DOI:
10.1039/C2CE26147B
(Paper)
CrystEngComm, 2013,
15, 73-77
Cocrystals of 5-fluorouracil†
Received
13th July 2012
, Accepted 1st September 2012
First published on 3rd September 2012
Molecules containing a purine or pyrimidine skeleton are important1 and many find applications as drugs2 and agrochemicals.3 The most important of these are the nucleobases (adenine (A), guanine (G), cytosine (C), uracil (U) and thymine (T)), which play an important role in maintaining the structure and function of important biomolecules such as DNA and RNA. In these biomolecules, the nucleobases recognize each other, forming AT, AU and GC base pairs. AU and AT base pairs are present in RNA and DNA respectively and the GC base pair is present in both.4 Surprisingly, these molecules are not well explored for the formation of cocrystals.5 For example, to date there are no reports of the solid state structure of a cocrystal between G and C6 or A and U,7 and even the crystal structure of the AT cocrystal has only recently been revealed.8 In this paper, we describe cocrystals of 5-fluorouracil (a pyrimidine derivative) with various aza-donors (Chart 1). Given the developments in the use of the Crystallographic Database in examining the likelihood of formation of a cocrystal1d,9 a systematic study of the crystal structures of such molecules would be beneficial.
5-Fluorouracil is a drug first synthesized in 1957 by Heidelberger et al.10 and is now widely used for the treatment of colorectal,11 breast,11b gastrointestinal,11a and ovarian cancers.11b The drug is usually given intravenously (due to its poor water solubility), but can also be applied as an ointment, especially in the case of skin cancer.12 It acts against cancer by inhibiting thymidylate synthase, which in turn stops the production of thymine, required by the cancerous cells for the production of DNA.13 From a crystal engineering viewpoint, 5-fluorouracil is an interesting and challenging molecule due to the presence of multiple hydrogen bond donors and acceptors.
There are two known polymorphic forms of 5-fluorouracil, the crystal structure of the first of which (Form 1) was reported by Fallon et al.14 in 1973. In Form 1, molecules of 1 interact through either single N(1)–H⋯O(7) or pair-wise N(3)–H⋯O(8) hydrogen bonds to form sheets. The likely existence of a second polymorph (Form 2) of 5-fluorouracil was identified during crystal structure prediction studies by Hulme et al., and later confirmed experimentally.15 In Form 2, molecules of 1 recognize each other through pairwise N(3)–H⋯O(8) and N(1)–H⋯O(7) hydrogen bonds (Fig. 1) to form tapes.
In this paper, we explore the molecular recognition properties of 5-fluorouracil by synthesizing cocrystals (1a–1c) with various aza donors (acridine (a), phenazine (b) and 4,4-bispyridylethene (c) respectively). Acridine and its derivatives are known to bind DNA. Because of this property, derivatives of acridine (for example acridine orange) find applications as nucleic acid dyes. In addition, they show a range of properties, from being carcinogenic (e.g. dibenzo[a,h]acridine, dibenzo[a,j]acridine) to showing activity against cancer (e.g. amsacrine).
A 2
:
1 cocrystal between 5-fluorouracil and acridine was obtained on cocrystallizing 1 and a in a 1
:
1 stoichiometry from methanol. The asymmetric unit of 1a contains half of an acridine molecule. In three dimensions this cocrystal forms a layered structure (Fig. 2(a)).
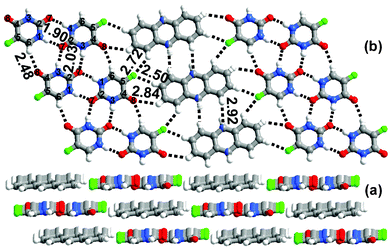 |
| Fig. 2 (a) Layered packing observed in 1a. (b) In the sheet the molecules of a are sandwiched between the tapes of 1. | |
In each layer, the molecules of 1 recognize each other by pairwise N(1)–H⋯O(7), N(3)–H⋯O(7) and single C(6)–H⋯O(8) hydrogen bonds forming tapes. The disordered molecules of acridine are sandwiched between the tapes and interact with molecules of 1 through C–H⋯O or C–H⋯F hydrogen bonds (Fig. 2). The adjacent molecules of acridine interact by C–H⋯N hydrogen bonds to form ribbons. The molecules of 1 and a in adjacent layers interact by π–π interactions. It is interesting that the nitrogen atom of acridine is not involved in a hydrogen bond with 1. To investigate this further, 1 was cocrystallized with phenazine (a molecule with a similar shape to acridine, but with two nitrogens instead of one) in a 1
:
1 ratio from methanol. A 2
:
1 5-fluorouracil
:
phenazine cocrystal was obtained. As with 1a this cocrystal forms a layered structure, and the aza molecules (phenazine) are sandwiched between the tapes of 1. In 1b the molecules of 1 recognize each other forming tapes through pairwise N(3)–H⋯O(8) and N(1)–H⋯O(7) hydrogen bonds, as observed in the crystal structure of Form 2 of 1. In contrast to 1a, however, phenazine interacts with the molecular tapes of 1 through C–H⋯N hydrogen bonds as well as through C–H⋯O and C–H⋯F hydrogen bonds, as shown in Fig. 3(b).
