DOI:
10.1039/C2RA21271D
(Communication)
RSC Adv., 2012,
2, 12164-12168
Fluorescent turn-off based sensing of nitrated explosives using porphyrins and their Zn(II)-derivatives†
Received
24th June 2012
, Accepted 17th October 2012
First published on 19th October 2012
Abstract
A series of porphyrins and their corresponding Zn(II)-derivatives possessing both electron donating and electron withdrawing substituents at their periphery, have been investigated for the detection of nitrated explosives, which includes nitroaromatics, plastic explosive taggant (DMNB) and explosive nitramines (RDX and HMX), using a fluorescence turn-off mechanism. Among them, β-octamethoxyporphyrin displays the maximum response towards TNT with a KSV of 324 M−1.
Detection of chemical explosives is of great interest owing to the security issues and threat perception across the globe in the present times.1 In particular, there is a great demand for chemosensors that can do rapid detection of ultra-trace analytes from the explosives.2 Among the chemical explosives, 2,4,6-trinitrotoluene (TNT) is the most popular nitroaromatic compounds (NACs), being one of the constituents of many standard explosive compositions, including being a primary high energy material (HEM) in landmines. Furthermore, 2,4-dinitrotoluene (DNT) is one of the major byproducts during TNT preparation and also one of its degradation products and, owing to its moderate vapor pressure, can be detected by canines. Many plastic explosives do not possess NACs and hence detection becomes problematic. Consequently, all commercial plastic explosives contain 2,3-dimethyl-2,3-dinitrobutane (DMNB) as a taggant by law, for detection by trained canines owing to its high vapor pressure.3 However, in order to develop more reliable methods, several new techniques are reported in the literature, including ion mobility spectroscopy (IMS)4 and X-ray scanning,5 which are currently in use. In order to design portable systems for on-field non-contact detection of explosives, the fluorescence quenching method is emerging as a sensitive and potential route towards development of compact low cost sensors.2a In this regard, several polymer-based fluorophores have been explored and display encouraging results.6 Fluorescent dendrimers were also studied in this regard very recently.7 Molecular imprinted polymer,8 coordination polymer,9 quantum dots,10 graphene,11 nanoparticles12 were also explored in recent times for the sensing of high energy materials. However, there are only very few reports where single molecule fluorophores were used as explosive sensors, although they are very promising with regard to their binding pattern and sensitivity.13,3b In this regard, porphyrin based sensors appear to be of particular interest, owing to their superior photo-stability and ease of synthesis. Therefore, in the last few years several reports appeared, where porphyrin derivatives are used for detection of nitroaromatics, in particular TNT.14 Separately, Suslick and coworkers used metalloporphyrins as a platform for colorimetric sensing and discrimination of volatile organic vapors along with toxic gases, food aromas and amines and applied them for the detection of lung cancer, tuberculosis and early stage colon cancer.15 However, to the best of our knowledge, there is no report on the sensing ability of differently substituted porphyrins with regard to different nitrated explosives. As porphyrins are endowed with several peripheral positions for selective modulation of their physico-chemical properties via suitable functionalization and various metal ions can also be incorporated into their core in order to manipulate their coordination behavior and emission characteristics, we envisaged a greater scope for this molecule and hence proposed to explore in detail the sensitivity of various porphyrinic systems towards several nitrated explosives in organic media. For our present study we used meso-tetraphenylporphyrin (H2TPP), several of its meso-fluoroaryl substituted derivatives, β-octaethylporphyrin (H2OEP) and β-octamethoxyporphyrin (H2OMP) and also their corresponding Zn(II)-derivatives as the fluorophores (Scheme 1). 9 and 10 were used as electron rich porphyrins, whereas the fluoroporphyrins, in spite of being relatively electron deficient compared to 1 (and hence were expected to interact weakly with the analytes), were used to see if there is any peripheral interaction between the fluorine atoms and the relatively electron rich components of the nitrated explosives, enhancing their efficiencies, as Fujiki's group reported an enhanced response towards TNT by some of the fluoro-substituted polysilanes.6e In this regard, very recently Ajayaghosh and coworkers reported a fluoro-substituted organogelator, which shows attogram sensing of TNT16a and Pradeep's group reported anchoring of silver clusters embedded in bovine serum albumin onto silica-coated gold mesoflowers, for selective visual detection of TNT at sub zeptomolar level in solution.16b The nitrated explosives used as analytes consist of nitroaromatics, viz. nitrobenzene (NB), 1,3-dinitrobenzene (DNB), DNT, TNT, and alkylnitro compounds such as nitromethane (NM) and plastic explosive taggant DMNB, along with nitramines like RDX and HMX. Furthermore, we have used benzophenone along with benzonitrile as electron deficient and toluene as electron-rich controls of our experiments.
