DOI:
10.1039/C3NJ00940H
(Paper)
New J. Chem., 2014,
38, 348-357
Efficient synthesis of novel spiro-furo-pyrido-pyrimidine-indolines by manganese ferrite nanoparticles as a highly active magnetically reusable nanocatalyst in water†
Received
(in Montpellier, France)
14th August 2013
, Accepted 16th October 2013
First published on 17th October 2013
Abstract
A one-pot and three-component reaction for the efficient, green and economical synthesis of novel spiro-furo-pyrido-pyrimidine-indolines using 2,6-diaminopyrimidine-4(3H)-one or uraciles, isatins and tetronic acid or anilinolactones in the presence of manganese ferrite nanoparticles as a magnetic catalyst in water is described. The present synthesis shows attractive characteristics such as; the use of magnetically recoverable and reusable catalyst, convenient one-pot operation, short reaction periods, good to high yields and the use of water as a green reaction medium and is considered to be relatively environmentally benign.
Introduction
The conventional multi-step preparation of complex molecules generally involves a large number of synthetic operations, including extraction and purification processes in each individual step. This leads to not only synthetic inefficiency but also generates large amounts of waste. Multicomponent reactions (MCRs) are special types of synthetically useful organic reactions in which three or more different starting materials come together in a single reaction vessel to give a final product containing substantial elements of all the reactants.1–3 MCRs allow the formation of several bonds in a single operation and offer remarkable advantages such as, operational simplicity, a reduction in the number of workup and extraction and purification processes, a high degree of atom economy and they are also less time consuming4,5 The design of new MCRs with green and eco-friendly procedures has attracted great attention, especially in areas such as drug discovery, organic synthesis, and material science.6 Therefore, development of novel, efficient, and green MCRs focused on a target product is one of the major challenges in organic synthesis. The use of water as a green solvent for organic synthesis has recently attracted considerable attention. Organic reactions carried out in water, without the use of any hazardous and flammable organic solvents, are one of the current challenges especially in our environmentally conscious society and, in recent years, studies have been focused on finding new water-compatible and reusable catalysts.7–9
Magnetic nanoparticles have gained considerable interest in various disciplines such as Ferro fluids,10 drug delivery,11 magnetic resonance imaging (MRI),12 cancer hyperthermia treatment,13 biomolecular sensors,14 bioseparation,15 and have emerged as a useful group of heterogeneous catalysts because of their extremely small size and large surface to volume ratio and the fact that they can be separated from the reaction medium after magnetization by an external magnet.16–18 Magnetic nanoparticles possess significant advantages such as, remarkable catalytic activity, easy synthesis, operational simplicity, eco-friendliness, and recoverability with an external magnetic field. Accordingly, magnetic separation could be regarded as an attractive alternative to filtration or centrifugation as it prevents the loss of catalyst and enhances its reusability.19,20 These advantages encouraged us to utilize manganese ferrite nanoparticles as a recoverable and reusable catalyst for the synthesis of novel spirooxindole derivatives. The spirooxindole unit is a privileged heterocyclic motif that forms the core of a large family of alkaloids and natural products,21 with interesting structural features and a wide range of useful pharmacological and biological properties, such as antimicrobial22,23 and antitumoral24 activities; the spirooxindole unit also has the capacity to inhibit human NK-1 receptor function,25 and microtubule assembly.26 For instance, Spirotryprostatin A, a well-known spirooxindole unit, has anti-mitotic properties and Pteropodine has been shown to modulate the function of muscarinic serotonin receptors (Fig. 1).27,28 Due to their remarkable biological activity, in recent years, significant efforts have been devoted to find efficient, new and simple synthetic methods for the preparation of new substituted spirooxindoles.29–33
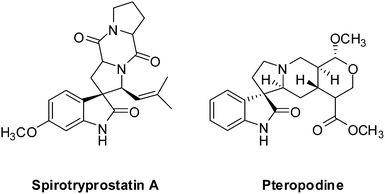 |
| Fig. 1 Representative spirooxindole-containing compounds. | |
Considering the highly prominent pharmacological activities of spirooxindoles and in continuation of our interest in the development of simple and efficient multi-component reactions for the preparation of heterocyclic compounds, especially new spirooxindoles,34–36 we report, herein, a facile, efficient, eco-friendly, and one-pot process for the synthesis of novel spiro-furo-pyrido-pyrimidine-indoline derivatives 4 through a three-component condensation reaction of isatins 1, tetronic acid 2 and 2,6-diaminopyrimidine-4(3H)-one or 6-aminopyrimidine-2,4(1H,3H)-dione and 6-amino-1,3-dimethylpyrimidine-2,4(1H,3H)-dione 3 in the presence of manganese ferrite nanoparticles as an efficient and reusable catalyst in water (Scheme 1).
