DOI:
10.1039/C3NJ00863K
(Paper)
New J. Chem., 2014,
38, 338-347
Palladium(II) complexes with planar chiral ferrocenyl phosphane–(benz)imidazol-2-ylidene ligands†
Received
(in Victoria, Australia)
30th July 2013
, Accepted 31st October 2013
First published on 19th November 2013
Abstract
We describe here the first examples of planar chiral ferrocenyl phosphane–benzimidazol-2-ylidene ligands and their coordination chemistry with palladium(II). All ligand precursors, namely enantiopure ferrocenyl phosphane–(benz)imidazolium salts, and all enantiopure palladium complexes have been fully characterised by 1H, 31P and 13C NMR, mass spectrometry and X-ray diffraction methods for seven examples. The potential of these very bulky bidentate ligands in catalysis was evaluated and compared to their imidazol-2-ylidene analogues. The influence of sterics was shown to be non-negligible as the bulkiest ligand gave the lowest activities in the asymmetric Suzuki–Miyaura reaction.
Introduction
The synthesis and coordination chemistry of functionalised N-heterocyclic carbene (NHC) ligands and their applications in catalysis have attracted the attention of several groups,1–3 including ours.4–7 These strong σ-donor ligands proved very efficient in catalysis, even surpassing phosphanes in many cases.8 One advantage of using N-heterocyclic carbenes is the high stability conferred on the metal complexes, thus avoiding the use of excess ligand or catalyst deactivation. Fine tuning the stereoelectronic properties of the N-heterocyclic carbene may allow us finding useful trends in the search of the best catalyst, particularly in terms of enantioselectivity. Recently, we described the synthesis of 1,1′-disubstituted ferrocenyl phosphane–benzimidazol-2-ylidene ligands for applications in rhodium-catalysed hydrosilylation of carbonyl groups. It was shown in this case that the replacement of the imidazol-2-ylidene moiety by the more bulky, electron-rich benzimidazol-2-ylidene bearing two methyl groups on the aromatic ring enhanced the catalytic activity dramatically.6
It is now commonly admitted that strongly electron-donating, bulky ligands favour oxidative addition on palladium and help stabilise the intermediate species in C–C coupling reactions such as for instance the Suzuki–Miyaura reaction.9–11 Furthermore, we have recently turned our attention to the asymmetric version of this reaction, obtaining encouraging results with the use of palladium complexes containing enantiomerically pure phosphane–imidazol-2-ylidene ligands (Fig. 1).7 We therefore turned our efforts towards the synthesis of palladium complexes containing novel chiral 1,2-disubstituted ferrocenyl phosphane–(benz)imidazol-2-ylidene ligands in enantiomerically pure form and describe here their synthesis. Their catalytic properties in the asymmetric Suzuki–Miyaura reaction11–15 have been evaluated and compared to those of the above mentioned phosphane–imidazol-2-ylidene complexes.7 Finally, the activity of a phosphane–imidazol-2-ylidene ligand in the asymmetric 1,2-addition of phenylboronic acid to 4-nitrobenzaldehyde has been evaluated.16–19
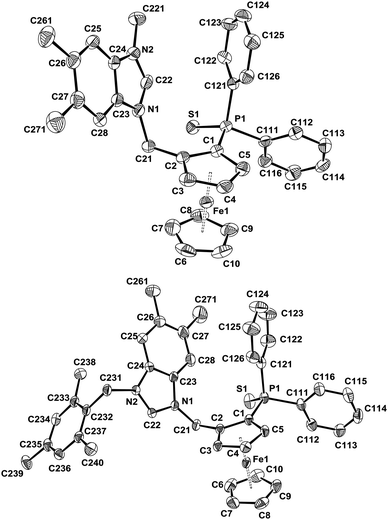 |
| Fig. 1 ORTEP representations of benzimidazolium salts rac-2c (top) and rac-2d (bottom). Ellipsoids are shown at the 50% level. All hydrogens are omitted for clarity. Selected bonds (Å), rac-2c: C(23)–C(24) = 1.388(8); rac-2d: C(23)–C(24) = 1.390(3). | |
Results and discussion
Synthesis of (benz)imidazolium salts
With the intention of varying the stereoelectronic properties of the ferrocenyl phosphane–NHC ligands in a rational way, we decided to focus on the NHC moiety without changing the diphenylphosphano group. The σ-donating properties of benzimidazol-2-ylidene ligands are situated between those of unsaturated imidazol-2-ylidenes and of saturated imidazolin-2-ylidenes, which has been demonstrated in several examples of catalytic applications.20,21 Apart from the electronic properties, their structure should bring more bulkiness to the bifunctional ligands. In order to probe the effect of bulky substituents on both activity and enantioselectivity, we have also changed the nature of the N-substituent.
The synthesis of the phosphane–imidazolium salt with R = Me (3a) has already been described, both in racemic5 and enantiomerically pure form.7 The synthesis of other racemic and enantiomerically pure imidazolium and benzimidazolium salts was carried out following these established protocols.5 Racemic alcohol rac-1 was used mainly to develop and optimise the synthesis of various salts and palladium complexes, but only enantiopure (S)-palladium complexes were used in catalytic reactions since we expected them to exhibit similar levels of activity compared to the previously described neutral palladium complex [PdCl2(ImMe)] (Scheme 1).7 Imidazolium salt 2b was obtained in moderate yield from ferrocenyl alcohol 1 and N-[(2,4,6-trimethylphenyl)methyl] imidazole (Scheme 2).
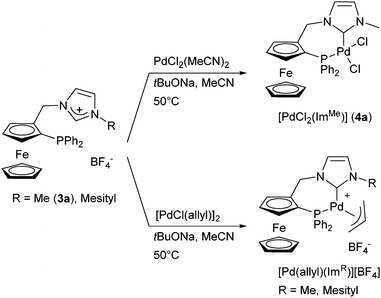 |
| Scheme 1 Palladium(II) complexes described in our previous work.7 | |
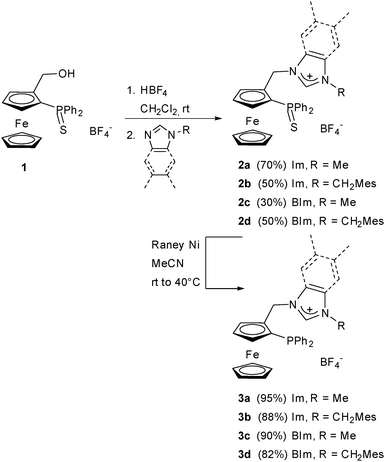 |
| Scheme 2 Synthesis of phosphane-(benz)imidazolium salts. | |
The synthesis of benzimidazolium salts 2c,d, however, proved to be more difficult. Benzimidazoles are poorer nucleophiles compared to imidazoles, thus giving greater amounts of side products and lower yields of benzimidazolium salts. Their purification was also less straightforward: the excess of N-substituted benzimidazoles that are not consumed during the reaction cannot be removed with aqueous acidic washings due to their lower hydrophilicity and have a tendency to trail on silica when the same eluting conditions as for the imidazolium salts are applied. Eventually, their purification was successful by eluting the N-substituted benzimidazole excess with pure diethyl ether and then the benzimidazolium salts with a DCM–acetone mixture on a silica gel chromatography column. The 1H NMR spectra of (benz)imidazolium salts 2b–d show the expected signal above δ 8.5, characteristic of the acidic C2 proton. AB systems were observed for the diastereotopic protons of the methylene bridge situated between the ferrocenyl and (benz)imidazolyl moieties for all salts, as well as for protons of the methylene bridge linking the (benz)imidazolyl and mesityl groups (salts 2b and 2d), which is consistent with the introduction of planar chirality at ferrocene and with the generation of rather rigid structures due to steric crowding.
The structure of the intermediates 2c and 2d (Fig. 1) was confirmed by X-ray diffraction methods. The structures of the two salts as well as that of 2a‡
7 are very close: the only notable structural difference resides in the length of the C–C bond in the imidazolium motif, which is significantly shorter in the imidazolium salt (C(7)–C(8) = 1.344(4) Å) than in the benzimidazolium salts. This has already been observed in similar structures and indicates delocalisation of the C–C bond π-electron density over the fused benzene ring.6
The imidazolium and benzimidazolium salts exhibited different reactivity during the diphenylthiophosphano group deprotection by RANEY® nickel. Indeed, whereas the desulfurisation of the thiophosphane–imidazolium salt 2b to give 3b went to completion at room temperature in 16 h, longer reaction times and moderate heating (40 °C) were necessary to achieve complete desulfurization of the thiophosphane–benzimidazolium salts 2c,d to give 3c,d (31P NMR monitoring). This is a first confirmation that steric crowding is more important in the benzimidazolium family, with possible implications in catalysis. As expected, upon deprotection of the diphenylthiophosphano group, the 31P NMR signal of (benz)imidazolium salts shifted from δ ca. 40.5 for 2 to ca. −27 for 3.
Synthesis of palladium(II) complexes
The synthesis and X-ray structural characterisation of complex 4a, obtained by deprotonation of 3a both in racemic and enantiopure forms, have been previously published.‡
7 We have now extended this procedure to the synthesis of the analogous complexes with the NHC ligands obtained from 3b, 3c and 3d (Scheme 3).