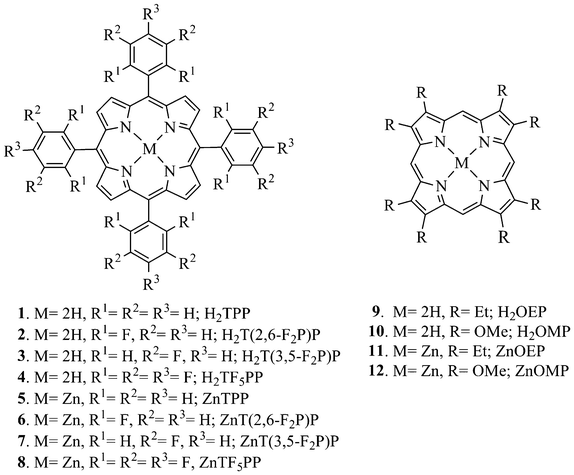 |
| Scheme 1 Designed porphyrins and their Zn(II) -derivatives used for sensing studies. | |
All porphyrins and their Zn(II)-derivatives (Scheme 1) were prepared using reported procedures and their structures were confirmed by standard spectral techniques.17–21 The UV-Vis and fluorescence spectral data for compounds 1–12 are reported in Table 1 and Table S1†. Freebase porphyrins (1–4, 9 and 10) display strong near-UV Soret absorption bands between 377–418 nm accompanied with four weaker Q-bands in the visible region (Fig. S1a†), whereas the corresponding Zn(II)-porphyrin derivatives (5–8, 11 and 12) possess strong Soret absorption bands between 398–423 nm with two characteristic weaker Q-bands (Fig. S1b†). Porphyrins 1–12 show two emission bands (λmax 574–714 nm) when excited at their Soret bands with large Stokes shift (180–308 nm).
Table 1 Absorption and emission maxima along with fluorescence quantum yields for porphyrins 1–12 in chloroform at 25 °C
Porphyrin |
λ
max in nm (abs) |
λ
max in nm (emission) |
Φ
em
|
Quantum yield measured in benzene, taken from ref. 22;
Quantum yield measured in benzene, taken from ref. 23.