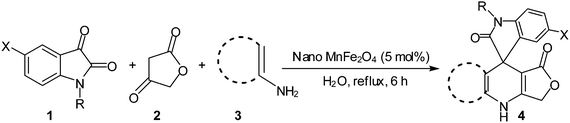 |
| Scheme 1 The reaction leading to the synthesis of novel spirooxindole derivatives 4a–l. | |
Results and discussion
Development of environmental friendly techniques is one of the priority goals of chemical research. In contrast to many organic solvents with disadvantages such as volatility and toxicity, water, as a unique solvent, is the most environmentally accepted, safest, and most abundant solvent. In addition, water generally enables facile work-up protocols, while most organic compounds, due to their lipophilic structure, are readily segregated from aqueous media. Furthermore, reactions performed in water can not only be faster and more selective than in conventional organic solvents but also exhibit unique reactivity and selectivity leading to novel assembly processes. In this study, we employed water in a three-component reaction as a green medium.
Initially, the three-component reaction of isatin 1a, tetronic acid 2, and 2,6-diaminopyrimidin-4(3H)-one 3a as a simple model substrate was investigated to establish the feasibility of the strategy and to optimize the reaction conditions (Scheme 2).
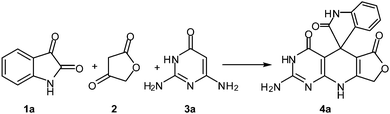 |
| Scheme 2 Screening of solvents and catalysts for the synthesis of 4a. | |
A catalyst plays a crucial role in the success of a reaction in terms of the reaction rate and yield. In the absence of any catalyst, the model reaction could be carried out but, as determined by TLC analysis, the obtained yield was too low after 48 h reflux in water. Therefore, we focused on finding a suitable catalyst to improve the yields and to optimize the reaction conditions. To find the best catalyst, the model reaction was performed in presence of different catalysts in refluxing water. The employed catalysts are listed in Table 1. These results indicated that, when manganese ferrite nanoparticles were used together with other Bronsted and Lewis acids the reaction was completed in a shorter time, affording an enhanced yield of 82% (Table 1, Entry 2). On one hand, among various acid catalysts, as shown in Table 1, spinel structures are more suitable than other metal oxides. The cause of this observation is the existence of more metal ions in MFe2O4 cluster structures that can act as Lewis acids in comparison with other metal oxides. On the other hand, it was shown that metal ferrites (MFe2O4) have a spinel structure of oxygen-packed, face-centered cubic lattices, with tetrahedral (Td) and octahedral holes (Oh), which can be occupied by magnetic atoms. These spinels have three kinds of structures: normal, inverse and a blend of both. Fe3O4 and CuFe2O4 have an inverse structure. In inverse spinel structures the Td sites are occupied by Fe3+ and the Oh sites are occupied by M2+ and Fe3+ atoms. However, when MnFe2O4 adopts a mixed spinel structure the Td sites are occupied by Mn1−x2+ Fex3+ (0 < x < 1) and the Oh sites are occupied by Mnx2+ Fe2−x3+. In the Td sites, metal ions have interactions with four O atoms, whereas in the Oh sites, metal ions have interactions with six O atoms. Due to the low steric hindrance in the Td sites in comparison with the Oh sites, these sites are Lewis acidic and interaction between the starting materials and Mn in the catalyst is more favourable than with other metal ions. So, it can be concluded that MnFe2O4 is much more effective than the others.37,38 The magnetic nature of the manganese ferrite nanoparticles facilitated their easy and quantitative removal from the reaction medium in the presence of an external magnetic field for further use.
Table 1 Various catalysts used for the synthesis of 2-amino-3H-spiro[furo[3,4-g]pyrido[2,3-d]pyrimidine-5,3′-indoline]-2′,4,6(8H,9H)-trione (4a)a
Entry |
Catalyst |
Time (h) |
Yieldb (%) |
Reaction conditions: isatin (1 mmol), tetronic acid (1 mmol), 2,6-diaminopyrimidin-4(3H)-one (1 mmol), H2O (5 mL), 90 °C, catalyst (10 mol%).
Isolated yields.
|
1 |
Nano CuFe2O4 |
1 |
74 |
2 |
Nano MnFe2O4 |
1 |
82 |
3 |
Nano Fe3O4 |
1 |
60 |
4 |
Nano ZnO |
5 |
>50 |
5 |
Nano MgO |
5 |
52 |
6 |
Nano TiO2 |
5 |
>50 |
7 |
Nano SnO2 |
5 |
53 |
8 |
P-TSA |
6 |
65 |
9 |
CH3COOH |
8 |
58 |
10 |
Silica sulfuric acid |
6 |
55 |
Characterization of the catalyst
The manganese ferrite nanoparticles were prepared by co-precipitation of MnCl2 and FeCl3 in basic solution at 95 °C using the previously reported procedure.39 The synthesized MnFe2O4 was characterized by XRD, TEM, and VSM. The position and relative intensities of all peaks were confirmed as well with the standard XRD pattern of MnFe2O4 (JCPDS card No. 75-0035), the manganese ferrite calcined at 800 °C presents a particle size of 33 nm, calculated from the broadening of the peak at 2θ = 35.31 using the Scherer equation (Fig. 2). The TEM image showed that the manganese ferrite nanoparticles have a mean diameter of about 25–30 nm. The magnetization curve for MnFe2O4 nanoparticles is shown in Fig. 3. It should be emphasized that a catalyst should possess sufficient magnetic and super paramagnetic properties for its practical application. Magnetic hysteresis measurements on MnFe2O4 were conducted in an applied magnetic field at room temperature, with the field sweeping from −10
000 to +10
000 oersted. As shown in Fig. 3, the hysteresis loop for the sample was completely reversible confirming its super paramagnetic nature. The catalyst showed high permeability in magnetization and high reversibility in the hysteresis loop.