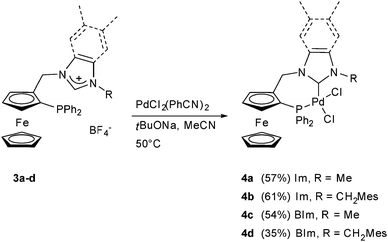 |
| Scheme 3 Synthesis of neutral NHC–phosphane palladium(II) complexes. | |
All phosphane–(benz)imidazolium salts were deprotonated with tBuONa in the presence of the palladium precursor PdCl2(PhCN)2 to give neutral N-heterocyclic carbene–phosphane palladium complexes 4b–d in moderate yields. All products are air-stable and could be purified by column chromatography on silica gel. The absence of the acidic proton characteristic of (benz)imidazolium salts in the 1H NMR spectra indicated carbene formation. The 31P NMR spectra of all complexes showed a major species with a signal between δ 3.86 and 4.56, along with ca. 2% of a second species at around δ 15. This minor species was obtained whatever the complex and mass spectrometry analysis of the mixture by electrospray revealed a small amount of palladium dimer along with the expected monomeric structure. The carbenic carbon in 13C NMR was found at δ 156.0 for complex 4b bearing an imidazol-2-ylidene moiety and went downfield for complexes 4c,d possessing the benzimidazol-2-ylidene group with, respectively, δ 166.70 and 166.74. The same trend has been observed for other neutral palladium(II) NHC complexes in the literature.21,22
The structures of all new complexes were established by X-ray crystallography and compared with that of 4a.7 The bond lengths and angles are all within the expected range for neutral palladium complexes with a slightly distorted square-planar geometry (Fig. 2 and 3, Table 1). Pd–C(NHC) (1.973(2) Å, 4b, to 1.992(6) Å, 4d) and Pd–P (2.2444(8) Å, 4a, to 2.2546(19) Å, 4d) bond lengths compare well with those in other previously published neutral palladium complexes bearing chelating NHC–phosphane ligands.21,22 As observed previously for complex 4a,9 the Pd–Cl bonds trans to the NHC are all slightly but significantly shorter than the Pd–Cl bonds trans to the diphenylphosphano groups, which is surprising since the more donating NHC should exert a greater trans influence than the phosphane, but this was also observed elsewhere.21,22 Similar to the (benz)imidazolium salts, the C–C bond of the heteroaromatic unit is slightly longer in the case of benzimidazol-2-ylidenes (1.372(5) Å for 4c and 1.391(8) Å for 4d) than in the case of imidazol-2-ylidenes (1.350(6) Å for 4a and 1.345(4) Å for 4b), which could denote some delocalisation of the double bond due to the presence of the fused benzene ring. No other significant differences in bond lengths are notable between the four structures. However, the C(NHC)–Pd–P bite angle is significantly narrower in complex 4a with 84.95(9)° and increases with the steric bulkiness of the ligand to reach 91.94(19)° in complex 4d.
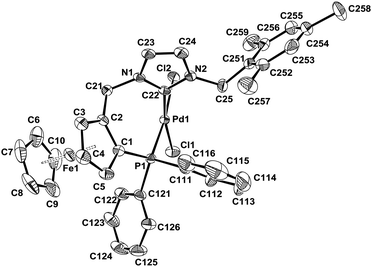 |
| Fig. 2 ORTEP representation of complex (S)-4b. Ellipsoids are shown at the 50% level. All hydrogens are omitted for clarity. | |
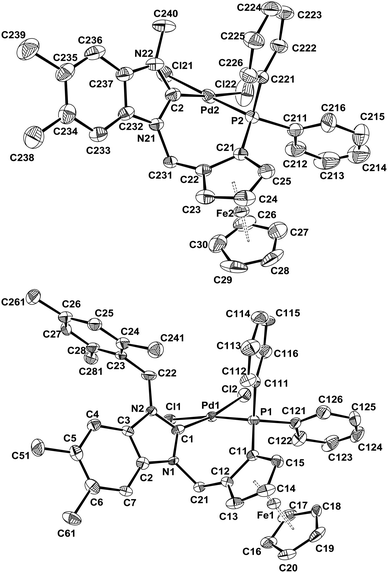 |
| Fig. 3 ORTEP representations of complexes (S)-4c (top) and rac-4d (bottom). Ellipsoids are shown at the 50% level. All hydrogens are omitted for clarity. | |
Table 1 Selected distances (Å) and angles (°) for complexes 4b–d and comparison with those of 4a7
|
4a
|
4b
|
4c
|
4d
|
Distance (Å) |
|
|
|
|
Pd–C(NHC) |
1.991(3) |
1.973(2) |
1.975(4) |
1.992(6) |
Pd–P |
2.2444(8) |
2.2449(5) |
2.2475(9) |
2.2546(19) |
Pd–Cl(trans-NHC) |
2.3397(8) |
2.3414(6) |
2.3043(12) |
2.3395(16) |
Pd–Cl(trans-P) |
2.3614(9) |
2.3798(5) |
2.3347(10) |
2.3725(18) |
C C (NHC) |
1.350(6) |
1.345(4) |
1.372(5) |
1.391(8) |
|
Angle (°) |
N–C(NHC)–N |
106.4(3) |
106.1(2) |
106.4(3) |
107.3(5) |
C(NHC)–Pd–P |
84.95(9) |
88.90(6) |
89.94(10) |
91.94(19) |
N–C(CH2)–C(Cp) |
112.2(3) |
109.22(19) |
111.1(3) |
110.7(5) |
N–C(CH2)–C(Mes) |
— |
115.3(2) |
— |
114.7(5) |
Asymmetric Suzuki–Miyaura reaction
Palladium(II) complexes 4b–d were evaluated in the asymmetric version of the Suzuki–Miyaura cross-coupling reaction between naphthyl bromides and naphthylboronic acid. We previously demonstrated that complex 4a is active for the coupling of substituted naphthyl bromides with naphthylboronic acid at low catalyst loadings and gives, as expected, similar levels of enantioselectivities with the (R) and (S) enantiomers.7 Therefore, the other complexes were tested directly under the same conditions with the enantiomerically pure ligands possessing the (S) configuration. As expected, all complexes are active for the coupling of various naphthyl bromides with a catalytic charge of 0.5 mol% at 40 °C. All reactions were stopped after 24 h to maximise conversions and allow comparison of the results (Scheme 4).
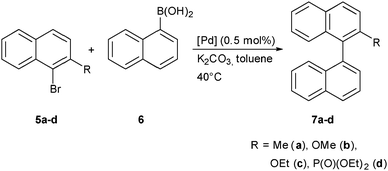 |
| Scheme 4 Asymmetric Suzuki–Miyaura reaction between 2-substituted α-naphthyl bromides and α-naphthylboronic acid. | |
The first observation to be made is that steric hindrance in the catalyst indeed plays a role since for each reaction the product yield decreases as the steric crowding on the complex increases (Table 2). Whereas 4a gives excellent yields of the expected coupling product for most substrates, 4b gives the products in only moderate yields and the unexpectedly low yield for the ethoxy substrate cannot be rationally explained.
Table 2 Catalytic performances of complexes 4a–d in the reaction of Scheme 4a
Entry |
R |
Pd cat. |
Conv.b (%) |
Yieldc (%) |
e.e.d (%) |
Reagents and conditions: naphthyl bromide (1.0 equiv.), boronic acid (1.2 equiv.), Pd cat. (0.5 mol%), K2CO3 (2.4 equiv.), toluene, 24 h.
Determined by integration of characteristic 1H NMR signals in the crude mixture.
Isolated yield.
Determined by HPLC with a Chiracel-OJ column.
The commercial 1-bromo-2-methylnaphthalene contains ca. 6% of 2-methylnaphthalene.
Ref. 7.
