DOI:
10.1039/C2RA20823G
(Paper)
RSC Adv., 2012,
2, 8104-8109
Organocatalytic domino reaction of salicyl N-thiophosphoryl imines and methyl vinyl ketone initiated by an aza-MBH reaction with bifunctional phosphine catalysts†
Received
15th March 2012
, Accepted 27th June 2012
First published on 27th June 2012
Abstract
We report two domino reactions between salicyl N-thiophosphoryl imines and methyl vinyl ketone catalyzed by bifunctional phosphine catalysts and DBU. These reactions provide two simple and efficient methods to construct chromans and chromens under mild conditions, respectively.
Introduction
Recent advances in domino reactions have provided a new approach for the construction of complex organic molecules.1 These reactions do not require the isolation and purification of each intermediate, so they save time, chemicals and labour. Therefore, considerable effort has been made to develop domino transformations catalyzed by organocatalysts.2 With its similarity to the Morita–Baylis–Hillman (MBH) reaction,3 the aza version of this reaction is extremely fascinating because it affords α-methylene-β-aminocarbonyl derivatives.4 For example, N-tosylimines, N-(arylmethylene)diphenylphosphinamides, N-thiophosphoryl imines and salicyl N-tosylimines are good reactants for the aza-MBH reaction, since they have a greater reactivity than their corresponding aldehydes.
Chroman derivatives are an important class of oxygenated heterocycles that have a broad and interesting range of biological activities.5 Thus, various synthetic methods for the formation of these compounds have been reported. Among these methods, reactions of salicylaldehydes with various conjugated olefins such as α,β-unsaturated ketones,6 acrylate derivatives,7 and nitroalkenes8 have been the most popular in recent years. Although many methods for their synthesis have been reported, it is still necessary to develop new methods.9 Recently, we reported a PPh3 catalyzed domino reaction between salicyl N-thiophosphoryl imines and allenes. This reaction was proven to be an efficient method for the synthesis of 2,4-substituted chroman derivatives (Scheme 1a).10 Furthermore, a simple change to the allenes led to an interesting [4 + 1] cycloaddition reaction, in which a series of cis-2,3-dihydrobenzofurans was formed (Scheme 1b).11 In these two domino reactions, the first step was an aza-MBH reaction. Recently, Shi and Qi have reported a domino reaction between salicyl N-tosylimine and methyl vinyl ketone catalyzed by DABCO (DABCO = 1,4-diazabicyclo[2.2.2]octane). An inseparable mixture of cis and trans chromans was obtained.12 As an extension of our previous studies,13 herein, we describe a bifunctional phosphine-catalyzed domino reaction between salicyl N-thiophosphoryl imines and methyl vinyl ketone, leading to the generation of chromans (Scheme 1c). Furthermore, the cis and trans isomers can be separated by column chromatography.
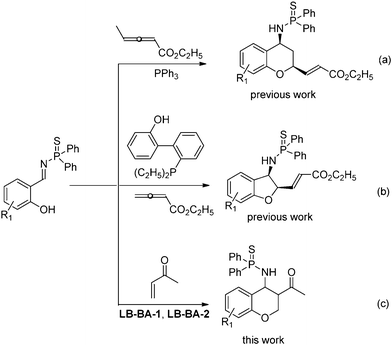 |
| Scheme 1 Examples of domino reactions concerning salicyl N-thiophosphoryl imines. | |
Results and discussion
We previously reported a bifunctional phosphine catalyzed domino reaction between salicyl N-thiophosphoryl imines and allenes (Scheme 1b). We next focused on the use of methyl vinyl ketone as the nucleophile. The reaction of salicyl N-thiophosphoryl imine (1a) and methyl vinyl ketone (2) was selected as a model reaction and the results are shown in the Table 1. We first selected LB-BA-1 (Fig. 1) as the catalyst and acetonitrile as the solvent. To our delight, after 6 h, TLC analysis showed that the salicyl N-thiophosphoryl imine 1a had been consumed and two new compounds had been produced. Based on NMR, HRMS (experimental section), and X-ray (Fig. 2) analysis results, a mixture of two chroman derivatives consisting of cis (47%) and trans (36%) isomers was obtained (entry 1). These results prompted us to further screen the reaction conditions. Initially, several catalysts were examined. Using LB-BA-2, a stronger base than LB-BA-1, as a catalyst, the reaction smoothly yielded the cyclized chroman product 3a in 72% yield along with 8% 1-(6-bromo-2H-chromen-3-yl)ethanone (4a) (entry 2). In order to check the effect of the hydroxyl group of the bifunctional phosphine, LB-3 was selected as the catalyst, only 60% 3a and 25% 4a was obtained (entry 3). Furthermore, when DBU (DBU = 1,8-diazabicyclo[5.4.0]undec-7-ene) was used as the catalyst, 52% 4a was obtained exclusively (entry 5). One other catalyst, DMAP (DMAP = 4-dimethylaminopyridine), was less efficient than LB-BA-1 (entry 4).
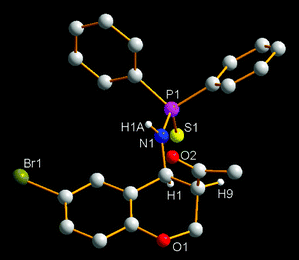 |
| Fig. 2 The X-ray structure of the syn-isomer of 3a. | |
Table 1 Optimization of the reaction conditionsa
Entry |
Catalyst |
Solvent |
Time (h) |
Yield (%)b3a (cis/trans) |
4a
|
Reactions were carried out using 1a (1.0 mmol), methyl vinyl ketone (1.2 mmol) and catalyst (0.2 mmol) in 2 ml solvent at room temperature.
Isolated yield.
The reaction was carried out at 20 °C.
The reaction was carried out at 10 °C.