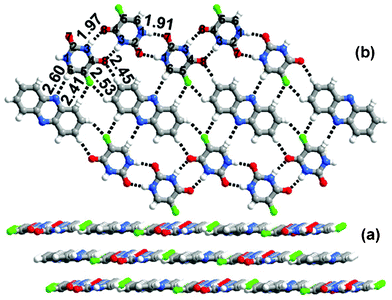 |
| Fig. 3 (a) Layered packing observed in 1b. (b) Sheet network observed in 1b. | |
Extending the study of cocrystals 1a and 1b, 5-fluorouracil was cocrystallized with 4,4-bispyridylethene,16 a larger molecule than a and b. Cocrystal 1c, a crystal form containing four molecules of 1 for every molecule of c, was obtained on crystallizing 1 and c in a 1
:
1 ratio from methanol. The crystal structure revealed the formation of a host–guest assembly between 1 and c (Fig. 4(a)).There are two types of molecules of 1 (labelled A and B, Fig. 4(b)). A type molecules interact with molecules of their own kind (A) through a pairwise N(1)–H⋯O(7) hydrogen bond and with B type molecules through single C–H⋯O and C–H⋯F hydrogen bonds and a pairwise N(3)–H⋯O(7)/N(3)–H⋯O(8) hydrogen bond to form a cyclic host, which is filled by the guest molecules of c. The guest molecules interact with the host through N–H⋯N and C–H⋯O hydrogen bonds. In each of the cocrystals, therefore, the ratio of cocrystal components is different to that used during crystallization.
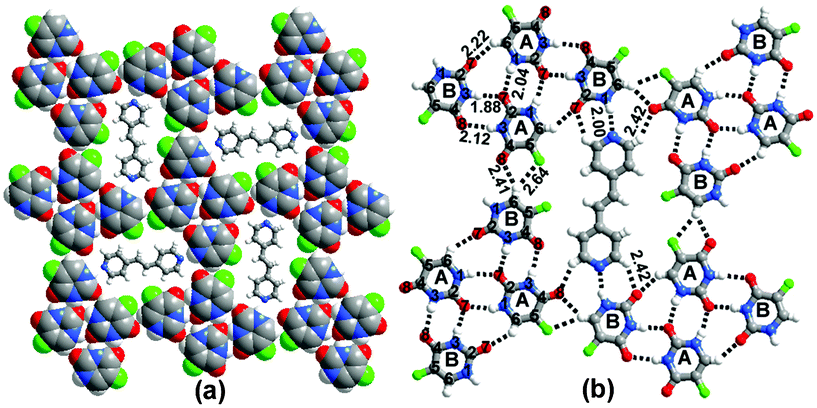 |
| Fig. 4 Host–guest network observed in 1c. The host network is formed by the molecules of 1 and the molecules of c behave as guests. (b) H-bond interactions. | |
Given the layered characteristics of the cocrystal structures, attempts were made to prepare thin, flat crystals suitable for examination by transmission electron microcopy.17 (See ESI† for TEM details.) Cocrystal formers a–c were further crystallized with 5-fluorouracil by evaporation of a single drop of a methanol solution on a glass slide (the solutions contained the same ratio of reactants as found in the respective cocrystals 1a–1c). The resulting samples were analysed by TEM.17,18 A majority of the crystals in the 5-fluorouracil–acridine sample were found to have a rectangular plate morphology, but a small number of crystals with a more rounded plate-like appearance were also identified (Fig. 5). Diffraction patterns from the rectangular crystals could be indexed to crystal structure 1a, whereas, interestingly, diffraction patterns from the rounded plate crystals were inconsistent with crystal structure 1a and with all of the known polymorphs of 5-fluorouracil and acridine. This phase may be a second polymorph of the 5-fluorouracil
:
acridine cocrystal which grows concomitantly with 1a, or a new polymorph of either 5-fluorouracil or acridine. The small size of the individual crystals made confirmation by single crystal X-ray diffraction impossible, and work is ongoing to determine the nature of this new crystal form.