2 mol% of Pd catalyst were used.
|
1 |
Mee |
(S)-4a |
n.d. |
88 |
40 (S)f |
2 |
|
(R)-4a |
95 |
88 |
42 (R)f |
3 |
|
(S)-4b |
63 |
58 |
46 (S) |
4 |
|
(S)-4c |
60 |
48 |
9 (S) |
5 |
|
(S)-4d |
62 |
47 |
37 (S) |
6 |
OMe |
(S)-4a |
n.d. |
95 |
35 (R)f |
7 |
|
(R)-4a |
n.d. |
93 |
33 (S)f |
8 |
|
(S)-4b |
88 |
65 |
31 (R) |
9 |
|
(S)-4c |
67 |
54 |
17 (S) |
10 |
|
(S)-4d |
67 |
57 |
22 (R) |
11 |
OEt |
(S)-4a |
n.d. |
95 |
23 (R)f |
12 |
|
(R)-4a |
n.d. |
92 |
24 (S)f |
13 |
|
(S)-4b |
22 |
22 |
26 (R) |
14 |
|
(S)-4c |
80 |
65 |
13 (S) |
15 |
|
(S)-4d |
79 |
62 |
23 (R) |
16 |
|
(S)-4d |
75 |
54 |
28 (R)g |
17 |
P(O)(OEt)2 |
(S)-4a |
26 |
0 |
— |
18 |
|
(S)-4b |
28 |
0 |
— |
19 |
|
(S)-4c |
35 |
0 |
— |
20 |
|
(S)-4d |
8 |
0 |
— |
Complexes 4c and 4d bearing the more bulky benzimidazol-2-ylidene backbone also give moderate yields of the expected coupling products. Although the yields were low with all complexes except for 4a, it seems that steric bulk could be slightly beneficial for the asymmetric induction in some cases, since slightly better e.e.s were obtained with 4b (up to 46%), bearing a CH2Mes substituent in place of a methyl group. Again, unexpectedly low e.e.s were obtained with complex 4c, which cannot only be explained in terms of steric congestion since complex 4d, which possesses the bulkiest ligand, gives better e.e.s than complex 4c. Increasing the catalytic charge from 0.5 mol% to 2 mol% did not improve the level of enantioselectivity or the yield of coupling product (entry 16). The naphthyl bromide bearing a phosphonate substituent at the 2-position has been widely used in asymmetric Suzuki–Miyaura reactions and usually gives better e.e.s than other substrates, as it seems that a chelating functionality on one coupling partner is beneficial to achieve good enantioselectivities.11,14 However, in our case none of the expected coupling product was obtained whatever the complex used, only a mixture of naphthyl bromide and the corresponding dehalogenated product. Hydrodehalogenation has already been observed under the conditions of the Suzuki–Miyaura coupling23 and this could indicate that the oxidative addition takes place but the following step, i.e. the transmetallation of the boronic acid, does not occur. One of the reasons could be the bulkiness of our ligands, but chelating diphosphanes have also been reported not to give conclusive results with this substrate.13 Therefore, the chelating nature of our ligands, which have two strongly coordinating groups, could be detrimental to the reaction in certain cases.
Asymmetric 1,2-addition of phenylboronic acid to 4-nitrobenzaldehyde
The addition products such as diarylmethanols are very important intermediates in the synthesis of biologically active compounds.24 This reaction has been well studied with rhodium complexes, with the first example of enantioselective 1,2-addition of boronic acids to aldehydes described by Miyaura in 1998.25 Diarylmethanols have thus been obtained with very good enantioselectivities (up to 94% e.e.),26 but the use of cheaper palladium complexes in the asymmetric version of this reaction is much less common.17–19 Indeed, only two recent examples show poor to moderate enantioselectivities, using a planar chiral ferrocenyl phosphine (up to 11% e.e.)18 or axially chiral NHC ligands (up to 65% e.e.).19 We thus turned to the 1,2-addition of PhB(OH)2 to 4-nitrobenzaldehyde, hoping to improve the previously measured enantioselectivities (Scheme 5, Table 3). As the synthesis of 4a is more straightforward than that of 4b–d, we therefore carried out preliminary tests with enantiopure (R)-4a only. The reaction was first conducted in THF using KOH as base and 5 mol% of palladium complex (entry 1).
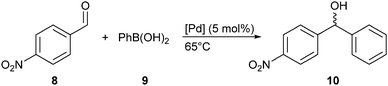 |
| Scheme 5 1,2-Addition of phenylboronic acid to 4-nitrobenzaldehyde. | |
Table 3 Catalytic performances of complex (R)-4a in the reaction of Scheme 5
Entry |
t (h) |
Solvent |
Base |
Yieldc (%) |
e.e.d (%) |
Reagents and conditions: 4-nitroenzaldehyde (1 equiv.), phenylboronic acid (2 equiv.), KOH (3 equiv.), THF (5 mL), 24 h.
Reagents and conditions: 4-nitroenzaldehyde (1 equiv.), phenylboronic acid (1.5 equiv.), base (2 equiv.), solvent (1 mL).
Isolated yield.
Determined by HPLC with a Chiracel-AD-H column and compared with published results.18,19
|
1a |
24 |
THF |
KOH |
22 |
13 (R) |
2b |
24 |
Dioxane |
CsF |
22 |
3 (R) |
3b |
4 |
Dioxane |
CsF |
32 |
7 (R) |
4b |
24 |
Toluene |
K2CO3 |
69 |
12 (R) |
5b |
4 |
Toluene |
K2CO3 |
45 |
11 (R) |
The selectivity with THF/KOH was not satisfactory as a non-negligible amount of 4-nitrobenzyl alcohol from the Cannizzaro reaction, as well as other minor by-products were observed in the 1H NMR spectrum of the crude mixture. Changing the solvent–base couple to dioxane–CsF was no better: although the 1H NMR spectrum of the crude mixture showed almost complete consumption of the starting aldehyde, several unidentified by-products were also observed (entries 2 and 3). Only the toluene–K2CO3 couple gave interesting results in terms of selectivity and yield (entries 4 and 5). Unfortunately, the levels of enantioselectivity measured for complex (R)-4a were poor, with up to 13% e.e. in THF (entry 1), and are comparable to those obtained by Suzuma et al.18 However, this is a preliminary work and a more thorough study of the reaction conditions with this and other chiral palladium complexes will be carried out in order to improve the efficiency of this challenging reaction.
Conclusions
We have described in this paper the synthesis of a new family of chiral Pd complexes, containing enantiomerically pure phosphane–(benz)imidazol-2-ylidene ligands. A first application of these complexes to the asymmetric Suzuki–Miyaura reaction has revealed that the bulkiness of ferrocene conjugated with that of benzimidazol-2-ylidene seems to be detrimental to the catalytic activity. The influence of the NHC structure on enantioselectivity is not entirely predictable and the best e.e. of 46% was obtained with 4b, only a 4% improvement compared to the previously investigated complex 4a, and to the detriment of the yield. Preliminary tests in the catalysed 1,2-addition of phenylboronic acid to 4-nitrobenzaldehyde with complex 4a showed moderate activities and low enantioselectivities. Future work will aim at designing other types of chiral functionalised N-heterocyclic carbenes in order to find the best compromise between activity and enantioselectivity.
Experimental
General considerations
All manipulations were performed under an inert atmosphere of dry argon by using vacuum line and Schlenk tube techniques. Solvents for syntheses were dried and degassed by standard methods before use. 1D- and 2D-NMR spectra were recorded on Bruker AV500 and Bruker AV300 spectrometers. 1H and 13C chemicals shifts (δ) are given in ppm (the residual peak of deuterated solvent was used as reference). 31P chemical shifts are reported in ppm. Peaks are labelled as singlet (s), doublet (d), triplet (t), multiplet (m) and broad (br). The proton and carbon assignments were performed by COSY, NOESY, HSQC, 1H-13C HMBC, 1H-31P HMBC experiments. MS spectra were performed by the mass spectrometry service of the Paul Sabatier University. Optical rotations were measured in a 1 dm cell using a Perkin-Elmer 241 photopolarimeter. GC chromatograms were recorded on a HP 4890A GC equipped with a DB-5 MS UI capillary column and the products were identified by comparison with authentic samples. HPLC chromatograms were recorded on a Shimadzu LC-2010A(HT) equipped with Chiralcel OJ or Chiralcel AD-H columns. Commercial chemicals were from Acros, Aldrich, Alfa Aesar, or Fluka and used as received. 1,2-Ferrocenyl alcohol 1,27 imidazolium salts 2a, 3a5 and palladium complex 4a7 were prepared according to previously reported procedures. Abbreviations: CpA: cyclopentadienyl ring with PPh2 or PPh2(=S) substituents; CpB: unsubstituted cyclopentadienyl ring; Fc: ferrocenyl; Im: imidazolyl; BIm: benzimidazolyl; Mes: mesityl. Cq: quaternary carbon.
Syntheses
FcPPh2(=S)CH2Im+CH2MesBF4−2b.