10 mol% LA-BA-1 was used.
|
1 |
LB-BA-1
|
CH3CN |
6 |
83 (47/36) |
0 |
2 |
LB-BA-2
|
CH3CN |
3 |
72 (40/32) |
8 |
3 |
LB-3
|
CH3CN |
24 |
60 (30/30) |
25 |
4 |
DMAP |
CH3CN |
7 |
52 (32/20) |
0 |
5 |
DBU |
CH3CN |
24 |
0 |
52 |
6 |
LB-BA-1
|
CH2CI2 |
6 |
65 (35/30) |
0 |
7 |
LB-BA-1
|
CHCI3 |
6 |
68 (36/32) |
0 |
8 |
LB-BA-1
|
THF |
6 |
0 |
0 |
9 |
LB-BA-1
|
C2H5OH |
6 |
75 (42/31) |
0 |
10 |
LB-BA-1
|
PhMe |
6 |
89 (49/40) |
0 |
11 |
LB-BA-1
|
DMF |
6 |
0 |
0 |
12 |
LB-BA-1
|
DMSO |
6 |
0 |
0 |
13 |
LB-BA-1
|
PhMe |
24 |
75 (40/35)c |
0 |
14 |
LB-BA-1
|
PhMe |
40 |
70 (38/32)d |
0 |
15 |
LB-BA-1
|
PhMe |
24 |
62 (32/30)e |
|
Next, we examined how the choices of solvent affected this reaction. As shown in the Table 1, toluene was an excellent solvent (entry 10), but dichloromethane, chloroform and ethanol were not (entries 6, 7 and 9). Using THF, DMF and DMSO failed to produce the desired product (entries 8, 11 and 12). By comparison of the reactions carried out at different temperatures, we found that 30 °C was the optimal temperature for this reaction (entries 13 and 14). Lower reaction temperatures required longer reaction times. Finally, lowering the catalyst loading (10 mol%) led to a slow conversion with a decreased yield of 3a (entry 15).
Under the optimized reaction conditions, we next carried out the aza-MBH reaction of methyl vinyl ketone with a number of other salicyl N-thiophosphoryl imines catalyzed by bifunctional phosphine organocatalysts. The results are summarized in Table 2. In general, functionalized salicyl N-thiophosphoryl imines can be transformed to the corresponding chroman derivatives in 61–92% yields. For instance, with salicyl N-thiophosphoryl imines bearing electron withdrawing groups on the benzene ring (entries 1, 2 and 3), the reactions were catalyzed by LB-BA-1 to give the desired products within a short reaction time. This is because the electron withdrawing groups improve the reactivity of salicyl N-thiophosphoryl imines. The reaction yield of 3c is slight lower than 3b, which is most likely attributed to the steric hindrance adjacent to the oxygen atom of salicyl N-thiophosphoryl imines. In contrast, for the nitro substituted imine, long reaction times were needed and a moderate yield was obtained (entry 4), since the strong electron withdrawing property of the nitro group could have reduced the nucleophilicity of the neighboring oxygen atom. On the other hand, for salicyl N-thiophosphoryl imines bearing electron donating groups on the benzene ring, the electrophilicity of the salicyl N-thiophosphoryl imines was decreased. Therefore, the nucleophilic catalyst LB-BA-2 and a long reaction time were needed, affording the high yield of chroman derivatives with the almost 1
:
1 cis and trans isomers (entries 5, 6, 7 and 8). However, in the case of the salicyl N-thiophosphoryl imine with a tert-butyl group (entry 5), the anti-isomer chroman product was the main product. This may be because of the steric hindrance of the tert-butyl group.
Table 2 The substrate scope of the domino aza-MBH reactiona
Entry |
R |
Catalyst |
Time (h) |
Yield (cis/trans) (%)b |
Reactions were carried out using 1 (1.0 eq), methyl vinyl ketone (1.2 eq) and LB-BA (0.2 eq) in toluene at room temperature.
Isolated yield.
|
1 |
5-Br |
LB-BA-1
|
6 |
3a 89 (49/40) |
2 |
5-CI |
LB-BA-1
|
6 |
3b 92 (47/45) |
3 |
3-CI |
LB-BA-1
|
8 |
3c 82 (42/40) |
4 |
5-NO2 |
LB-BA-1
|
24 |
3d 61 (31/30) |
5 |
5-tBu |
LB-BA-2
|
12 |
3e 76 (21/55) |
6 |
H |
LB-BA-2
|
12 |
3f 86 (45/41) |
7 |
5-CH3 |
LB-BA-2
|
12 |
3g 88 (46/42) |
8 |
3-CH3 |
LB-BA-2
|
12 |
3h 72 (40/32) |
We also carried out the domino reaction catalyzed by DBU in toluene at room temperature. The results are shown in Table 3. Chromens were obtained in good yields irrespective of the electron-deficient and electron-rich salicyl N-thiophosphoryl imines. The mechanism of this domino reaction was unclear.
Table 3 Domino reactions catalyzed by DBUa
Entry |
R |
Time (h) |
Yield (%)b |
Reactions were carried out using 1 (1.0 eq), methyl vinyl ketone (1.2 eq) and DBU (0.2 eq) in toluene at room temperature.
