Photopheresis (extracorporeal photochemotherapy)†
Received
10th May 2012
, Accepted 23rd July 2012
First published on 3rd August 2012
Abstract
Photopheresis is a form of phototherapy where specialized equipment is used to isolate a leukocyte fraction from the peripheral blood which is then exposed to photoactivated 8-methoxypsoralen and reinfused into the patient. At the time of its invention the treatment was conceptually based on the hypothesis of T cell vaccination, i.e. the observation in experimental studies that exposure of the immune system to physically modified T cell clones leads to a specific inhibition of T cell mediated autoimmunity. Consequently, photopheresis has been tried in a variety of conditions where T cells are thought to have a critical role and has shown clinical efficacy mainly in variants of cutaneous T cell lymphomas, graft-versus-host disease, systemic sclerosis, in solid organ transplant rejection and Crohn's disease. Evidence has accumulated that alterations in antigen presentation and the generation of regulatory T cells are induced by photopheresis and might be related to the observed clinical effects. Summarizing what has been published in the 25 years since its introduction into the clinic, photopheresis to date has found its place in the treatment of the above mentioned conditions as a well tolerated treatment option that can safely be combined with other established modalities. It can be expected that further research will help refine its clinical indications and close the gaps that still exist in our knowledge on when, how, and why photopheresis works.
Introduction
Photopheresis (ECP; which has been variously called also extracorporeal photopheresis, extracorporeal photochemotherapy, or extracorporeal photoimmunotherapy) is a form of phototherapy where blood is exposed extracorporeally to the photoactivated drug 8-methoxypsoralen (8-MOP). The development of ECP was initially based on the concept that reinfusion of extracorporeally modified leukocytes might initiate a beneficial immune reaction in patients with leukemic and autoimmune diseases.1 Studies have shown clinical efficacy of ECP in certain variants of cutaneous T cell lymphomas (CTCL; for which it is approved by the U.S. Food and Drug Administration), systemic sclerosis (SSc), graft-versus-host disease (GVHD), solid organ transplant rejection, and Crohn's disease (CD).2 Although the mechanisms of action of ECP are still not fully elucidated recent studies indicate that regulatory T cells (T-reg) and possibly also monocyte-derived antigen presenting cells are induced during treatment and might be responsible for the immunomodulation occurring during ECP.3 This paper reviews the development of ECP, experimental evidence on its mechanisms, and its clinical use in the above mentioned conditions.
Development of ECP and mechanisms of action
The modern history of photochemotherapy with 8-MOP and UVA (PUVA) started in the middle of the last century and is reviewed elsewhere in this issue.4 PUVA turned out to be highly efficacious for the treatment of psoriasis and other skin diseases. Prominent among these PUVA-responsive skin diseases is mycosis fungoides (MF), the most common CTCL variant.5
It was in the early 1980s that Richard Edelson, a dermatologist then at Columbia University, invented the concept of treating with PUVA leukocytes isolated from the bloodstream of patients with Sezary syndrome (SS; another CTCL variant, characterized in addition to generalized skin involvement by a high burden of malignant lymphocytes in the peripheral blood) instead of the skin surface.1 This intriguing idea was based on the concept of T cell vaccination as postulated by Irun Cohen and his group at the Weizmann Institute.6 During T cell vaccination autoreactive T cells from rats with experimental autoimmune disease are stimulated ex vivo (with antigen or mitogen) and exposed to irradiation, hydrostatic pressure, or glutaraldehyde before inoculation. Experimentally T cell vaccination can prevent the development of subsequent autoimmune disease and induce long lasting remissions of established disease. Further evidence indicated that an anti-idiotypic response to disease specific T cell receptors was behind the observed clinical effects.7 According to initial experiments performed in Richard Edelson's laboratory similar results could be obtained when substituting the extracorporeal treatments used by Cohen's group with PUVA.8 Furthermore, evidence was obtained that protective effects can also be achieved when unfractionated splenocytes instead of isolated autoreactive T cell clones were used.9,10 These observations, namely that 8-MOP can be used, a drug whose safety in humans had already been demonstrated, and that isolation of T cell clones is not required, paved the way for further development of a new form of treatment which later became known as photopheresis. For this purpose an apparatus was devised that combined a centrifuge for the separation of white from red blood cells based on their sedimentation rate and a UV exposure system. Based upon cytotoxicity experiments in culture the optimal UVA dose was determined to be 1–2 J cm−2, which – combined with 100 ng ml−1 of 8-MOP – leads to growth arrest and apoptosis in almost all treated lymphocytes.1 Homogeneous UVA dose distribution is achieved by passing the leukocytes between fluorescent UVA lamps in a plastic chamber at a film thickness of 1 mm. Coagulation is inhibited by extracorporeal addition of heparin or sodium citrate with only little and transient influence on coagulation in the patient.11
Although the machine has undergone various technical improvements and is now in its 4th generation the basic procedure as described in Fig. 1 has remained largely unchanged. One major improvement was the introduction of the parenteral application of 8-MOP, which was originally given by mouth prior to ECP as in PUVA. The disadvantages of oral 8-MOP include gastrointestinal intolerance, unreliable blood levels, and the need for photoprotection to avoid phototoxicity. In 1993 the first report appeared describing the use of a liquid formulation that can be applied directly to the isolated leukocyte fraction prior to UVA exposure.12 Extracorporeal application of 8-MOP has now generally replaced the oral route with the advantage of minimal systemic exposure to the drug, eliminating the need for photoprotection, lack of nausea, and predictable 8-MOP concentrations during UVA exposure. Photopheresis received approval by the U.S. Food and Drug Administration in 1987 supplemented in 2000 with the approval of a sterile liquid formulation of 8-MOP for extracorporeal use (UVADEX®).