To a solution of ferrocenyl alcohol 1 (100 mg, 0.23 mmol) in dry CH2Cl2 (10 mL) was quickly added HBF4 (54 wt% in Et2O, 50 μL, 0.35 mmol), immediately followed by N-2,4,6 trimethylbenzylimidazole (110 mg, 0.55 mmol). After 5 minutes, the mixture was washed with 2 M aq. HCl, water, sat. aq. NaHCO3 and water, dried (MgSO4), filtered and concentrated in vacuo. The residue was purified by precipitation with methyl tert-butyl ether from a CH2Cl2 solution to give a yellow solid (80 mg, 50% yield). 1H NMR (300 MHz, CDCl3, 298 K) δ 8.61 (1H, s, NCHN), 7.78–7.71 (2H, m, CH PPh2), 7.60–7.47 (4H, m, CH PPh2), 7.40 (1H, m, CH PPh2), 7.30 (3H, m, CH PPh2), 7.15 (1H, s, CH Mes), 6.88 (2H, s, NCH = CH), 6.51 (1H, s, CH Mes), 6.40 (1H, AB syst., JHH = 14 Hz, CH2-Fc), 5.22 (1H, AB syst., JHH = 14 Hz, CH2-Fc), 5.21 (1H, br. s, CpA), 5.14 (1H, AB syst., JHH = 14 Hz, CH2-Mes), 4.91 (1H, AB syst., JHH = 14 Hz, CH2-Mes), 4.53 (1H, br. s, CpA), 4.35 (5H, s, CpB), 3.86 (1H, br. s, CpA), 2.28 (3H, s, p-CH3 Mes), 2.13 (6H, s, o-CH3 Mes). 13C{1H} NMR (75.5 MHz, CDCl3, 298 K) δ 139.88 (Cq Mes, C-CH2), 137.93 (2 Cq Mes, C-o-CH3), 134.91 (NCHN), 134.66 (d, JCP = 86 Hz, Cq PPh2), 132.08 (d, JCP = 56 Hz, Cq PPh2), 131.90 (d, JCP = 11 Hz, CH PPh2), 131.70 (d, JCP = 3 Hz, CH PPh2), 131.25 (d, JCP = 10 Hz, CH PPh2), 129.89 (2 CH, NCH = CH), 128.47 (d, JCP = 11 Hz, CH PPh2), 128.33 (d, JCP = 12 Hz, CH PPh2), 125.35 (Cq Mes, C-p-CH3), 122.04 (CH Mes), 120.24 (CH Mes), 83.44 (d, JCP = 12 Hz, Cq CpA), 76.76 (d, JCP = 10 Hz, CH CpA), 76.00 (d, JCP = 11 Hz, CH CpA), 73.55 (d, JCP = 93 Hz, Cq CpA), 71.39 (d, JCP = 9 Hz, CH CpA), 71.23 (5 CH CpB), 47.91 (CH2-Fc or CH2-Mes), 47.40 (CH2-Fc or CH2-Mes), 21.04 (p-CH3 Mes), 19.61 (o-CH3 Mes). 31P{1H} NMR (121 MHz, CDCl3, 298 K) δ 40.65. MS (ESI) m/z 615 [M+] (100), 415 [M+ – C13H16N2] (8). HRMS (ES+) calcd for C36H36N2PSFe 615.1687; found 615.1688. (S)-2b: [α]20D = −11 (c = 0.55, CH2Cl2).
FcPPh2(=S)CH2BIm+CH3BF4−2c.
To a solution of ferrocenyl alcohol 1 (418 mg, 0.97 mmol) in dry CH2Cl2 (20 mL) was quickly added HBF4 (54 wt% in Et2O, 330 μL, 2.42 mmol), immediately followed by N-methyl benzimidazole (466 mg, 2.91 mmol). After 5 minutes, the mixture was washed with 2 M aq. HCl, water, sat. aq. NaHCO3 and water, dried (MgSO4), filtered and concentrated in vacuo. The residue was purified by precipitation with a mixture of petroleum ether and methyl tert-butyl ether (80/20) from a CH2Cl2 solution to give an orange solid (193 mg, 27% yield). X-ray quality crystals of rac-2c were obtained by slow diffusion of diethyl ether from a dichloromethane solution. 1H NMR (300 MHz, CDCl3, 298 K) δ 8.79 (1H, s, NCHN), 7.73–7.69 (3H, m, CH PPh2 + BIm), 7.55–7.47 (4H, m, CH PPh2), 7.21–7.15 (3H, m, CH PPh2), 6.95–6.93 (3H, m, CH PPh2 + BIm), 6.57 (1H, AB syst., JHH = 14 Hz, Fc-CH2), 5.23 (1H, br. s, CpA), 5.21 (1H, AB syst., JHH = 14 Hz, Fc-CH2), 4.58 (1H, br. s, CpA), 4.48 (5H, s, CpB), 3.78 (1H, br. s, CpA), 3.64 (3H, s, NCH3), 2.37 (3H, s, CH3 BIm), 2.16 (3H, s, CH3 BIm). 13C{1H} NMR (75.5 MHz, CDCl3, 298 K) δ 140.47 (NCHN), 136.95 + 136.98 (Cq BIm), 133.08 (d, JCP = 80 Hz, Cq PPh2), 131.99 (d, JCP = 86 Hz, Cq PPh2), 131.85 (d, JCP = 2 Hz, CH PPh2), 131.77 (d, JCP = 12 Hz, CH PPh2), 131.25 (d, JCP = 11 Hz, CH PPh2), 130.94 (d, JCP = 2 Hz, CH PPh2), 129.78 + 129.24 (Cq BIm), 128.34 (d, JCP = 13 Hz, CH PPh2), 127.61 (d, JCP = 12 Hz, CH PPh2), 114.22 + 111.58 (CH BIm), 82.37 (d, JCP = 13 Hz, Cq CpA), 77.24 (CH CpA), 76.38 (d, JCP = 10 Hz, CH CpA), 75.27 (d, JCP = 91 Hz, Cq CpA), 71.39 (5 CH CpB), 71.18 (d, JCP = 10 Hz, CH CpA), 45.60 (CH2-Fc), 33.37 (NCH3), 20.64 + 20.52 (CH3 BIm). 31P{1H} NMR (121.5 MHz, CDCl3, 298 K) δ 40.34. MS (ESI) m/z 575 [M+] (100), 415 [M+ − C10H12N2] (18). HRMS (ES+) calcd for C33H32N2PSFe 575.1374; found 575.1367. (S)-2c: [α]20D = −81 (c = 0.54, CH2Cl2).
FcPPh2(=S)CH2BIm+CH2MesBF4−2d.
To a solution of ferrocenyl alcohol 1 (474 mg, 1.09 mmol) in dry CH2Cl2 (20 mL) was quickly added HBF4 (54 wt% in Et2O, 380 μL, 2.75 mmol, 2.5 equiv.), immediately followed by N-2,4,6 trimethylbenzyl benzimidazole (800 mg, 2.88 mmol, 2.7 equiv.). After 5 minutes, the mixture was washed with 2 M aq. HCl, water, sat. aq. NaHCO3 and water, dried (MgSO4), filtered and concentrated in vacuo. The residue was purified by precipitation with a mixture of petroleum ether and methyl tert-butyl ether (80/20) from a CH2Cl2 solution, followed by column chromatography on silica gel (eluents: diethyl ether, then CH2Cl2, then CH2Cl2–Acetone 90/10) to give an orange solid (481 mg, 57% yield). X-ray quality crystals of rac-2d were obtained by slow diffusion of diethyl ether in a dichloromethane solution. 1H NMR (500 MHz, CDCl3, 301 K) δ 8.68 (1H, s, NCHN), 7.72–7.68 (3H, m, CH PPh2 + BIm), 7.58–7.55 (1H, m, CH PPh2), 7.51–7.47 (2H, m, CH PPh2), 7.22–7.18 (1H, m, CH PPh2), 7.07–7.00 (3H, m, CH PPh2 + BIm), 6.94 (2H, s, CH Mes), 6.90–6.85 (2H, m, CH PPh2), 6.48 (1H, AB syst., Fc-CH2), 5.51 (1H, AB syst., Fc-CH2), 5.40 (1H, br, CpA), 5.32 (1H, AB syst., CH2-Mes), 4.98 (1H, AB syst., CH2-Mes), 4.57 (1H, br s, CpA), 4.42 (5H, s, CpB), 3.81 (1H, s, CpA), 2.34–2.33 (9H, m, CH3 BIm + p-CH3Mes), 2.17 (6H, s, o-CH3 Mes). 13C NMR{1H} (125.8 MHz, CDCl3, 301K) δ 139.97 (Cqp-CH3C Mes), 139.51 (NCHN), 137.94 (Cqo-CH3C Mes), 137.23 + 137.20 (Cq CH3C BIm), 134.01 (d, JCP = 86 Hz, Cq PPh2), 132.00 (d, JCP = 11 Hz, CH PPh2), 131.99 (d, JCP = 87 Hz, Cq PPh2), 131.92 (d, JCP = 3 Hz, CH PPh2), 131.20 (d, JCP = 3 Hz, CH PPh2), 130.83 (d, JCP = 11 Hz, CH PPh2), 130.07 (CH Mes), 129.72 + 129.60 (Cq BIm), 128.66 (d, JCP = 13 Hz, CH PPh2), 127.75 (d, JCP = 12 Hz, CH PPh2), 124.95 (Cq NCH2C Mes), 114.79 + 112.33 (CH BIm), 83.05 (d, JCP = 12 Hz, Cq CpA), 77.28 (CH CpA, seen by HSQC{31P}), 76.12 (d, JCP = 12 Hz, CH CpA), 73.91 (d, JCP = 94 Hz, Cq CpA), 71.59 (CH CpB), 71.17 (d, JCP = 10 Hz, CH CpA), 46.72 (CH2-Fc), 45.77 (CH2-Mes), 21.10 (p-CH3 Mes), 20.73 + 20.69 (CH3 BIm), 20.03 (o-CH3 Mes). 31P{1H} NMR (202.5 MHz, CDCl3, 301 K) δ 40.88. MS (ESI) m/z 693 [M+] (100). HRMS (ES+) calcd for C42H42N2PSFe 693.2156; found 693.2164. (S)-2d: [α]20D = −158 (c = 0.53, CH2Cl2).
General procedure for the synthesis of (benz)imidazolium salts FcPPh2CH2Im/BIm+RBF4−3b–d
RANEY® nickel (50 to 100 equivalents) was washed with degassed methanol (3 × 10 mL), degassed diethyl ether (3 × 10 mL), and finally degassed acetonitrile (3 × 10 mL). A solution of (benz)imidazolium salt 2b–d in acetonitrile (20 mL) was added and the mixture was heated at 40 °C for 20 h. After cooling to room temperature, the supernatant was filtered through a short path of Celite® and the RANEY® nickel was rinsed with acetonitrile. The filtrate was concentrated and the solid residue was dried in vacuo.