|
1
|
418 |
652 |
0.11a |
2
|
414 |
711 |
0.053 |
3
|
416 |
714 |
0.051 |
4
|
413 |
709 |
0.052 |
5
|
423 |
654 |
0.03a |
6
|
421 |
649 |
0.026 |
7
|
422 |
652 |
0.018 |
8
|
420 |
647 |
0.018 |
9
|
400 |
622 |
0.13a |
10
|
377 |
622 |
0.061b |
11
|
402 |
574 |
0.045a |
12
|
398 |
578 |
0.031b |
Quantitative evaluation of solution phase quenching of fluorescence was performed using the Stern–Volmer (SV) equation.24 All compounds display varied degrees of quenching of fluorescence upon successive addition of nitroaromatic compounds (Fig. 1). However, upon addition of nitroaromatics no observable changes in peak maxima are noticed either in absorption (Fig. S3†) or in emission spectra; indicating a lack of strong interaction between porphyrin derivatives and nitroaromatics. Steady state SV plots of all sensing molecules display linearity (Fig. 2) and are independent of the concentration of fluorophores (Fig. S6†). It is noteworthy to mention that the electron rich porphyrins (9 and 10) and their Zn(II)-derivatives (11 and 12) show nonlinearity at higher concentrations of quencher, therefore, in these cases, titrations were performed at a lower concentration (10−4 M) to observe linear SV plots (Fig. S5†). Assuming that both static and dynamic quenching are occurring simultaneously at the higher concentrations, when the modified Stern–Volmer equation was applied,24 the plot of (F0/F − 1)/[Q] vs. [Q] again displayed nonlinearity (Fig. S4†). Therefore, we conclude that at higher concentrations fast quenching of fluorescence may result from aggregation and/or a quenching sphere of action between fluorophore and analyte molecules.6b Best fit data of SV plots reveal moderate to good sensitivity of all porphyrin derivatives towards HEMs. KSV for compounds 1–12 are summarized in Table 2. From the data it can be inferred that quenching of fluorescence is observed probably due to photo-excited electron transfer from electron rich porphyrins to electron deficient nitroaromatics. Of the sensing molecules 10 shows the highest KSV for all nitroaromatics, which may be due to facile electron transfer from electron rich porphyrin (owing to the presence of eight electron donating methoxy groups at the β-positions of the porphyrin moiety) to electron deficient nitroaromatic entities.25 Furthermore, as the number of nitro groups increases on the aromatic ring, their quenching efficiency concomitantly increases as a result of their increased electron deficient character, which facilitates the formation of a charge transfer complex with the porphyrins. Consequently, among the nitroaromatics, the decreasing order of KSV is TNT > DNT ≈ DNB > NB. The presence of one additional binding site in Zn(II)-porphyrins compared to freebase porphyrins led to the emergence of another mode of interaction between the metalloporphyrins and the nitroaromatics via the axial ligation of nitro group to the Zn as well as π–π stacking interactions. The decreasing order of quenching efficiency of the freebase porphyrins is 10 > 9 > 1 > 2 ≈ 3 ≈ 4 and for Zn(II)-porphyrins is 12 > 11 > 5 > 6 > 7 > 8 towards nitroaromatics. However, freebase porphyrins (1–4, 9 and 10) were found to display better quenching ability than the Zn(II)-derivatives (5–8, 11 and 12), which may be attributed to the depletion in electron density at the porphyrin core upon insertion of the Lewis acidic metal ion.
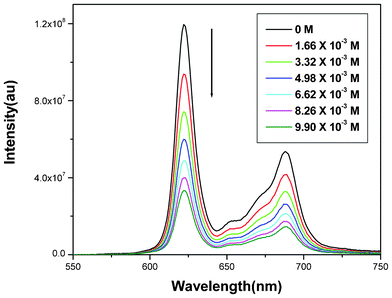 |
| Fig. 1 Quenching of fluorescence after successive addition of aliquots of TNT to the chloroform solution of 9 at 25 °C. | |
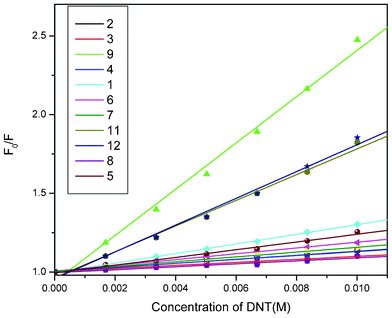 |
| Fig. 2 Stern–Volmer plots for all sensing molecules (except 10) for DNT. | |
Table 2 Stern–Volmer quenching constants (M−1) for all sensing molecules with nitroaromatics
Porphyrin |
Conc. (× 10−7 M) |
TNT |
DNT |
DNB |
NB |
Titrations were done at 10−4 M concentration of nitroaromatics in chloroform solution.