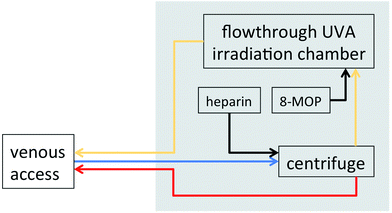 |
| Fig. 1 Schematic representation of the photopheresis procedure. Blood is removed from a peripheral venous access (typically in the cubital vein), an anticoagulant (typically heparin, sometimes sodium citrate) is added, white blood cells are separated through centrifugation, and red blood cells are returned through the venous line. 8-MOP is added to the white cell fraction prior to exposure to UVA (1–2 J cm−2). After extracorporeal photochemotherapy cells are returned to the patient. Blue: venous blood; red: red blood cell fraction; yellow: white blood cell fraction (“buffy coat”); black: pharmaceuticals. | |
The standard treatment schedule for ECP has basically remained unchanged since its invention and usually involves treatment on 2 successive days, by which 10–20% of white blood cells are exposed to 8-MOP and UVA. Treatments are repeated at 2–4-week intervals for as long as is clinically indicated. In responding patients, treatment intervals may be gradually lengthened for maintenance.
Various lines of research have tried to elucidate the mechanisms behind the observed clinical effects. Prominent among these is the above mentioned concept of T cell vaccination that has been consequently followed and investigated by Edelson and his group. Eventually these researchers provided evidence for a hypothesis that includes the rapid and synchronized generation of large numbers of what they call ECP-induced dendritic cells (EI-DCs) from monocytes during the ECP procedure.13 These cells take up antigens from intermixed T cells as they undergo PUVA-induced apoptosis and present them to the immune system in a way that leads to the desired effect depending on the state of T cell activation and the state of EI-DC maturation. Through further research into the specific features of these cells the seeming paradox of ECP, namely that it can be used to treat a T cell malignancy on the one hand (where a targeted immune response would be desired) and autoimmune-mediated inflammatory conditions on the other hand (where specific inhibition of a pathological immune response should be achieved), could be resolved.14
Another recent line of research is based on the observation in a murine model of contact hypersensitivity that apoptotic, antigen specific T cells induced by experimental ECP lead to the induction of specific regulatory T cells (T-reg) that mediate specific immunological tolerance.15 Transfer of these ECP-induced T-reg to syngeneic mice induces tolerance in the recipients. In addition, the production of anti-inflammatory cytokines upon presentation of apoptotic cells to APCs has been demonstrated, contributing further to the clinically observed immunomodulation.16 The importance of T-reg in ECP has been confirmed also in experimental GVHD,17 a murine model of organ transplantation,18 and recently also in SSc.19
Although these models are intriguing and – since they are not mutually exclusive – might well both be at work at the same time, other aspects of ECP have been largely neglected. Among these is the photochemistry of blood plasma occurring in the UV exposure chamber. Cellular components are suspended in diluted autologous plasma during UVA exposure. Since human plasma contains UVA absorbing molecules and probably also substances that can bind to 8-MOP photochemical reactions are likely to occur as has been shown with the example of folic acid that is degraded during ECP.20 Another neglected field is the role of cells other than lymphocytes and monocytes that are present in the treatment chamber in high numbers. These include neutrophils and platelets, both known to be highly reactive and able to quickly respond to various external triggers. Although early research has shown that the respiratory burst capability of neutrophils is inhibited by ECP this observation has not been followed up and the role of plasma and non-lymphoid cellular components in ECP remains to be investigated.21
Another basic question in ECP with initial evidence emerging not earlier than over 20 years after the first clinical report appeared is the fate of the irradiated cells. We have recently shown that these cells can be reliably labelled extracorporeally with indium-111 oxine and thus followed after reinfusion by whole body scintigraphy.22 Application of this technique to specific clinical conditions will probably help elucidate the target organs to which exposed cells migrate and where therapeutically relevant immunological reactions might occur.
