DOI:
10.1039/C2RA20702H
(Paper)
RSC Adv., 2012,
2, 10519-10528
Preparation and evaluation of the effect of particle size on the properties of chitosan-montmorillonite nanoparticles loaded with isoniazid
Received
18th April 2012
, Accepted 25th August 2012
First published on 29th August 2012
Abstract
In this report, efforts have been made to develop isoniazid loaded chitosan-montmorillonite nanoparticles by ionic gelation of chitosan with pentasodium tripolyphosphate. The nanoparticles have been characterized by FTIR, XRD, SEM and TEM. The effect of surfactant and particle size on chitosan nanoparticles have been assessed with regard to swelling, encapsulation efficiency and release of isoniazid in different mediums. Swelling experiments provide important information on drug diffusion properties, which indicates that the chitosan nanoparticles are highly sensitive to the pH environment. The drug release mechanism has been studied during different time periods using a UV-visible spectrophotometer. Cytotoxicity has been assessed by MTT assay analysis. Mucoadhesion properties have been appraised by an in vitro wash off test and an ex vivo mucoadhesion test. The results imply that chitosan-montmorillonite nanoparticles can be exploited as potential drug carriers for controlled-release applications.
1. Introduction
In the current scenario of pharmaceutical research, polymers have been extensively used as an active agent for drug delivery. They can control the release of a drug over a prolonged period by forming a matrix, a membrane or (nano)carriers thereby avoiding repetitive dosing.1 Generally, natural biodegradable polymers are preferred as controlled drug delivery systems due to their low toxicity, easy availability and cell biocompatibility.2 Among the natural polymers, chitosan plays an important role in drug delivery systems. Chitosan is an omnipresent modified natural polymer obtained from partial deacetylation of the biopolymer chitin, which is present in the shells of crustacean, such as crabs and lobsters.3 It can also be obtained from some microorganisms and yeasts. Chitosan is a mucoadhesive polycation polymer at acidic pH which is not noxious and is biocompatible.4–6 Chitosan also has a fungicidal effect, wound healing properties and can reduce cholesterol level.7 Owing to its cationic nature, chitosan has good mucoadhesive and membrane permeation enhancing properties.8
Nanoparticles have evoked much interest recently for the delivery of drugs such as peptides, proteins and genes due to their ability to protect these from degradation by proteolytic enzymes in the gastrointestinal tract. They possess mucoadhesive properties and are capable of being adhered to the mucosal tissues. Different bioactive compounds have been successfully encapsulated within polymers and have been tested for their therapeutic activity.9
Montmorillonite (MMT) clay is a smectite clay having silica tetrahedral sheets layered between alumina octahedral sheets. Due to its good physical and chemical properties, MMT has received considerable attention in recent years for drug delivery systems applications.10 Isoniazid is an antituberculosis drug which can be used as a model drug.
Various methods are used to prepare chitosan nanoparticles, such as coacervation, emulsion-droplet coalescence, reverse micelles, ionic gelation and self assembly chemical modification. Extensive research has been done to synthesize chitosan nanoparticles loaded with drugs by ionic gelation processes.11–14 The amine groups of chitosan are protonated to form ammonium ions in acidic media. These can be crosslinked either chemically with glutaraldehyde and genipin or physically with sodium tripolyphosphate, sulphate, citrate, etc. Varieties of crosslinkers are reported to be used for controlling the release behavior of drugs.14–16 The nanoparticles prepared by physical crosslinking swell faster in release media compared to those of chemically crosslinked nanoparticles. Therefore, a synergistic approach by using both physical and chemical crosslinking seems to be more viable to control the release behavior of a drug. A similar approach has reported by Gupta et.al..17 To the best of our knowledge, there is no report on synthesizing drug loaded chitosan-MMT nanoparticles by using this method. In this paper, efforts have been made to prepare isoniazid loaded chitosan-MMT nanoparticles by an ionic gelation method followed by chemical crosslinking with glutaraldehyde (GA). This report aims at the study of the effect of clay and surfactant on various properties of nanoparticles.