|
1
|
1.78 |
65 ± 1.1 |
31 ± 0.5 |
36 ± 0.9 |
2.4 ± 0.1 |
2
|
2.39 |
25 ± 0.5 |
13 ± 0.6 |
7 ± 0.2 |
1.6 ± 0.8 |
3
|
2.63 |
24 ± 1.3 |
10 ± 0.4 |
7 ± 1.1 |
2.8 ± 1.1 |
4
|
2.36 |
14 ± 0.6 |
13 ± 0.6 |
7 ± 0.6 |
5.5 ± 0.5 |
5
|
1.77 |
34 ± 0.7 |
24 ± 1.3 |
27 ± 1.2 |
9 ± 0.5 |
6
|
2.03 |
32 ± 0.6 |
18 ± 0.4 |
22 ± 0.8 |
3.0 ± 0.6 |
7
|
1.43 |
18 ± 0.6 |
15 ± 0.8 |
15 ± 0.9 |
6.9 ± 0.7 |
8
|
2.25 |
15 ± 0.7 |
9 ± 0.9 |
9 ± 0.8 |
2.6 ± 0.4 |
9
a
|
4.67 |
192 ± 2.9 |
108 ± 3.3 |
113 ± 5.7 |
29 ± 2.3 |
10
a
|
6.00 |
324 ± 13.7 |
226 ± 7.6 |
136 ± 4.8 |
78 ± 6.7 |
11
a
|
2.89 |
99 ± 1.9 |
85 ± 1.8 |
70 ± 2.9 |
34 ± 2.9 |
12
a
|
4.34 |
106 ± 4.2 |
78 ± 2.6 |
57 ± 5.3 |
25 ± 2.2 |
Fluoroporphyrins (2–4) and their Zn(II)-derivatives (6–8) show poor response towards nitroaromatics as compared to non-fluorinated analogues due to their relatively electron deficient nature and hence we can conclude that here fluoro groups are not playing any additional role towards sensing, unlike the fluoro-substituted polysilanes.6e Interestingly, in the case of the Zn(II)-derivatives of the electron deficient fluoroporphyrins, greater quenching was observed compared to their freebase analogues, which may be attributed to the additional interaction via axial ligation of the nitro group of the analyte to the metal ion.
Another interesting point noticed is that in the case of some porphyrins, DNT exhibits a greater quenching ability compared to DNB, although DNB is relatively more electron deficient in nature. For example, while 1, 6 and 9 show the normal trend of DNB showing greater quenching constants compared to DNT, in the case of freebase fluoroporphyrins 2–4 this order is reversed, i.e. DNT shows greater KSV compared to DNB. This may be attributed to a weak H-bonding type interaction between a methyl hydrogen of DNT with fluoro groups at the porphyrin periphery, however for their corresponding zinc derivatives, owing to the reduced π-electron density at the porphyrin ring, this interaction becomes negligible.
Furthermore, a marked difference was observed in the binding of DNT and DNB with 9 and 10, where the latter displays an almost 1.66 times stronger affinity towards DNT compared to DNB. On the other hand, 9 shows a relatively higher affinity for DNB than DNT. This trend can be explained on the basis of a H-bonding type interaction between methyl hydrogen of DNT with oxygen of the methoxy groups at the porphyrin periphery and a similar trend was also observed for the Zn(II)-derivative of 10 (i.e.12). The relatively higher preference of 11 to DNT than DNB may be attributed to better axial ligation of the relatively electron rich DNT to the Zn(II)-porphyrin moiety.
