Ultraviolet radiation-induced upregulation of antimicrobial proteins in health and disease†
Received
22nd May 2012
, Accepted 20th August 2012
First published on 4th September 2012
Abstract
This article reviews recent data on the expression, regulation and activation of antimicrobial peptides (AMP) in human skin, and considers their potential protective and pro-inflammatory roles following upregulation by ultraviolet radiation (UVR). Antimicrobial peptides are small peptides that are key components of the innate immune system, originally identified by their vital role in protecting the body-environment interface from infection. However, it has now become clear that AMP have more extensive actions, including the provision of pivotal links with the adaptive immune system. Moreover, aberrant AMP expression may contribute to immuno-modulated inflammatory dermatoses including psoriasis, eczema and the photoaggravated condition lupus erythematosus. Recent work has demonstrated the direct upregulation of AMP in healthy skin by cutaneous UVR exposure. This may serve to protect the skin from risks imposed by both the biophysical barrier-compromise and the immunosuppression that are attributable to UVR exposure. Furthermore, it is observed that UVR provokes upregulation of AMP in an atypical manner in the photosensitivity disorder polymorphic light eruption. Dysregulated UVR responses of these pro-inflammatory proteins may play a role in the pathogenesis of certain immune-mediated diseases caused or aggravated by sunlight.
Introduction
Antimicrobial peptides (AMP) are small peptides that guard the body-environment interface. To date, over 1900 AMP have been entered into the online database.1 They are present across epithelial surfaces including the skin, where due to their relative ease of accessibility for sampling they have become a rapidly expanding area of dermatological research. Whilst some AMP are present constitutively on the skin surface to provide a chemical barrier, others are rapidly inducible from keratinocytes or recruited, for instance from leukocytes, on skin wounding. As their name suggests, they were first discovered as a major component of the innate immune system. They have antimicrobial activity via a number of mechanisms, usually incorporating the disruption of microbial membranes.2 Of particular importance in human cutaneous defence are the human beta defensins (HBD)-2 and -3,3,4 psoriasin,5 ribonuclease (RNase)-76 and the cathelicidin peptide LL-37.6,7 Their antimicrobial efficacies vary and this is reflected in their distribution and inducibility. As an example, psoriasin, which is particularly effective against E. coli, is most abundant on the uninjured body surface in areas exposed to high bacterial colonization, specifically the hands, axillae, face and scalp and is rapidly inducible above its constitutive expression upon microbial infection or after skin barrier disruption.5,8
More recently, non-antimicrobial effects of AMP have been revealed, such as the promotion of cell growth, healing and angiogenesis.2,9,10 They can be upregulated by pro-inflammatory cytokines and, similarly, AMP themselves have diverse effects on inflammatory responses, their so-called ‘alarmin’ function: In some instances they stimulate the production of pro-inflammatory cytokines and chemokines11 and in other situations suppress tumor necrosis factor (TNF)-α release from monocytes and macrophages.12 Recently, Navid et al. (2012) demonstrated that defensins can contribute to immunosuppression via induction of regulatory T-cells.13 These conflicting actions suggest a possible role in balancing cutaneous inflammatory responses. AMP provide pivotal links between the innate and adaptive immune systems (Fig. 1). They are chemoattractant for effector cells including neutrophils, monocytes, macrophages, dendritic cells and lymphocytes. Furthermore, AMP activate antigen presenting cells, particularly dendritic cells, and modulate T-cell function.14–17 Regulatory factors in their expression include toll-like receptors (TLR).12
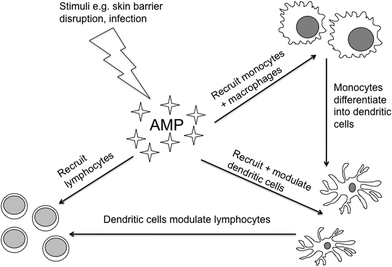 |
| Fig. 1 Schematic diagram illustrating links between AMP, a component of innate immunity, and the adaptive immune system. | |
Expression and role of AMP in inflammatory skin disorders
Psoriasis
Investigations into the contribution of AMP to skin disease began with the common inflammatory dermatosis psoriasis. Psoriasin, cathelicidin and β-defensins have been shown to be strongly upregulated in psoriatic keratinocytes18,19 and it is proposed that phototherapy may treat psoriasis by normalizing these aberrant AMP. Vahavihu et al. (2010) examined the effects of narrowband UVB (NB-UVB) therapy upon cutaneous AMP expression in patients with atopic dermatitis and psoriasis.20 Prior to treatment, psoriatic plaques displayed significantly elevated cathelicidin and HBD-2 expression as compared with healthy controls. The initial NB-UVB dose for each individual was one standard erythemal dose (SED) and doses were then gradually incremented. After six NB-UVB treatments, cathelicidin levels had increased further above baseline but this was to an insignificant degree, whilst HBD-2 expression did significantly decrease as the skin lesions improved (and serum vitamin D increased). Similar changes were seen in atopic dermatitis patients but were less marked.