Clinical applications
Cutaneous T cell lymphomas
According to the concept of T cell vaccination described above the clinical development of ECP focussed on the treatment of conditions where T cells were thought to play an essential pathogenic role. In the 1980s it was assumed that CTCL is a unifying disease entity encompassing T cell non-Hodgkin lymphomas that present in the skin. Among these lymphomas Sezary syndrome (SS) seemed particularly suitable for treatment with ECP since it is characterized by a high burden of circulatory malignant T lymphocytes in the peripheral blood. To date the classification of cutaneous lymphomas has progressed and CTCL is not regarded as a separate entity but rather as a group of heterogeneous diseases, among them MF and SS.23 The first clinical study of ECP was published in 1987 and reported results in the treatment of 37 patients with what the authors then called resistant CTCL.24 Clinical response was seen in 27 patients with an average decrease in cutaneous involvement of 64%. This response was reached after 22 ± 10 weeks (mean ± SD). Notably the treatment was shown to be almost free of adverse events. As expected nausea related to 8-MOP was observed (and later eliminated with the introduction of the extracorporeally added liquid formulation). Other side-effects included rare cases of transient hypotension and discomfort and complications due to venipuncture.
Since then, ECP has been studied in a number of uncontrolled trials summarized in 2003 by Zic.25 At that time treatment of MF and SS with ECP had been reported in over 400 patients with an overall response rate of 55.7%, a complete remission rate of 17.6% and almost absent toxicity. Notably only in 5 of the 19 trials and case series analysed by Zic ECP was used as a single treatment modality. In many patients ECP was combined with other treatments, either from the outset or after treatment for 6–8 months had resulted in insufficient response. Although from these and other data a conclusion as to the superiority of any combination over the other or over single therapy cannot be made it is evident that ECP can be safely combined with other therapies, including PUVA, radiotherapy, interferons, and retinoids. A typical clinical case is shown in Fig. 2.
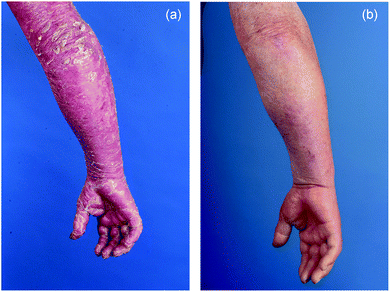 |
| Fig. 2 Right arm of a patient with SS (a) before ECP showing diffuse redness and severe scaling and (b) after 5 years of continuous treatment. | |
Some efforts have been made to identify in advance patients who will respond best to ECP. The lack of prospective randomized trials, however, makes it difficult to define reliable prognostic parameters. The following clinical and laboratory parameters are discussed among experts to best define a patient's positive response to ECP: a disease course of under 2 years, the presence of Sezary cells in the bloodstream, a normal number of CD8+ T cells, and lack of the following: bulky lymphadenopathy, other organ involvement, high leukocytosis, previous chemotherapy, plaque stage with extensive skin involvement. Evidence for the value of these parameters, however, is anecdotal and awaits scientific confirmation.26
In summary, based on the available data, recent consensus reports on the treatment of MF and SS have recommended ECP as a first-line treatment for SS and for patients with erythroderma from MF.27–29 Emerging evidence indicates that ECP might also be effective in extracutaneous lymphomas, but this awaits confirmation.30
Systemic sclerosis
Systemic sclerosis (SSc) is characterised by inflammation, vasculopathy, and abnormal deposition of collagen in the connective tissues of the skin and visceral organs, such as the kidneys, heart, lungs, and gastrointestinal tract. The disease takes a chronically progressive course and results in stiffness of the skin and dysfunction of the involved organs. Accordingly, SSc is associated with substantial morbidity and increased mortality. SSc is thought to have an autoimmune pathogenesis making it – according to the above described T cell vaccination concept – a potential candidate for treatment with ECP.31
Evidence from small studies and case series indicated that ECP might have beneficial effects on SSc with improvement in various clinical surrogate parameters including collagen synthesis in the skin,32 dermal oedema,33 and skin elasticity.34 However, the influence of ECP on the progression of visceral organ involvement could not be consistently demonstrated in these uncontrolled trials.35,36
The first randomised, observer blinded, prospective trial of ECP in SSc compared ECP with D-penicillamine, a substance with purported antifibrotic activity, in 79 patients of recent disease onset.37 A skin score was used as the primary endpoint and 21 of 31 patients (68%) showed an improvement after 6 months of ECP compared with eight of 25 patients (32%) given D-penicillamine. Another randomised, observer blinded study compared ECP with no treatment in a crossover design and could not show significant improvements in skin scores in 19 patients with SSc of less than 5 years’ duration.38 To exclude the influence of a possible placebo effect, a more recent multicenter, randomised study compared ECP and a sham procedure in a double-blind fashion in 64 patients with SSc of recent onset.39 Comparison of skin scores between the two study arms did not result in a statistical significance difference. However, after treatment for 6 and 12 months skin scores were significantly improved when compared to baseline in patients on active treatment but remained unchanged in the control group. As described above for the treatment of CTCL no substantial toxicity of ECP was observed.
In summary, in the absence of other effective treatment options for the cutaneous involvement of SSc ECP can be safely employed either alone or in combination with other therapies currently used in the treatment of SSc including prostaglandins, endothelin receptor antagonists, phosphodiesterase-5-inhibitors, immunosuppressive agents, and others.