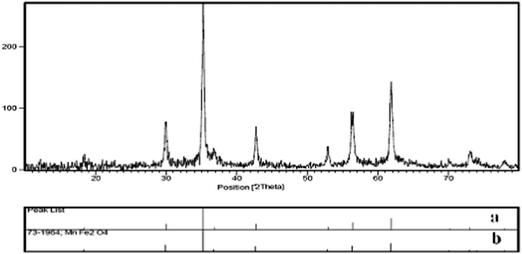 |
| Fig. 2 (a) The X-ray diffraction patterns of calcinated MnFe2O4. (b) The authentic X-ray diffraction patterns of MnFe2O4. | |
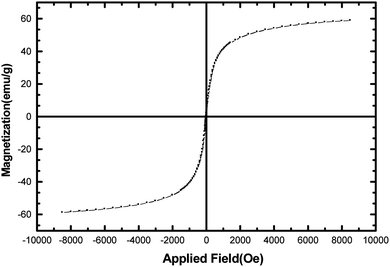 |
| Fig. 3 The vibrating sample magnetometer curve of synthesized MnFe2O4 nanoparticles at room temperature. | |
In order to optimize the reaction conditions, we also evaluated the amount of catalyst required for this transformation. It was found that when the reaction was carried out in the presence of 2 mol% catalyst the yield was 63%. As the mol amount (%) of the catalyst was increased, the yields also increased up to 83%, it was found that beyond 5 mol% there was no significant improvement of the rate or yield of the reaction and further increases in the quantity of catalyst did not show appreciable improvement in the yield of product. Thus, 5 mol% of catalyst was chosen as the maximum amount of catalyst for the reaction (Table 2).
Table 2 Different amounts of the MnFe2O4 nanoparticles as catalyst in model reactiona
Entry |
MnFe2O4 (mol%) |
Time (h) |
Yieldb (%) |
Reaction conditions: isatin (1 mmol), tetronic acid (1 mmol), 2,6-diaminopyrimidin-4(3H)-one (1 mmol), H2O (5 mL), 90 °C.
Isolated yields.
|
1 |
2 |
8 |
63 |
2 |
5 |
6 |
82 |
3 |
10 |
6 |
82 |
4 |
15 |
6 |
83 |
The recovery and reuse of catalysts is very important for a greener process. Finally, as shown in Table 3, the possibility of recycling the magnetic catalyst was studied by using isatin 1a, tetronic acid 2 and 2,6 diaminopyrimidin-4(3H)-one 3a, as model substrates. It is important to highlight that the catalyst could be magnetically recovered by an external magnetic field and washed with acetone to remove the residual product. After being dried, it was subjected to further reactions. The procedure was repeated and the results indicated that the recycled catalyst could be reused. The isolated yields remained similar with no detectable loss (Fig. 4). TEM analysis of the recovered nano MnFe2O4 revealed that the morphology and size of the nano particles remained relatively unchanged (Fig. 5).
Table 3 Synthesis of spiro-furo-pyrido-pyrimidine-indoline derivativesa
Entry |
1
|
3
|
Product |
Yieldb (%) |
Reaction conditions: 1a–e (1 mmol), 2 (1 mmol), 3a–c (1 mmol), MnFe2O4 (5 mol%), H2O (5 mL), 90 °C, reaction time (6 h).