Isolated yield.
|
1 |
5-Br |
24 |
4a 56 |
2 |
5-CI |
24 |
4b 58 |
3 |
5-tBu |
24 |
4c 59 |
Generally, there might be two possible mechanisms for this type of domino reaction based on the previous reports (Scheme 2). Path I is started with a Michael addition and followed by a Mannich reaction. Path II is initiated by an aza-MBH reaction and then a Michael addition reaction occurred. In order to clarify the mechanism, we carried out the reaction between 4-bromophenol and methyl vinyl ketone under the standard reaction conditions. The oxa-Michael addition reaction did not occur (Scheme 3a). Based on the results by Tang and coworkers,4h the aza-MBH reaction between (E)-N-benzylidene-P,P-diphenylphosphinothioic amide and methyl vinyl ketone smoothly gave the desired product catalyzed by PPh3 (Scheme 3b). Thus, we proposed a possible mechanism for this domino reaction as follows. The bifunctional phosphine catalyst LB-BA-1 first attacks the β carbon of 2 to produce intermediate A. The intermediate A subsequently undergoes an aza-MBH reaction to give the intermediate B, which will form intermediate Cvia proton transfer from the OH to the nitrogen anion. Finally, oxa-Michael addition takes place to produce the product 3a and regenerates LB-BA-1 (Scheme 4).
 |
| Scheme 2 The plausible reaction pathways for the domino reaction. | |
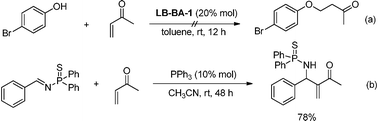 |
| Scheme 3 Experiments for mechanistic study. | |
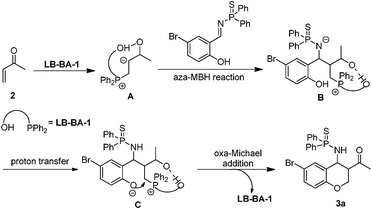 |
| Scheme 4 The possible catalytic cycle. | |
Conclusions
In summary, we developed a domino reaction between salicyl N-thiophosphoryl imines and methyl vinyl ketone catalyzed by bifunctional phosphine catalysts and DBU. The reaction proceeded under mild conditions to produce densely functionalized chroman and chromen derivatives. Our future efforts will focus on developing the asymmetric versions of this reaction and the application of the method described here in the construction of natural products.
Experimental section
All the solvents were purified according to standard procedures. All the reagents were obtained commercially and used without further purification. 1H, 13C and 31P NMR spectra were recorded on a Varian Mercury Plus 400 spectrometer or Bruker 300. 1H and 13C NMR chemical shifts were calibrated to tetramethylsilane as the external reference. 31P NMR chemical shifts were calibrated to 85% H3PO4 as the external standard. The proton and carbon spectral chemical shifts are reported in ppm, respectively. X-Ray diffraction analysis was performed on a Bruker Smart-1000 X-ray diffraction meter. HRMS (high-resolution mass spectrometry) was recorded on a VG ZAB-HS mass spectrometer with an EI source or an IonSpec FT-ICR (Fourier transform ion cyclotron resonance) mass spectrometer with a MALDI source.
The typical procedure for the preparation of 3
To a 25 ml round bottom flask with salicyl N-thiophosphoryl imine 0.48 mmol and LB-BA-1 33.98 mg (0.096 mmol), methyl vinyl ketone (47 μl, 0.58 mmol) and toluene (2 ml) were added. The solution was stirred at 30 °C for various lengths of time (see Table 2). Subsequently, the solvent was removed under reduced pressure. The pure product was obtained by silica gel column chromatography.
N-(cis-3-Acetyl-6-bromochroman-4-yl)-P,P-diphenylphosphinothioic amide (3a-cis).
White solid; mp: 175–177 °C; 1H NMR (300 MHz, CDCl3) δ 2.17 (s, 3H), 3.45 (dd, J = 4.8, 7.8 Hz, 1H), 3.76 (dd, J = 6.9, 10.8 Hz, 1H), 4.18 (dd, J = 2.7, 12.3 Hz, 1H), 4.39 (dd, J = 5.4, 12.3 Hz, 1H), 4.89 (ddd, J = 5.1, 11.1, 15.9 Hz, 1H), 6.50 (d, J = 8.7 Hz, 1H), 7.07 (dd, J = 2.4, 8.7 Hz, 1H), 7.33–7.42 (m, 6H), 7.47 (d, J = 2.1 Hz, 1H), 7.72–7.92 (m, 4H). 13C NMR (75 MHz, CDCl3) δ 28.97, 47.48, 49.96, 65.60, 113.27, 118.30, 126.31, 126.41, 128.36, 128.57, 128.74, 131.09, 131.24, 131.45, 131.66, 131.81, 131.90, 131.94, 132.04, 153.13, 207.84. 31P NMR (121.5 MHz, CDCl3) δ 58.56 ppm. HRMS for C23H21BrNO2PSNa [M + Na]+, calcd 508.0106, found 508.0110.
N-(trans-3-Acetyl-6-bromochroman-4-yl)-P,P-diphenylphosphinothioic amide (3a-trans).
White solid; mp: 149–151 °C; 1H NMR (300 MHz, CDCl3) δ 2.13 (s, 3H), 2.96 (t, J = 7.0, 1H), 3.39 (d, J = 2.4 Hz, 1H), 4.26 (dd, J = 2.7, 12 Hz, 1H), 4.58–4.62 (m, 1H), 4.89 (t, J = 9.4 Hz, 1H), 6.64 (d, J = 8.7 Hz, 1H), 7.20 (dd, J = 2.1, 8.7 Hz, 1H), 7.29 (d, J = 1.8 Hz, 1H), 7.44–7.55 (m, 6H), 7.88–8.06 (m, 4H). 13C NMR (75 MHz, CDCl3) δ 28.10, 45.51, 53.05, 62.43, 113.24, 119.03, 124.17, 124.28, 128.59, 128.65, 128.76, 128.82, 131.21, 131.36, 131.91, 132.06, 132.24, 133.11, 153.17, 205.11. 31P NMR (121.5 MHz, CDCl3) δ 57.58 ppm. HRMS for C23H21BrNO2PSNa [M + Na]+, calcd 508.0106, found 508.0103.
N-(cis-3-Acetyl-6-chlorochroman-4-yl)-P,P-diphenylphosphinothioic amide (3b-cis).