Graft-versus-host disease
In allogeneic haematopoietic stem cell transplantation (HSCT) multipotent stem cells from healthy donors are transferred to reconstitute the bone marrow of patients with mostly hematologic malignancies after conditioning with radiation and/or chemotherapy. Acute and chronic GVHD are a serious and frequent complication of HSCT and contribute substantially to post-transplantation morbidity and mortality. Similar to an autoimmune reaction an immunological response of the grafted lymphoid cells against healthy tissue of the recipient is considered causal to GVHD.40 Thus, given the original concept of ECP as a means of T cell vaccination, its effect was investigated in clinical trials, particularly since established treatments consist of corticosteroids and other immunosuppressive agents with their inherently related toxicity.
An early pilot study included 21 patients with steroid-refractory acute GVHD. 60% of patients achieved a complete resolution of GVHD symptoms within 3 months of therapy.41 The subsequent Phase II study included 59 patients again with steroid-refractory acute GVHD.42 In this study an intensified ECP schedule was used, consisting of weekly 2-day treatments. After a median treatment period of 1.3 months and a median number of four treatment cycles 82% of patients with cutaneous involvement, 61% with liver involvement and 61% with gut involvement achieved complete remission. The intensified treatment was not associated with an increased rate of adverse events. Overall survival at 4 years was significantly increased in patients achieving CR compared with those who did not. These and other results indicate that ECP might be highly effective in acute GVHD inducing durable long term remissions in a high percentage of patients.43
In contrast to acute GVHD, the chronic form of the disease usually occurs later than 100 days after transplantation. The disease takes a chronic course and in some clinical aspects resembles SSc. Results of small studies and case series indicate that ECP might have beneficial effects in patients with chronic GVHD.44–46 A larger retrospective study of 71 patients reported an overall response rate to ECP of 61%, with 20% of patients showing CRs.47 More recently the efficacy of ECP in chronic GVHD was investigated in a randomized prospective trial.48 In this Phase II study 95 patients received either conventional treatment or conventional treatment combined with ECP. A skin score was used as the primary endpoint and although there was no statistical difference in median percent change from baseline between the ECP and the control arm some of the secondary endpoints showed encouraging results (including a steroid-sparing effect and enhanced quality of life) with again absent toxicity.
In summary, ECP has objective activity in the treatment of acute GVHD and has shown some promise in chronic GVHD.49
Solid organ transplant rejection
Since the first human kidney transplant in 1950 and the first human heart transplant in 1967 transplantation medicine has advanced substantially and solid organ transplants have become routine in specialized centers world-wide. Although improved immunosuppressive regimens have improved long term graft survival, adverse effects of immunosuppressants and graft rejection are still medical challenges. As with the conditions discussed above ECP might again be able to interfere with the immunological response of the host against an allogeneic donor organ. Early studies in heart transplantation indicated that ECP combined with conventional treatment could reduce the incidence of rejection episodes and support conventional treatment in patients who have already developed rejection.50–54 In addition, ECP might help to reduce exposure to immunosuppressants and thus contribute to a reduction of therapy-related toxicity.52,53 A small prospective pilot study in 23 patients undergoing heart transplants compared conventional treatment alone with conventional treatment combined with ECP and found that ECP is capable of decreasing post-transplant graft intimal hyperplasia, an indicator of chronic rejection.55 In a subsequent larger study with a similar protocol, 60 patients were included.56 Patients in the ECP group experienced a significant reduction in the number of acute rejection episodes and the number of patients with one or no rejection episode was significantly higher in the photopheresis group. The authors concluded that the addition of photopheresis to triple-drug immunosuppressive therapy significantly decreased the risk of cardiac rejection without increasing the incidence of infection. A more recent retrospective single-center analysis showed that ECP can reduce the risk of rejection and/or death from rejection after heart transplant when initiated for patients with high rejection risks.57
Only limited experience exists with ECP in the treatment of rejection reactions after lung, liver, and kidney transplantation.58
Taken together ECP has shown promise in the management of rejection across the spectrum of solid-organ transplants. Further studies will be necessary to define the optimal target population, time-points for initiation of treatment, and treatment schedules.
Other conditions
Crohn's disease, atopic dermatitis, lupus erythematodes, oral lichen planus, pemphigus vulgaris and diabetes mellitus type I are among the list of other conditions where ECP has been tried with at least some success. Results have been described recently elsewhere and are not covered in this review.59–61
Summary
Among the various forms of phototherapy ECP is unique in its basic principle, namely the reinfusion of extracorporeally modified blood components after exposure to photochemotherapy, as well as in its broad clinical application in malignant and inflammatory systemic diseases. The fact that the available clinical evidence for most of its applications is moderate is mainly due to the chronic and slowly progressive nature of most of its indications making prospective research with meaningful endpoints difficult. A remarkable exception to this is acute GVHD, an aggressive disease with often fatal outcome, where the clinical efficacy of ECP has been shown in randomised prospective trials. Thus, ECP remains fascinating for both the clinician and the scientist, allowing at the same time the exploration of photoimmunology, photomedicine, and their impact on the pathophysiology and management of rare and difficult to treat diseases.