2. Experimental section
2.1. Materials
Low molecular weight chitosan, montmorillonite K-10, isoniazid, histopaque 1077 and [3-(4, 5-dimethylthiazol-2-yl)-2,5-diphenyl tetrazolium bromide] (MTT) were obtained from Sigma Aldrich, Germany. Tween 80, glutaraldehyde (25%) and sodium tripolyphosphate (TPP) were purchased from Merck, India. RPMI 1640 and fetal bovine serum (FBS) were procured from HiMedia Laboratories (Mumbai, India). The rest of the chemicals were of analytical grade and used directly.
Chitosan nanoparticles were prepared by ionic crosslinking of chitosan with sodium TPP and employing the process as reported by Calvo.11 1% (w/v) chitosan solution was prepared in water containing 0.5% (v/v) acetic acid. 0.005 g of MMT was swelled in 50 mL of water for 24 h. It was then stirred vigorously by mechanical stirring for 48 h and sonicated for 30 min. This dispersed MMT solution was added to 50 mL of 1% (w/v) chitosan solution. To this solution, isoniazid (0.01 g) and Tween 80 (0.5–5% v/w of chitosan i.e. 0.0025–0.025 mL) were added, stirred and sonicated for 15 min. Then, 1% (w/v) TPP solution was prepared in water. Nanoparticles were obtained by the slow addition of 15 mL of TPP solution to 100 mL of isoniazid containing chitosan-MMT solution under constant magnetic stirring at room temperature for 30 min. The temperature of the container containing nanoparticles was brought down to 5–10 °C to harden the nanoparticles. GA (0.1 mmol) was added and the temperature was increased to 45 °C. The reaction was continued for 45 min. The nanoparticles were separated by centrifuging the solution for 30 min. The product was washed several times with water and dried. The dried nanoparticles were kept in an ampule, stored in a refrigerator and redispersed in deionised water for further use. Scheme 1 and Scheme 2 depict the preparation process and the plausible mechanism of formation of isoniazid loaded chitosan- MMT nanoparticles. A series of six samples were prepared for the present study as represented in Table 1.
Sample code |
Chitosan (% w/v) (amount in g in 50 mL water) |
MMT (% w/v) w.r.t chitosan (amount in g in 50 mL water) |
Tween 80 (% v/w) w.r.t. chitosan (amount in mL) |
Isoniazid/g |
TPP (% w/v) w.r.t water (amount in g in 100 mL water) |
GA/mmol |
NPI |
1 (0.5) |
1 (0.005) |
0 (0.0) |
0.01 |
1 (1) |
0.1 |
NPII |
1 (0.5) |
1 (0.005) |
0.5 (0.0025) |
0.01 |
1 (1) |
0.1 |
NPIII |
1 (0.5) |
1 (0.005) |
1 (0.005) |
0.01 |
1 (1) |
0.1 |
NPIV |
1 (0.5) |
1 (0.005) |
3 (0.015) |
0.01 |
1 (1) |
0.1 |
NPV |
1 (0.5) |
1 (0.005) |
5 (0.025) |
0.01 |
1 (1) |
0.1 |
NPVI |
1 (0.5) |
0 (0.00) |
5 (0.025) |
0.01 |
1 (1) |
0.1 |
2.3. Calculation of process yield
Process yield was calculated using the following equation as described in the literature.18
Process Yield (%) = [(Weight of |
2.4. Calibration curve of isoniazid
A calibration curve is essential to estimate the release rate of drug from nanoparticles in a suitable solvent medium. The calibration curve was drawn as per a previously reported procedure.19
A known concentration of isoniazid (in double distilled water) was scanned in the range 200–400 nm by using a UV-Visible spectrophotometer (UV-2001Hitachi, Tokyo, Japan). A sharp peak at 262 nm was noticed for isoniazid having a concentration in the range of 0.001–0.01 g/100 mL. The absorbance values at 262 nm obtained with the respective concentration were recorded and plotted. From this calibration curve, the unknown concentration of isoniazid was obtained by knowing the absorbance value.