Whether such AMP dysregulation is actually cause or consequence of cutaneous inflammation has been a matter of ongoing debate. Evidence in support of the former comes from the demonstration of complexes of the cathelicidin peptide, LL-37, together with self-DNA themselves activating plasmacytoid dendritic cells, and driving autoimmunity within psoriatic skin.21 Furthermore, an increased β-defensin gene copy number positively correlates with the risk of developing psoriasis22 and similarly serum HBD-2 levels correlate with psoriasis severity.23
Atopic eczema
It has been speculated that the relatively high incidence of cutaneous infections in eczema may be attributable to an abnormal suppression of AMP expression in sufferers,24 but recent studies have failed to consistently uphold this: Gläser et al. (2009)25 and Harder et al. (2010)8 demonstrated AMP induction (psoriasin, RNase-7, HBD-2, HBD-3) in lesional eczematous skin as compared to healthy controls, but these increases were smaller than those seen in psoriatic skin. This group also performed tape-stripping of healthy skin to simulate the epidermal barrier dysfunction that is found in atopic eczema.26 Tape-stripping alone led to the rapid secretion of psoriasin and RNase-7, and tape-stripping followed by occlusion stimulated an increase in HBD-2. Again these findings do not demonstrate causality but nevertheless evidence barrier disruption as a major stimulus to AMP upregulation.
Rosacea
Rosacea is a common, often photoaggravated inflammatory skin disease characterised by recurrent episodes of facial flushing and the development of papules, pustules and/or nodules. Following the discovery of raised cathelicidin levels in rosacea-affected skin,27 the potential role of AMP in its pathogenesis has been considered. In normal skin cathelicidin is broken down into smaller peptides that themselves have greater antimicrobial but lesser inflammatory activities28 but, in contrast, cathelicidins in rosacea-affected skin remain in their larger peptide forms.27 LL-37 is the dominant member of this subgroup and does itself promote inflammation and angiogenesis,29 which may be important particularly in the erythematotelangiectatic subgroup of rosacea.
Lupus erythematosus
Photosensitivity, a malar rash and discoid rash are three of the eleven American College of Rheumatology criteria (1982, updated 1997) for the classification of systemic lupus erythematosus (SLE), a chronic, multisystem, autoimmune disease (a total of four criteria being required for SLE diagnosis).30 A study focussing on cutaneous variants of lupus erythematosus (LE), specifically discoid LE (DLE), subacute cutaneous LE (SCLE) and lupus tumidus (LET) has revealed differential elevation and altered patterns of AMP expression dependent upon LE subtype.31 Thus, HBD-2 and HBD-3 were increased in DLE and SCLE as compared with healthy skin, but not present in LET; LL-37 was higher in all LE subtypes than healthy controls; and psoriasin was higher in SCLE and DLE as compared with healthy controls. However, study biopsies were only taken from pre-existing lupus lesions and not a newly-developed or photo-provoked rash.
It is still possible however that AMP dysregulation may drive the immune system dysfunction that is seen in lupus patients, and this is supported by the findings of Lande et al. (2011)32 who, in a similar fashion to the AMP–DNA complexes demonstrated in psoriasis,21 identified complexes of self-DNA together with neutrophil-derived AMP in the sera of SLE patients. Moreover, these complexes themselves activated plasmacytoid dendritic cells through the activation of TLR-9. Patients additionally developed autoantibodies against both AMP and self-DNA, thereby implicating AMP–DNA complexes as potential autoantigens that may activate the adaptive immune system.
Expression and potential role of AMP in UVR-injured healthy skin
UVR exposure is an injurious agent to the skin, inducing a variety of changes. Indeed even suberythemogenic exposures affect the barrier function of skin, evidenced by increased trans-epidermal water loss.33 UVR also directly affects the immune system, causing immunosuppression particularly by the induction of regulatory T-cells.34 Much controversy has surrounded the role of UVR in cutaneous infections. UVR exposure is proposed to exacerbate skin infections through its immunosuppressive effects. Indeed, provocation of labial herpes outbreaks (“cold sores”) by UVR in susceptible individuals is well-documented.35 However, there is little other supporting evidence for this proposal. Rather, impetiginised eczema can improve solely with UVR treatment.36 Similarly, reports of secondary infection after sunburn from prolonged solar UVR exposure or following UVR treatments for skin disease are virtually non-existent. The question then arises how this is possible, given that UVR can impair the skin barrier and is an effective immunosuppressant?37
Gläser et al. (2009) irradiated normal human keratinocytes with UVR (TL 20/W12 lamps, Philips, Eindhoven, The Netherlands; 290–320 nm; emission peak 313 nm; 0, 150 and 350 J m−2) and demonstrated dose-dependent increases in HBD-2, HBD-3, RNase-7 and psoriasin expression 24 hours after irradiation.38 Additionally, buttock skin of six human volunteers (3 male, 3 female; ages 25–51 years; skin phototype II or III) was irradiated with 1 minimal erythemal dose (MED) of UVB on three consecutive days.38 Biopsy samples taken 1 day after the last irradiation showed RNase-7 and psoriasin to be most and HBD-2 least strongly induced by UVR. Furthermore, the patterns of expression varied between AMP. For instance, RNase-7 was homogenously expressed close to the corneal layer, whilst psoriasin showed a more focal and follicular pattern. This enhanced expression persisted in buttock skin for at least 6 days after the UVR exposure. It is therefore postulated that whilst UVR suppresses T-cell-mediated immune responses,39 its simultaneous induction of innate immunity, i.e. AMP, may serve to counteract any potential increased risk of cutaneous infections.