References
- R. L. Edelson, Photopheresis: a new therapeutic concept, Yale J. Biol. Med., 1989, 62, 565–577 Search PubMed.
- R. Knobler, M. L. Barr, D. R. Couriel, J. L. M. Ferrara, L. E. French, P. Jaksch, W. Reinisch, A. H. Rook, T. Schwarz and H. Greinix, Extracorporeal photopheresis: past, present, and future, J. Am. Acad. Dermatol., 2009, 61, 652–665 CrossRef.
- P. Szodoray, G. Papp, B. Nakken, M. Harangi and M. Zeher, The molecular and clinical rationale of extracorporeal photochemotherapy in autoimmune diseases, malignancies and transplantation, Autoimmun. Rev., 2012, 9, 459–464.
- H. Hönigsmann, History of phototherapy in dermatology, Photochem. Photobiol. Sci., 2012 10.1039/C2PP25120E.
- F. Trautinger, Phototherapy of mycosis fungoides, Photodermatol. Photoimmunol. Photomed., 2011, 27, 68–74 CrossRef.
- A. Ben-Nun, H. Wekerle and I. R. Cohen, Vaccination against autoimmune encephalomyelitis with T lymphocyte line cells reactive against myelin basic protein, Nature, 1981, 292, 60–61 CrossRef CAS.
- O. Lider, T. Reshef, E. Beraud, A. Ben-Nun and I. R. Cohen, Anti-idiotypic network induced by T cell vaccination against experimental autoimmune encephalomyelitis, Science, 1988, 239, 181–183 CrossRef CAS.
- P. A. Khavari, R. L. Edelson, O. Lider, F. Gasparro, H. L. Weiner and I. R. Cohen, Specific vaccination against photoinactivated cloned T cells (abstract), Clin. Res., 1988, 36, 662A Search PubMed.
- M. Perez, R. Edelson, L. Laroche and C. Berger, Inhibition of antiskin allograft immunity by infusions with syngeneic photoinactivated effector lymphocytes, J. Invest. Dermatol., 1989, 92, 669–676 CrossRef CAS.
- C. L. Berger, M. Perez, L. Laroche and R. Edelson, Inhibition of autoimmune disease in a murine model of systemic lupus erythematodes induced by exposure to syngeneic photoactivated lymphocytes, J. Invest. Dermatol., 1990, 94, 52–57 CrossRef CAS.
- E. Ivancic, R. Knobler, P. Quehenberger, H. Hönigsmann and F. Trautinger, The course of anticoagulation after extracorporeal photochemotherapy, Photodermatol. Photoimmunol. Photomed., 2005, 21, 150–151 CrossRef CAS.
- R. M. Knobler, F. Trautinger, W. Graninger, W. Macheiner, C. Grünwald, R. Neumann and W. Ramer, Parenteral administration of 8-methoxypsoralen in photopheresis, J. Am. Acad. Dermatol., 1993, 28, 580–584 CrossRef CAS.
- C. Berger, K. Hoffmann, J. G. Vasquez, S. Mane, J. Lewis, R. Filler, A. Lin, H. Zhao, T. Durazzo, A. Baird, W. Lin, F. Foss, I. Christensen, M. Girardi, R. Tigelaar and R. Edelson, Rapid generation of maturationally synchronized human dendritic cells: contribution to the clinical efficacy of extracorporeal photochemotherapy, Blood, 2010, 116, 4838–4847 CrossRef CAS.
- M. Girardi, J. Schechner, E. Glusac, C. Berger and R. Edelson, Transimmunization and the evolution of extracorporeal photochemotherapy, Transfus. Apher. Sci., 2002, 26, 181–190 CrossRef.
- A. Maeda, A. Schwarz, K. Kernebeck, N. Gross, Y. Aragane, D. Peritt and T. Schwarz, Intravenous infusion of syngeneic apoptotic cells by photopheresis induces antigen-specific regulatory T cells, J. Immunol., 2005, 174, 5968–5976 CAS.
- M. Di Renzo, P. Rubegni, G. De Aloe, L. Paulesu, A. L. Pasqui, L. Andreassi, A. Auteri and M. Fimiani, Extracorporeal photochemotherapy restores Th1/Th2 imbalance in patients with early stage cutaneous T-cell lymphoma, Immunology, 1997, 92, 99–103 CrossRef CAS.
- E. Gatza, C. E. Rogers, S. G. Clouthier, K. P. Lowler, I. Tawara, C. Liu, P. Reddy and J. L. M. Ferrara, Extracorporeal photopheresis reverses experimental graft-versus-host disease through regulatory T cells, Blood, 2008, 112, 1515–1521 CrossRef CAS.
- J. F. George, C. W. Gooden, L. Guo and J. K. Kirklin, Role for CD4(+)CD25(+) T cells in inhibition of graft rejection by extracorporeal photopheresis, J. Heart Lung Transplant., 2008, 27, 616–622 CrossRef.