Among all the porphyrins 10 displays the highest binding affinity with TNT and the significantly greater magnitude of KSV of 10 compared to 9 may be attributed to the additional interaction between a methyl of TNT with porphyrin methoxy groups like in the case of DNT. The SV constant of 324 M−1 observed for 10 is better than all single molecule fluorophores reported so far except pyrene, which is toxic to the environment.3b,7a,13a Poly(iptycenebutadiynylene) based conjugated polymers designed by Swager's group for detection of nitroaromatics, in comparison displayed relatively moderate KSV (50–170 M−1) for TNT where quenching of fluorescence was observed through exciton transfer over the entire polymer moiety, thereby creating an amplified sensory response compared to a single molecule fluorophore.6b There are only few reports of single molecule fluorophores where higher KSV values were observed.13f,13h,26c
For alkylnitro compounds we have studied DMNB (plastic explosive taggant) and nitromethane (rocket propellant). Unlike nitroaromatics, these alkylnitro compounds bind only weakly due to the lack of π–π stacking interactions. Here we have observed that the zinc complexes of electron rich porphyrins (11, 12 and 5) showing moderate KSV may be due to axial ligation of nitro group to zinc and/or by a collisional quenching mechanism (Table S2†). The KSV for DMNB is comparable to that reported previously.7a,3b Nitromethane also displays similar behavior like DMNB (Table S2†).
We have used HMX and RDX (high explosives) among the nitramines for our studies. Due to their poor solubility in chloroform, acetone and methanol were used as the solvents for titrations, respectively. There are only a few reports on sensing of RDX and HMX in the solution phase, the majority of them showing no quenching of fluorescence.26 Trogler and coworkers reported hollow silica nanospheres containing a silafluorene-fluorene conjugated polymer for detection of RDX in aqueous solution26a and recently they have used organosilicon polymers for the separation and detection of RDX and HMX.26b However, McGuffin's group demonstrated that pyrene (in acetonitrile) possesses the highest affinity towards RDX and HMX.26c In the case of the porphyrins, only electron rich analogues 9–12 show moderate interactions with RDX and HMX (Table S2†).
The control experiments reveal only electron rich porphyrins (1, 9 and 10) and their zinc(II)-derivatives (5, 11 and 12) exhibit moderate quenching of fluorescence by benzophenone and these are quite a lot lower in magnitude compared to all nitroaromatics (Table S2†). Fluoroporphyrins and their zinc complexes did not display any quenching of fluorescence in the presence of benzophenone. On the other hand, benzonitrile and toluene did not show any quenching of fluorescence with any of the studied porphyrin derivatives (Fig. S6†). Therefore, from the above control experiments we can conclude that our molecules are almost selective towards explosive molecules.
In conclusion, a series of porphyrins and their Zn(II)-derivatives endowed with both electron rich and electron deficient porphyrin cores were explored for the detection of nitrated energetic materials, by introducing appropriate substituents at the porphyrin periphery. The effect of substituents at the porphyrin periphery could be clearly observed with regard to their interactions with nitrated explosive molecules along with the effect of the metal ion at its core. Significantly, as a single molecule fluorophore, some of these porphyrins display binding with HEMs that are either stronger or similar in magnitude to those displayed by conjugated polymeric fluorophore molecules. Presently, a vapour phase sensing study of explosive molecules using these porphyrins and their derivatives is underway.
Acknowledgements
We thank ACRHEM (Phase I), University of Hyderabad for financial support during this work. A.R thanks CSIR, India for fellowship. Authors also thank HEMRL, Pune, India for supplying TNT, RDX and HMX for this work.
References
- A. W. Czarnik, Nature, 1998, 394, 417 CrossRef CAS.
-
(a) C. J. Cumming, C. Aker, M. Fisher, M. Fox, M. J. Grone, D. Reust, M. G. Rockley, T. M. Swager, E. Tower and V. William, IEEE Trans. Geosci. Remote Sens., 2001, 39, 1119 CrossRef;
(b) S. W. Thomas III, G. D. Joly and T. M. Swager, Chem. Rev., 2007, 107, 1339 CrossRef;
(c) S. J. Toal and W. C. Trogler, J. Mater. Chem., 2006, 16, 2871 RSC;
(d) S. Singh, J. Hazard. Mater., 2007, 144, 15 CrossRef CAS;
(e) M. E. Germain and M. J. Knapp, Chem. Soc. Rev., 2009, 38, 2543 RSC;
(f) M. S. Meaney and V. L. McGuffin, Anal. Bioanal. Chem., 2008, 391, 2557 CrossRef CAS;
(g) Y. Salinas, R. Martínez-Máñez, M. D. Marcos, F. Sancenón, A. M. Costero, M. Parra and S. Gil, Chem. Soc. Rev., 2012, 41, 1261 RSC.