White solid; mp: 178–179 °C; 1H NMR (300 MHz, CDCl3) δ 2.16 (s, 3H), 3.48 (dd, J = 4.5, 7.2 Hz, 1H), 3.76 (dd, J = 6.6, 10.8 Hz, 1H), 4.18 (dd, J = 2.4, 12.3 Hz, 1H), 4.40 (dd, J = 5.1, 12.3 Hz, 1H), 4.86 (ddd, J = 5.1, 11.1, 15.9 Hz, 1H), 6.55 (d, J = 8.7 Hz, 1H), 6.94 (dd, J = 2.4, 8.7 Hz, 1H), 7.35–7.38 (m, 6H), 7.39 (d, J = 2.7 Hz, 1H), 7.74–7.89 (m, 4H). 13C NMR (75 MHz, CDCl3) δ 28.90, 47.64, 49.96, 65.72, 117.85, 125.83, 125.93, 125.99, 128.01, 128.55, 128.74, 131.11, 131.26, 131.65, 131.80, 131.94, 131.98, 152.62, 207.86. 31P NMR (121.5 MHz, CDCl3) δ 58.49 ppm. HRMS for C23H21ClNO2PSNa [M + Na]+, calcd 464.0611, found 464.0606.
N-(trans-3-Acetyl-6-chlorochroman-4-yl)-P,P-diphenylphosphinothioic amide (3b-trans).
White solid; mp: 66–68 °C; 1H NMR (400 MHz, CDCl3) δ 2.15 (s, 3H), 2.91 (t, J = 7.0 Hz, 1H), 3.43 (s, 1H), 4.27 (d, J = 12.0 Hz, 1H), 4.61 (d, J = 11.2 Hz, 1H), 4.87 (t, J = 9.4 Hz, 1H), 6.69 (dd, J = 2.8, 8.8 Hz, 1H), 7.06 (d, J = 8.8 Hz, 1H), 7.15 (s, 1H), 7.44–7.55 (m, 6H), 7.90–8.05 (m, 4H). 13C NMR (100 MHz, CDCl3) δ 28.30, 45.78, 53.23, 62.66, 118.82, 121.21, 121.94, 123.81, 123.86, 128.80, 128.87, 128.93, 128.99, 129.61, 130.31, 131.44, 131.55, 132.12, 132.23, 132.47, 152.84, 205.29. 31P NMR (162 MHz, CDCl3) δ 58.47 ppm. HRMS for C23H21ClNO2PSNa [M + Na]+, calcd 464.0611, found 464.0608.
N-(cis-3-Acetyl-8-chlorochroman-4-yl)-P,P-diphenylphosphinothioic amide (3c-cis).
Colorless oil; 1H NMR (400 MHz, CDCl3) δ 2.21 (s, 3H), 3.52 (br, 1H), 3.78 (dd, J = 6.8, 10.4 Hz, 1H), 4.35 (d, J = 12.0 Hz, 1H), 4.57 (dd, J = 5.6, 12.0 Hz, 1H), 5.03 (ddd, J = 4.8, 11.2, 12.0 Hz, 1H), 6.79 (t, 1H), 7.16 (d, J = 7.6 Hz, 1H), 7.39–7.47 (m, 7H), 7.81–7.90 (m, 4H). 13C NMR (100 MHz, CDCl3) δ 29.24, 47.84, 50.13, 66.10, 121.39, 126.18, 126.26, 127.17, 128.74, 128.87, 129.44, 131.41, 131.52, 131.76, 131.87, 132.11, 149.86, 207.82. 31P NMR (162 MHz, CDCl3) δ 58.43 ppm. HRMS for C23H21ClNO2PSNa [M + Na]+, calcd 464.0611, found 464.0609.
N-(trans-3-Acetyl-8-chlorochroman-4-yl)-P,P-diphenylphosphinothioic amide (3c-trans).
White solid; mp: 206–207 °C; 1H NMR (400 MHz, CDCl3) δ 2.13 (s, 3H), 2.95 (t, J = 7.0 Hz, 1H), 3.40 (s, 1H), 4.38 (d, J = 12.0 Hz, 1H), 4.74 (d, J = 11.6 Hz, 1H), 4.94 (t, J = 9.2 Hz, 1H), 6.82 (t, 1H), 7.18–7.21 (m, 2H), 7.42–7.53 (m, 6H), 7.88–8.02 (m, 4H). 13C NMR (100 MHz, CDCl3) δ 28.33, 45.83, 53.26, 63.32, 121.74, 124.31, 124.39, 126.44, 128.78, 128.82, 128.90, 128.95, 129.31, 130.00, 131.49, 131.60, 131.99, 132.10, 132.25, 132.34, 150.01, 205.12. 31P NMR (162 MHz, CDCl3) δ 58.39 ppm. HRMS for C23H21ClNO2PSNa [M + Na]+, calcd 464.0611, found 464.0610.
N-(cis-3-Acetyl-6-nitrochroman-4-yl)-P,P-diphenylphosphinothioic amide (3d-cis).
1H NMR (400 MHz, CDCl3) δ 2.30 (s, 3H), 3.76 (dd, J = 7.5, 4.3 Hz, 1H) 3.91 (dd, J = 11.0, 6.7 Hz, 1H), 4.41 (dd, J = 12.4, 2.8 Hz, 1H), 4.66 (dd, J = 12.4, 4.2 Hz, 1H), 4.93 (td, J = 11.0, 4.9 Hz, 1H), 6.78 (d, J = 8.8 Hz, 1H), 7.47–7.56 (m, 6H), 7.89 (dd, J = 13.5, 7.1 Hz, 2H), 7.97 (dd, J = 9.0, 2.7 Hz, 1H), 8.07–7.99 (m, 2H), 8.48 (d, J = 2.5 Hz, 1H). 31P NMR (162 MHz, CDCl3) δ 58.80 ppm. 13C NMR (100 MHz, CDCl3) δ 28.79, 47.68, 49.43, 66.72, 117.08, 124.32, 124.39, 125.26, 125.35, 128.69, 128.81, 131.01, 131.12, 131.77, 131.88, 132.05, 132.08, 132.12, 132.15, 132.72, 133.74, 133.97, 134.99, 141.81, 159.13, 207.59. HRMS for C23H21N2O4PSNa [M + Na]+, calcd 475.0852, found 475.0849.