- G. Papp, I. F. Horvath, S. Barath, E. Gyimesi, J. Vegh, P. Szodoray and M. Zeher, Immunomodulatory effects of extracorporeal photochemotherapy in systemic sclerosis, Clin. Immunol., 2012, 142, 150–159 CrossRef CAS.
- M. Der-Petrossian, M. Födinger, R. Knobler, H. Hönigsmann and F. Trautinger, Photodegradation of folic acid during extracorporeal photopheresis, Br. J. Dermatol., 2007, 156, 117–121 CrossRef CAS.
- F. Trautinger, R. M. Knobler, W. Macheiner, C. Grünwald and M. Micksche, Release of oxygen-free radicals by neutrophils is reduced by photopheresis, Ann. N. Y. Acad. Sci., 1991, 636, 383–385 CrossRef CAS.
- U. Just, R. Knobler, G. Klosner, E. Ivancic-Brandenberger, H. Greinix, A. Becherer and F. Trautinger, Leukocyte scintigraphy with 111In-oxine for assessment of cell trafficking after extracorporeal photopheresis, Exp. Dermatol., 2012, 21, 443–447 CrossRef CAS.
- R. Willemze, E. S. Jaffe, G. Burg, L. Cerroni, E. Berti, S. H. Swerdlow, E. Ralfkiaer, S. Chimenti, J. L. Diaz-Perez, L. M. Duncan, F. Grange, N. L. Harris, W. Kempf, H. Kerl, M. Kurrer, R. Knobler, N. Pimpinelli, C. Sander, M. Santucci, W. Sterry, M. H. Vermeer, J. Wechsler, S. Whittaker and C. J. L. M. Meijer, WHO-EORTC classification for cutaneous lymphomas, Blood, 2005, 105, 3768–3785 CrossRef CAS.
- R. Edelson, C. Berger, F. Gasparro, B. Jegasothy, P. Heald, B. Wintroub, E. Vonderheid, R. Knobler, K. Wolff and G. Plewig,
et al., Treatment of cutaneous T-cell lymphoma by extracorporeal photochemotherapy. Preliminary results, N. Engl. J. Med., 1987, 316, 297–303 CAS.
- J. A. Zic, The treatment of cutaneous T-cell lymphoma with photopheresis, Dermatol. Ther., 2003, 16, 337–346 CrossRef.
- R. Knobler and C. Jantschitsch, Extracorporeal photochemoimmunotherapy in cutaneous T-cell lymphoma, Transfus. Apher. Sci., 2003, 28, 81–89 CrossRef CAS.
- F. Trautinger, R. Knobler, R. Willemze, K. Peris, R. Stadler, L. Laroche, M. D'Incan, A. Ranki, N. Pimpinelli, P. Ortiz-Romero, R. Dummer, T. Estrach and S. Whittaker, EORTC consensus recommendations for the treatment of mycosis fungoides/Sezary syndrome, Eur. J. Cancer, 2006, 42, 1014–1030 CrossRef.
-
J. J. Scarisbrick, P. Taylor, U. Holtick, Y. Makar, K. Douglas, G. Berlin, E. Juvonen, S. Marshall and G. Photopheresis Expert, U.K. consensus statement on the use of extracorporeal photopheresis for treatment of cutaneous T-cell lymphoma and chronic graft-versus-host disease, Br. J. Dermatol., 2008, 158, 659–678 Search PubMed.
- J. A. Zic, Photopheresis in the treatment of cutaneous T-cell lymphoma: current status, Curr. Opin. Oncol., 2012, 24(Suppl 1), S1–S10 CrossRef CAS.
- F. Garban, S. Carras, P. Drillat, M. C. Jacob, B. Fabre, M. Callanan, S. Courby, C. Makowski, J. Y. Cahn and R. Gressin, Extracorporeal photopheresis as a curative treatment strategy in non epidermotropic T-cell lymphoma and large granular lymphocyte leukemia, Ann. Oncol., 2012 DOI:10.1093/annonc/mds014.
- C. Charles, P. Clements and D. E. Furst, Systemic sclerosis: hypothesis-driven treatment strategies, Lancet, 2006, 367, 1683–1691 CrossRef.
- T. Ohtsuka, H. Okita, A. Yamakage and S. Yamazaki, The effect of extracorporeal photochemotherapy on alpha1(I) and alpha1(III) procollagen mRNA expression in systemic sclerosis skin tissue, Arch. Dermatol. Res., 2002, 293, 642–645 CrossRef.
- M. Hashikabe, T. Ohtsuka and S. Yamazaki, Quantitative echographic analysis of photochemotherapy on systemic sclerosis skin, Arch. Dermatol. Res., 2005, 296, 522–527 CrossRef CAS.
- M. Fimiani, P. Rubegni, M. L. Flori, C. Mazzatenta, G. D'Ascenzo and L. Andreassi, Three cases of progressive systemic sclerosis treated with extracorporeal photochemotherapy, Arch. Dermatol. Res., 1997, 289, 120–122 CrossRef CAS.
