PubMed.
- W. J. Qi, D. Wu, J. Ling and C. Z. Huang, Chem. Commun., 2010, 46, 4893–4895 RSC.
- J. R. Kalluri, T. Arbneshi, S. Afrin Khan, A. Neely, P. Candice, B. Varisli, M. Washington, S. McAfee, B. Robinson, S. Banerjee, A. K. Singh, D. Senapati and P. C. Ray, Angew. Chem., Int. Ed., 2009, 48, 9668–9671 CrossRef CAS PubMed.
- H. Jans, X. Liu, L. Austin, G. Maes and Q. Huo, Anal. Chem., 2009, 81, 9425–9432 CrossRef CAS PubMed.
- X. Liu, Q. Dai, L. Austin, J. Coutts, G. Knowles, J. Zou, H. Chen and Q. Huo, J. Am. Chem. Soc., 2008, 130, 2780–2782 CrossRef CAS PubMed.
- B.-A. Du, Z.-P. Li and C.-H. Liu, Angew. Chem., Int. Ed., 2006, 45, 8022–8025 CrossRef CAS PubMed.
- J.-Y. Kim and J.-S. Lee, Nano Lett., 2009, 9, 4564–4569 CrossRef CAS PubMed.
- W. Haiss, N. T. K. Thanh, J. Aveyard and D. G. Fernig, Anal. Chem., 2007, 79, 4215–4221 CrossRef CAS PubMed.
- J. Liu and Y. Lu, Nat. Protoc., 2006, 1, 246–252 CrossRef CAS PubMed.
- S. Zeng, M. Cai, H. Liang and J. Hao, Anal. Methods, 2012, 4, 2499–2505 RSC.
- H. Chi, B. Liu, G. Guan, Z. Zhang and M.-Y. Han, Analyst, 2010, 135, 1070–1075 RSC.
- G. Braun, I. Pavel, A. R. Morrill, D. S. Seferos, G. C. Bazan, N. O. Reich and M. Moskovits, J. Am. Chem. Soc., 2007, 129, 7760–7761 CrossRef CAS PubMed.
- F. Chun-Liu, Q. Kun, Z. Jianhua, W. Shangbin, L. Xiaoxuan and Y. Shu-Hong, Nanotechnology, 2008, 19, 125601 CrossRef PubMed.
- J. Minglei, Y. Wuli, R. Qingguang and L. Daru, Nanotechnology, 2009, 20, 075101 CrossRef PubMed.
- G. B. Braun, S. J. Lee, T. Laurence, N. Fera, L. Fabris, G. C. Bazan, M. Moskovits and N. O. Reich, J. Phys. Chem. C, 2009, 113, 13622–13629 CAS.
- K.-W. Huang, C.-J. Yu and W.-L. Tseng, Biosens. Bioelectron., 2010, 25, 984–989 CrossRef CAS PubMed.
- Y.-L. Hung, T.-M. Hsiung, Y.-Y. Chen and C.-C. Huang, Talanta, 2010, 82, 516–522 CrossRef CAS PubMed.
- Z. Jiang, J. Li, G. Wen, Q. Liu and A. Liang, Sci. China: Chem., 2010, 53, 1049–1054 CrossRef CAS PubMed.
- B. Dusemund, A. Hoffmann, T. Salzmann, U. Kreibig and G. Schmid, Z. Phys. D: At., Mol. Clusters, 1991, 20, 305–308 CrossRef CAS.
- J. J. Storhoff, A. A. Lazarides, R. C. Mucic, C. A. Mirkin, R. L. Letsinger and G. C. Schatz, J. Am. Chem. Soc., 2000, 122, 4640–4650 CrossRef CAS.
- W.-H. Yang, G. C. Schatz and R. P. Van Duyne, J. Chem. Phys., 1995, 103, 869–875 CrossRef CAS.
- R. Elghanian, J. J. Storhoff, R. C. Mucic, R. L. Letsinger and C. A. Mirkin, Science, 1997, 277, 1078–1081 CrossRef CAS.
- A. Bozzi, M. Dhananjeyan, I. Guasaquillo, S. Parra, C. Pulgarin, C. Weins and J. Kiwi, J. Photochem. Photobiol., A, 2004, 162, 179–185 CrossRef CAS.
|
This journal is © The Royal Society of Chemistry 2014 |