N-(trans-3-Acetyl-6-nitrochroman-4-yl)-P,P-diphenylphosphinothioic amide (3d-trans).
1H NMR (400 MHz, CDCl3) δ 2.19 (s, 3H), 2.99 (dd, J = 8.8, 6.0 Hz, 1H), 3.65 (dd, J = 4.2, 2.4 Hz, 1H), 4.39 (dd, J = 12.0, 2.8 Hz, 1H), 4.69–4.76 (m, 1H), 4.91 (t, J = 9.8 Hz, 1H), 6.84 (d, J = 9.2 Hz, 1H), 7.42–7.49 (m, 2H), 7.51 (dd, J = 7.2, 1.6 Hz, 1H), 7.54–7.64 (m, 3H), 7.93 (dd, J = 14.6, 7.2 Hz, 2H), 7.99 (dd, J = 9.2, 2.8 Hz, 1H), 8.08 (ddd, J = 9.5, 7.6, 1.4 Hz, 2H), 8.13 (d, J = 2.8 Hz, 1H). 31P NMR (162 MHz, CDCl3) δ 58.03 ppm. 13C NMR (100 MHz, CDCl3) δ 28.15, 45.61, 52.62, 63.06, 117.92, 122.68, 122.77, 124.89, 127.06, 128.66, 128.81, 128.95, 131.12, 131.23, 132.02, 132.13, 132.18, 132.21, 132.39, 132.46, 132.49, 133.39, 133.54, 134.57, 141.74, 159.11, 204.76. HRMS for C23H21N2O4PSNa [M + Na]+, calcd 475.0852, found 475.0856.
N-(cis-3-Acetyl-6-(tert-butyl)chroman-4-yl)-P,P-diphenylphosphinothioic amide (3e-cis).
Colorless oil; 1H NMR (400 MHz, CDCl3) δ 1.20 (s, 9H), 2.25 (s, 3H), 3.48 (br, 1H), 3.68 (t, J = 6.8 Hz, 1H), 4.31 (dd, J = 2.4, 12.0 Hz, 1H), 4.42 (dd, J = 6.4, 12.0 Hz,1H), 5.19 (ddd, J = 4.4, 11.2, 15.2 Hz, 1H), 6.66 (d, J = 8.8 Hz, 1H), 7.12 (d, J = 8.8 Hz, 1H), 7.37–7.50 (m, 6H), 7.53 (s, 1H), 7.77–7.91 (m, 4H). 13C NMR (100 MHz, CDCl3) δ 29.43, 31.64, 34.37, 47.88, 50.88, 65.09, 116.20, 123.34, 123.40, 125.79, 126.11, 128.62, 128.67, 128.75, 128.80, 131.34, 131.45, 131.80, 131.91, 143.94, 151.85, 208.38. 31P NMR (162 MHz, CDCl3) δ 59.41 ppm. HRMS for C27H30NO2PSNa [M + Na]+, calcd 486.1627, found 486.1629.
N-(trans-3-Acetyl-6-(tert-butyl)chroman-4-yl)-P,P-diphenylphosphinothioic amide (3e-trans).
White solid; mp: 192–194 °C; 1H NMR (400 MHz, CDCl3) δ 1.24 (s, 9H), 2.17 (s, J = 7.0 Hz, 3H), 2.95 (t, 1H), 3.43 (d, J = 2.4 Hz, 1H), 4.28 (dd, J = 2.4, 11.6 Hz, 1H), 4.60–4.64 (m, 1H), 4.91 (t, J = 9.4 Hz, 1H), 6.71 (d, J = 8.8 Hz, 1H), 7.17 (dd, J = 2.4, 8.8 Hz, 1H), 7.23 (d, J = 2.4 Hz, 1H), 7.43–7.56 (m, 6H), 7.87–8.08 (m, 4H). 13C NMR (100 MHz, CDCl3) δ 20.75, 28.35, 31.79, 46.00, 53.67, 62.51, 117.08, 121.97, 122.04, 128.74, 128.87, 130.33, 130.77, 130.94, 131.48, 131.60, 132.11, 132.22, 152.10, 205.75. 31P NMR (162 MHz, CDCl3) δ 58.19 ppm. HRMS for C27H30NO2PSNa [M + Na]+, calcd 486.1627, found 486.1632.
N-(cis-3-Acetylchroman-4-yl)-P,P-diphenylphosphinothioic amide (3f-cis).
White solid; mp: 120–121 °C; 1H NMR (400 MHz, CDCl3) δ 2.24 (s, 3H), 3.58 (br, 1H), 3.81 (dd, J = 6.8, 10.8 Hz, 1H), 4.30 (dd, J = 2.4, 12.0 Hz, 1H), 4.48 (dd, J = 4.2, 12.0 Hz, 1H), 5.00 (ddd, J = 4.8, 10.8, 14.8 Hz, 1H), 6.72 (d, J = 8.0 Hz, 1H), 6.89 (t, J = 7.6, 7.6 Hz, 1H), 7.10 (t, J = 7.6, 7.6 Hz, 1H), 7.42–7.50 (m, 6H), 7.56 (d, J = 7.6 Hz, 1H), 7.86–7.95 (m, 4H). 13C NMR (100 MHz, CDCl3) δ 28.96, 47.82, 50.28, 65.41, 116.48, 117.45, 118.98, 119.26, 119.91, 121.17, 128.61, 128.78, 131.08, 131.23, 131.91, 131.95, 134.79, 136.05, 154.00, 208.03. 31P NMR (162 MHz, CDCl3) δ 59.18 ppm. HRMS for C23H22NO2PSNa [M + Na]+, calcd 430.1001, found 430.1005.