- K. Krasagakis, E. Dippel, J. Ramaker, M. Owsianowski and C. E. Orfanos, Management of severe scleroderma with long-term extracorporeal photopheresis, Dermatol., 1998, 196, 309–315 Search PubMed.
- R. R. Muellegger, A. Hofer, W. Salmhofer, H. P. Soyer, H. Kerl and P. Wolf, Extended extracorporeal photochemotherapy with extracorporeal administration of 8-methoxypsoralen in systemic sclerosis. An Austrian single-center study, Photodermatol. Photoimmunol. Photomed., 2000, 16, 216–223 CrossRef CAS.
- A. H. Rook, B. Freundlich, B. V. Jegasothy, M. I. Perez, W. G. Barr, S. A. Jimenez, R. L. Rietschel, B. Wintroub, M. B. Kahaleh and J. Varga,
et al., Treatment of systemic sclerosis with extracorporeal photochemotherapy. Results of a multicenter trial, Arch. Dermatol., 1992, 128, 337–346 CAS.
- D. N. Enomoto, J. R. Mekkes, P. M. Bossuyt, S. L. Yong, T. A. Out, R. Hoekzema, M. A. de Rie, P. T. Schellekens, I. J. ten Berge, C. A. de Borgie and J. D. Bos, Treatment of patients with systemic sclerosis with extracorporeal photochemotherapy (photopheresis), J. Am. Acad. Dermatol., 1999, 41, 9159–9122.
-
R. M. Knobler, L. E. French, Y. Kim, E. Bisaccia, W. Graninger, H. Nahavandi, F. J. Strobl, E. Keystone, M. Mehlmauer, A. H. Rook, I. Braverman and G. Systemic Sclerosis Study, A randomized, double-blind, placebo-controlled trial of photopheresis in systemic sclerosis, J. Am. Acad. Dermatol., 2006, 54, 793–799 Search PubMed.
- J. L. M. Ferrara, J. E. Levine, P. Reddy and E. Holler, Graft-versus-host disease, Lancet, 2009, 373, 1550–1561 CrossRef CAS.
- H. T. Greinix, B. Volc-Platzer, P. Kalhs, G. Fischer, A. Rosenmayr, F. Keil, H. Hönigsmann and R. M. Knobler, Extracorporeal photochemotherapy in the treatment of severe steroid-refractory acute graft-versus-host disease: a pilot study, Blood, 2000, 96, 2426–2431 CAS.
- H. T. Greinix, R. M. Knobler, N. Worel, B. Schneider, A. Schneeberger, P. Hoecker, M. Mitterbauer, W. Rabitsch, A. Schulenburg and P. Kalhs, The effect of intensified extracorporeal photochemotherapy on long-term survival in patients with severe acute graft-versus-host disease, Haematologica, 2006, 91, 405–408.
- E. Calore, A. Calo, G. Tridello, S. Cesaro, M. Pillon, S. Varotto, M. V. Gazzola, R. Destro, P. Marson, L. Trentin, M. Carli and C. Messina, Extracorporeal photochemotherapy may improve outcome in children with acute GVHD, Bone Marrow Transplant., 2008, 42, 421–425 CrossRef CAS.
- H. T. Greinix, B. Volc-Platzer, W. Rabitsch, B. Gmeinhart, C. Guevara-Pineda, P. Kahls, J. Krutmann, H. Hönigsmann, M. Ciovica and R. M. Knobler, Successful use of extracorporeal photochemotherapy in the treatment of severe acute and chronic graft-versus-host disease, Blood, 1998, 92, 3098–3104 CAS.
- F. M. Foss, G. M. DiVenuti, K. Chin, K. Sprague, H. Grodman, A. Klein, G. Chan, K. Stiffler and K. B. Miller, Prospective study of extracorporeal photopheresis in steroid-refractory or steroid-resistant extensive chronic graft-versus-host disease: analysis of response and survival incorporating prognostic factors, Bone Marrow Transplant., 2005, 35, 1187–1193 CrossRef CAS.
- N. Apisarnthanarax, M. Donato, M. Korbling, D. Couriel, J. Gajewski, S. Giralt, I. Khouri, C. Hosing, R. Champlin, M. Duvic and P. Anderlini, Extracorporeal photopheresis therapy in the management of steroid-refractory or steroid-dependent cutaneous chronic graft-versus-host disease after allogeneic stem cell transplantation: feasibility and results, Bone Marrow Transplant., 2003, 31, 459–465 CrossRef CAS.
- D. R. Couriel, C. Hosing, R. Saliba, E. J. Shpall, P. Anderlini, B. Rhodes, V. Smith, I. Khouri, S. Giralt, M. de Lima, Y. Hsu, S. Ghosh, J. Neumann, B. Andersson, M. Qazilbash, S. Hymes, S. Kim, R. Champlin and M. Donato, Extracorporeal photochemotherapy for the treatment of steroid-resistant chronic GVHD, Blood, 2006, 107, 3074–3080 CrossRef CAS.