Crystals of 1b prepared by slow evaporation of 5-fluorouracil and phenazine from methanol, as described above, have a block morphology. In contrast, crystallization from a single drop of methanol was more rapid and crystals with a rectangular plate habit were isolated (Fig. 6). Electron diffraction analysis demonstrated that these rectangular plates were not crystal form 1b, nor any of the known polymorphs of 5-fluorouracil or phenazine, indicating that a new crystal form of the cocrystal or of 5-fluorouracil or phenazine had been obtained by this crystallization method. These crystals had sub-micron sized dimensions making them potentially interesting in terms of high dissolution rates.19 Again, due to the small size of these crystals, analysis by single crystal X-ray diffraction was not possible.
Electron diffraction patterns from samples of 5-fluorouracil
:
4,4-bispyridylethene grown by evaporative crystallisation from methanol were consistent with crystal structure 1c.
Conclusions
Molecular recognition studies of 5-fluorouracil were performed with various aza-donors (a–c). The composition of the resulting cocrystals differed from that present in the growth solutions. TEM analysis, with the combination of high magnification imaging and crystal phase identification (through indexing of electron diffraction patterns), provided additional information about crystal morphology and about new crystal forms in these cocrystal systems that could not have been obtained using standard analytical techniques. Further work to fully identify the nature of these new forms is in progress.
Acknowledgements
We thank the Pfizer Institute of Pharmaceutical Materials Science (Fellowship for AD), the EPSRC and the EU INTERREG IVA 2 Mers Seas Zeeën Cross-border Cooperation Program (Fellowship for MDE) for funding. Dr. John E. Davies is thanked for collecting single-crystal X-ray data for the reported cocrystals.
References
-
(a) K. S. Jain, T. S. Chitre, P. B. Miniyar, M. K. Kathiravan, V. S. Bendre, V. S. Veer, S. R. Shahane and C. J. Shishoo, Curr. Sci., 2006, 90, 793–803 CAS;
(b) R. Mishra and I. Tomar, Int. J. Pharm. Sci. Res., 2011, 2, 758–771 CAS;
(c) A. Delori, T. Friscic and W. Jones, CrystEngComm, 2012, 14, 2350–2362 RSC;
(d) A. Delori, P. T. A. Galek, E. Pidcock and W. Jones, Chem.–Eur. J., 2012, 18, 6835–6846 CAS.
-
(a) P. M. Bhatt, Y. Azim, T. S. Thakur and G. R. Desiraju, Cryst. Growth Des., 2009, 9, 951–957 CrossRef CAS;
(b) Some of the common drugs containing purine or pyrimidine skeleton are 6-mercaptopurine, fludarabine, 5-florouracil, arabinosylcytosine, capecitabine, gemcitabine, decitabine, pyrimethamine, trimethoprim.
- Examples of common agrochemicals containing a purine or pyrimidine skeleton are pyrimethanil, diflumetorim, dimethirimol, ethirimol, fenarimol, ferimzone, nuarimol, triarimol, cyprodinil, mepanipyrim, caffeine.
-
(a) J. D. Watson and F. H. Crick, Nature, 1953, 171, 737–738 CrossRef CAS;
(b) J. H. Cate, A. R. Gooding, E. Podell, K. Zhou, B. L. Golden, C. E. Kundrot, T. R. Cech and J. A. Doudna, Science, 1996, 273, 1678–1685 CrossRef CAS.