We have subsequently noted findings consistent with the above study in 5 further healthy volunteers. Three volunteers (2 females of skin phototype II, ages 30- and 59-years; 1 male of phototype V, age 23-years) were given a prolonged course of suberythemal UVR exposures to an area of buttock skin which was irradiated three times a week for 6 weeks with 1.3 SED, using fluorescent lamps with UVR emission spectrum similar to sunlight (Philips HB598, Eindhoven, The Netherlands, fitted with Arimed B [Cosmedico GmbH, Stuttgart, Germany] and Cleo Natural [Philips, Eindhoven, The Netherlands] lamps; emission 290–400 nm, 95% UVA: 320–400 nm, 5% UVB: 290–320 nm). Two further female volunteers (phototype II, ages 50- and 61-years) were irradiated on just one occasion with a higher dose of UVB, of twice their MED, to an area of buttock skin (Waldmann UV 236B, Herbert Waldmann GmbH & Co. KG, Germany; 285–350 nm, peak emission 312 nm). Ethical approval was granted by the North West ethics committee (ref. 09/H1014/73). Biopsy samples were taken 24 hours after UVR (i.e. 24 hours following the last UVR dose in the multiple-dose group) from irradiated skin and also non-irradiated buttock skin for comparison, and sections were immunohistochemically stained for AMP expression [goat anti-HBD-2 antibodies (Peprotech Cell Concepts, NJ, U.S.A.), rabbit anti-HBD-3 antibodies (Peprotech Cell Concepts, NJ, U.S.A.), a polyclonal goat antibody directed against human RNAse 7 (kindly provided by J. Harder, Kiel Germany), and a monoclonal antibody directed against human psoriasin (kindly provided by H. Lange, Kiel, Germany)]. Despite the above differing UVR treatment protocols, similar patterns of AMP upregulation were seen, which were also consistent with the previous report.38 Large focal increases in psoriasin expression, and moderate increases in RNase-7 in the upper epidermis, were prominent findings (Fig. 2, central column), supporting the induction of these AMP in healthy human skin by UVR. In contrast, HBD-3 showed modest increase while HBD-2 appeared unaffected by the UVR exposure in healthy skin.
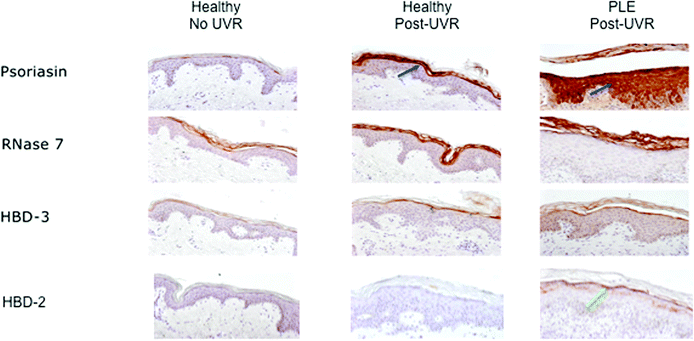 |
| Fig. 2 AMP expression in healthy skin and UVR-provoked PLE rash. From left: unexposed skin from healthy volunteer; healthy skin post-2 MED; PLE-affected skin following UVR provocation. Blue arrows indicate strong upregulation of psoriasin expression in the upper epidermis of healthy skin post-UVR, and dramatic increases in its expression throughout the epidermis following UVR-provocation of PLE; RNase-7 (strongly) and HBD-3 (modestly) were similarly upregulated in UVR-exposed healthy skin and provoked PLE compared with unexposed healthy skin; HBD-2 was not upregulated in healthy skin following UVR exposure but did show some increase in the upper epidermis of PLE-affected skin (green arrow). | |
Abnormal AMP response in polymorphic light eruption and potential contribution to pathogenesis
Since exposure to UVR is thus known to upregulate certain AMP, we examined whether aberrant expression of these pro-inflammatory proteins or ‘alarmins’ might potentially underpin the commonest immune-mediated inflammatory photosensitivity disorder in Northern Europeans, namely polymorphic light eruption (PLE; Fig. 3).40 This exploratory work was approved by the North West ethics committee (ref. 09/H1014/73, U.K.). In brief, patients were recruited from the supraregional Photobiology Unit, Dermatology Centre, Manchester, U.K., where they underwent clinical and histological confirmation of PLE. Volunteers (n = 3; skin phototypes I–III; one male; median age 39-years, range 33–72; median duration of PLE 18-years, range 6–51) underwent provocation testing to UVR according to our standard provocation regimen.41 Thus, provocation testing was performed to broadband UVA using a custom-built arm exposure unit incorporating Cleo Performance bulbs (310–400 nm, Philips Healthcare U.K. Ltd, Guildford, U.K.) and solar simulated radiation (SSR; 290–400 nm, 1 KW xenon arc plus atmospheric attenuation filter, Newport Spectra-Physics Ltd, Newport, California, U.S.A.). A 5 × 5 cm area of each ventral forearm skin was exposed on up to three consecutive days to 15 J cm−2 broadband UVA or 10 J cm−2 SSR.