FcPPh2CH2Im+CH2MesBF4−3b.
Yellow solid (220 mg, 88% yield). 1H NMR (300 MHz, CDCl3, 298 K) δ 8.73 (1H, s, NCHN), 7.49 (2H, m, CH PPh2), 7.39 (3H, m, CH PPh2), 7.16 (3H, m, CH PPh2), 6.97 (2H, m, CH PPh2), 6.87 (2H, br s, CH Mes), 6.82 (1H, br s, NCH = CH), 6.42 (1H, br s, NCH = CH), 5.50 (1H, AB syst., JHH = 15 Hz, CH2-Fc), 5.37 (1H, AB syst., JHH = 15 Hz, CH2-Fc), 5.09 (2H, s, CH2-Mes), 4.93 (1H, br s, CH CpA), 4.48 (1H, br s, CH CpA), 4.11 (5H, s, CH CpB), 3.92 (1H, br s, CH CpA), 2.27 (3H, s, p-CH3 Mes), 2.01 (6H, s, o-CH3 Mes). 13C{1H} NMR (75.5 MHz, CDCl3, 298 K) δ 139.98 (Cq Mes), 138.94 (d, JCP = 7 Hz, Cq PPh2), 138.00 (Cq Mes), 135.56 (d, JCP = 7 Hz, Cq PPh2), 135.10 (NCHN), 134.71 (d, JCP = 21 Hz, CH PPh2), 132.19 (d, JCP = 19 Hz, CH PPh2), 129.89 (CH Mes), 129.68 (CH Mes), 128.43 (d, JCP = 8 Hz, CH PPh2), 128.32 (d, JCP = 7 Hz, CH PPh2), 124.93 (Cq Mes), 121.48 (NCH = CH), 120.06 (NCH = CH), 84.23 (d, JCP = 28 Hz, Cq CpA), 76.92 (d, JCP = 9 Hz, Cq CpA), 73.73 (CH CpA), 72.93 (d, JCP = 4 Hz, CH CpA), 71.52 (CH CpA), 70.25 (CH CpB), 49.00 (d, JCP = 11 Hz, CH2-Fc), 47.40 (CH2-Mes), 22.63 (p-CH3 Mes), 21.01 (o-CH3 Mes). 31P{1H} NMR (121.5 MHz, CDCl3, 298 K): δ −27.18. MS (ESI) m/z 583 [M+] (85) 383 [M+ − C13H16N2] (100). HRMS (ES+) calcd for C36H36N2PFe 583.1966; found 583.1962. (S)-3b: [α]20D = −161 (c = 0.51, CH2Cl2).
FcPPh2CH2BIm+MeBF4−3c.
Orange solid (164 mg, 90% yield). 1H NMR (300 MHz, CDCl3, 298 K) δ 8.75 (1H, s, NCHN), 7.51 (1H, s, CH BIm), 7.46–7.37 (4H, m, CH PPh2), 7.28 (1H, s, CH PPh2), 7.08 (1H, s, CH BIm), 7.01 (1H, m, CH PPh2), 6.83 (2H, m, CH PPh2), 6.65 (2H, m, CH PPh2), 5.79 (1H, AB syst., JHH = 15 Hz, CH2-Fc), 5.34 (1H, AB syst., JHH = 15 Hz, CH2-Fc), 5.06 (1H, br s, CH CpA), 4.50 (1H, br s, CH CpA), 4.22 (5H, s, CH CpB), 3.84 (1H, br s, CpA), 3.67 (3H, s, N-CH3), 2.37 (6H, br s, CH3 BIm). 13C{1H} NMR (75 MHz, CDCl3, 298 K) δ 140.35 (NCHN), 138.10 (d, JCP = 7 Hz, Cq PPh2), 136.92 (Cq CH3C BIm), 136.82 (Cq CH3C BIm), 135.28 (d, JCP = 7.5 Hz, Cq PPh2), 134.62 (d, JCP = 20 Hz, CH PPh2), 131.81 (d, JCP = 19 Hz, CH PPh2), 130.93 (Cq BIm), 129.94 (Cq BIm), 129.55 (CH PPh2), 128.38 (d, JCP = 8 Hz, CH PPh2), 127.88 (CH PPh2), 127.65 (d, JCP = 6.5 Hz, CH PPh2), 113.11 (CH BIm), 111.89 (CH BIm), 83.97 (Cq CpA), 77.21 (Cq CpA), 74.30 (CH CpA), 72.94 (CH CpA), 71.06 (CH CpA), 70.30 (CH CpB), 46.26 (d, JCP = 9 Hz, CH2-Fc), 32.93 (CH3-N), 20.61 + 20.47 (CH3 BIm). 31P{1H} NMR (121.5 MHz, CDCl3, 298K) δ −26.43. MS (ESI) m/z 543 [M+] (100), 575 [M+ + S] (70), 383 [M+ − C10H12N2] (65). HRMS (ES+) calcd for C33H32N2PFe 543.1699; found 543.1662. (S)-3c: [α]20D = −201 (c = 0.55, CH2Cl2).
FcPPh2CH2BIm+CH2MesBF4−3d.
Yellow solid (500 mg, 82% yield). 1H NMR (500 MHz, CDCl3, 301 K) δ 8.90 (1H, s, NCHN), 7.50–7.47 (3H, m, CH BIm + PPh2), 7.44–7.38 (3H, m, CH PPh2), 6.98 (1H, t, JHH = 7.5 Hz, CH PPh2), 6.94 (2H, s, CH Mes), 6.81 (2H, t, JHH = 7.5 Hz, CH PPh2), 6.79 (1H, s, CH BIm), 6.63–6.60 (2H, m, CH PPh2), 5.78 (1H, dd, JHH = 14.9 Hz, JPH = 1.8 Hz, CH2-Fc), 5.57 (1H, AB syst., JHH = 14.9 Hz, CH2-Fc), 5.33 (1H, AB syst., JHH = 14.7 Hz, CH2-Mes), 5.26 (1H, AB syst., JHH = 14.6 Hz, CH2-Mes), 5.08 (1H, br. s, CH CpA), 4.49 (1H, br. s, CH CpA), 4.13 (5H, s, CH CpB), 3.88 (1H, br. s, CH CpA), 2.33 (3H, s, p-CH3 Mes), 2.23 (6H, s, CH3 BIm), 2.17 (6H, s, o-CH3 Mes). 13C{1H} NMR (125.8 MHz, CDCl3, 301 K) δ 139.88 (Cqp-CH3C Mes), 139.83 (d, JCP = 1.8 Hz, NCHN), 139.11 (d, JCP = 8 Hz, Cq PPh2), 138.00 (Cqo-CH3C Mes), 136.81 (Cq CH3C BIm), 136.10 (d, JCP = 6 Hz, Cq PPh2), 135.02 (d, JCP = 21 Hz, o-CH PPh2), 131.28 (d, JCP = 18 Hz, o-CH PPh2), 130.04 (CH Mes), 129.70 (d, p-CH PPh2), 129.68 (Cq BIm), 129.45 (d, p-CH PPh2), 128.34 (d, JCP = 8 Hz, m-CH PPh2), 127.58 (d, JCP = 6 Hz, m-CH PPh2), 127.48 (Cq BIm), 124.65 (Cq NCH2C Mes), 113.62 (d, JCP = 5 Hz, CH BIm), 112.46 (CH BIm), 85.17 (d, JCP = 28 Hz, Cq CpA), 76.21 (d, JCP = 9 Hz, Cq CpA), 73.82 (d, JCP = 3.7 Hz, CH CpA), 72.79 (d, JCP = 4.5 Hz, CH CpA), 71.39 (CH CpA), 70.35 (CH CpB), 46.53 (dd, JCP = 9.6 Hz, CH2-Fc), 46.28 (d, CH2-Mes), 21.07 (p-CH3 Mes), 20.59 + 20.46 (CH3 BIm), 19.75 (o-CH3 Mes). 31P{1H} NMR (202.5 MHz, CDCl3, 301 K) δ −25.68. MS (ESI) m/z 677 [M+ + O] (10), 661 [M+] (100), 383 [M+ − C19H22N2] (10). HRMS (ES+) calcd for C42H42N2PFe 661.2435; found 661.2443. (S)-3d: [α]20D = −224 (c = 0.55, CH2Cl2).
General procedure for the synthesis of PdCl2[L] complexes 4b–d
A mixture of PdCl2(PhCN)2 (1 equiv.), FcPPh2CH2Im/BIm+RBF4−3b–d (50 mg, 1 equiv.) and NaOtBu (1.1 equiv.) in dry CH3CN (50 mL) was heated at 50 °C for 16 h. The solution was allowed to cool to room temperature, concentrated in vacuo and the residue was purified by flash chromatography on silica gel (eluent: CH2Cl2/MeOH 9/1).