-
(a) R. Thomas and G. U. Kulkarni, J. Mol. Struct., 2008, 873, 160–167 CrossRef CAS;
(b) G. Portalone and M. Colapietro, Acta Crystallogr., Sect. C: Cryst. Struct. Commun., 2007, 63, o423–o425 Search PubMed;
(c) S. R. Perumalla, E. Suresh and V. R. Pedireddi, Angew. Chem., Int. Ed., 2005, 44, 7752–7757 CrossRef CAS;
(d) M. Byres, P. J. Cox, G. Kay and E. Nixon, CrystEngComm, 2009, 11, 135–142 RSC;
(e) D. H. Voet and A. Rich, J. Am. Chem. Soc., 1969, 91, 3069–3075 CrossRef CAS;
(f) R. Thomas and G. U. Kulkarni, Beilstein J. Org. Chem., 2007, 3 Search PubMed;
(g) S. H. Kim and A. Rich, J. Mol. Biol., 1969, 42, 87–95 CrossRef CAS;
(h) S. H. Kim and A. Rich, Science, 1967, 158, 1046–1048 CAS;
(i) T. Ishida, M. Katsuta, M. Inoue, Y. Yamagata and K. Tomita, Biochem. Biophys. Res. Commun., 1983, 115, 849–854 CrossRef CAS;
(j) V. R. Pedireddi, A. Ranganathan and K. N. Ganesh, Org. Lett., 2001, 3, 99–102 CrossRef CAS;
(k) R. W. Gellert and I. N. Hsu, J. Crystallogr. Spectrosc. Res., 1983, 13, 99–105 CrossRef CAS;
(l) V. Langer, K. Huml and J. Zachova, Acta Crystallogr., Sect. C: Cryst. Struct. Commun., 1984, 40, 2080–2082 CrossRef;
(m) D. Schindler, F. Eissmann and E. Weber, Org. Biomol. Chem., 2009, 7, 3549–3560 RSC;
(n) S. Fujii, K. Kawasaki, A. Sato, T. Fujiwara and K. Tomita, Arch. Biochem. Biophys., 1977, 181, 363–370 CrossRef CAS;
(o) K. Aiba, T. Hata, S. Sato and C. Tamura, Acta Crystallogr., Sect. B: Struct. Crystallogr. Cryst. Chem., 1978, 34, 1259–1263 CrossRef;
(p) W. H. Ojala, W. B. Gleason, T. I. Richardson and R. E. Lovrien, Acta Crystallogr., Sect. C: Cryst. Struct. Commun., 1994, 50, 1615–1620 CrossRef;
(q) G. Portalone, Acta Crystallogr., Sect. C: Cryst. Struct. Commun., 2010, 66, o295–o301 Search PubMed;
(r) S. Zaitu, Y. Miwa and T. Taga, Acta Crystallogr., Sect. C: Cryst. Struct. Commun., 1995, 51, 1857–1859 CrossRef;
(s) T. Lee and P. Y. Wang, Cryst. Growth Des., 2010, 10, 1419–1434 CrossRef CAS;
(t) B. Sridhar and K. Ravikumar, Acta Crystallogr., Sect. C: Cryst. Struct. Commun., 2008, 64, o566–o569 Search PubMed;
(u) T. Murata, G. Saito, K. Nishimura, Y. Enomoto, G. Honda, Y. Shimizu, S. Matsui, M. Sakata, O. O. Drozdova and K. Yakushi, Bull. Chem. Soc. Jpn., 2008, 81, 331–344 CrossRef CAS;
(v) T. Murata, Y. Enomoto and G. Saito, Solid State Sci., 2008, 10, 1364–1368 CrossRef CAS;
(w) N. Benali-Cherif, W. Falek and A. Direm, Acta Crystallogr., Sect. E: Struct. Rep. Online, 2009, 65, o3058–o3059 Search PubMed;
(x) B. Sridhar and K. Ravikumar, Acta Crystallogr., Sect. C: Cryst. Struct. Commun., 2010, 66, o414–o417 Search PubMed;
(y) C. M. Weeks, D. C. Rohrer and W. L. Duax, Science, 1975, 190, 1096–1097 CAS;
(z) J. S. Nirmalram, D. Tamilselvi and P. T. Muthiah, J. Chem. Crystallogr., 2011, 41, 864–867 CrossRef CAS;
(a
a) P. Amo-Ochoa, P. J. S. Miguel, P. Lax, I. Alonso, M. Roitzsch, F. Zamora and B. Lippert, Angew. Chem., Int. Ed., 2005, 44, 5670–5674 CrossRef CAS;
(a
b) C. B. Aakeröy, N. Schultheiss, J. Desper and C. Moore, Cryst. Growth Des., 2007, 7, 2324–2331 CrossRef;
(a
c) A. Delori and W. Jones, CrystEngComm, 2011, 13, 6315–6318 RSC.
-
(a) S. Fujita, A. Takenaka and Y. Sasada, Biochemistry, 1985, 24, 508–512 CrossRef CAS;
(b) E. J. O'Brien, Acta Crystallogr., 1967, 23, 92–106 CrossRef CAS.
-
(a) L. Katz, K. Tomita and A. Rich, Acta Crystallogr., 1966, 21, 754–764 CrossRef CAS;
(b) K. Tomita, L. Katz and A. Rich, J. Mol. Biol., 1967, 30, 545–549 CrossRef CAS;
(c) T. D. Sakore, S. S. Tavale and H. M. Sobell, J. Mol. Biol., 1969, 43, 361–374 CrossRef CAS.
