DOI:
10.1039/C2TX20035J
(Paper)
Toxicol. Res., 2012,
1, 201-205
Cellular responses of aniline oligomers: a preliminary study†
Received
13th June 2012
, Accepted 20th August 2012
First published on 23rd August 2012
Abstract
The biomedical applications of aniline oligomers and their derivatives have attracted increasing interest due to their electroactive and biodegradable properties. However, no reports have systematically examined the toxicity of these electroactive materials, which has severely hindered their biomedical applications. In this work, the cellular responses of aniline oligomers including aniline dimer, trimer and tetramer to mouse embryo fibroblast (NIH-3T3) cells and adenocarcinomic human alveolar basal epithelial (A549) cells were determined and compared for the first time. Our results demonstrated that the aniline trimer showed the highest cytotoxicity to both types of cells. Compared with the NIH-3T3 cells, aniline oligomers exhibited the least cytotoxicity to A549 cells. Taken together, we demonstrate that both the properties of aniline oligomers and cell types could influence the cellular responses of aniline oligomers. As the first report focused on the cytotoxicity of aniline oligomers, this work provides some fundamental and important information about the cytotoxicity of aniline oligomers, which should be valuable for their biomedical applications.
1. Introduction
Since their discovery in the 1970s, conducting polymers (CPs) such as polypyrrole (PPy), polyheterocycles, polyaniline (PANI) and poly(3,4-ethylenedioxythiophene) (PEDOT) have been the subject of many exciting research studies.1–6 Because of their excellent electrical and optical properties, CPs and their derivatives have been extensively investigated for various applications, including electronic nanodevices, chemical and biological sensors, catalysis and electrocatalysis, energy, microwave absorption, electromagnetic interference shielding, electrorheological fluids, biomedicine, etc.7–20 Especially, given the fact that CPs could tune cellular activities such as cell adhesion, cell differentiation and cell growth through electrical stimulus, the biomedical applications of CPs for tissue engineering, and neural probes have gained considerable attention over the past few decades.21–25 However, one of the challenges for the biomedical applications of CPs is their lack of solubility in aqueous media. The other challenge for the biomedical applications of CPs is their nondegradability, which thus leading to adverse effects for their long time in vivo applications. Therefore, the searching of alternative electroactive materials which could overcome these limitations is of great importance.
Compared with CPs, conjugated oligomers and their derivatives should be more suitable for biomedical applications due to their biodegradability, ease of synthesis, and similar electroactivity like CPs.7,26–29 A series of conjugated oligomers have thus been synthesized and incorporated into biodegradable polymers for biomedical applications.30 For example, Albertsson et al. have demonstrated that degradable and conductive hydrogels could be obtained by incorporation of aniline oligomers into biodegradable polymers via coupling reactions between the epoxy groups in the network and amine group of aniline oligomers.31–33 Our previous reports also found that incorporation of aniline oligomers into biodegradable polymers could stimulate the growth and differentiation of rat adrenal pheochromocytoma cell line (PC12), suggesting the potential biomedical applications of aniline oligomers for tissue engineering.34–38 Although the biomedical applications of conjugated oligomers have gained increasing attention, systematic investigation of their biocompatibility has not been reported.
In the current work, cellular responses of the aniline dimer, trimer, and tetramer to mouse embryo fibroblast (NIH-3T3) cells and adenocarcinomic human alveolar basal epithelial (A549) cells were evaluated and compared for the first time. Our results showed that both properties of aniline oligomers and cell types could influence their cellular responses. Among them, the aniline trimer showed the highest toxicity to both types of cells. Compared with NIH-3T3 cells, A549 cells exhibited relative better tolerance with aniline oligomers. As the first report focused on the cellular responses of aniline oligomers, we expected our results could provide fundamental information about the cytotoxicity of aniline oligomers and be valuable for their biomedical applications.