Isolated yields.
|
1 |
|
|
|
82 |
2 |
|
|
|
80 |
3 |
|
|
|
77 |
4 |
|
|
|
79 |
5 |
|
|
|
76 |
6 |
|
|
|
83 |
7 |
|
|
|
78 |
8 |
|
|
|
75 |
9 |
|
|
|
76 |
10 |
|
|
|
72 |
11 |
|
|
|
74 |
12 |
|
|
|
75 |
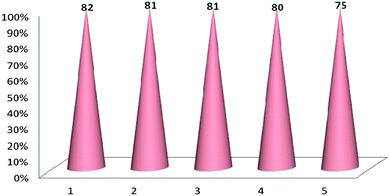 |
| Fig. 4 Catalyst recyclability study on the synthesis of 2-amino-3H-spiro[furo[3,4-g]pyrido[2,3-d]pyrimidine-5,3′-indoline]2′,4,6(8H,9H)-trione 4a. | |
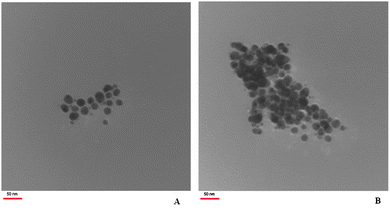 |
| Fig. 5 The TEM image of MnFe2O4 before the reaction (A) and the TEM image of MnFe2O4 after the reaction (B). | |
We have not established an exact mechanism for the formation of spirooxindoles 4a–l. However, two reasonable possibilities according to the literature40 are indicated in Scheme 3. (Pathway A) Addition of tetronic acid 2 to activated isatin 1a by nano MnFe2O4 led to highly reactive intermediate 5. This step was regarded as a fast Knoevenagel condensation reaction. Interception of 5 by 2,6-diaminopyrimidin-4(3H)-one 3a and the following intra molecular cyclization catalyzed by nano MnFe2O4 produces the corresponding spirooxindole 4a. (Pathway B) A Knoevenagel condensation reaction of activated isatin 1a by nano MnFe2O4 with 2,6-diaminopyrimidin-4(3H)-one 3b is proposed to afford highly reactive intermediate 6, followed by a nucleophilic addition with tetronic acid 2, finally intra molecular ring closure leading to the formation of 2-amino-3H-spiro[furo[3,4-g]pyrido[2,3-d]pyrimidine-5,3′-indoline]-2′,4,6(8H,9H)-trione 4a catalyzed by nano MnFe2O4.
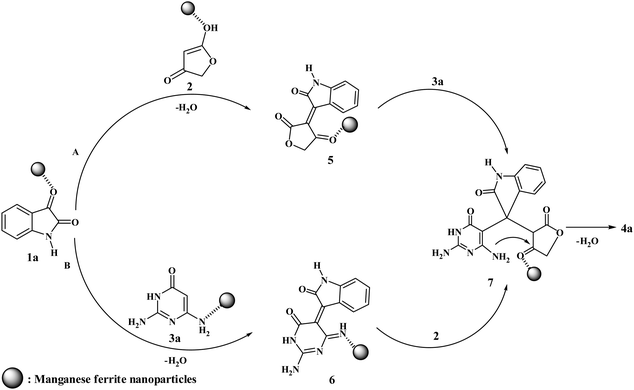 |
| Scheme 3 Proposed mechanism for the formation of 4a. | |
In an extension of the program to confirm the proposed mechanism, reactions using 6-aminopyrimidine-2,4(1H,3H)-dione 3b and 6-amino-1,3-dimethylpyrimidine-2,4(1H,3H)-dione 3c instead of 2,6-diaminopyrimidin-4(3H)-one 3a were carried out and the related spirocyclic compounds were obtained successfully under similar reaction conditions (Scheme 4).
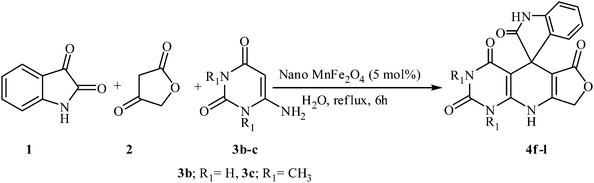 |
| Scheme 4 Synthesis of 1H-spiro[furo[3,4-g]pyrido[2,3-d]pyrimidine-5,3′-indoline]-2,2′,4,6(3H,8H,9H)-tetraones 4f–l. | |
Subsequently to verify the general procedure of the reaction, a variety of isatins 1a–e, with both electron-donating and electron-withdrawing groups were reacted with tetronic acid 2 and 2,6 diaminopyrimidine-4(3H)-one 3a or 6-aminopyrimidine-2,4(1H,3H)-dione 3b and 6-amino-1,3-dimethylpyrimidine-2,4(1H,3H)-dione 3c under appropriate reaction conditions (5 mL water, reflux, 5 mol% MnFe2O4 nanoparticles). The influence of electron-withdrawing and electron-donating substituents on the aromatic ring of isatin on the reaction yields was investigated. The results showed that in isatin, both electron-withdrawing and electron-donating substituents had no significant effect on the reaction yields and a series of new spirooxindole derivatives were synthesized. The results have been summarised in Table 3.