-
(a) S. Chandrasekhar, T. R. R. Naik, S. K. Nayak and T. N. Guru Row, Bioorg. Med. Chem. Lett., 2010, 20, 3530–3533 CrossRef CAS;
(b) S. Mahapatra, S. K. Nayak, S. J. Prathapa and T. N. Guru Row, Cryst. Growth Des., 2008, 8, 1223–1225 CrossRef CAS;
(c) M. N. Frey, T. F. Koetzle, M. S. Lehmann and W. C. Hamilton, J. Chem. Phys., 1973, 59, 915–924 CrossRef CAS;
(d) K. Hoogsteen, Acta Crystallogr., 1963, 16, 907–916 CrossRef CAS.
- P. T. A. Galek, E. Pidcock, P. A. Wood, I. J. Bruno and C. R. Groom, CrystEngComm, 2012, 14, 2391–2403 RSC.
- C. Heidelberger, N. K. Chaudhuri, P. Danneberg, D. Mooren, L. Griesbach, R. Duschinsky, R. J. Schnitzer, E. Pleven and J. Scheiner, Nature, 1957, 179, 663–666 CrossRef CAS.
-
(a) P. G. Johnston, H.-J. Lenz, C. G. Leichman, K. D. Danenberg, C. J. Allegra, P. V. Danenberg and L. Leichman, Cancer Res., 1995, 55, 1407–1412 CAS;
(b) E. Raymond, C. Buquet-Fagot, S. Djelloul, J. Mester, E. Cvitkovic, P. Allain, C. Louvet and C. Gespach, Anti-Cancer Drugs, 1997, 8, 876–885 CrossRef CAS.
- C. M. Perrett, J. M. McGregor, J. Warwick, P. Karran, I. M. Leigh, C. M. Proby and C. A. Harwood, Br. J. Dermatol., 2007, 156, 320–328 CrossRef CAS.
- D. B. Longley, D. P. Harkin and P. G. Johnston, Nat. Rev. Cancer, 2003, 3, 330–338 CrossRef CAS.
- L. Fallon, Acta Crystallogr., Sect. B: Struct. Crystallogr. Cryst. Chem., 1973, 29, 2549–2556 CrossRef CAS.
-
(a) A. T. Hulme, S. L. Price and D. A. Tocher, J. Am. Chem. Soc., 2005, 127, 1116–1117 CrossRef CAS;
(b) M. Tutughamiarso, M. Bolte and E. Egert, Acta Crystallogr., Sect. C: Cryst. Struct. Commun., 2009, 65, o574–o578 Search PubMed;
(c) A. T. Hulme and D. A. Tocher, Acta Crystallogr., Sect. E: Struct. Rep. Online, 2004, 60, o1781–o1782 Search PubMed;
(d) S. A. Barnett, A. T. Hulme, N. Issa, T. C. Lewis, L. S. Price, D. A. Tocher and S. L. Price, New J. Chem., 2008, 32, 1761–1775 RSC;
(e) A. T. Hulme and D. A. Tocher, Acta Crystallogr., Sect. E: Struct. Rep. Online, 2004, 60, o1783–o1785 Search PubMed;
(f) A. T. Hulme and D. A. Tocher, Acta Crystallogr., Sect. E: Struct. Rep. Online, 2004, 60, o1786–o1787 Search PubMed.
-
(a) J. Xiong, M. L. Hu, Y. J. Lan and S. F. Zhang, Acta Crystallogr., Sect. E: Struct. Rep. Online, 2006, 62, o2371–o2373 Search PubMed;
(b) G. S. Nichol and W. Clegg, Cryst. Growth Des., 2009, 9, 1844–1850 CrossRef CAS;
(c) M. L. Hu, Acta Crystallogr., Sect. E: Struct. Rep. Online, 2005, 61, o3063–o3065 Search PubMed.
- M. D. Eddleston, E. G. Bithell and W. Jones, J. Pharm. Sci., 2010, 99, 4072–4083 CAS.
-
(a) Z. G. Li, R. L. Harlow, C. M. Foris, H. Li, P. Ma, R. D. Vickery, M. B. Maurin and B. H. Toby, Microsc. Microanal., 2002, 8, 134–138 CrossRef CAS;
(b) J. M. Thomas and P. A. Midgley, Chem. Commun., 2004, 1253–1267 RSC;
(c) W. Jones and J. M. Thomas, Prog. Solid State Chem., 1979, 12, 101–124 CrossRef CAS.
- J. R. G. Sander, D.-K. Bučar, R. F. Henry, G. G. Z. Zhang and L. R. MacGillivray, Angew. Chem., Int. Ed, 2010, 49(7284–S7288), S7284/7281–S7284/7286 Search PubMed.
|
This journal is © The Royal Society of Chemistry 2013 |
Click here to see how this site uses Cookies. View our privacy policy here.