-
(a) S. W. Thomas III, J. P. Amara, R. E. Bjork and T. M. Swager, Chem. Commun., 2005, 4572 RSC;
(b) M. E. Germain, T. R. Vargo, P. G. Khalifah and M. J. Knapp, Inorg. Chem., 2007, 46, 4422 CrossRef CAS;
(c) A. Lan, K. Li, H. Wu, D. H. Olson, T. J. Emge, W. Ki, M. Hong and J. Li, Angew. Chem., Int. Ed., 2009, 48, 2334 CrossRef CAS;
(d) S. Pramanik, C. Zheng, X. Zhang, T. J. Emge and J. Li, J. Am. Chem. Soc., 2011, 133, 4153 CrossRef CAS.
- P. Kolla, Anal. Chem., 1995, 67, 184A–189A CrossRef CAS.
- R. D. Luggar, M. J. Farquharson, J. A. Horrocks and R. J. Lacey, X-Ray Spectrom., 1998, 27, 87 CrossRef CAS.
-
(a) J-S. Yang and T. M. Swager, J. Am. Chem. Soc., 1998, 120, 11864 CrossRef CAS;
(b) D. Zhao and T. M. Swager, Macromolecules, 2005, 38, 9377 CrossRef CAS;
(c) H. Sohn, M. J. Sailor, D. Magde and W. C. Trogler, J. Am. Chem. Soc., 2003, 125, 3821 CrossRef CAS;
(d) J. C. Sanchez and W. C. Trogler, J. Mater. Chem., 2008, 18, 3143 RSC;
(e) A. Saxena, M. Fujiki, R. Rai and G. Kwak, Chem. Mater., 2005, 17, 2181 CrossRef CAS;
(f) H. Nie, Y. Zhao, M. Zhang, Y. Ma, M. Baumgarten and K. Müllen, Chem. Commun., 2011, 47, 1234 RSC;
(g) S. Chen, Q. Zhang, J. Zhang, J. Gu and L. Zhang, Sens. Actuators, B, 2010, 149, 155 CrossRef;
(h) C-P. Chang, C-Y. Chao, J. H. Huang, A-K. Li, C-S. Hsu, M. S. Lin, B. R. Hsieh and A-C. Su, Synth. Met., 2004, 144, 297 CrossRef CAS;
(i) Y. Liu, R. C.Mills, J. M. Boncella and K. S. Schanze, Langmuir, 2001, 17, 7452 CrossRef CAS;
(j) P. Long, H. Chen, Y. Yang, H. Wang, Y. Yang, N. Li, K. Li, J. Pei and F. Liu, Macromolecules, 2009, 42, 6501 CrossRef.
-
(a) D. A. Olley, E. J. Wren, G. Vamvounis, M. J. Fernee, X. Wang, P. L. Burn, P. Meredith and P. E. Shaw, Chem. Mater., 2011, 23, 789 CrossRef CAS;
(b) H. Cavaye, P. E. Shaw, X. Wang, P. L. Burn, S-C. Lo and P. Meredith, Macromolecules, 2010, 43, 10253 CrossRef CAS;
(c) M. Guo, O. Varnavski, A. Narayanan, O. Mongin, J-P. Majoral, M. Blanchard-Desce and T. Goodson III, J. Phys. Chem. A, 2009, 113, 4763 CrossRef CAS.
- J. LI, C. E. Kenclig and E. E. Nesterov, J. Am. Chem. Soc., 2007, 129, 15911 CrossRef CAS.