In order to evaluate the versatility of this procedure and in continuation of our efforts on the synthesis of new spirooxindoles, we performed the three-component reaction under the same reaction conditions, between isatins 1, 2,6-diaminopyrimidine-4(3H)-one or 6-aminopyrimidine-2,4(1H,3H)-dione and 6-amino-1,3-dimethylpyrimidine-2,4(1H,3H)-dione 3 and anilinolactones 8 instead of tetronic acid 2 in order to synthesize compounds 9. The anilinolactones were prepared from the condensation reaction of tetronic acid with various anilines, when tetronic acid was reacted with an equimolar amount of various anilines in 1,4-dioxane solvent at room temperature. The corresponding products were obtained in excellent yields, short reaction times, and high purity.41 In contrast to what we expected, the spectra of the products showed that the anticipated products were not produced and spiro-furo-pyrido-pyrimidine-indoline 4 was obtained again. By using anilinolactones instead of tetronic acid, the reaction time decreased to 3 h, on the other hand, the reaction efficiency is more than the first procedure on this occasion. These observations led to the conclusion that it could be due to the high reactivity of anilinolactones (Scheme 5). Under these conditions, the desired spiro-furo-pyrido-pyrimidine-indolines 4 were prepared in excellent yields by using the anilinolactones 8 as starting materials. The obtained results are outlined in Table 4.
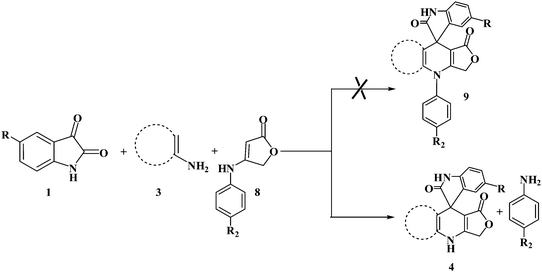 |
| Scheme 5 One-pot synthesis of spiro-furo-pyrido-pyrimidine-indoline derivatives using anilinolactones. | |
Table 4 Synthesis of spiro-furo-pyrido-pyrimidine-indoline derivatives using anilinolactones 4a
Entry |
1
|
3
|
8
|
Product |
Yieldb (%) |
Reagents and conditions: 1 (1 mmol), 2 (1 mmol), 8 (1 mmol), MnFe2O4 (5 mol%), H2O (5 mL), 90 °C, (3 h).
Yield of isolated product.
|
1 |
|
|
|
|
86 |
2 |
|
|
|
|
87 |
3 |
|
|
|
|
89 |
4 |
|
|
|
|
84 |
5 |
|
|
|
|
80 |
Although the detailed mechanism of the above mentioned reaction has not been clarified yet, the formation of 4 can be explained by a possible mechanism presented in Scheme 6. In the initial step, activated isatin 1 condenses with enamine 3 to afford reactive intermediate 9 in the presence of nano MnFe2O4 in water. This step was regarded as a fast Knoevenagel condensation. Then 9 was attacked via Michael addition of anilinolactones 8 to give the reactive intermediate 10. Finally, the product 4 was formed by intra molecular attack of the amino group onto the carbon atom of the activated imine group in 10 to produce mediated 11. This was followed by the aniline, as a leaving group, departing from 11 producing spiro-furo-pyrido-pyrimidine-indoline 4.
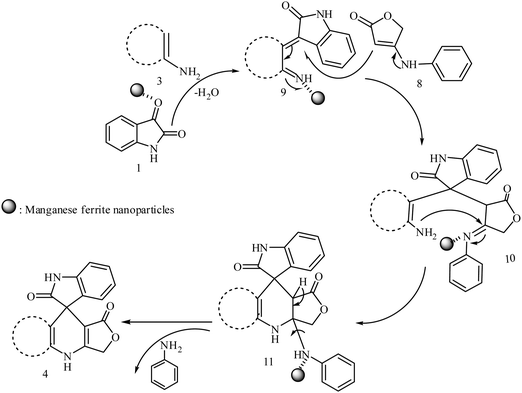 |
| Scheme 6 A plausible mechanism for the synthesis of 4. | |
To determine the percent leaching of ions from the catalyst surface, the model reaction was carried out in the presence of catalyst for 2 h, and at that point the catalyst was separated by an external magnet. The residue was then allowed to react, but no significant progress was observed after 10 h.
Conclusions
In summary, we have demonstrated a facile, highly efficient, and eco-friendly procedure for the one-pot synthesis of novel spirooxindoles via a three-component condensation reaction of isatins, tetronic acid and 2,6-diaminopyrimidin-4(3H)-one or uracils using manganese ferrite nanoparticles as a powerful, recoverable, and reusable nano catalyst in water. Mild reaction conditions, high yields and simple work-up are the important features of this protocol. Most importantly of all, easy magnetic separation of the catalyst eliminates the requirement of catalyst filtration after completion of the reaction. Furthermore, the catalyst remained highly active even after 5 repeated uses.