2. Experimental details
2.1 Materials and characterization
The aniline dimer and other solvents and chemicals were purchased from commercial sources and used directly without further purification. The aniline trimer and tetramer were synthesized according to our previous reports.29,39 As evidenced by the electrospray ionisation mass spectrometry (ESI-MS) results, the molecular weights of the aniline trimer and aniline tetramer are 291.0 and 366.3 Da (see Fig. S1†), demonstrating that aniline oligomers were successfully synthesized. Before the biological assays, aniline oligomers were first doped with concentrated hydrochloric acid (w/w, 37%) to render them water soluble. The structural characteristics of aniline oligomers as well as the dispersibility of the aniline oligomers in phosphate buffer solution (PBS) are shown in Fig. 1. It can be seen that the aniline trimer has the highest water solubility due to two primary amine groups on the termini.
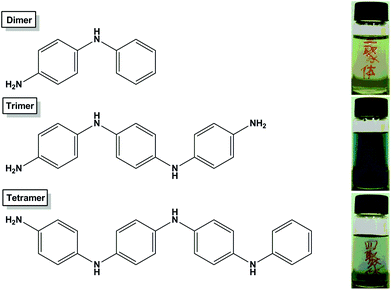 |
| Fig. 1 Structural characteristics of aniline oligomers. The aniline dimer and tetramer possess one amine group, and the aniline trimer has two amine groups. Inset bottles show the optical images of aniline oligomers dispersed in PBS after doping with concentrated hydrochloric acid (w/w, 37%). | |
2.2 Cytotoxicity of aniline oligomers
2.2.1 Cell morphology observation.
NIH-3T3 cells and A549 cells were cultured in Dulbecco's modified eagle medium (DMEM) supplemented with 10% heat-inactivated fetal bovine serum (FBS), 2 mM glutamine, 100 U mL−1 penicillin, and 100 μg mL−1 of streptomycin. Cell culture was maintained at 37 °C in a humidified condition of 95% air and 5% CO2 in culture medium. Culture medium was changed every two or three days for maintaining the exponential growth of the cells.
The cell morphology changes of cells exposed to aniline oligomers were examined by optical microscopy (Leica, Germany). Briefly, cells were seeded in 6-well microplates at a density of 1 × 105 cells mL−1 in 2 mL of respective media containing 10% FBS. After cell attachment, plates were washed with PBS and the cells were treated with complete cell culture medium, or 80 μg mL−1 of dimer, trimer, and tetramer prepared in 10% FBS containing media for 12, 24 and 48 h. The morphology of the cells was observed using an optical microscopy, the overall magnification was ×100.
2.2.2 Cell viability assay.
The cell viability of aniline oligomers to NIH-3T3 and A549 cells was evaluated using cell count kit-8 (CCK-8) assay according to our previous reports.40–43 Briefly, cells were seeded in 96-well microplates at a density of 1 × 105 cells mL−1 in 160 μL of respective media containing 10% FBS. After 24 h of cell attachment, the cells were incubated with 20, 40, 80, 160 and 320 μg mL−1 of aniline oligomers (dimer, trimer, tetramer) for 6 and 24 h. Then the aniline oligomers were removed and cells were washed with PBS three times. 10 μL of CCK-8 dye and 100 μL of DMEM cell culture media was added to each well and incubated for 2 h at 37 °C. Plates were then analyzed with a microplate reader (Victor #xX_CYR_HEX_428;, Perkin-Elmer). Measurements of formazan dye absorbance were carried out at 450 nm, with the reference wavelength at 620 nm. The values were proportional to the number of live cells. The percent reduction of CCK-8 dye was compared to controls (cells not exposed to aniline oligomers), which represented 100% CCK-8 dye reduction. Three replicate wells were used for each control and test concentrations per microplate, and the experiment was repeated three times. Cell survival was expressed as absorbance relative to that of untreated controls. Results are presented as mean ± standard deviation (SD). The half maximal inhibitory concentration 50% (IC50) values of aniline oligomers were calculated using SPSS 15.0 and used to compare their cytotoxicity.