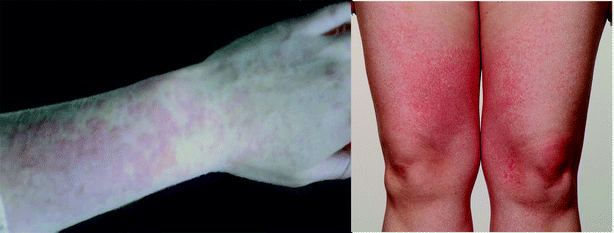 |
| Fig. 3 Polymorphic light eruption: typical appearance of erythematous papules on photoexposed sites. Patient consent gained for publication of these photographs. | |
Skin was biopsied once PLE-rash had developed, which required one SSR-challenge in one patient, three SSR-challenges in another patient, and two UVA-challenges in the third. Punch biopsy samples were taken from the involved area at 24 hours following the last provocation challenge, and from a non-irradiated, distant site of ventral arm skin. Immunohistochemical assessment was performed of a range of AMP utilizing the antibodies mentioned above. Non-irradiated skin from the PLE-patients had no noticeable abnormalities in AMP expression when compared to healthy volunteers. However, the most striking difference in the PLE-provoked skin was that of dramatically upregulated psoriasin expression and a change in its expression-pattern to expression throughout the epidermis rather than the smaller, focal increases seen in healthy skin post-irradiation (Fig. 2). This was accompanied by augmented HBD-2 expression within PLE-affected skin compared with UVR-exposed healthy skin that was limited to the stratum corneum and upper epidermis, while HBD-3 and RNase-7 showed no obvious difference from that of UVR-exposed healthy skin.
This novel finding of upregulation and altered expression patterns of AMP in the early stages of PLE rash development i.e. in UVR-provoked PLE, as compared with healthy skin post-UVR exposure, was found in all three patients. Potentially, dysregulated AMP expression following UVR could play some part in the relative failure of photoimmunosuppression that is described in PLE.42 Whilst our early findings cannot be assumed to be causal in PLE, the upregulation of such peptides that are known to be pro-inflammatory does however suggest a potential role of AMP in disease pathogenesis or amplification, and indicate a need for continued study of AMP and PLE/photosensitivity.
Vitamin D3 exerts pleiotropic effects on skin immune responses and there is recent evidence that this includes some AMP. Cells of both the innate and adaptive immune systems possess vitamin D receptors43 so enabling, for instance, vitamin D3-induced suppression of antigen presenting cell (particularly dendritic cell) maturation and T-cell proliferation, in addition to the modulation of cytokine secretion and induction of regulatory T-cells.44 The promoter regions of cathelicidin and HBD-2 have been shown to possess vitamin D-response elements that mediate vitamin D3-dependent gene expression in a variety of cell types including keratinocytes, monocytes and neutrophils.45 Mallbris et al. (2005) showed that UVB exposure (which triggers vitamin D synthesis), but importantly not UVA exposure, stimulates production of the AMP cathelicidin, with correlated increases in vitamin D receptor expression.46
Topical vitamin D3 analogues such as calcipotriol suppress cutaneous immune responses,47 with recent investigations suggesting that AMP modulation within psoriatic keratinocytes may contribute to the success of these agents in psoriasis treatment. Clinically effective treatment with calcipotriol is associated with decreased HBD-2 and HBD-3 expression48 and, similarly, a suppression of the psoriasin (and koebnerisin, another AMP highly homologous to psoriasin, implicated in psoraisis pathogenesis) upregulation found in lesional psoriatic skin.49 The latter group found evidence that the beneficial action of calcipotriol may be due to modulation of Th17-mediated AMP regulation. Psoriasin and koebnerisin upregulate inflammatory cytokines, so further amplifying the immune response. Calcipotriol treatment was seen to reduce the increase in psoriasin and koebnerisin in epidermal keratinocytes triggered by IL-17A.49
Interestingly, in PLE-sufferers, treatment of skin with calcipotriol before UVR provocation challenge led to a reduction in PLE symptoms, evidenced by a 32% reduction in overall PLE test scores.50 Conceivably, effects may be mediated via actions on AMP/innate immunity in addition to adaptive immunity.51,52 Photosensitive patients have lower serum 25-dihydroxyvitamin D3 levels than a seasonally-matched healthy population53 and potentially this might serve to further exacerbate underlying AMP dysregulation. A complex relationship may thus exist between UVR exposure and the vitamin D3 synthesis and AMP upregulation that it triggers. We highlight this schematically (Fig. 4).