2.5. Calculation of drug loading efficiency and encapsulation efficiency of the nanoparticles
The drug loading efficiency of nanoparticles with different formulations was determined by ultracentrifugation of samples at room temperature for 30 min. The amount of free isoniazid was determined by noting the absorbance value of the supernatant at 262 nm using a UV-Visible spectrophotometer. The drug loading efficiency (LE) of the nanoparticles was calculated using the following equations.11
2.6. Fourier transmission infra-red spectroscopy (FTIR) study
FTIR spectra of nanoparticles were taken using a Nicolet (model Impact-410) spectrophotometer. The nanoparticles were ground to a powder, mixed with KBr and spectra were recorded in the range of 4000–400 cm−1.
The degree of intercalation of MMT and the distribution of isoniazid in chitosan-clay nanoparticles were examined by X-ray diffractometry. It was carried out in a Rigaku X-ray diffractometer (Miniflax, UK) using Cu-Kα (λ = 0.154 nm) radiation at a scanning rate of 1°min−1 with an angle ranging from 2° to 70° of 2θ.
2.8. Particle size determination
Particle size was determined by a dynamic light scattering (DLS) analyzer (model DLS—Nano ZS, Zetasizer, Nanoseries, Malvern Instruments).
The samples were mounted on a brass holder sputtered with platinum. The surface morphologies of chitosan-MMT nanoparticles loaded with isoniazid were studied by using a scanning electron microscope (JEOL JSM–6390LV) at an accelerated voltage of 5–10 KV.
2.10. Transmission emission microscopy (TEM) study
The dispersion of the silicate layers of clay in chitosan nanoparticles was examined by using a transmission electron microscope (JEOL JEM-2100) at an accelerated voltage of 100 KV.
2.11. Water uptake studies
Water uptake studies were performed in both phosphate buffer (pH 7.4) and 0.1 N HCl solution (pH 1.2) according to the procedure described in the literature.20
Nanoparticles (0.1 g) were taken in a pouch made of nylon cloth. The empty pouch was first conditioned by immersing it in either 0.1 N HCl (pH 1.2) or phosphate buffer (pH 7.4) for different time periods (1–8 h). The pouch containing the nanoparticles was immersed in a similar way in either 0.1 N HCl (pH 1.2) or phosphate buffer (pH 7.4) for similar time periods. The weights of wet nanoparticles after a definite time period were determined by deducting the respective conditioned weight of the empty nylon pouch from this. Water uptake (%) was determined by measuring the change in the weight of the nanoparticles. The percentage of water uptake for each sample determined at time t was calculated using the following equation.
Water uptake (%) = [(w2 − w1)/w1] × 100 |
where, w1 is initial weight of nanoparticles before swelling, and w2 is the final weight of nanoparticles after swelling for a predetermined time t.
The experiments were performed in triplicate and represented as a mean value.
2.12.
In vitro drug release studies
To study the release profile of the isoniazid loaded chitosan-MMT nanoparticles, dried drug loaded samples were immersed in a solution of different pH, namely 1.2 and 7.4, and stirred continuously. At scheduled time intervals, 5 mL solution was withdrawn, filtered and assayed spectrophotometrically at 262 nm by using a UV-Visible spectrophotometer for the determination of the cumulative amount of drug release up to a time t. To maintain a constant volume, 5 mL of the solution having same pH was returned to the container.21,22 Each determination was carried out in triplicate.