Experimental
4.1. Chemicals and apparatus
The chemicals used in this work were obtained from Fluka and Merck and were used without purification. Melting points were measured on an Electrothermal 9200 apparatus. Mass spectra were recorded on a Shimadzu QP 1100 Ex mass spectrometer operating at an ionization potential of 70 eV. IR spectra were recorded as KBr pellets on a Perkin-Elmer 781 spectrophotometer and an Impact 400 Nicolet FT-IR spectrophotometer. 1H NMR and 13C NMR spectra were recorded in DMSO-d6 solvents on a Bruker DRX-400 spectrometer with tetramethylsilane as the internal reference. The elemental analyses (C, H, N) were obtained from a Carlo ERBA Model EA 1108 analyzer. The X-ray diffraction (XRD) pattern of the as-synthesized material was obtained using a Holland Philips Xpert X-ray powder diffraction (XRD) diffractometer (CuK, radiation, λ = 0.154056 nm), at a scanning speed of 2° per min from 10° to 100° (2θ). The nanocatalyst was characterized using a Philips EM 208 transmission electron microscope (TEM) operating at a 100 kV accelerating voltage. The purity determination of the substrates and reaction monitoring were accomplished by TLC on silica-gel polygram SILG/UV 254 plates (from Merck Company).
4.2. Typical experimental procedure for the preparation of catalyst
MnFe2O4 nanoparticles have been prepared following the reported standard protocol by co-precipitation of MnCl2 and FeCl3 in water in the presence of sodium hydroxide. Briefly, MnCl2·4H2O and FeCl3·6H2O were taken in a molar ratio of Mn2+
:
Fe3+ = 1
:
2 to prepare a 0.3 mol L−1 metal ion solution of 100 mL containing 0.1 mol L−1 Mn2+ and 0.2 mol L−1 Fe3+, which was then dropped slowly into 100 mL of 3 mol L−1 NaOH solution at the preheated temperature of 95 °C. After aging for 2 h with continuous stirring, the mixture was filtered, washed and dried at 60 °C for 12 h. The XRD patterns of calcined precipitate indicate that the powder is mainly composed of MnFe2O4.37
4.3. Typical procedure for the preparation of spiro-furo-pyrido-pyrimidine-indoline derivatives 4(a–l)
Isatins (1 mmol), tetronic acid (1 mmol) and 2,6-diaminopyrimidin-4(3H)-one or uraciles (1 mmol) were placed in a 10 mL round-bottomed flask in H2O (5 mL). Then MnFe2O4 (5 mol%) was added and the resulting mixture was stirred at 90 °C for an appropriate time and monitored by TLC until conversion of the starting materials was satisfactory. After completion of the reaction, the mixture was magnetically concentrated with the aid of an external magnet to separate the catalyst. The separated catalyst was washed several times with acetone followed by water, then dried under vacuum and reused four times for the same reaction. The reaction mixture was filtered and the precipitate washed with water and recrystallized from EtOH to afford the pure products. All novel products were identified by physical and spectroscopic data.
2-Amino-3H-spiro[furo[3,4-g]pyrido[2,3-d]pyrimidine-5,3′-indoline]-2′,4,6(8H,9H)-trione (4a).
White powder (yield: 82%, 0.277 g). mp: >350 °C. IR (KBr) (νmax/cm−1): 3420, 3156, 1751, 1713, 1638; 1H NMR (DMSO-d6, 400 MHz): δ/ppm: 4.84 (2H, s, OCH2), 6.58–7.06 (6H, m, ArH and NH2), 10.06 (1H, s, NH), 10.19 (1H, s, NH), 10.47 (1H, s, NH); 13C NMR (DMSO-d6, 100 MHz): δppm: 47.4, 65.7, 91.0, 98.7, 108.9, 121.5, 123.8, 127.9, 136.0, 142.7, 155.0, 155.4, 158.8, 161.2, 170.2, 179.0; Anal. Calcd for C16H11N5O4: C, 56.98; H, 3.29; N, 20.76%; Found C, 56.97; H, 3.31; N, 20.71%; MS: m/z 337.
Acknowledgements
This study is part of Zahra Rashid’s PhD thesis entitled: “Synthesis, modification and functionalization of magnetic nanoparticles for catalytic application in the synthesis of heterocyclic compounds and biomedical applications” which has been conducted in the Nanobiotechnology Research Center, Avicenna Research Institute. We are also thankful to University of Kashan for supporting this work by grant number 159148/15, and gratefully acknowledge financial support from the Avicenna Research Institute.
References
-
(a) A. Dömling, Chem. Rev., 2006, 106, 17 CrossRef PubMed;
(b) B. B. Toure and D. G. Hall, Chem. Rev., 2009, 109, 4439 CrossRef CAS PubMed;
(c) J. D. Sunderhaus and S. F. Martin, Chem.–Eur. J., 2009, 15, 1300 CrossRef CAS PubMed.
-
(a) H. Wang and D. Shi, ACS Comb. Sci., 2013, 15, 261 CrossRef CAS PubMed;
(b) Z. Xiao, M. Lei and L. Hu, Tetrahedron Lett., 2011, 52, 7099 CrossRef CAS PubMed.