4b: orange solid (35 mg, 61% yield). X-ray quality crystals of (S)-4b were obtained by slow diffusion of petroleum ether in a solution of dichloromethane. 1H NMR (500 MHz, CDCl3, 298 K) δ 7.92 (2H, m, CH PPh2), 7.51 (1H, m, CH PPh2), 7.44 (2H, m, CH PPh2), 7.41 (1H, m, CH PPh2), 7.32 (2H, m, CH PPh2), 7.18 (2H, m, CH PPh2), 6.79 (1H, s, NCH = CH), 6.78 (2H, s, CH Mes), 6.54 (1H, AB syst., JHH = 15 Hz, CH2-Fc), 5.97 (1H, s, NCH = CH), 5.94 (1H, AB syst., JHH = 15 Hz, CH2-Mes), 4.75 (1H, AB syst., JHH = 15 Hz, CH2-Fc), 4.74 (1H, br s, CH CpA), 4.42 (1H, br s, CH CpA), 4.28 (5H, s, CH CpB), 4.00 (1H, AB syst., JHH = 15 Hz, CH2-Mes), 3.70 (1H, br s, CH CpA), 2.24 (3H, s, p-CH3 Mes), 1.91 (6H, s, o-CH3 Mes). 13C{1H} NMR (125.8 MHz, CDCl3, 298 K) δ 156 ppm (Cq NCN-Pd, determined by HMBC), 139.03 (2 Cq Mes, C-o-CH3), 138.72 (Cq Mes, C-p-CH3), 135.75 (d, JCP = 10 Hz, CH PPh2), 134.46 (d, JCP = 54 Hz, Cq PPh2), 131.33 (d, JCP = 11 Hz, CH PPh2), 131.16 (CH PPh2), 130.68 (CH PPh2), 129.18 (CH Mes), 128.86 (d, JCP = 54 Hz, Cq PPh2), 128.71 (d, JCP = 11 Hz, CH PPh2), 127.39 (d, JCP = 11 Hz, CH PPh2), 126.78 (Cq Mes, C-CH2), 121.00 (NCH = CH), 119.00 (NCH = CH), 86.99 (d, JCP = 14 Hz, Cq CpA), 74.85 (CH CpA), 72.90 (d, JCP = 56 Hz, CH CpA), 71.83 (d, JCP = 58 Hz, Cq CpA), 71.43 (5 CH CpB), 70.13 (CH CpA), 50.08 (CH2-Fc), 48.03 (CH2-Mes), 20.92 (p-CH3 Mes), 20.60 (o-CH3 Mes). 31P{1H} NMR (202.5 MHz, CDCl3, 298 K) δ 4.56. MS (ESI) m/z 723 [M – Cl−] (100), 242 [PdCl2Im] (80). HRMS (ES+) calcd for C36H35N2PFePdCl 723.0623; found 723.0652. (S)-4b: [α]20D = −5 (c = 0.5, CH2Cl2).
4c: orange solid (30 mg, 54% yield). X-ray quality crystals of (S)-4c were obtained by slow diffusion of acetone in a solution of dichloromethane. 1H NMR (500 MHz, CD2Cl2, 298 K) δ 8.08 (2H, m, CH o-PPh2), 7.61 (1H, m, CH p-PPh2), 7.52 (2H, m, CH m-PPh2), 7.28 (1H, m, CH p-PPh2), 7.20 (1H, s, CH BIm), 7.06 (2H, m, CH o-PPh2), 6.83 (1H, s, CH BIm), 6.76 (2H, m, CH m-PPh2), 6.64 (1H, AB syst., JHH = 15 Hz, CH2-Fc), 5.21 (1H, AB syst., JHH = 15 Hz, CH2-Fc), 4.94 (1H, br s, CH CpA), 4.43 (1H, br s, CH CpA), 4.42 (1H, br s, CH CpB), 3.62 (3H, s, N-CH3), 2.38 (3H, s, CH3-BIm), 2.32 (3H, s, CH3-BIm). 13C{1H} NMR (125.8 MHz, CD2Cl2, 298 K) δ 166.70 (Cq NCN-Pd), 136.02 (d, JCP = 10 Hz, o-CH PPh2), 134.31 (d, JCP = 55 Hz,Cq PPh2), 133.49 (Cq BIm), 133.10 (2 Cq CH3-C BIm), 131.40 (d, JCP = 2.5 Hz, p-CH PPh2), 130.90 (Cq BIm), 130.65 (d, JCP = 11 Hz, o-CH PPh2), 130.00 (d, JCP = 2.5 Hz, p-CH PPh2), 128.34 (d, JCP = 10 Hz, m-CH PPh2), 127.58 (d, JCP = 54 Hz, Cq PPh2), 127.48 (d, JCP = 11 Hz, m-CH PPh2), 110.45 (CH BIm), 109.71 (CH BIm), 86.80 (d, JCP = 14 Hz, Cq CpA), 74.23 (d, JCP = 2.5 Hz, CH CpA), 73.51 (d, JCP = 8 Hz, CH CpA), 71.36 (5 CH CpB), 71.00 (d, JCP = 58 Hz, Cq CpA), 70.39 (d, JCP = 6 Hz, CH CpA), 46.15 (CH2-Fc), 33.60 (N-CH3), 20.04 (CH3 BIm), 19.95 (CH3 BIm). 31P{1H} NMR (202.5 MHz, CD2Cl2, 298 K) δ 4.38. MS (ESI) m/z 683 [M – Cl−] (100), 647 [M – 2Cl−] (33). HRMS (ES+) calcd for C33H31N2PFePdCl 683.0309; found 683.0313. (S)-4c: [α]20D = −59 (c = 0.54, CH2Cl2).
4d: yellow solid (20 mg, 35% yield). X-ray quality crystals of rac-4d were obtained by slow diffusion of diethyl ether in a solution of dichloromethane. 1H NMR (500 MHz, CDCl3, 298 K) δ 8.00 (2H, m, CH PPh2), 7.52–7.46 (2H, m, CH PPh2), 7.31–7.29 (2H, m, CH PPh2), 7.12 (1H, m, CH BIm), 6.96–6.94 (2H, m, CH PPh2), 6.77 (2H, s, CH PPh2), 6.78 (2H, 2s, CH Mes), 6.77 (1H, AB syst., JHH = 15 Hz, Fc-CH2), 6.28 (1H, AB syst., JHH = 15 Hz, CH2-Mes), 5.41 (1H, s, CH BIm), 5.14 (1H, AB syst., JHH = 15 Hz, Fc-CH2), 4.85 (1H, br. s, CpA), 4.40 (1H, br. s, CpA), 4.32 (5H, s, CpB), 4.31 (1H, AB syst., JHH = 15 Hz, CH2-Mes), 3.65 (1H, br. s, CpA), 2.29 (6H, s, CH3 BIm), 1.95 (6H, s, o-CH3 Mes), 1.91 (3H, s, p-CH3 Mes). 13C{1H} NMR (125.8 MHz, CDCl3, 298 K) δ 166.74 (Cq NCN-Pd), 138.47 (Cq BIm), 135.93 (d, JCP = 10 Hz, o-CH PPh2), 134.19 (d, JCP = 54 Hz, Cq PPh2), 133.49 (Cq BIm), 132.45 (Cq CH3-C BIm), 132.34 (Cq CH3-C BIm), 131.47 (Cq Mes, C-p-CH3), 131.18 (Cq Mes, C-o-CH3), 131.02 (d, JCP = 11 Hz, CH PPh2), 129. 89 (Cq Mes, C-o-CH3), 129.10 (CH Mes), 128.79 (d, JCP = 55 Hz, Cq PPh2), 127.43 (d, JCP = 11 Hz, CH PPh2), 128.49 (d, JCP = 10 Hz, CH PPh2), 126.84 (CH Mes), 112.45 (CH BIm), 109.23 (CH BIm), 86.52 (d, JCP = 14 Hz, Cq CpA), 74.42 (CH CpA), 72.05 (Cq, CpA), 72.93 (d, JCP = 1.5 Hz, CH CpA), 71.39 (5 CH,CpB), 70.16 (d, JCP = 1.5 Hz, CH CpA), 49.00 (CH2-Mes), 46.52 (CH2-Fc), 21.12 (o-CH3 Mes), 20.92 (CH3 BIm), 20.37 (p-CH3 Mes), 20.26 (CH3 BIm). 31P{1H} NMR (202.5 MHz, CDCl3, 298 K) δ 3.86. MS (DCI-CH4) m/z 801 [M – Cl] (100), 767 [M – 2Cl] (58). HRMS (Cl+) calcd for C42H41N2PFePdCl 801.1080; found 801.1118. (S)-4d: [α]20D = −32 (c = 0.51, CH2Cl2).
General procedure for Suzuki–Miyaura coupling reactions
In a typical run, a mixture of naphthyl bromide (0.29 mmol, 1 equiv.), naphthylboronic acid (0.34 mmol, 1.2 equiv.), potassium carbonate (0.69 mmol, 2.4 equiv.) and catalyst (0.5 mol%) in 2 mL of toluene (+50 μL of CH2Cl2 for complete solubilisation) was stirred for 24 h at 40 °C. The solution was allowed to cool to room temperature, washed with water and the aqueous phase was extracted with diethyl ether. The organic phase was dried over MgSO4, filtered and concentrated in vacuo. The residue was purified by flash chromatography on silica gel (hexane or hexane/ethyl acetate 9/1). Enantiomeric excesses were determined by HPLC with a Chiralcel OJ column (eluent: Hexane/iPrOH 9/1, rate: 1 mL min−1, T = 30 °C). Retention time for 2-methylbinaphthyl: 6.42 min (R isomer)–9.93 min (S isomer). Retention time for 2-methoxybinaphthyl: 9.22 min (S isomer)–15.66 min (R isomer). Retention time for 2-ethoxybinaphthyl: 7.66 min (S isomer)–11.90 min (R isomer).