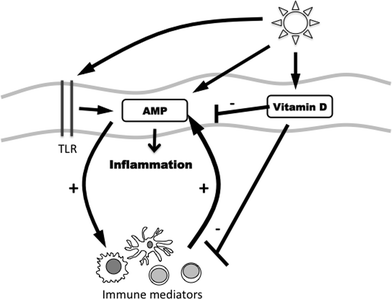 |
| Fig. 4 Schematic illustrating the hypothesised interactions between sunlight exposure and the cutaneous immune systems, including their regulation by TLR. Furthermore, vitamin D may act to suppress innate and adaptive immune responses. Abbreviations: Toll-like receptor (TLR), antimicrobial peptide (AMP). | |
Conclusions
AMP are fundamental constituents of the innate immune system, providing a link between the external environment and adaptive immune system. In addition to their antimicrobial role, their altered expression has been demonstrated and their pathological role considered in certain inflammatory dermatoses. The reproducible patterns of AMP upregulation in healthy human skin following UVR exposure may potentially protect the UVR-injured epithelium from invading microorganisms and promote wound healing. In contrast, dysregulated AMP responses to UVR may play a role in the photoaggravated conditions of LE and rosacea, and the photosensitivity disorder PLE. In the latter, UVR exposure rapidly alters the expression of these pro-inflammatory proteins, particularly psoriasin, which shows dramatically increased expression throughout the epidermis in the initial part of PLE-rash induction, where it may play an early role in the pathogenesis or contribute to the inflammatory milieu. Further research is clearly indicated to explore the role of UVR-induced AMP, and their regulation, in skin health and disease.
References
- G. Wang, X. Li and Z. Wang, APD2: the updated antimicrobial peptide database and its application in peptide design, Nucleic Acids Res., 2009, 37, D933–D937 CrossRef CAS . The Antimicrobial Peptide online database, available from http://aps.unmc.edu/ap/main.php [accessed 8 May 2012].
- M. Zanetti, Cathelicidins, multifunctional peptides of the innate immunity, J. Leukocyte Biol., 2004, 75, 39–48 CrossRef CAS.
- J. Harder, R. Siebert, Y. Zhang, P. Matthiesen, E. Christophers, B. Schlegelberger and J. M. Schröder, Mapping of the gene encoding human beta-defensin-2 (DEFB2) to chromosome region 8p22-p23.1, Genomics, 1997, 46, 472–475 CrossRef CAS.
- J. M. Schröder and J. Harder, Human beta-defensin-2, Int. J. Biochem. Cell Biol., 1999, 31, 645–651 CrossRef.
- R. Gläser, J. Harder, H. Lange, J. Bartels, E. Christophers and J. M. Schröder, Antimicrobial psoriasin (S100A7) protects human skin from Escherichia coli infection, Nat. Immunol., 2005, 6, 57–64 CrossRef.
- J. Harder and J. M. Schröder, RNase 7, a novel innate immune defense antimicrobial protein of healthy human skin, J. Biol. Chem., 2002, 277, 46779–46784 CrossRef CAS.
- R. A. Dorschner, V. K. Pestonjamasp, S. Tamakuwala, T. Ohtake, J. Rudisill, V. Nizet, B. Agerberth, G. H. Gudmundsson and R. L. Gallo, Cutaneous injury induces the release of cathelicidin anti-microbial peptides active against group A Streptococcus, J. Invest. Dermatol., 2001, 117, 91–97 CrossRef CAS.
- J. Harder, S. Dressel, M. Wittersheim, J. Cordes, U. Meyer-Hoffert, U. Mrowietz, R. Fölster-Holst, E. Proksch, J. M. Schröder, T. Schwarz and R. Gläser, Enhanced expression and secretion of antimicrobial peptides in atopic dermatitis and after superficial skin injury, J. Invest. Dermatol., 2010, 130, 1355–1364 CrossRef CAS.
- R. Bucki, K. Leszczyńska, A. Namiot and W. Sokołowski, Cathelicidin LL-37: a multitask antimicrobial peptide, Arch. Immunol. Ther. Exp., 2010, 58, 15–25 CrossRef CAS.
- R. Ramos, J. P. Silva, A. C. Rodrigues, R. Costa, L. Guardão, F. Schmitt, R. Soares, M. Vilanova, I. Domingues and M. Gama, Wound healing activity of the human antimicrobial peptide LL37, Peptides, 2011, 32, 1469–1476 CrossRef CAS.
- F. Niyonsaba, H. Ushio, N. Nakano, W. Ng, K. Sayama, K. Hashimoto, I. Nagaoka, K. Okumura and H. Ogawa, Antimicrobial peptides human beta-defensins stimulate pidermal keratinocyte migration, proliferation and production of proinflammatory cytokines and chemokines, J. Invest. Dermatol., 2007, 127, 594–604 CrossRef CAS.