2.13. Isolation of lymphocytes, culture and treatment
Chicken blood was collected from source. It was diluted in a 1
:
1 ratio with phosphate buffer saline (PBS) and 6 mL was layered into 6 mL histopaque (1.077 g mL−1). The isolation of lymphocytes and the study of cell viability were done as per the procedure cited in the literature.23 Lymphocytes were isolated from the sample after centrifugation for 30 min at 400 g, washed with PBS and finally with serum free media separately through centrifugation for 10 min at 250 g. Cell pellets were then suspended in PBS and cell viability was checked by the Trypan blue exclusion method using a haemocytometer. Cell viability more than 90% was used for subsequent study.
Aliquots of 200 μL of isolated cells were cultured in RPMI supplemented with 10% heat inactivated FBS. Initially cells were maintained for 4 h in RPMI without FBS at 37 °C in 5% CO2 in an incubator. Cells were then treated as per experimental requirements and maintained in the presence of FBS for 6 h, 12 h and 24 h.
2.14. Cytotoxicity experiments
A cytotoxicity assay was performed by measuring the viability of cells according to the method as described by Denizot and Lang.24 The key component [3-(4, 5-dimethylthiazol-2-yl)-2, 5-diphenyl tetrazolium bromide] (MTT) is yellowish in color and mitochondrial dehydrogenase of viable cells cleave the tetrazolium ring, yielding purple insoluble formazan crystals, which were dissolved in a suitable solvent. In this report, DMSO is used as the control solvent. The resulting purple solution was spectrophotometrically measured. An increase or decrease in cell number resulted in a concomitant change in the amount of formazan formed, indicating the degree of cytotoxicity caused by the test material. Briefly, after treatments, cells were treated with 10% of MTT for 2 h followed by dissolving the formazan crystals in solvent and measuring the absorbance of solution at 570 nm. The absorbance of control cells at 6, 12 and 24 h were separately set as 100% viability and the values of treated cells were calculated as a percentage of the control.
2.15. Mucoadhesion study
2.15.1.
In vitro wash-off test.
The mucoadhesive property of the nanoparticles was evaluated by an in vitro adhesion test method known as the wash-off test method.25 Freshly excised pieces of goat intestinal mucosa (5 × 5 cm) were mounted with the mucous side exposed on to glass slides with cotton thread. About 50 nanoparticles were spread onto each prepared glass slide and immediately thereafter the slides were hung up to a USP tablet disintegration test apparatus (Tab. Machines, Mumbai). When the test apparatus was operated, the sample was subjected to slow up and down movement in the test fluid at 37 °C contained in the 1L vessel of the apparatus. Readings were taken at intervals of 30 min up to 5 h by stopping the machine and counting the number of nanoparticles still adhering to the mucosal surface. The test was performed at intestinal (pH 7.4) and simulated gastric fluid (pH 1.2) conditions.
2.15.2.
Ex vivo mucoadhesive test.
In this method, the force required to separate bio-adhesive samples from freshly excised goat intestine was determined.26 Keeping the mucosal side out, the intestine was secured on to each glass vial using nylon thread. The diameter of each exposed mucosal membrane was 2 cm. The vials with the nasal tissue were kept at 37 °C for 10 min. To the exposed tissue on this vial, a constant amount of nanoparticle was applied. The height of the vial was adjusted so that the nanoparticles could adhere to the mucosal tissues of both vials. Water was added at a constant rate to the pan on the other side of the modified balance until the two vials were separated. The weight of water showed the weight required for displacement.
The adhesive force expressed as the detachment stress (dyne cm−2) is calculated using the following equation.
Detachment stress (dyne cm −2) = mgA−1 |
where, m is the mass (g) required to detach the membrane, g is acceleration due to gravity taken as 980 cm sec−2. and A is the area of tissue exposed which is equal to π r2 (r is the radius of the exposed mucosal membrane).
2.16. Statistical analysis
All the data were expressed as the mean ± SD. Results were statistically analyzed by Student's t test for significant differences between the group mean using GraphPad software.27 The significant difference between the experimental and the control group was set at different levels as p <0.05, p <0.01 and p <0.001.