General procedure for 1,2-addition reactions
A mixture of 4-nitrobenzaldehyde (0.2 mmol, 1 equiv.), phenylboronic acid (0.4 mmol, 2 equiv. (THF) or 0.3 mmol, 1.5 equiv. (other solvents)), base (0.6 mmol, 3 equiv. (THF) or 0.4 mmol, 2 equiv. (other solvents)) and catalyst (5 mol%) in a solvent (THF: 5 mL, other solvents: 1 mL) was stirred for 24 h at 65 °C. After cooling to room temperature, water was added and the aqueous phase was extracted with tBuOMe. The organic phases were washed with brine, dried (MgSO4), filtered and concentrated in vacuo. After analysis by 1H NMR (d6-acetone), the residue was purified by flash chromatography on silica gel (petroleum ether/EtOAc 8/1 then 4/1) to give pure (4-nitrophenyl)(phenyl)methanol as a yellow solid. It was analysed by chiral HPLC using a Chiracel AD-H column, (eluent: Hexane/iPrOH 80/20, flow rate = 0.5 mL min−1, wavelength = 214 nm, 13.9 min (R isomer) and 16.4 min (S isomer)).
X-ray structural analyses
A single crystal of each compound was mounted under inert perfluoropolyether at the tip of a glass fiber and cooled in the cryostream of either an Oxford-Diffraction XCALIBUR CCD diffractometer for rac-2c and (S)-4c, a Bruker APEXII diffractometer for rac-2d and (S)-4b or an Agilent Technologies GEMINI EOS diffractometer for rac-4d.
The structures were solved by direct methods (SIR9728) and refined by least-squares procedures on F2 using SHELXL-97.29 All H atoms attached to carbon were introduced in calculation in idealised positions and treated as riding models. In four of the five compounds, rac-2d, (S)-4b, (S)-4c and rac-4d, it was difficult to locate some disordered solvents, and the SQUEEZE option from PLATON30 has been used. The absolute configuration for compounds (S)-4b and (S)-4c has been determined by refining the Flack's parameter.31 The drawing of the molecules was realised with the help of ORTEP32.32
Acknowledgements
We thank the CNRS, the Ministère de l'Enseignement Supérieur et de la Recherche (doctoral grant to P.L.), the Institut Universitaire de France (grant to S.G.) and the Agence Nationale de la Recherche (ANR-07-JCJC-0041, postdoctoral grant to N.D.) for financial support of this work.
Notes and references
- Selected reviews: H. M. Lee, C. C. Lee and P. Y. Cheng, Curr. Org. Chem., 2007, 11, 1491 CrossRef CAS; O. Kühl, Chem. Soc. Rev., 2007, 36, 592 RSC; A. T. Normand and K. J. Cavell, Eur. J. Inorg. Chem., 2008, 2781 CrossRef; A. John and P. Ghosh, Dalton Trans., 2010, 39, 7183 RSC.
- NHC-P, selected papers: C. Yang, H. M. Lee and S. P. Nolan, Org. Lett., 2001, 3, 1511 CrossRef CAS PubMed; N. Tsoureas, A. A. Danopoulos, A. A. D. Tulloch and M. E. Light, Organometallics, 2003, 22, 4750 CrossRef; H. Seo, H.-J. Park, B. Y. Kim, J. H. Lee, S. U. Son and Y. K. Chung, Organometallics, 2003, 22, 618 CrossRef; E. Bappert and G. Helmchen, Synlett, 2004, 1789 Search PubMed; L. D. Field, B. A. Messerle, K. Q. Vuong and P. Turner, Organometallics, 2005, 24, 4241 CrossRef; F. E. Hahn, M. C. Jahnke and T. Pape, Organometallics, 2006, 25, 5927 CrossRef; F. Visentin and A. Togni, Organometallics, 2007, 26, 3746 CrossRef; C.-C. Lee, W.-C. Ke, K.-T. Chan, C.-L. Lai, C.-H. Hu and H. M. Lee, Chem.–Eur. J., 2007, 13, 582 CrossRef PubMed; C.-C. Ho, S. Chatterjee, T.-L. Wu, K.-T. Chan, Y.-W. Chang, T.-H. Hsiao and H. M. Lee, Organometallics, 2009, 28, 2837 CrossRef; E. Kühnel, I. V. Shishkov, F. Rominger, T. Oeser and P. Hofmann, Organometallics, 2012, 31, 8000 CrossRef; S. Gaillard and J.-L. Renaud, Dalton Trans., 2013, 42, 7255 RSC.
- M. Bierenstiel and E. D. Cross, Coord. Chem. Rev., 2011, 255, 574 CrossRef CAS PubMed and references therein; C. Fliedel and P. Braunstein, Organometallics, 2010, 29, 5614 CrossRef; H. V. Huynh, D. Yuan and Y. Han, Dalton Trans., 2009, 7262 RSC; D. Krishnan, S. A. Pullarkat, M. Wu, Y. Li and P.-H. Leung, Chem.–Eur. J., 2013, 19, 5468 CrossRef PubMed; Y.-H. Chang, Z.-Y. Liu, Y.-H. Liu, S.-M. Peng, J.-T. Chen and S.-T. Liu, Dalton Trans., 2011, 40, 489 RSC; C. Lohre, T. Dröge, C. Wang and F. Glorius, Chem.–Eur. J., 2011, 17, 6052 CrossRef PubMed; H. V. Huynh and Q. Teng, Chem. Commun., 2013, 49, 4244 RSC; Y.-C. Lin, H.-H. Hsueh, S. Kanne, L.-K. Chang, F.-C. Liu, I. J. B. Lin, G.-H. Lee and S.-M. Peng, Organometallics, 2013, 32, 3859 CrossRef.
- J. Wolf, A. Labande, M. Natella, J.-C. Daran and R. Poli, J. Mol. Catal. A: Chem., 2006, 259, 205 CrossRef CAS PubMed; J. Wolf, A. Labande, J.-C. Daran and R. Poli, Eur. J. Inorg. Chem., 2007, 5069 CrossRef; A. Labande, N. Debono, A. Sournia-Saquet, J.-C. Daran and R. Poli, Dalton Trans., 2013, 42, 6531 RSC.
- A. Labande, J.-C. Daran, E. Manoury and R. Poli, Eur. J. Inorg. Chem., 2007, 1205 CrossRef CAS.
- S. Gülcemal, A. Labande, J.-C. Daran, B. Çetinkaya and R. Poli, Eur. J. Inorg. Chem., 2009, 1806 CrossRef.
- N. Debono, A. Labande, E. Manoury, J.-C. Daran and R. Poli, Organometallics, 2010, 29, 1879 CrossRef CAS.
-
S. Díez-González, N-Heterocyclic Carbenes: From Laboratory Curiosities to Efficient Synthetic Tools, RSC Publishing, Cambridge, UK, 2011 CrossRef CAS PubMed;
F. Glorius, N-Heterocyclic Carbenes in Transition Metal Catalysis, Springer-Verlag, Heidelberg, Germany, 2007 CrossRef CAS PubMed; D. Bourissou, O. Guerret, F. P. Gabbaï and G. Bertrand, Chem. Rev., 2000, 100, 39 CrossRef CAS PubMed; F. E. Hahn and M. C. Jahnke, Angew. Chem., Int. Ed., 2008, 47, 3122 CrossRef PubMed; S. Díez-González, N. Marion and S. P. Nolan, Chem. Rev., 2009, 109, 3612 CrossRef PubMed; J. C. Y. Lin, R. T. W. Huang, C. S. Lee, A. Bhattacharyya, W. S. Hwang and I. J. B. Lin, Chem. Rev., 2009, 109, 3561 CrossRef PubMed; P. L. Arnold and I. J. Casely, Chem. Rev., 2009, 109, 3599 CrossRef PubMed ; chiral NHCs: V. César, S. Bellemin-Laponnaz and L. H. Gade, Chem. Soc. Rev., 2004, 33, 619 RSC.
- General reviews on Suzuki-Miyaura cross-coupling reaction:
N. Miyaura, in Metal-Catalyzed Cross-Coupling Reactions, ed. A. de Meijere and F. Diederich, Wiley-VCH, Weinheim, Germany, 2nd edn, 2004, ch. 2, pp. 41–123 CrossRef CAS; N. Miyaura and A. Suzuki, Chem. Rev., 1995, 95, 2457 CrossRef CAS; G. A. Molander and B. Canturk, Angew. Chem., Int. Ed., 2009, 48, 9240 CrossRef PubMed; A. Suzuki, Angew. Chem., Int. Ed., 2011, 50, 6722 CrossRef PubMed; G. C. Fortman and S. P. Nolan, Chem. Soc. Rev., 2011, 40, 5151 RSC.