2.2.3 Radical oxygen assay (ROS) generation.
Oxidative stress was measured in relation to the generation of ROS. The ability of aniline oligomers to induce intracellular ROS formation was determined using 2,7-dichlorodihydrofluorescein diacetate (DCFH-DA) assay according to our previous work.44–46 In brief, NIH-3T3 cells were cultured in 96 well plates and incubated with different concentrations (20, 40, 80, 160 and 320 μg mL−1) of aniline dimer, trimer and tetramer for 1 and 4 h, respectively. After washing three times with PBS to remove the uninternalized aniline oligomers, cells were subsequently incubated in 200 μL of a working solution of DCFH-DA, a fluorogenic probe commonly used to detect intracellular generation of ROS at 37 °C for 30 min. Fluorescence data of oxidized DCFH-DA were recorded by using a microplate reader (Victor #xX_CYR_HEX_428;, Perkin-Elmer) with the excitation and emission wavelengths set at 485 and 535 nm, respectively. The fluorescence of cells without incubation with dyes was defined as the background (F0), and cells incubated with 0.5 and 1.0 mg mL−1 of Rosup for 30 min were served as positive control. The values were expressed as percent of fluorescence intensity relative to control wells. All the procedures were performed without exposure to light. Three replicate wells were used for each control and test concentrations per microplate, and the experiment was repeated three times. Results are presented as mean ± SD.
3. Results and discussion
3.1 Cell morphology observation
Optical microscopy observation was first used to evaluate the biocompatibility of aniline oligomers to different cell types. Fig. 2 shows the optical images of NIH-3T3 cells exposed to aniline oligomers for 12 h. It can be seen that the cells kept normal morphology when they were incubated with 80 μg mL−1 of the aniline dimer (Fig. 2B) and tetramer (Fig. 2D). In sharp contrast, most of the cells died when they were exposed to the same concentration of aniline trimer (Fig. 2C). These results given us the preliminary impression that the aniline trimer is more toxic than the aniline dimer and tetramer. Almost no normal cell morphology was observed when cells were incubated with the same concentrations of aniline trimer for 24 and 48 h (Fig. S2C and S3C†). However, some viable cells were still found when they were incubated with the same concentration of the aniline dimer and tetramer, further confirming the high cytotoxicity of the aniline trimer.
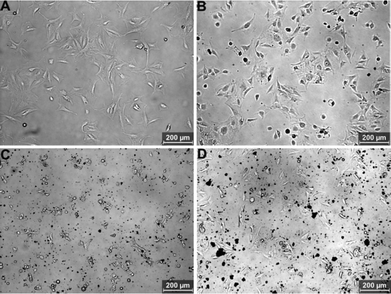 |
| Fig. 2 Optical microscopy images of NIH-3T3 cells incubated with 80 μg mL−1 of aniline oligomers for 12 h. (A) Control cells, (B) aniline dimer, (C) aniline trimer, (D) aniline tetramer. | |
Fig. 3 shows optical microscopy images of A549 cells exposed to 80 μg mL−1 of aniline oligomers for 12 h. Compared with NIH-3T3 cells, the aniline oligomers showed relatively good biocompatibility with the A549 cells. Especially, cells still kept normal morphology when they were incubated with the aniline trimer (Fig. 3C). These results demonstrated that apart from the properties of aniline oligomers, cell types could also influence the cellular responses of the aniline oligomers. Even when the incubation time was extended to 24 and 48 h, cells still kept normal morphology, further evidencing the influence of cell types on their cellular responses (Fig. S4 and S5†).