3. Results and discussion
3.1. Effect of variation of surfactant concentration on the different properties of chitosan nanoparticles
The results showing the effects of variation of surfactant concentration on different properties of chitosan nanoparticles are shown in Table 2. In the case of MMT containing chitosan nanoparticles, yield (%) remained almost unchanged irrespective of surfactant concentration. Encapsulation efficiency (%) was also found to remain the same. It was also observed that neither the surfactant nor the MMT alone produced any significant effect on the drug loading efficiency, except the yield. Surfactant alone provided a somewhat low yield which might be due to the loss during isolation. At a fixed level of MMT concentration, drug loading efficiency showed an increasing trend while particle size exhibited a decreasing trend as the concentration of the surfactant increased. The presence of surfactant could increase the interlayer distance of silicate layers of MMT. The higher the concentration of surfactant, the higher the interlayer distance to accommodate the polymer as well as the drug. This could have resulted in an increase in drug loading efficiency. As the surfactant was soluble in water, it could decrease the solubility of ionically crosslinked chitosan in water thus favoring the solid particle formation and consequently decreasing the viscosity of the dispersion phase. The lower dispersion phase viscosity facilitated the formation of smaller particles. The dispersing effect of the surfactant also favored the formation of smaller particles as the concentration of surfactant increased. Besides this, the physical interaction between the chitosan macromolecules may have been destructed due to shear resulting in the formation of a dispersing phase of lower viscosity28 which favors the formation of smaller particles. Zeta potential values of the nanoparticles showed that the zeta potential increased with the decrease in particle size. The zeta potential of nanoparticles which did not contain tween 80 and isoniazid was found to be less than that of the formulations containing both tween 80 and isoniazid. The zeta potential was found to be positive for all the formulations. Nanoparticles prepared without surfactant had a lower zeta potential indicating that they would aggregate in acidic or basic medium. On the other hand, particles prepared with surfactant exhibited a higher zeta potential probably because the surfactant prevented the counter-ions from binding within chitosan. Such particles would be expected to be stable.
Sample code |
Yield of nanoparticle (%) |
Encapsulation efficiency (%) |
Drug loading efficiency (%) |
Average diameter/nm |
Zeta potential/mV |
Zeta potential of the same formulations without drug/mV |
Zeta potential of nanoparticles after 3 h of drug release/mV |
Each value represents average of five readings, standard deviation in parenthesis.
|
NPI |
92.81 (± 0.01) |
73.10 (± 0.02) |
25.39 (± 0.08) |
451.8 (± 15) |
21.22 (± 0.03) |
17.05 (± 0.01) |
18.53 (± 0.04) |
NPII |
91.27 (± 0.05) |
72.98 (± 0.04) |
25.61 (± 0.04) |
— |
— |
— |
— |
NPIII |
91.38 (± 0.01) |
73.32 (± 0.04) |
28.18 (± 0.01) |
322.4 (± 12) |
28.24 (± 0.1) |
21.67 (± 0.07) |
23.89 (± 0.01) |
NPIV |
91.19 (± 0.01) |
73.48 (± 0.01) |
29.27 (± 0.01) |
305.7 (± 13) |
30.01 (± 0.06) |
23.12 (± 0.01) |
26.33 (± 0.04) |
NPV |
92.52 (± 0.05) |
73.41 (± 0.03) |
31.63 (± 0.02) |
282.2 (± 15) |
36.93 (± 0.01) |
30.41 (± 0.03) |
33.12 (± 0.01) |
NPVI |
86.16 (± 0.08) |
73.60 (± 0.02) |
25.58 (± 0.01) |
— |
— |
— |
— |
Isoniazid has normally three pKa values: 1.8 based on the hydrazine nitrogen, 3.5 based on the pyridine nitrogen and 10.8 based on the acidic group.29 At pH 1.2, the pKa of isoniazid is found to be around 2 due to protonation of the hydrazine nitrogen whereas at a basic pH of 7.4 it is found to be around 12. The increase in the zeta potential with the incorporation of the drug suggests that at least a part of the drug–polymer association was surface-adsorbed. Thus, a part of the drug was embedded within the chitosan matrix and the rest was adsorbed on the surface of the nanoparticles.