-
(a) A. Lan, K. Li, H. Wu, D. H. Olson, T. J. Emge, W. Ki, M. Hong and J. Li, Angew. Chem., Int. Ed., 2009, 48, 2334 CrossRef CAS;
(b) C. Zhang, Y. Che, Z. Zhang, X. Yang and L. Zang, Chem. Commun., 2011, 47, 2336 RSC.
-
(a) Y. Xia, L. Song and C. Zhu, Anal. Chem., 2011, 83, 1401 CrossRef CAS;
(b) K. Zhang, H. Zhou, Q. Mei, S. Wang, G. Guan, R. Liu, J. Zhang and Z. Zhang, J. Am. Chem. Soc., 2011, 133, 8424 CrossRef CAS.
- L. Tang, H. Feng, J. Cheng and J. Li, Chem. Commun., 2010, 46, 5882 RSC.
-
(a) Y. Long, H. Chen, Y. Yang, H. Wang, Y. Yang, N. Li, K. Li, J. Pei and F. Liu, Macromolecules, 2009, 42, 6501 CrossRef CAS;
(b) Y. Salinas, E. Climent, R. Martínez-Máñez, F. Sancenón, M. Dolores Marcos, J. Soto, A. M. Costero, S. Gil, M. Parra and A. P. de Diego, Chem. Commun., 2011, 47, 11885 RSC.
-
(a) M. S.Meaney and V. L. McGuffin, Anal. Chim. Acta, 2008, 610, 57 CrossRef;
(b) M. E. Germain, T. R. Vargo, B. McClure, J. J. Rack, P. G. Vanpatten, M. Odai and M. J. Knapp, Inorg. Chem., 2008, 47, 6203 CrossRef CAS;
(c) M. E. Germain and M. J. Knapp, J. Am. Chem. Soc., 2008, 130, 5422 CrossRef CAS;
(d) M. E. Germain and M. J. Knapp, Inorg. Chem., 2008, 47, 9748 CrossRef CAS;
(e) K. J. Albert and D. R. Walt, Anal. Chem., 2000, 72, 1947 CrossRef CAS;
(f) S. Shanmugaraju, S. A. Joshi and P. S. Mukherjee, J. Mater. Chem., 2011, 21, 9130 RSC;
(g) Y. Peng, A-J. Zhang, M. Dong and Y-W. Wang, Chem. Commun., 2011, 47, 4505 RSC;
(h) B. Gole, S. Shanmugaraju, A. K. Bar and P. S. Mukherjee, Chem. Commun., 2011, 47, 10046 RSC;
(i) Y. H. Lee, H. Liu, J. Y. Lee, S. H. Kim, S. K. Kim, J. L. Sessler, Y. Kim and J. S. Kim, Chem. Eur. J., 2010, 16, 5895 CAS;
(j) G. V. Zyryanov, M. A. Palacios and P. Anzenbacher Jr, Org. Lett., 2008, 10, 3681 CrossRef CAS.
-
(a) S. Tao, Z. Shi, G. Li and P. Li, ChemPhysChem, 2006, 7, 1902 CrossRef CAS;
(b) S. Tao, G. Li and H. J. Zhu, J. Mater. Chem., 2006, 16, 4521 RSC;
(c) S. Tao, J. Yin and G. Li, J. Mater. Chem., 2008, 18, 4872 RSC;
(d) T. Ema, N. Ura, K. Eguchi, Y. Ise and T. Sakai, Chem. Commun., 2011, 47, 6090 RSC;
(e) A. Yildirim, H. Budinoglu, H. Deniz, M. O. Guler and M. Bayinder, ACS Appl. Mater. Interfaces, 2010, 2, 2892 CrossRef CAS;
(f) M. Rahman and H. J. Harmon, Spectrochim. Acta, Part A, 2006, 65, 901 CrossRef;
(g) S. Tao, G. Li and J. Yin, J. Mater. Chem., 2007, 17, 2730 RSC;
(h)
G. M. Murrey, B. M. Arnold and D. S. Lawrence, US Pat. Appl., 2001077664, 2001 Search PubMed;
(i) T. Ema, N. Ura, K. Eguchi, Y. Ise and T. Sakai, Chem. Commun., 2011, 47, 6090 RSC;
(j) M. Stępień, B. Donnio and J. L. Sessler, Angew. Chem., Int. Ed., 2007, 46, 1431 CrossRef;
(k) R. S. Dudhe, S. P. Tewari, H. N. Raval, M. A. Khaderbad, R. Singh, J. Sinha, M. Yedukondalu, M. Ravikanth, A. Kumar and V. R. Rao, Appl. Phys. Lett., 2008, 93, 263306 CrossRef;
(l) W. Chen, Y. Wang, C. Brückner, C. M. Li and Y. Lei, Sens. Actuators, B, 2010, 147, 191 CrossRef.