- N. Mookherjee, K. L. Brown, D. M. Bowdish, S. Doria, R. Falsafi, K. Hokamp, F. M. Roche, R. Mu, G. H. Doho, J. Pistolic, J. P. Powers, J. Bryan, F. S. Brinkman and R. E. Hancock, Modulation of the TLR-mediated inflammatory response by the endogenous human host defense peptide LL-37, J. Immunol., 2006, 176, 2455–2464 CAS.
- F. Navid, M. Boniotto, C. Walker, K. Ahrens, E. Proksch, T. Sparwasser, W. Müller, T. Schwarz and A. Schwarz, Induction of regulatory T cells by a murine β-defensin, J. Immunol., 2012, 188, 735–743 CrossRef CAS.
- D. Yang, O. Chertov, S. N. Bykovskaia, Q. Chen, M. J. Buffo, J. Shogan, M. Anderson, J. M. Schröder, J. M. Wang, O. M. Howard and J. J. Oppenheim, Beta-defensins: linking innate and adaptive immunity through dendritic and T cell CCR6, Science, 1999, 286, 525–528 CrossRef CAS.
- A. Biragyn, P. A. Ruffini, C. A. Leifer, E. Klyushnenkova, A. Shakhov, O. Chertov, A. K. Shirakawa, J. M. Farber, D. M. Segal, J. J. Oppenheim and L. W. Kwak, Toll-like receptor 4-dependent activation of dendritic cells by beta-defensin 2, Science, 2002, 298, 1025–1029 CrossRef CAS.
- K. Wuerth and R. E. Hancock, New insights into cathelicidin modulation of adaptive immunity, Eur. J. Immunol., 2011, 41, 2817–2819 CrossRef CAS.
- F. Morgera, S. Pacor, L. Creatti, N. Antcheva, L. Vaccari and A. Tossi, Effects on antigen-presenting cells of short-term interaction with the human host defence peptide β-defensin 2, Biochem. J., 2011, 436, 537–546 CrossRef CAS.
- J. Harder and J. M. Schröder, Psoriatic scales: a promising source for the isolation of human skin-derived antimicrobial proteins, J. Leukocyte Biol., 2005, 77, 476–486 CrossRef CAS.
- T. Gambichler, M. Skrygan, N. S. Tomi, N. Othlinghaus, N. H. Brockmeyer, P. Altmeyer and A. Kreuter, Differential mRNA expression of antimicrobial peptides and proteins in atopic dermatitis as compared to psoriasis vulgaris and healthy skin, Int. Arch. Allergy Immunol., 2008, 147, 17–24 CrossRef CAS.
- K. Vähävihu, M. Ala-Houhala, M. Peric, P. Karisola, H. Kautiainen, T. Hasan, E. Snellman, H. Alenius, J. Schauber and T. Reunala, Narrowband ultraviolet B treatment improves vitamin D balance and alters antimicrobial peptide expression in skin lesions of psoriasis and atopic dermatitis, Br. J. Dermatol., 2010, 163, 321–328 CrossRef.
- R. Lande, J. Gregorio, V. Facchinetti, B. Chatterjee, Y. H. Wang, B. Homey, W. Cao, Y. H. Wang, B. Su, F. O. Nestle, T. Zal, I. Mellman, J. M. Schröder, Y. J. Liu and M. Gilliet, Plasmacytoid dendritic cells sense self-DNA coupled with antimicrobial peptide, Nature, 2007, 449, 564–569 CrossRef CAS.
- E. J. Hollox, U. Huffmeier, P. L. Zeeuwen, R. Palla, J. Lascorz, D. Rodijk-Olthuis, P. C. van de Kerkhof, H. Traupe, G. de Jongh, M. den Heijer, A. Reis, J. A. Armour and J. Schalkwijk, Psoriasis is associated with increased beta-defensin genomic copy number, Nat. Genet., 2008, 40, 23–25 CrossRef CAS.
- P. A. M. Jansen, D. Rodijk-Olthuis, E. J. Hollox, M. Kamsteeg, S. Geuranne, G. S. Tjabringa, G. J. de Jongh, M. J. J. van Vlijmen-Willems, J. G. M. Bergboer, M. M. van Rossum, E. M. G. J. de Jong, M. den Heijer, A. W. M. Evers, M. Bergers, J. A. L. Armour, P. L. J. M. Zeeuwen and J. Schalkwijk, β-Defensin-2 protein is a serum biomarker for disease activity in psoriasis and reaches biologically relevant concentrations in lesional skin, PLoS One, 2009, 4, e4725 Search PubMed.
- M. Boguniewicz and D. Y. Leung, Atopic dermatitis, J. Allergy Clin. Immunol., 2006, 117, S475–S480 CrossRef CAS.