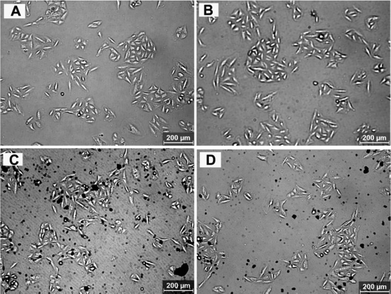 |
| Fig. 3 Optical microscopy images of A549 cells incubated with 80 μg mL−1 of aniline oligomers for 12 h. (A) Control cells, (B) aniline dimer, (C) aniline trimer, (D) aniline tetramer. | |
3.2 Cell viability of aniline oligomers
The effect of aniline oligomers on the growth and viability of NIH-3T3 and A549 cells were examined after 6 and 24 h culture. As shown in Fig. 4, time- and concentration-dependent cytotoxicity of the aniline oligomers to NIH-3T3 cells was confirmed when compared with the negative control (cells did not incubate with aniline oligomers were served as the negative control). It can be seen that the cell viability decreased with increasing aniline oligomer concentrations. Furthermore, when the incubation time increased from 6 to 24 h, the cell viability also decreased correspondingly. Consistent with the results of optical microscopy observation, the cell viability measurement further confirmed the different cytotoxicity of aniline oligomers. As shown in Fig. 4A, when cells were incubated with 40 μg mL−1 of aniline trimer, the cell viability value is about 60%. However, the cell viability values are greater than 90% when cells were incubated with the same concentration of aniline dimer and tetramer. On the basis of the cell viability results, IC50 values were calculated and used to compare the biocompatibility of aniline oligomers. The IC50 values of the aniline dimer, trimer, and tetramer are 642.2, 210.8 and 532.2 μg mL−1 after 6 h incubation. When the incubation time was extended to 24 h, the IC50 values are 198.3, 69.3 and 316.1 μg mL−1 for the aniline dimer, trimer and tetramer, respectively. These results further demonstrated the different cytotoxicity of aniline oligomers.
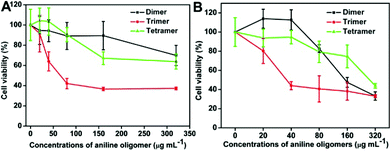 |
| Fig. 4 Time- and concentration-dependent cell cytotoxicity of aniline oligomers to NIH-3T3 cells. (A) Incubation time is 6 h; (B) Incubation time is 24 h. The concentrations of aniline oligomers range from 20 to 320 μg mL−1. Cells that did not incubate with aniline oligomers served as the negative control. The IC50 values are 642.2, 210.8 and 532.2 μg mL−1 for the aniline dimer, trimer and tetramer after they were incubated with NIH-3T3 cells for 6 h. When the incubation time was extended to 24 h, the IC50 values for the aniline dimer, trimer and tetramer are 198.3, 69.3 and 316.1 μg mL−1, respectively. | |
Fig. 5 shows the cell viability of aniline oligomers to A549. It can be seen that almost no cytotoxicity was observed when the aniline oligomers were incubated with A549 cells for 6 h. The IC50 values are 1529.1, 403.8 and 1180.1 μg mL−1 for the aniline dimer, trimer and tetramer, respectively (Fig. 5A). However, significant cytotoxicity was observed when the incubation time was extended to 24 h. The IC50 values of the aniline dimer, trimer and tetramer are 656.7, 192.2 and 565.7 μg mL−1, respectively. These results further confirmed that cell types could also influence the cellular responses of aniline oligomers, which is consistent with the optical microscopy observation.