3.2. Fourier transmission infra-red spectroscopy (FTIR) study
In the spectrum of pure chitosan (Fig. 1a), the broad absorption band that appeared in the range 3200–3450 cm−1 was due to the hydrogen-bonded OH stretching and NH2 asymmetric stretching. The characteristic peaks of amide I and amide II appeared at 1646 cm−1 (C
O stretching) and 1569 cm−1 (N–H in plane deformation coupled with C≡N stretching), respectively.30 The peaks exhibited in the spectrum for MMT (Fig. 1b) at 3435, 1639 and 1051–544 cm−1 were for –OH stretching, –OH bending and oxide bands of metals like Si, Al, Mg, etc.Fig. 1c represents the spectrum of isoniazid. The absorption peaks that appeared at 1664 and 1551 cm−1 were due to the amide I (C
O stretching) and amide II (N–H bending of secondary amide group), respectively. Besides this, multiple peaks appeared in the range 1410–669 cm−1.20
All the characteristic peaks of chitosan, MMT and isoniazid appeared and their intensities decreased in the spectrum of isoniazid loaded chitosan-MMT nanoparticles (Fig. 1d). Moreover, the intensity of the peak that appeared in the range 3450–3200 cm−1 (Fig. 1a) decreased and shifted to lower wave number. This indicated an interaction between MMT and chitosan. The decrease in hydroxyl peak intensity was reported by Maji et al.31 while studying the properties of wood polymer nanocomposites. All these indicated a better dispersion of MMT and isoniazid in the chitosan-MMT nanoparticles.
Isoniazid (Fig. 2, curve 2a) shows multiple peaks at 2θ = 12 to 50° due to its crystalline nature. A similar type of diffractogram was reported by Fukuoka et al.32 Chitosan (curve 2b) shows its characteristic diffraction peak at 2θ = 20.3° which corresponds to the (100) plane of the orthorhombic crystal.33 MMT exhibits the two characteristic peaks at 2θ = 9.01° and 26.7° which are assigned to the (001) and (002) planes (curve 2c).34 The characteristic peaks for MMT and isoniazid were found to disappear in the diffractogram of MMT/chitosan nanoparticles (curve 2d). It could be said that either the full expansion of the MMT gallery occurred, which was not possible to detect by XRD, or the MMT layers became delaminated and no crystal diffraction peak appeared.35 These findings suggested the occurrence of a molecular level dispersion of isoniazid in isoniazid loaded chitosan-MMT nanoparticles. The molecular level dispersion of isoniazid in the chitosan-hydroxyethyl cellulose blended microsphere was reported by Aminabhavi et al.36
SEM micrographs of chitosan-MMT nanoparticles and isoniazid loaded nanoparticles are shown in Fig. 3a and 3b, respectively. The surface of the nanoparticles without isoniazid appeared less smooth and agglomerated as compared to isoniazid loaded nanoparticles. Isoniazid loaded nanoparticles had a spherical shape and a smooth surface. Selvaraj et al.11 reported that acyclovir loaded chitosan nanoparticles had a solid dense structure with a smooth spherical shape.
TEM micrographs of isoniazid loaded chitosan nanoparticles devoid of MMT and with MMT are shown in Fig. 3c and 3d, respectively. Fig. 3d shows the presence of platelets of MMT tactoids in which the dark lines are the intersection of MMT layers. The bright areas are polymer matrix and isoniazid. A similar observation was reported by Wang et al.37 while studying the biopolymer/MMT structure by TEM. The results indicated that MMT was incorporated and dispersed in the chitosan matrix.