N-(trans-3-Acetylchroman-4-yl)-P,P-diphenylphosphinothioic amide (3f-trans).
Colorless oil; 1H NMR (400 MHz, CDCl3) δ 2.15 (s, 3H), 2.91 (t, J = 7.0 Hz, 1H), 3.42 (d, J = 2.4 Hz, 1H), 4.32 (dd, J = 2.4, 11.6 Hz, 1H), 4.64 (dd, J = 1.6, 11.6 Hz, 1H), 4.95 (t, J = 9.4 Hz, 1H), 6.78 (d, J = 8.0 Hz, 1H), 6.91 (t, J = 7.2, 7.2 Hz, 1H), 7.15 (t, J = 7.2, 7.2 Hz, 1H), 7.29 (s, 1H), 7.43–7.56 (m, 6H), 7.92–8.07 (m, 4H). 31P NMR (162 MHz, CDCl3) δ 57.99 ppm. HRMS for C23H22NO2PSNa [M + Na]+, calcd 430.1001, found 430.1008.
N-(cis-3-Acetyl-6-methylchroman-4-yl)-P,P-diphenylphosphinothioic amide (3g-cis).
White solid; mp: 120–121 °C; 1H NMR (400 MHz, CDCl3) δ 2.15 (s, 3H), 2.28 (s, 3H), 3.47–3.50 (m, 1H), 3.69 (dd, J = 6.8, 10.4 Hz, 1H), 4.28 (dd, J = 2.4, 12.0 Hz, 1H), 4.42 (dd, J = 6.4, 12.0 Hz, 1H), 5.05 (ddd, J = 4.8, 11.2, 15.6 Hz, 1H), 6.60 (d, J = 8.0 Hz, 1H), 6.87 (d, J = 8.4 Hz, 1H), 7.15 (s, 1H), 7.41–7.50 (m, 6H), 7.78–7.93 (m, 4H). 13C NMR (100 MHz, CDCl3) δ 20.83, 29.39, 47.69, 50.73, 65.19, 116.41, 123.78, 123.84, 128.58, 128.70, 128.83, 129.22, 129.54, 130.40, 131.31, 131.42, 131.95, 132.06, 151.94, 208.32. 31P NMR (162 MHz, CDCl3) δ 59.31 ppm. HRMS for C24H25NO2PS [M + H]+, calcd 422.1338, found 422.1334.
N-(trans-3-Acetyl-6-methylchroman-4-yl)-P,P-diphenylphosphinothioic amide (3g-trans).
Colorless oil; 1H NMR (400 MHz, CDCl3) δ 2.11 (s, 3H), 2.19 (s, 3H), 2.98 (t, J = 7.0 Hz, 1H), 3.29 (d, J = 2.4 Hz, 1H), 4.27 (dd, J = 2.8 Hz, 11.6Hz, 1H), 4.55–4.59 (m, 1H), 4.93 (t, J = 9.4 Hz, 1H), 6.65 (d, J = 8.4 Hz, 1H), 6.91 (dd, J = 2.0, 8.0 Hz, 1H), 6.97 (s, 1H), 7.40–7.55 (m, 6H), 7.89–8.05 (m, 4H). 13C NMR (100 MHz, CDCl3) δ 20.75, 28.35, 46.00, 53.67, 62.51, 117.08, 121.97, 122.04, 128.74, 128.87, 130.33, 130.77, 130.94, 131.48, 131.60, 132.11, 132.22, 152.10, 205.75. 31P NMR (162 MHz, CDCl3) δ 58.15 ppm. HRMS for C24H25NO2PS [M + H]+, calcd 422.1338, found 422.1336.
N-(cis-3-Acetyl-8-methylchroman-4-yl)-P,P-diphenylphosphinothioic amide (3h-cis).
1H NMR (400 MHz, CDCl3) δ 2.11 (s, 3H), 2.25 (s, 3H), 3.57 (dd, J = 8.0, 4.2 Hz, 1H), 3.74 (dd, J = 10.6, 6.6 Hz, 1H), 4.34 (dd, J = 12.0, 2.8 Hz, 1H), 4.52 (dd, J = 12.0, 5.6 Hz, 1H), 5.03 (td, J = 11.0, 5.0 Hz, 1H), 6.79 (t, J = 7.4 Hz, 1H), 6.79 (d, J = 7.2 Hz, 1H), 7.37–7.53 (m, 7H), 7.91 (td, J = 14.0, 7.3 Hz, 4H). 31P NMR (162 MHz, CDCl3) δ 58.08 ppm. 13C NMR (100 MHz, CDCl3) δ 15.92, 29.02, 47.93, 50.26, 65.21, 120.40, 123.63, 125.61, 125.92, 128.42, 128.55, 129.78, 131.23, 131.34, 131.62, 131.71, 131.74, 131.76, 131.79, 133.60, 134.53, 134.62, 135.55, 152.10, 208.11. HRMS for C24H25NO2PS [M + H]+, calcd 422.1338, found 422.1330.
N-(trans-3-Acetyl-8-methylchroman-4-yl)-P,P-diphenylphosphinothioic amide (3h-trans).