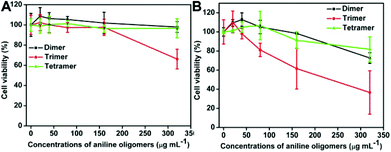 |
| Fig. 5 Time- and concentration-dependent cell cytotoxicity of aniline oligomers to A549 cells. (A) Incubation time is 6 h; (B) Incubation time is 24 h. The concentrations of aniline oligomers range from 20 to 320 μg mL−1. Cells that did not incubate with aniline oligomers served as the negative control. The IC50 values of aniline oligomers to A549 cells are 1529.1, 403.8 and 1180.1 μg mL−1 for the aniline dimer, trimer and tetramer after they were incubated with A549 cells for 6 h. When the incubation time was extended to 24 h, the IC50 value for the aniline dimer, trimer and tetramer were 656.7, 192.2 and 565.7 μg mL−1, respectively. | |
Based on the results of optical microscopy observation and cell viability measurement, we demonstrated that the aniline trimer is highly toxic when compared with the aniline dimer and tetramer. And A549 cells showed relatively good biocompatibility to aniline oligomers compared with NIH-3T3 cells. Although the real reasons for the different cellular responses of aniline oligomers are still not clear, the amine group at the end of the aniline oligomers could play a central role. Compared with the aniline dimer and tetramer, two amine groups exist at the end of the aniline trimer, which may render the good water solubility of the aniline trimer. Although the molecular weight of the aniline dimer and tetramer is different, their cell viability shows no significant difference, further evidencing the importance of functional groups on the cellular responses of aniline oligomers. Therefore, the influence of functional groups on the cellular responses of aniline oligomers should be further investigated to better understand the effects of functional groups on the biocompatibility of aniline oligomers, which may facilitate their biomedical applications. Furthermore, considering the practical biomedical applications of aniline oligomers, other work including the biodegradablity of aniline oligomer derivatives and their pharmacokinetics behavior and in vivo biocompatibility should be further investigated.47–49
3.3 Generation of ROS
The generation of ROS is regarded as one of the possible mechanisms of cytotoxicity for many toxic materials.50–58 In this work, ROS induced by aniline oligomers was determined using DCFH-DA assay to understand the cytotoxicity mechanism of aniline oligomers. Fig. 6 shows the ROS level of aniline oligomers when they were incubated with cells for 1 and 4 h. Compared with the control cells, no significant ROS generation was observed. On the contrary, the ROS level decreased with increase in concentration and incubation time. These results suggested that the cytotoxicity of aniline oligomers may be not due to the generation of ROS. And the decrease of ROS is likely due to the antioxidant properties of aniline oligomers and decrease in cell numbers resulting from the toxicity of aniline oligomers.59–61 Another possible mechanism for the different cytotoxicity of aniline oligomers is likely due to their unique structural characteristics. As shown in Fig. 1, the aniline dimer and tetramer can be regarded as diblock amphiphilic molecules after doping with hydrochloric acid. However, the aniline trimer, with two primary amine groups on the termini and hydrophobic benzene at the center, is similar to an amphiphilic triblock molecule. The aniline oligomers may form novel structures that are likely to be incorporated into the cell membrane and thus disrupt the cell membrane integrity. Compared with the aniline dimer and tetramer, the aniline trimer with two primary amine groups on the termini possesses higher water solubility and positive charge density, thus exhibiting higher cytotoxicity.
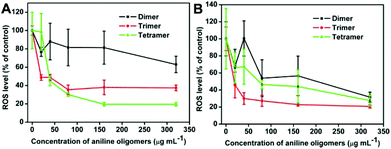 |
| Fig. 6 Generation of ROS from NIH-3T3 cells determined by the hydrolysis of DCFH-DA after incubation with the aniline dimer, trimer and tetramer for 1 and 4 h. | |
4. Conclusion
The cellular responses of aniline oligomers to NIH-3T3 cells and A549 cells were evaluated and compared for the first time. Time- and concentration-dependent cytotoxicity of aniline oligomers has been confirmed. Among the aniline oligomers, aniline trimer showed the highest cytotoxicity to both of NIH-3T3 cells and A549 cells. Furthermore, except from the properties of aniline oligomers, cell types could also influence their cellular responses. Compared with NIH-3T3 cells, A549 cells exhibited less cytotoxicity to aniline oligomers. As the first report focused on the cytotoxicity of aniline oligomers, our results are fundamental and valuable for their further biomedical applications. However, it should also be noted that here we only provided the preliminary results about the cytotoxicity of aniline oligomers, more information about their biodegradability, pharmacokinetics behavior and in vivo toxicity should be further investigated.