- R. Gläser, U. Meyer-Hoffert, J. Harder, J. Cordes, M. Wittersheim, J. Kobliakova, R. Fölster-Holst, E. Proksch, J. M. Schröder and T. Schwarz, The antimicrobial protein psoriasin (S100A7) is upregulated in atopic dermatitis and after experimental skin barrier disruption, J. Invest. Dermatol., 2009, 129, 641–649 CrossRef.
- C. N. Palmer, A. D. Irvine, A. Terron-Kwiatkowski, Y. Zhao, H. Liao, S. P. Lee, D. R. Goudie, A. Sandilands, L. E. Campbell, F. J. Smith, G. M. O'Regan, R. M. Watson, J. E. Cecil, S. J. Bale, J. G. Compton, J. J. DiGiovanna, P. Fleckman, S. Lewis-Jones, G. Arseculeratne, A. Sergeant, C. S. Munro, B. El Houate, K. McElreavey, L. B. Halkjaer, H. Bisgaard, S. Mukhopadhyay and W. H. McLean, Common loss-of-function variants of the epidermal barrier protein filaggrin are a major predisposing factor for atopic dermatitis, Nat. Genet., 2006, 38, 441–446 CrossRef CAS.
- K. Yamasaki, A. Di Nardo, A. Bardan, M. Murakami, T. Ohtake, A. Coda, R. A. Dorschner, C. Bonnart, P. Descargues, A. Hovnanian, V. B. Morhenn and R. L. Gallo, Increased serine protease activity and cathelicidin promotes skin inflammation in rosacea, Nat. Med., 2007, 13, 975–980 CrossRef CAS.
- J. Schauber and R. L. Gallo, Antimicrobial peptides and the skin immune defense system, J. Allergy Clin. Immunol., 2008, 122, 261–266 CrossRef CAS.
- R. Koczulla, G. von Degenfeld, C. Kupatt, F. Krötz, S. Zahler, T. Gloe, K. Issbrücker, P. Unterberger, M. Zaiou, C. Lebherz, A. Karl, P. Raake, A. Pfosser, P. Boekstegers, U. Welsch, P. S. Hiemstra, C. Vogelmeier, R. L. Gallo, M. Clauss and R. Bals, An angiogenic role for the human peptide antibiotic LL-37/hCAP-18, J. Clin. Invest., 2003, 111, 1665–1672 CAS.
- M. C. Hochberg, Updating the American College of Rheumatology revised criteria for the classification of systemic lupus erythematosus, Arthritis Rheum., 1997, 40, 1725 CrossRef CAS.
- A. Kreuter, M. Jaouhar, M. Skrygan, C. Tigges, M. Stücker, P. Altmeyer, R. Gläser and T. Gambichler, Expression of antimicrobial peptides in different subtypes of cutaneous lupus erythematosus, J. Am. Acad. Dermatol., 2011, 65, 125–133 CrossRef CAS.
- R. Lande, D. Ganguly, V. Facchinetti, L. Frasca, C. Conrad, J. Gregorio, S. Meller, G. Chamilos, R. Sebasigari, V. Riccieri, R. Bassett, H. Amuro, S. Fukuhara, T. Ito, Y. J. Liu and M. Gilliet, Neutrophils activate plasmacytoid dendritic cells by releasing self-DNA-peptide complexes in systemic lupus erythematosus, Sci. Transl. Med., 2011, 3, 73ra19 CrossRef.
- S. H. Lim, S. M. Kim, Y. W. Lee, K. J. Ahn and Y. B. Choe, Change of biophysical properties of the skin caused by ultraviolet radiation-induced photodamage in Koreans, Skin Res. Technol., 2008, 14, 93–102 CAS.
- T. Schwarz and A. Schwarz, Molecular mechanisms of ultraviolet radiation-induced immunosuppression, Eur. J. Cell Biol., 2011, 90, 560–564 CrossRef CAS.
- M. Norval, The effect of ultraviolet radiation on human viral infection, Photochem. Photobiol., 2006, 82, 1495–1504 CAS.
- S. H. Silva, A. C. Guedes, B. Gontijo, A. M. Ramos, L. S. Carmo, L. M. Farias and J. R. Nicoli, Influence of narrow-band UVB phototherapy on cutaneous microbiota of children with atopic dermatitis, J. Eur. Acad. Dermatol. Venereol., 2006, 20, 1114–1120 CrossRef CAS.
- T. Schwarz, The dark and the sunny sides of UVR-induced immunosuppression: photoimmunology revisited, J. Invest. Dermatol., 2010, 130, 49–54 CrossRef CAS.
- R. Gläser, F. Navid, W. Schuller, C. Jantschitsch, J. Harder, J. M. Schröder, A. Schwarz and T. Schwarz, UV-B radiation induces the expression of antimicrobial peptides in human keratinocytes in vitro and in vivo, J. Allergy Clin. Immunol., 2009, 123, 1117–1123 CrossRef.
- T. Schwarz, 25 years of UV-induced immunosuppression mediated by T cells-from disregarded T suppressor cells to highly respected regulatory T cells, Photochem. Photobiol., 2008, 84, 10–18 CrossRef CAS.