1H NMR (400 MHz, CDCl3) δ 2.13 (s, 3H), 2.15 (s, 3H), 2.90 (t, J = 7.0 Hz, 1H), 3.41 (dd, J = 5.6, 3.2 Hz, 1H), 4.33 (dd, J = 11.6, 2.8 Hz, 1H), 4.69 (d, J = 11.6 Hz, 1H), 4.95 (t, J = 9.4 Hz, 1H), 6.81 (t, J = 7.6 Hz, 1H), 7.00 (d, J = 6.8 Hz, 1H), 7.11 (d, J = 7.6 Hz, 1H), 7.40–7.59 (m, 6H), 7.94 (dd, J = 15.4, 7.0 Hz, 2H), 8.03 (dd, J = 13.6, 6.8 Hz, 2H). 31P NMR (162 MHz, CDCl3) δ 56.68 ppm. 13C NMR (100 MHz, CDCl3) δ 15.89, 28.16, 45.99, 53.18, 62.26, 120.73, 121.52, 121.61, 126.37, 128.05, 128.50, 128.55, 128.63, 128.68, 130.34, 131.26, 131.37, 131.84, 131.92, 131.95, 132.02, 132.05, 133.12, 134.05, 134.12, 135.07, 152.27, 205.53. HRMS for C24H25NO2PS [M + H]+, calcd 422.1338, found 422.1332.
Typical procedure for the preparation of 4
To a 25 ml round bottom flask with salicyl N-thiophosphoryl imine 0.48 mmol and DBU 14.61 mg (0.096 mmol), methyl vinyl ketone (47 μl, 0.58 mmol) and toluene (2 ml) were added. The solution was stirred at 30 °C for 24 h. Then, the solvent was removed under reduced pressure. The pure product was obtained by silica gel column chromatography.
1-(6-Bromo-2H-chromen-3-yl)ethanone (4a)14.
White solid; mp: 67–69 °C; 1H NMR (300 MHz, CDCl3) δ 2.33 (s, 3H), 4.92 (s, 2H), 6.67 (d, J = 8.4 Hz, 1H), 7.14 (s, 1H), 7.19–7.21 (m, 1H), 7.25 (dd, J = 2.4, 8.7 Hz, 1H). 13C NMR (75 MHz, CDCl3) δ 25.07, 64.43, 113.61, 118.03, 122.53, 131.22, 131.64, 132.27, 134.74, 154.52, 195.65.
1-(6-Chloro-2H-chromen-3-yl)ethanone (4b)14.
White solid; mp: 55–56 °C; 1H NMR (300 MHz, CDCl3) δ 2.41 (s, 3H), 5.00 (d, J = 1.2 Hz, 2H), 6.80 (d, J = 8.7 Hz, 1H), 7.14 (d, J = 2.4 Hz, 1H), 7.18–7.22 (m, 2H).). 13C NMR (75 MHz, CDCl3) δ 25.07, 64.45, 117.65, 121.99, 126.51, 128.33, 131.90, 132.46, 154.02,
1-(6-(tert-Butyl)-2H-chromen-3-yl)ethanone (4c).
Colorless oil; 1H NMR (400 MHz, CDCl3) δ 1.30 (s, 9H), 2.41 (s, 3H), 4.98 (s, 2H), 6.79 (d, J = 8.4 Hz, 1H), 7.18 (d, J = 2.4 Hz, 1H), 7.29 (dd, J = 2.4, 8.4 Hz, 1H), 7.34 (s, 1H). 13C NMR (100 MHz, CDCl3) δ 25.19, 31.57, 34.34, 64.45, 115.99, 120.38, 126.14, 129.91, 130.83, 134.92, 144.86, 153.61, 196.24. HRMS for C15H18O2Na [M + Na]+, calcd 253.1199, found 253.1190.
Acknowledgements
We thank the National Natural Science Foundation of China (20972076, 21172115) and the Natural Science Foundation of Tianjin (10JCYBJC04000) for financial support. We also thank Dr Christopher J. Hipolito for the revision of the manuscript.
References
- For reviews on domino reactions see:
(a)
L. F. Tietze, G. Brasche and K. Gericke, Domino Reactions in Organic Synthesis, Wiley-VCH, Weinheim, 2006 Search PubMed;
(b) H. C. Guo and J. A. Ma, Angew. Chem., Int. Ed., 2006, 45, 354 CrossRef CAS;
(c) L. F. Tietze and U. Beifuss, Angew. Chem., Int. Ed. Engl., 1993, 32, 131 CrossRef;
(d) L. F. Tietze, Chem. Rev., 1996, 96, 115 CrossRef CAS;
(e) D. Enders, C. Grondal and M. R. M. Hüttl, Angew. Chem., Int. Ed., 2007, 46, 1570 CrossRef CAS.
- For recent examples on organocatalytic reactions see:
(a) A.-N. Alba, X. V. M. Companyo and R. Rios, Curr. Org. Chem., 2009, 13, 1432 CrossRef CAS;
(b) P. Hélène, Adv. Synth. Catal., 2012, 354, 237 CrossRef;
(c) J. G. Hernandez and E. Juaristi, Chem. Commun., 2012, 48, 5396 RSC;
(d) D. Bonne, T. Constantieux, Y. Coquerel and J. Rodriguez, Org. Biomol. Chem., 2012, 10, 3969 RSC;
(e) I. P. Andrews, B. R. Blank and O. Kwon, Chem. Commun., 2012, 48, 5373 RSC;
(f) U. Das, C.-H. Huang and W. Lin, Chem. Commun., 2012, 48, 5590 RSC.
- For reviews on MBH reactions see:
(a) D. Basavaiah, A. J. Rao and T. Satyanarayana, Chem. Rev., 2003, 103, 811 CrossRef CAS;
(b) D. Basavaiah, K. V. Rao and R. J. Reddy, Chem. Soc. Rev., 2007, 36, 1581 RSC;
(c) G. Masson, C. Housseman and J. Zhu, Angew. Chem., Int. Ed., 2007, 46, 4614 CrossRef CAS.