Acknowledgements
This research was supported by the National Science Foundation of China (no. 21134004, 21104039), and the National 973 Project (no. 2011CB935700), China Postdoctoral Science Foundation (2011M500280).
References
- H. Shirakawa, E. J. Louis, A. G. MacDiarmid, C. K. Chiang and A. J. Heeger, J. Chem. Soc., Chem. Commun., 1977, 578–580 RSC.
- J. D. Stenger-Smith, Prog. Polym. Sci., 1998, 23, 57–79 CrossRef CAS.
- J. Stejskal, I. Sapurina and M. Trchova, Prog. Polym. Sci., 2010, 35, 1420–1481 CrossRef CAS.
- X. Lu, W. Zhang, C. Wang, T. C. Wen and Y. Wei, Prog. Polym. Sci., 2011, 36, 671–712 CrossRef CAS.
- B. Somboonsub, M. A. Invernale, S. Thongyai, P. Praserthdam, D. A. Scola and G. A. Sotzing, Polymer, 2010, 51, 1231–1236 CrossRef CAS.
- S. Bose, R. A. Khare and P. Moldenaers, Polymer, 2010, 51, 975–993 CrossRef CAS.
- Y. Wei, J. Wang, X. Jia, J. M. Yeh and P. Spellane, Polymer, 1995, 36, 4535–4537 CrossRef CAS.
- S. S. Jeon, H. H. An, C. S. Yoon and S. S. Im, Polymer, 2011, 52, 652–657 CrossRef CAS.
- H. Y. Huang, T. C. Huang, T. C. Yeh, C. Y. Tsai, C. L. Lai, M. H. Tsai, J. M. Yeh and Y. C. Chou, Polymer, 2011, 52, 2391–2400 CrossRef CAS.
- H. Korri-Youssoufi and A. Yassar, Biomacromolecules, 2001, 2, 58–64 CrossRef CAS.
- J. W. Lee, F. Serna, J. Nickels and C. E. Schmidt, Biomacromolecules, 2006, 7, 1692–1695 CrossRef CAS.
- D. Goldman and J. P. Lellouche, Carbon, 2010, 48, 4170–4177 CrossRef CAS.
- N. Gomez and C. E. Schmidt, J. Biomed. Mater. Res. A, 2007, 81, 135–149 CrossRef.
- P. Humpolicek, V. Kasparkova, P. Saha and J. Stejskal, Synth. Met., 2012, 162, 722–727 CrossRef CAS.
- E. Abouzari-Lotf, H. Ghassemi, A. Shockravi, T. Zawodzinski and D. Schiraldi, Polymer, 2011, 52, 4709–4717 CrossRef CAS.
- B. Somboonsub, S. Srisuwan, M. A. Invernale, S. Thongyai, P. Praserthdam, D. A. Scola and G. A. Sotzing, Polymer, 2010, 51, 4472–4476 CrossRef CAS.
- S. U. Celik and A. Bozkurt, Polymer, 2011, 52, 4670–4675 CrossRef.
- A. Madani, B. Nessark, R. Brayner, H. Elaissari, M. Jouini, C. Mangeney and M. M. Chehimi, Polymer, 2010, 51, 2825–2835 CrossRef CAS.
- M. Joubert, M. Bouhadid, D. Bégué, P. Iratçabal, N. Redon, J. Desbriéres and S. Reynaud, Polymer, 2010, 51, 1716–1722 CrossRef CAS.
- S. Ye, C. Shen, H. Pang, J. Wang and Y. Lu, Polymer, 2011, 52, 2542–2549 CrossRef CAS.
- T. J. Rivers, T. W. Hudson and C. E. Schmidt, Adv. Funct. Mater., 2002, 12, 33–37 CrossRef CAS.
- D. Beattie, K. H. Wong, C. Williams, L. A. Poole-Warren, T. P. Davis, C. Barner-Kowollik and M. H. Stenzel, Biomacromolecules, 2006, 7, 1072–1082 CrossRef CAS.