3.6. Swelling study
The effect of pH on the percentage swelling of isoniazid loaded nanoparticles at two different pH namely, 1.2 and 7.4, are shown in Fig. 4. It was observed that the swelling of isoniazid loaded chitosan-MMT nanoparticles was greater in gastric pH (1.2) than in intestinal pH (7.4). In an acidic medium, the amine groups of chitosan molecules were ionized to ammonium ions. These cationic charges acted as repulsive forces between the polymer molecules38 and hence increased the swelling. In alkaline pH, the inherent hydrophobicity of chitosan nanoparticles prevented them from fast swelling.39 Furthermore, the percentage swelling was found to increase with the increase in time and decrease in particle size of the nanoparticles. The lower the particle size, the higher was the surface area. Higher surface area facilitated better contact of the nanoparticles with the solvent and thus improved swelling.
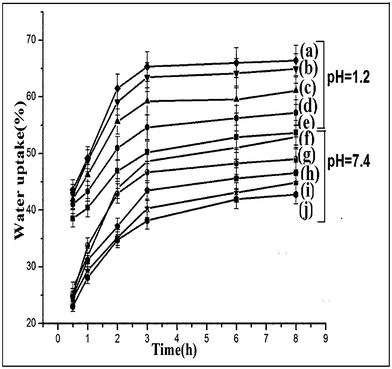 |
| Fig. 4 Percentage swelling degree at pH 1.2 of (a) NPV, (b) NPIV, (c) NPIII, (d)NPII and (e) NPI and at pH 7.4 of (f) NPV, (g) NPIV, (h) NPIII, (i) NPII and (j) NPI. | |
3.7.
In vitro Release Studies
The drug release profile of the nanoparticles at two different pH, namely 1.2 and 7.4, are shown in Fig. 5. The cumulative release (%) of isoniazid from chitosan-MMT nanoparticles was found to be pH-dependent. The cumulative release (%) of isoniazid decreased with the increase in the pH of the medium. The two main factors governing the release profile of isoniazid from nanoparticles were the swelling nature of the polymer and the solubility of the drug in the medium. The difference in release profile was due to the difference in the solubilities of chitosan in gastric and intestinal pH.40 Chitosan was more soluble in gastric pH compared to intestinal pH. The solubility of isoniazid increased at acidic pH due to its basic nature as reported in literature.41 A lower pH of the medium favoured both the swelling of the polymer and the solubility of the drug. Cumulative release (%) of isoniazid was also found to increase with the increase in time and decrease in particle size of the nanoparticles. The explanation for this observation is similar to that stated earlier.
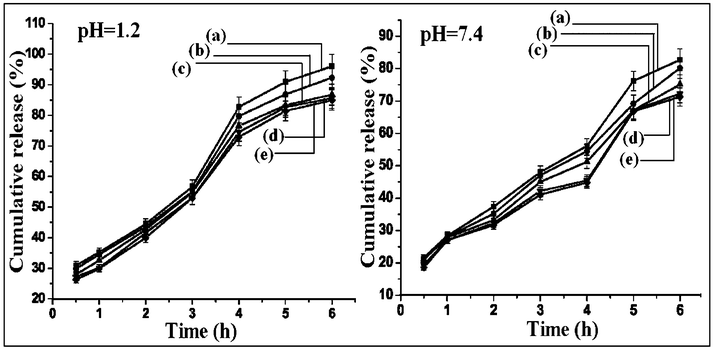 |
| Fig. 5 Cumulative percentage drug release at pH 1.2 of (a) NPV, (b) NPIV, (c) NPIII, (d)NPII and (e) NPI and at pH 7.4 of (a) NPV, (b) NPIV, (c) NPIII, (d) NPII and (e) NPI. | |
Moreover, a burst release of about 50% drug was observed in the first 3 h of the test. The lower diffusion path due to the lower particle size along with other already stated factors played an important role in controlling the release rate. The adsorbed drug on the surface of nanoparticles might be the main factor responsible for the initial burst release. This was supported by the zeta potential value.8
3.8. Cytotoxicity test
The results of the MTT-assay are shown in Fig. 6. It was observed that the cell viability varied between 85–90% within the studied surfactant (tween 80) concentration. This indicated that the surfactant was not highly cytotoxic to the cells (Fig. 6a). Tween 80 did not exhibit any significant cytotoxicity when evaluated in CaCo-2 cells.42