- R. Abonia, J. Castillo, B. Insuasty, J. Quiroga, M. Nogueras and J. Cobo, ACS Comb. Sci., 2013, 15, 2 CrossRef CAS PubMed.
-
(a) L. Fu, Q. Shi, Y. Shi, B. Jiang and S. Tu, ACS Comb. Sci., 2013, 15, 135 CrossRef CAS PubMed;
(b) S. Wang, C. Cheng, F. Wu, B. Jiang, F. Shi, S. Tu, T. Rajale and G. b. Li, Tetrahedron, 2011, 67, 4485 CrossRef CAS PubMed.
- M. Herrera, I. Knepper, N. Souza, A. Villinger, V. Sosnovskikh and V. Iaroshenko, ACS Comb. Sci., 2012, 14, 434 CrossRef PubMed.
- S. Rajesh, B. Bala, S. Perumal and J. C. Menendez, Green Chem., 2011, 13, 3248 RSC.
- M.-O. Simon and C. Li, J. Chem. Soc. Rev., 2012, 41, 1415 RSC.
- A. Moshtaghi Zonouz, I. Eskandari and H. R. Khavasi, Tetrahedron Lett., 2012, 53, 5519 CrossRef PubMed.
-
(a) B. H. Lipshutz, S. Ghorai, A. R. Abela, R. Moser, T. Nishikata, C. Duplais and A. Krasovskiy, J. Org. Chem., 2011, 76, 4379 CrossRef CAS PubMed;
(b) B. H. Lipshutz, S. Ghorai, W. W. Y. Leong and B. R. Taft, J. Org. Chem., 2011, 76, 5061 CrossRef CAS PubMed.
- M. Casula, A. Corrias, P. Arosio, A. Lascialfari, T. Sen, P. Floris and J. I. Bruce, J. Colloid Interface Sci., 2011, 357, 50 CrossRef CAS PubMed.
- K. Rudzka, J. L. Viota, J. A. Muñoz-Gamez, A. Carazo, A. Ruiz-Extremera and A. V. Delgado, Colloids Surf., B, 2013, 111, 88 CrossRef CAS PubMed.
- S. Thayyil, S. Schievano, J. Robertson, N. R. Jones, S. Chitty, L. J. Sebire and N. A. Taylor, Eur. J. Radiol., 2009, 72, 321 CrossRef PubMed.
- T. Sadhukha, T. Wiedmann and J. Panyam, Biomaterials, 2013, 34, 5163 CrossRef CAS PubMed.
- D. L. Graham, H. A. Ferreira and P. P. Freitas, Trends Biotechnol., 2004, 22, 455 CrossRef CAS PubMed.
- D. Wang, J. He, N. Rosenzweig and Z. Rosenzweig, Nano Lett., 2004, 4, 409 CrossRef CAS.
-
(a) Q. Du, W. Zhang, H. Ma, J. Zheng, B. Zhou and Y. Li, Tetrahedron, 2012, 68, 3577 CrossRef CAS PubMed;
(b) K. Fujita, S. Umeki, M. Yamazaki, T. Ainoya, T. Tsuchimoto and H. Yasuda, Tetrahedron Lett., 2011, 52, 3137 CrossRef CAS PubMed;
(c) B. Baruwati, V. Polshettiwar and R. S. Varma, Tetrahedron Lett., 2009, 50, 1215 CrossRef CAS PubMed;
(d) V. Polshettiwar and R. S. Varma, Tetrahedron, 2010, 66, 1091 CrossRef CAS PubMed.
-
(a) J. Deng, M. Li, F. Zhao, Z. Zhang and S. Liu, ACS Comb. Sci., 2012, 14, 335 CrossRef CAS PubMed;
(b) F. Nemati, R. Heravi and R. Saeeidi Rad, Chin. J. Catal., 2012, 33, 1825 CrossRef CAS;
(c) J. Davarpanah and A. R. Kiasat, Catal. Commun., 2013, 42, 98 CrossRef CAS PubMed.
-
(a) J. Wang, B. Xu, H. Sun and G. Song, Tetrahedron Lett., 2013, 54, 238 CrossRef CAS PubMed;
(b) A. R. Kiasat and S. Nazari, J. Mol. Catal. A: Chem., 2012, 365, 80 CrossRef CAS PubMed.
- S. Sayin, M. Yilmaz and M. Tavasli, Tetrahedron, 2011, 67, 3743 CrossRef CAS PubMed.
- B. Li, L. Gao, F. Bian and W. Yu, Tetrahedron Lett., 2013, 54, 1063 CrossRef CAS PubMed.
-
(a) M. M. Khafagy, A. H.F. A. El-Wahas, F. A. Eid and A. M. El- Agrody, Farmaco, 2002, 57, 715 CrossRef CAS;
(b) P. R. Sebahar and R. M. Williams, J. Am. Chem. Soc., 2000, 122, 5666 CrossRef CAS.