- B. Guo, Y. Sun, A. Finne-Wistrand, K. Mustafa and A. C. Albertsson, Acta Biomater., 2012, 8, 144–153 CrossRef CAS.
- E. Smela, Adv. Mater., 2003, 15, 481–494 CrossRef CAS.
- M. R. Abidian, D. H. Kim and D. C. Martin, Adv. Mater., 2006, 18, 405–409 CrossRef CAS.
- W. Zhang, J. Feng, A. MacDiarmid and A. Epstein, Synth. Met., 1997, 84, 119–120 CrossRef CAS.
- B. Guo, A. Finne-Wistrand and A. C. Albertsson, Macromolecules, 2010, 43, 4472–4480 CrossRef CAS.
- Y. Wei, W. W. Focke, G. E. Wnek, A. Ray and A. G. MacDiarmid, J. Phys. Chem., 1989, 93, 495–499 CrossRef CAS.
- Z. Y. Wang, C. Yang, J. P. Gao, J. Lin, X. Meng, Y. Wei and S. Li, Macromolecules, 1998, 31, 2702–2704 CrossRef CAS.
- B. Guo, A. Finne-Wistrand and A. C. Albertsson, Chem. Mater., 2011, 23, 1254–1262 CrossRef CAS.
- B. Guo, A. Finne-Wistrand and A. C. Albertsson, Biomacromolecules, 2010, 11, 855–863 CrossRef CAS.
- B. Guo, A. Finne-Wistrand and A. C. Albertsson, J. Polym. Sci., Part A: Polym. Chem., 2011, 49, 2097–2105 CrossRef CAS.
- B. Guo, A. Finne-Wistrand and A. C. Albertsson, Biomacromolecules, 2011, 12, 2601–2609 CrossRef CAS.
- L. Huang, J. Hu, L. Lang, X. Wang, P. Zhang, X. Jing, X. Chen, P. I. Lelkes and A. G. MacDiarmid, Biomaterials, 2007, 28, 1741–1751 CrossRef CAS.
- M. Li, Y. Guo, Y. Wei, A. G. MacDiarmid and P. I. Lelkes, Biomaterials, 2006, 27, 2705–2715 CrossRef CAS.
- J. Hu, L. Huang, X. Zhuang, P. Zhang, L. Lang, X. Chen, Y. Wei and X. Jing, Biomacromolecules, 2008, 9, 2637–2644 CrossRef CAS.
- L. Huang, X. Zhuang, J. Hu, L. Lang, P. Zhang, Y. Wang, X. Chen, Y. Wei and X. Jing, Biomacromolecules, 2008, 9, 850–858 CrossRef CAS.
- Y. Liu, J. Hu, X. Zhuang, P. Zhang, Y. Wei, X. Wang and X. Chen, Macromol. Biosci., 2012, 12, 245–250 Search PubMed.
- Y. Wei, C. Yang, G. Wei and G. Feng, Synth. Met., 1997, 84, 289–291 CrossRef CAS.
- X. Zhang, S. Wang, L. Xu, L. Feng, Y. Ji, L. Tao, S. Li and Y. Wei, Nanoscale, 2012, 4, 5581–5584 RSC.
- Y. Zhu, W. Li, Q. Li, Y. Li, X. Zhang and Q. Huang, Carbon, 2009, 47, 1351–1358 CrossRef CAS.
- X. Zhang, S. Wang, C. Zhu, L. Feng, Y. Ji, L. Tao, S. Li and Y. Wei, RSC Adv. Search PubMed , under review.
- J. Li, Y. Zhu, W. Li, X. Zhang, Y. Peng and Q. Huang, Biomaterials, 2011, 31, 8410–8418 CrossRef.
- X. Zhang, Y. Zhu, J. Li, Z. Zhu, W. Li and Q. Huang, J. Nanopart. Res., 2011, 13, 6941–6952 CrossRef CAS.
- X. Zhang, S. Wang, C. Fu, L. Feng, Y. Ji, L. Tao, S. Li and Y. Wei, Polym. Chem., 2012, 3, 2716–2719 RSC.