- M. E. D. Flowers, J. F. Apperley, K. van Besien, A. Elmaagacli, A. Grigg, V. Reddy, A. Bacigalupo, H.-J. Kolb, L. Bouzas, M. Michallet, H. M. Prince, R. Knobler, D. Parenti, J. Gallo and H. T. Greinix, A multicenter prospective phase 2 randomized study of extracorporeal photopheresis for treatment of chronic graft-versus-host disease, Blood, 2009, 112, 2667–2674.
- D. Wolff, M. Schleuning, S. von Harsdorf, U. Bacher, A. Gerbitz, M. Stadler, F. Ayuk, A. Kiani, R. Schwerdtfeger, G. B. Vogelsang, G. Kobbe, M. Gramatzki, A. Lawitschka, M. Mohty, S. Z. Pavletic, H. Greinix and E. Holler, Consensus conference on clinical practice in chronic GVHD: second-line treatment of chronic graft-versus-host disease, Biol. Blood Marrow Transplant., 2011, 17, 1–17 CrossRef.
- E. A. Rose, M. L. Barr, H. Xu, P. Pepino, M. P. Murphy, M. A. McGovern, A. J. Ratner, J. F. Watkins, C. C. Marboe and C. L. Berger, Photochemotherapy in human heart transplant recipients at high risk for fatal rejection, J. Heart Lung Transplant., 1992, 11, 746–750 CAS.
- M. Wieland, V. L. Thiede, R. G. Strauss, W. W. Piette, D. P. Kapelanski, S. K. Landas, L. G. Hunsicker, S. J. Vance and M. J. Randels, Treatment of severe cardiac allograft rejection with extracorporeal photochemotherapy, J. Clin. Apheresis, 1994, 9, 171–175 CrossRef CAS.
- R. Dall'Amico, U. Livi, A. Milano, G. Montini, B. Andreetta, L. Murer, G. Zacchello, G. Thiene, D. Casarotto and F. Zacchello, Extracorporeal photochemotherapy as adjuvant treatment of heart transplant recipients with recurrent rejection, Transplantation, 1995, 60, 454–459.
- R. Dall'Amico, G. Montini, L. Murer, B. Andreetta, G. Zacchello, A. Gambino, G. Feltrin, A. Caforio, V. Tursi and U. Livi, Extracorporeal photochemotherapy after cardiac transplantation: a new therapeutic approach to allograft rejection, Int. J. Artif. Organs, 2000, 23, 49–54 Search PubMed.
- M. S. Lehrer, A. H. Rook, J. E. Tomaszewski and D. DeNofrio, Successful reversal of severe refractory cardiac allograft rejection by photopheresis, J. Heart Lung Transplant., 2001, 20, 1233–1236 CrossRef CAS.
- M. L. Barr, C. J. Baker, F. A. Schenkel, S. N. McLaughlin, B. C. Stouch, V. A. Starnes and E. A. Rose, Prophylactic photopheresis and chronic rejection: effects on graft intimal hyperplasia in cardiac transplantation, Clin. Transplant., 2000, 14, 162–166 Search PubMed.
- M. L. Barr, B. M. Meiser, H. J. Eisen, R. F. Roberts, U. Livi, R. Dall'Amico, R. Dorent, J. G. Rogers, B. Radovancevic, D. O. Taylor, V. Jeevanandam and C. C. Marboe, Photopheresis for the prevention of rejection in cardiac transplantation. Photopheresis Transplantation Study Group, N. Engl. J. Med., 1998, 339, 1744–1751 CrossRef CAS.
- J. K. Kirklin, R. N. Brown, S. T. Huang, D. C. Naftel, S. M. Hubbard, B. K. Rayburn, D. C. McGiffin, R. B. Bourge, R. L. Benza, J. A. Tallaj, L. J. Pinderski, S. V. Pamboukian, J. F. George and M. Marques, Rejection with hemodynamic compromise: objective evidence for efficacy of photopheresis, J. Heart Lung Transplant., 2006, 25, 283–288 CrossRef CAS.
- M. B. Marques and H. H. Tuncer, Photopheresis in solid organ transplant rejection, J. Clin. Apheresis, 2006, 21, 72–77 CrossRef.
- T. Dani and R. Knobler, Extracorporeal photoimmunotherapy-photopheresis, Front. Biosci., 2009, 14, 4769–4777 CrossRef CAS.
- D. M. Ward, Extracorporeal photopheresis: how, when, and why, J. Clin. Apheresis, 2011, 26, 276–285 CrossRef.
- P. Wolf, D. Georgas, N. S. Tomi, C. M. Schempp, A. Bretterklieber and K. Hoffmann, Photochem. Photobiol. Sci. 10.1039/C2PP25203A , in press.
Footnote |
† This article is published as part of a themed issue on current topics in photodermatology. |
|
This journal is © The Royal Society of Chemistry and Owner Societies 2013 |