-
(a) J. F. M. Da Silva, S. J. Garden and A. C. Pinto, J. Braz. Chem. Soc., 2001, 12, 273 CrossRef CAS PubMed;
(b) A. H. Abdel-Rahman, E. M. Keshk, M. A. Hanna and S. M. El-Bady, Bioorg. Med. Chem., 2004, 12, 2483 CrossRef CAS PubMed.
- T. Okita and M. Isobe, Tetrahedron, 1994, 50, 11143 CrossRef CAS.
- M. J. Kornet and A. P. Thio, J. Med. Chem., 1976, 19, 892 CrossRef CAS.
- P. Rosenmond, M. Hosseini-Merescht and C. Bub, Liebigs Ann. Chem., 1994, 2, 151 CrossRef.
- T. Usui, M. Kondoh, C.-B. Cui, T. Mayumi and H. Osada, Biochem. J., 1998, 333 Search PubMed.
-
(a) M. M. Khafagy, A. H. F. A. El-Wahsa, F. A. Eid and A. M. El-Agrody, Fannaco, 2002, 57, 715 CAS;
(b) P. R. Sebahar and R. M. Williams, J. Am. Chem. Soc., 2000, 122, 5666 CrossRef CAS.
- T. H. Kang, K. Matsumoto, Y. Murakami, H. Takayama, M. Kitajima, N. Aimi and H. Watanabe, Eur. J. Pharmacol., 2002, 444, 39 CrossRef CAS.
-
(a) A. Alizadeh, A. Rezvanian and L.-G. Zhu, J. Org. Chem., 2012, 77, 4385 CrossRef CAS PubMed;
(b) H. Chen and D. Shi, J. Comb. Chem., 2010, 12, 571 CrossRef CAS PubMed.
-
(a) B. Maheshwar Rao, G. Niranjan Reddy, T. Vijaikumar Reddy, B. L. A. Prabhavathi Devi, R. B. N. Prasad, J. S. Yadav and B. V. Subba Reddy, Tetrahedron Lett., 2013, 54, 2466 CrossRef CAS PubMed;
(b) S.-J. Chai, Y.-F. Lai, J.-C. Xu, H. Zheng, Q. Zhu and P.-F. Zhang, Adv. Synth. Catal., 2011, 353, 371 CrossRef CAS.
- Z. Noroozi Tisseh, F. Ahmadi, M. Dabiri, H. R. Khavasi and A. Bazgir, Tetrahedron Lett., 2012, 53, 3603 CrossRef PubMed.
- S. Paul and A. Das, Tetrahedron Lett., 2013, 54, 1149 CrossRef CAS PubMed.
- S. A. El-Assaly, Pharma Chem., 2011, 3, 81 CAS.
-
(a) R. Ghahremanzadeh, G. Imani Shakibaei, S. Ahadi and A. Bazgir, J. Comb. Chem., 2010, 12, 191 CrossRef CAS PubMed;
(b) R. Ghahremanzadeh, T. Amanpour and A. Bazgir, J. Heterocycl. Chem., 2010, 47, 46 CAS.
- S. Ahadi, R. Ghahremanzadeh, P. Mirzaei and A. Bazgir, Tetrahedron, 2009, 65, 9316 CrossRef CAS PubMed.
- R. Ghahremanzadeh, T. Amanpour, M. Sayyafi and A. Bazgir, J. Heterocycl. Chem., 2010, 47, 421 CAS.
- J.-H. Lee, Y.-M. Huh, Y.-W. Jun, J.-W. Seo, J.-T. Jang, H.-T. Song, S. Kim, E.-J. Cho, H.-G. Yoon, J.-S. Suh and J. Cheon, Nat. Med., 2007, 13, 95 CrossRef CAS PubMed.
- P. Lahiri and S. Sengupta, Can. J. Chem., 1991, 69, 33 CrossRef CAS PubMed.
- H. Aijun, L. Juanjuan, Y. Mingquan, L. Yan and P. Xinhua, Chin. J. Chem. Eng., 2011, 19, 1047 CrossRef.
-
(a) H. Chen and D. Shi, J. Comb. Chem., 2010, 12, 571 CrossRef CAS PubMed;
(b) G. Verma, K. Raghuvanshi, R. Kumar and M. Shankar Singh, Tetrahedron Lett., 2012, 53, 399 CrossRef CAS PubMed;
(c) S. Tu, C. Li, F. Shi, D. Zhou, Q. Shao, L. Cao and B. Jiang, Synthesis, 2008, 369 CrossRef CAS PubMed.
- Y. Hitotsuyanagi, M. Kobayashi, M. Fukuyo, K. Takeya and H. Itokawa, Tetrahedron Lett., 1997, 38, 8295 CrossRef CAS.
Footnote |
† Electronic supplementary information (ESI) available. See DOI: 10.1039/c3nj00940h |
|
This journal is © The Royal Society of Chemistry and the Centre National de la Recherche Scientifique 2014 |