- X. Zhang, W. Hu, J. Li, L. Tao and Y. Wei, Toxicol. Res., 2012, 1, 62–68 RSC.
- X. Zhang, J. Yin, C. Kang, J. Li, Y. Zhu, W. Li, Q. Huang and Z. Zhu, Toxicol. Lett., 2010, 198, 237–243 CrossRef CAS.
- X. Zhang, J. Yin, C. Peng, W. Hu, Z. Zhu, W. Li, C. Fan and Q. Huang, Carbon, 2011, 49, 986–995 CrossRef CAS.
- L. Zhan, G. Yanxia, Z. Xiaoyong, Q. Wei, F. Qiaohui, L. Yan, J. Zongxian, W. Jianjun, T. Yuqin, D. Xiaojiang and W. Wangsuo, J. Nanopart. Res., 2011, 13, 2939–2947 CrossRef.
- X. Zhang, J. Li, Y. Zhu, Y. Qi, Z. Zhu, W. Li and Q. Huang, Nucl. Sci. Tech., 2011, 22, 99–104 CAS.
- A. Nel, T. Xia, L. Mädler and N. Li, Science, 2006, 311, 622–627 CrossRef CAS.
- K. Pulskamp, S. Diabaté and H. F. Krug, Toxicol. Lett., 2007, 168, 58–74 CrossRef CAS.
- T. Thurnherr, C. Brandenberger, K. Fischer, L. Diener, P. Manser, X. Maeder-Althaus, J. P. Kaiser, H. F. Krug, B. Rothen-Rutishauser and P. Wick, Toxicol. Lett., 2011, 200, 176–186 CrossRef CAS.
- Y. Chang, S. T. Yang, J. H. Liu, E. Dong, Y. Wang, A. Cao, Y. Liu and H. Wang, Toxicol. Lett., 2011, 200, 201–210 CrossRef CAS.
- A. M. Studer, L. K. Limbach, L. Van Duc, F. Krumeich, E. K. Athanassiou, L. C. Gerber, H. Moch and W. J. Stark, Toxicol. Lett., 2010, 197, 169–174 CrossRef CAS.
- S. J. Park, Y. C. Park, S. W. Lee, M. S. Jeong, K. N. Yu, H. Jung, J. K. Lee, J. S. Kim and M. H. Cho, Toxicol. Lett., 2011, 207, 197–203 CrossRef CAS.
- A. Lankoff, W. J. Sandberg, A. Wegierek-Ciuk, H. Lisowska, M. Refsnes, B. Sartowska, P. E. Schwarze, S. Meczynska-Wielgosz, M. Wojewodzka and M. Kruszewski, Toxicol. Lett., 2012, 208, 197–213 CrossRef CAS.
- X. Cai, J. Hao, X. Zhang, B. Yu, J. Ren, C. Luo, Q. Li, Q. Huang, X. Shi and W. Li, Toxicol. Appl. Pharmacol., 2010, 243, 27–34 CrossRef CAS.
- J. Yang, H. Yin, J. Jia and Y. Wei, Langmuir, 2011, 27, 5047–5053 CrossRef CAS.
- P. Bober, J. Stejskal, M. Trchová and J. Prokeš, Polymer, 2011, 52, 5947–5952 CrossRef CAS.
- L. Chen, Y. Yu, H. Mao, X. Lu, W. Zhang and Y. Wei, Mater. Lett., 2005, 59, 2446–2450 CrossRef CAS.
Footnotes |
† Electronic supplementary information (ESI) available: Detailed information about the MS spectra of aniline trimer and tetramer, cell morphology observation of aniline oligomers to NIH-3T3 and A549 cells for 24 and 48 h were provided in supplementary information. See DOI: 10.1039/c2tx20035j |
‡ These authors contributed equally to this work. |
|
This journal is © The Royal Society of Chemistry 2012 |
Click here to see how this site uses Cookies. View our privacy policy here.