- For reviews on aza-MBH reactions see:
(a) D. Basavaiah, B. S. Reddy and S. S. Badsara, Chem. Rev., 2010, 110, 5447 CrossRef CAS;
(b) V. Declerck, J. Martinez and F. Lamaty, Chem. Rev., 2009, 109, 1 CrossRef CAS;
(c) P. Perlmutter and C. C. Teo, Tetrahedron Lett., 1984, 25, 5951 CrossRef CAS;
(d) M. Shi and Y. M. Xu, Eur. J. Org. Chem., 2002, 696 CrossRef CAS;
(e) Y. M. Xu and M. Shi, J. Org. Chem., 2004, 69, 417 CrossRef CAS;
(f) M. Shi and G. L. Zhao, Tetrahedron Lett., 2002, 43, 4499 CrossRef CAS;
(g) M. Shi and G. L. Zhao, Adv. Synth. Catal., 2004, 346, 1205 CrossRef CAS;
(h) X. Xu, C. Wang, Z. Zhou, X. Tang, Z. He and C. Tang, Eur. J. Org. Chem., 2007, 4487 CrossRef CAS;
(i) M. J. Qi and M. Shi, Tetrahedron, 2007, 63, 10415 CrossRef CAS;
(j) Y.-L. Shi and M. Shi, Eur. J. Org. Chem., 2007, 2905 CrossRef CAS.
-
(a) M. Koufaki, E. Theodorou, D. Galaris, L. Nousis, E. S. Katsanou and M. N. Alexis, J. Med. Chem., 2006, 49, 300 CrossRef CAS;
(b) S. B. Lee, C. Y. L. Lin, P. M. W. Gill and R. D. Webster, J. Org. Chem., 2005, 70, 10466 CrossRef CAS;
(c) M. Toyota, I. Omatsu and Y. Asakawa, Chem. Pharm. Bull., 2001, 49, 924 CrossRef CAS.
-
(a) B. Lesch and S. Bräse, Angew. Chem., Int. Ed., 2004, 43, 115 CrossRef;
(b) K. Y. Lee, J. M. Kim and J. N. Kim, Bull. Korean Chem. Soc., 2003, 24, 17 CrossRef CAS;
(c) P. T. Kaya and X. W. Nocanda, J. Chem. Soc., Perkin Trans. 1, 2000, 1331 RSC;
(d) C. D. DeBoer, J. Org. Chem., 1974, 39, 2426 CrossRef CAS;
(e) V. Y. Korotaev, I. B. Kutyashev and V. Y. Sosnovskikh, Heteroat. Chem., 2005, 16, 492 CrossRef CAS.
-
(a) P. T. Kaya and R. S. Robinson, Synth. Commun., 1996, 26, 2085 CrossRef;
(b) P. T. Kaya, M. A. Musa and X. W. Nocanda, Synthesis, 2003, 531 CrossRef;
(c) P. T. Kaya, M. A. Musa, X. W. Nocanda and R. S. Robinson, Org. Biomol. Chem., 2003, 1, 1133 RSC.
-
(a) M. C. Yan, Y. J. Jang and C. F. Yao, Tetrahedron Lett., 2001, 42, 2717 CrossRef CAS;
(b) C. F. Yao, Y. J. Jang and M. C. Yan, Tetrahedron Lett., 2003, 44, 3813 CrossRef CAS;
(c) M. Nyerges, A. Virányi, G. Marth, A. Dancsό, G. Balaskό and L. Tőke, Synlett, 2004, 2761 CrossRef CAS.
- For selected recent examples on the synthesis of chromans see:
(a) F. Hua, X. Guan and M. Shi, Tetrahedron, 2012, 68, 4782 CrossRef;
(b) K.-S. Choi and S.-G. Kim, Eur. J. Org. Chem., 2012, 1119 CrossRef CAS;
(c) A. F. Ward, Y. Xu and J. P. Wolfe, Chem. Commun., 2012, 48, 609 RSC;
(d) J. S. Cannon, A. C. Olson, L. E. Overman and N. S. Solomon, J. Org. Chem., 2012, 77, 1961 CrossRef CAS;
(e) G. Urbanietz, R. Hahn and G. Raabe, Synthesis, 2012, 44, 773 CrossRef.
- X. Meng, Y. Huang, H. Zhao, P. Xie, J. Ma and R. Chen, Org. Lett., 2009, 11, 991 CrossRef CAS.
- X. Meng, Y. Huang and R. Chen, Org. Lett., 2009, 11, 137 CrossRef CAS.
- M. J. Qi and M. Shi, Tetrahedron, 2007, 63, 10415 CrossRef CAS.
-
(a) X. Meng, Y. Huang and R. Chen, Chem.–Eur. J., 2008, 14, 6852 CrossRef CAS;
(b) P. Xie, Y. Huang, W. Lai, X. Meng and R. Chen, Org. Biomol. Chem., 2011, 9, 6707 RSC;
(c) P. Xie, L. Wang, L. Yang, E. Li, J. Ma, Y. Huang and R. Chen, J. Org. Chem., 2011, 76, 7699 CrossRef CAS;
(d) J. Ma, P. Xie, C. Hu, Y. Huang and R. Chen, Chem.–Eur. J., 2011, 17, 7418 CrossRef CAS;
(e) X. Xie, Y. Huang and R. Chen, Org. Lett., 2010, 12, 3768 CrossRef;
(f) P. Xie, Y. Huang and R. Chen, Chem.-Eur. J., 2012, 18, 7362 CAS;
(g) P. Xie, W. Lai, Z. Geng, Y. Huang and R. Chen, Chem. Asian. J., 2012, 7, 1533 CrossRef CAS.
- P. T. Kaya and X. W. Nocanda, J. Chem. Soc., Perkin Trans. 1, 2000, 1331 RSC.
|
This journal is © The Royal Society of Chemistry 2012 |
Click here to see how this site uses Cookies. View our privacy policy here.