- D. Ortiz, M. Blug, X.-F. Le Goff, P. Le Floch, N. Mézailles and P. Maître, Organometallics, 2012, 31, 5975 CrossRef CAS; R. J. Lundgren and M. Stradiotto, Chem.–Eur. J., 2012, 18, 9758 CrossRef PubMed; S. Doherty, J. G. Knight, N. A. B. Ward, D. M. Bittner, C. Wills, W. McFarlane, W. Clegg and R. W. Harrington, Organometallics, 2013, 32, 1773 CrossRef.
- X. Shen, G. O. Jones, D. A. Watson, B. Bhayana and S. L. Buchwald, J. Am. Chem. Soc., 2010, 132, 11278 CrossRef CAS PubMed.
- O. Baudoin, Eur. J. Org. Chem., 2005, 4223 CrossRef CAS and references therein; M. Genov, A. Almorin and P. Espinet, Chem.–Eur. J., 2006, 12, 9346 CrossRef PubMed; Y. Uozumi, Y. Matsuura, T. Arakawa and Y. M. A. Yamada, Angew. Chem., Int. Ed., 2009, 48, 2708 CrossRef PubMed; T. Yamamoto, Y. Akai, Y.-U. Nagata and M. Suginome, Angew. Chem., Int. Ed., 2011, 50, 8844 CrossRef PubMed; T. Kamei, A. H. Sato and T. Iwasawa, Tetrahedron Lett., 2011, 52, 2638 CrossRef PubMed; X. Urbaneja, A. Mercier, C. Besnard and E. P. Kündig, Chem. Commun., 2011, 47, 3739 RSC; A. Ros, B. Estepa, A. Bermejo, E. Alvarez, R. Fernandez and J. M. Lassaletta, J. Org. Chem., 2012, 77, 4740 CrossRef PubMed; W. Wu, S. Wang, Y. Zhou, Y. He, Y. Zhuang, L. Li, P. Wan, L. Wang, Z. Zhou and L. Qiu, Adv. Synth. Catal., 2012, 354, 2395 CrossRef; A. Balanta Castillo, B. F. Perandones, E. Zangrando, S. Gladiali, C. Godard and C. Claver, J. Organomet. Chem., 2013, 743, 31 CrossRef PubMed.
- A. N. Cammidge and K. V. L. Crépy, Chem. Commun., 2000, 1723 RSC; J. Yin and S. L. Buchwald, J. Am. Chem. Soc., 2000, 122, 12051 CrossRef CAS.
- W. Tang, N. D. Patel, G. Xu, X. Xu, J. Savoie, S. Ma, M.-H. Hao, S. Keshipeddy, A. G. Capacci, X. Wei, Y. Zhang, J. J. Gao, W. Li, S. Rodriguez, B. Z. Lu, N. K. Yee and C. H. Senanayake, Org. Lett., 2012, 14, 2258 CrossRef CAS PubMed.
- NHC ligands in asymmetric Suzuki-Miyaura: G. Shigeng, J. Tang, D. Zhang, Q. Wang, Z. Chen and L. Weng, J. Organomet. Chem., 2012, 700, 223 CrossRef CAS PubMed; L. Wu, A. Salvador, A. Ou, M. W. Shi, B. W. Skelton and R. Dorta, Synlett, 2013, 1215 Search PubMed.
- S. Gibson, D. F. Foster, G. R. Eastham, R. P. Tooze and D. J. Cole-Hamilton, Chem. Commun., 2001, 779 RSC; T. Yamamoto, T. Ohta and Y. Ito, Org. Lett., 2005, 7, 4153 CrossRef CAS PubMed; K. Suzuki, T. Arao, S. Ishii, Y. Madea, K. Kondo and T. Aoyama, Tetrahedron Lett., 2006, 47, 5789 CrossRef PubMed; C. Qin, H. Wu, J. Cheng, X. Chen, M. Liu, W. Zhang, W. Su and J. Ding, J. Org. Chem., 2007, 72, 4102 CrossRef PubMed; S. Lin and X. Lu, J. Org. Chem., 2007, 72, 9757 CrossRef PubMed; P. He, Y. Lu, C.-G. Dong and Q.-S. Hu, Org. Lett., 2007, 9, 343 CrossRef PubMed; M. Kuriyama, R. Shimazawa and R. Shirai, J. Org. Chem., 2008, 73, 1597 CrossRef PubMed.
- A. Novodomskà, M. Dudičovà, F. R. Leroux and F. Colobert, Tetrahedron: Asymmetry, 2007, 18, 1628 CrossRef PubMed.
- Y. Suzuma, S. Hayashi, T. Yamamoto, Y. Oe, T. Ohta and Y. Ito, Tetrahedron: Asymmetry, 2009, 20, 2751 CrossRef CAS PubMed.
- R. Zhang, Q. Xu, X. Zhang, T. Zhang and M. Shi, Tetrahedron: Asymmetry, 2010, 21, 1928 CrossRef CAS PubMed.
- F. E. Hahn, L. Wittenbecher, R. Boese and D. Bläser, Chem.–Eur. J., 1999, 5, 1931 CrossRef CAS; O. Buisine, G. Berthon-Gelloz, J.-F. Brière, S. Stérin, G. Mignani, P. Branlard, B. Tinant, J.-P. Declercq and I. E. Markó, Chem. Commun., 2005, 3856 RSC; C. J. O'Brien, E. A. B. Kantchev, G. A. Chass, N. Hadei, A. C. Hopkinson, M. G. Organ, D. H. Setiadi, T.-H. Tang and D.-C. Fang, Tetrahedron, 2005, 61, 9723 CrossRef PubMed.
- F. E. Hahn, M. C. Jahnke and T. Pape, Organometallics, 2006, 25, 5927 CrossRef CAS.
- N. Tsoureas, A. A. Danopoulos, A. A. D. Tulloch and M. E. Light, Organometallics, 2003, 22, 4750 CrossRef CAS; H. M. Lee, P. L. Chiu and J. Y. Zeng, Inorg. Chim. Acta, 2004, 357, 4313 CrossRef PubMed.
- O. Navarro, H. Kaur, P. Mahjoor and S. P. Nolan, J. Org. Chem., 2004, 69, 3173 CrossRef CAS PubMed; O. Navarro, N. Marion, Y. Oonishi, R. A. Kelly and S. P. Nolan, J. Org. Chem., 2006, 71, 685 CrossRef PubMed; S. Berardi, M. Carraro, M. Iglesias, A. Sartorel, G. Scorrano, M. Albrecht and M. Bonchio, Chem.–Eur. J., 2010, 16, 10662 CrossRef PubMed.
- F. Schmidt, R. T. Stemmler, J. Rudolph and C. Bolm, Chem. Soc. Rev., 2006, 35, 454 CAS.
- M. Sakai, M. Ueda and N. Miyaura, Angew. Chem., Int. Ed., 1998, 37, 3279 CrossRef CAS.
- H.-F. Duan, J.-H. Xie, W.-J. Shi, Q. Zhang and Q.-L. Zhou, Org. Lett., 2006, 8, 1479 CrossRef CAS PubMed; T. Nishimura, H. Kumamoto, M. Nagaosa and T. Hayashi, Chem. Commun., 2009, 5713 RSC; S. Morikawa, K. Michigami and H. Amii, Org. Lett., 2010, 12, 2520 CrossRef PubMed.
- L. Routaboul, S. Vincendeau, J.-C. Daran and E. Manoury, Tetrahedron: Asymmetry, 2005, 16, 2685 CrossRef CAS PubMed; N. Mateus, L. Routaboul, J.-C. Daran and E. Manoury, J. Organomet. Chem., 2006, 691, 2297 CrossRef PubMed.
- A. Altomare, M. C. Burla, M. Camalli, G. L. Cascarano, C. Giacovazzo, A. Guagliardi, A. G. G. Moliterni, G. Polidori and R. Spagna, J. Appl. Crystallogr., 1999, 32, 115 CrossRef CAS.
- G. M. Sheldrick, Acta Crystallogr., Sect. A: Found. Crystallogr., 2008, 64, 112 CrossRef CAS PubMed.
- A. L. Spek, J. Appl. Crystallogr., 2003, 36, 7 CrossRef CAS.
- H. D. Flack, Acta Crystallogr., Sect. A: Found. Crystallogr., 1983, 39, 876 CrossRef.
- L. J. Farrugia, J. Appl. Crystallogr., 1997, 30, 565 CrossRef CAS;
M. N. Burnett and C. K. Johnson, ORTEPIII. Report ORNL-6895, Oak Ridge National Laboratory, Tennessee, USA, 1996 Search PubMed.
Footnotes |
† Electronic supplementary information (ESI) available: ORTEP representations of compounds 2a and 4a; crystal data and refinement parameters for 2c–d and 4b–d. CCDC 940117–940121. For ESI and crystallographic data in CIF or other electronic format see DOI: 10.1039/c3nj00863k |
‡ ORTEP representations of compounds 2a and 4a are given in the ESI.† |
|
This journal is © The Royal Society of Chemistry and the Centre National de la Recherche Scientifique 2014 |