-
(a) N. A. Rakow and K. S. Suslick, Nature, 2000, 406, 710 CrossRef CAS;
(b) S. H. Lim, L. Feng, J. W. Kemling, C. J. Musto and K. S. Suslick, Nat. Chem., 2009, 1, 562 CrossRef CAS;
(c) C. Zhang, D. P. Bailey and K. S. Suslick, J. Agric. Food Chem., 2006, 54, 4925 CrossRef CAS;
(d) N. A. Rakow, A. Sen, M. C. Janzen, J. B. Ponder and K. S. Suslick, Angew. Chem., Int. Ed., 2005, 44, 4528 CrossRef CAS.
-
(a) K. K. Kartha, S. S. Babu, S. Srinivasan and A. Ajayaghosh, J. Am. Chem. Soc., 2012, 134, 4834 CrossRef CAS;
(b) A. Mathew, P. R. Sajanlal and T. Pradeep, Angew. Chem., Int. Ed., 2012, 51, 9596 CrossRef CAS.
- A. Merz, R. Scropp and E. Detterl, Synthesis, 1995, 795 CrossRef CAS.
- J. L. Sessler, A. Mozaffari and M. R. Johnson, Org. Synth., 1992, 70, 68 CAS.
- F. R. Longo and J. D. Finarelli, J. Org. Chem., 1967, 32, 467 Search PubMed.
- J. S. Lindsey and R. W. Wagner, J. Org. Chem., 1989, 54, 828 CrossRef CAS.
- A. Merz, R. Schropp and J. Lex, Angew. Chem., Int. Ed. Engl., 1993, 32, 291 CrossRef.
- O. Ohno, Y. Kaizu and H. Kobayashi, J. Chem. Phys., 1985, 82, 1779 CrossRef CAS.
- A. Rana and P. K. Panda, Tetrahedron Lett., 2011, 52, 2697 CrossRef CAS.
-
J. R. Lakowicz, Principle of Fluorescence Spectroscopy (3rd Edition), Publishers: Springers, 2006, 282 Search PubMed.
- T. K. Chandrashekar and V. Krishnan, Inorg. Chem., 1981, 20, 2782 CrossRef CAS.
-
(a) J. Yang, S. Aschemeyer, H. P. Martinez and W. C. Trogler, Chem. Commun., 2010, 46, 6804 RSC;
(b) H. P. Martinez, C. D. Grant, J. G. Reynolds and W. C. Trogler, J. Mater. Chem., 2012, 22, 2908 RSC;
(c) J. V. Goodpaster and V. L. McGuffin, Anal. Chem., 2001, 73, 2004 CrossRef CAS;
(d) C. Jian and W. R. Seitz, Anal. Chim. Acta, 1990, 237, 265 CrossRef CAS;
(e) A. Ponnu and E. V. Anslyn, Supramol. Chem., 2010, 22, 65 CrossRef CAS.
Footnote |
† Electronic Supplementary Information (ESI) available: Experimental details are available in electronic supplementary information. See DOI: 10.1039/c2ra21271d |
|
This journal is © The Royal Society of Chemistry 2012 |
Click here to see how this site uses Cookies. View our privacy policy here.