- L. E. Rhodes, M. Bock, A. S. Janssens, T. C. Ling, L. Anastasopoulou, C. Antoniou, F. Aubin, T. Bruckner, B. Faivre, N. K. Gibbs, C. Jansen, S. Pavel, A. J. Stratigos, F. R. de Gruijl and T. L. Diepgen, Polymorphic light eruption occurs in 18% of Europeans and does not exhibit higher prevalence with increasing latitude: multicentre survey of 6895 individuals residing from the Mediterranean to Scandinavia, J. Invest. Dermatol., 2010, 130, 626–628 CrossRef CAS.
-
A. K. Haylett and L. E. Rhodes, Evaluation of the patient with photosensitivity, in Allergy Frontiers: Diagnosis and Health Economic, ed. S. T. Holgate, R. Pawankar and L. Rosenwaser, Springer-Verlag, Japan, 2009, pp. 387–402 Search PubMed.
- R. A. Palmer and P. S. Friedmann, Ultraviolet radiation causes less immunosuppression in patients with polymorphic light eruption than in controls, J. Invest. Dermatol., 2004, 122, 291–294 CrossRef CAS.
- E. Van Etten and C. Mathieu, Immunoregulation by 1,25-dihydroxyvitamin D3: basic concepts, J. Steroid Biochem. Mol. Biol., 2005, 97, 93–101 CrossRef CAS.
- E. May, K. Asadullah and U. Zugel, Immunoregulation through 1,25 dihydroxyvitamin D3 and its analogs, Curr. Drug Targets: Inflammation Allergy, 2004, 3, 377–393 CrossRef CAS.
- T. T. Wang, F. P. Nestel, V. Bourdeau, Y. Nagai, Q. Wang, J. Liao, L. Tavera-Mendoza, R. Lin, J. W. Hanrahan, S. Mader and J. H. White, Cutting edge: 1,25-dihydroxyvitamin D3 is a direct inducer of antimicrobial peptide gene expression, J. Immunol., 2004, 173, 2909–2912 CAS.
- L. Mallbris, D. W. Edström, L. Sunblind, F. Granath and M. Stahle, UVB upregulates the antimicrobial protein hCAP18 mRNA in human skin, J. Invest. Dermatol., 2005, 125, 1072–1074 CAS.
- D. L. Damian, Y. J. Kim, K. M. Dixon, G. M. Halliday, A. Javeri and R. S. Mason, Topical calcitriol protects from UV-induced genetic damage but suppresses cutaneous immunity in humans, Exp. Dermatol., 2010, 19, e23–e30 CrossRef.
- M. Peric, S. Koglin, Y. Dombrowski, K. Gross, E. Bradac, A. Büchau, A. Steinmeyer, U. Zügel, T. Ruzicka and J. Schauber, Vitamin D analogs differentially control antimicrobial peptide/“alarmin” expression in psoriasis, PLoS One, 2009, 22(4), e6340 Search PubMed.
- Z. Hegyi, S. Zwicker, D. Bureik, M. Peric, S. Koglin, A. Batycka-Baran, J. C. Prinz, T. Ruzicka, J. Schauber and R. Wolf, Vitamin D analog calcipotriol suppresses the Th17 cytokine-induced proinflammatory S100 “alarmins” psoriasin (S100A7) and koebnerisin (S100A15) in psoriasis, J. Invest. Dermatol., 2012, 132, 1416–1424 CrossRef CAS.
- A. Gruber-Wackernagel, I. Bambach, F. J. Legat, A. Hofer, S. N. Byrne, F. Quehenberger and P. Wolf, Randomized double-blinded placebo-controlled intra-individual trial on topical treatment with a 1,25-dihydroxyvitamin D3 analogue in polymorphic light eruption, Br. J. Dermatol., 2011, 165, 152–163 CrossRef CAS.
- T. N. Dam, B. Moller, J. Hindkjaer and K. Kragballe, The vitaminD3 analog calcipotriol suppresses the number and antigen-presenting function of Langerhans cells in normal human skin, J. Invest. Dermatol. Symp. Proc., 1996, 1, 72–77 CAS.
- M. Ghoreishi, P. Bach, J. Obst, M. Komba, J. C. Fleet and J. P. Dutz, Expansion of antigen-specific regulatory T cells with the topical vitamin D analog calcipotriol, J. Immunol., 2009, 182, 6071–6078 CrossRef CAS.
- S. J. Cooper, R. Kift, A. Webb, M. Durkin, A. Vail, J. Berry and L. E. Rhodes, Relative risk of vitamin D insufficiency in photosensitivity conditions: a comparative study of photosensitive and healthy people living at northerly latitude, Br. J. Dermatol., 2011, 165(Suppl 1), 129 Search PubMed (Abstr).
Footnote |
† This article is published as part of a themed issue on current topics in photodermatology. |
|
This journal is © The Royal Society of Chemistry and Owner Societies 2013 |