In our case, a slight decrease in cell viability was observed as the concentration of surfactant increased. The use of nonionic surfactants as penetration enhancers has been reported due to their non irritating nature and low toxicity.43 Addition of surfactant resulted in an increase in the dispersion of nanoparticles and enhanced their interaction with the cells leading to a decrease in cell viability. MMT showed very low cytotoxicity as is evident from Fig. 6b. A similar result was also reported by Wang et al.36Fig. 6c and 6d show the cell viability (%) of isoniazid alone and clay free isoniazid loaded nanoparticles. In both these cases, the cell viability decreased with the increase in the concentration of isoniazid. The cytotoxicity was found to be less in the case of isoniazid embedded polymeric nanoparticles compared to those of isoniazid alone. The polymer slowed down the release of isoniazid and hence decreased the interaction of the drug with the cell. It was observed further (Fig. 6d and 6e) that the cell viability of nanoparticles containing clay was greater compared to clay-free nanoparticles. This might be due to the fact that the silicate layers hindered the release of drug in the cell because of its tortuous path. Fig. 6(e–h) shows the results of cell viability of nanoparticles prepared under different concentrations of surfactants (0–0.025 ml). Nanoparticles prepared using surfactant exhibited higher cytotoxicity than those prepared without surfactant. The cytotoxicity of nanoparticles increased up to the use of 3% (v/w) surfactant, beyond that the value remained almost unchanged. The nanoparticles prepared without tween 80 had large particle size compared to nanoparticles prepared with tween 80. The higher the surfactant concentration, the lower the particle size observed and the higher was the surface area. Due to the higher surface area, the contact of the nanoparticles with the cell increased and hence the cytotoxicity also increased. The increase of cytotoxicity value with time was due to more contact between the drug and the cell wall. The cell viability of the nanoparticles synthesized with and without surfactant was within the range of 70–90%.
3.9.
In vitro wash-off test for evaluation of mucoadhesive properties
Results of the in vitro wash off test of chitosan-MMT nanoparticles containing isoniazid are given in Table 3. The results represented in table are the average readings of five samples. The test was carried out in gastric pH (pH = 1.2) and intestinal pH (pH = 7.4). The mucoadhesion was found to be higher in gastric pH than in intestinal pH. The mucoadhesive properties were also enhanced with the decrease in the particle size of the nanoparticles.
Table 3 Results of in vitro wash–off test to assess mucoadhesive properties of nanoparticles prepareda
pH |
Sampl code |
No. of particles taken |
Reading after every 30 min |
1 |
2 |
3 |
4 |
5 |
6 |
7 |
8 |
9 |
10 |
Each value represents the average of five readings, standard deviation in parenthesis.
|
1.2 |
NPI |
50 |
35.4 (± 0.5) |
32.4 (± 0.5) |
25.4 (± 1.0) |
20.4 (± 0.5) |
12.2 (± 0.7) |
10.8 (± 0.7) |
8.6 (± 0.5) |
5.0 (± 0.6) |
0 |
0 |
NPIII |
50 |
44 (± 0.6) |
42 (± 0.9) |
40 (± 1.4) |
35 (± 0.6) |
30 (± 0.6) |
20.4 (± 0.5) |
15 (± 0.6) |
12.4 (± 0.5) |
10.2 (± 0.7) |
4.8 (± 0.4) |
NPIV |
50 |
52.8 (± 1.3) |
50 (± 0.6) |
46.8 (± 1.2) |
45 (± 0.6) |
42.8 (± 0.7) |
37.8 (± 1.0) |
32 (± 0.6) |
23.2 (± 0.4) |
21.6 (± 0.5) |
17 (± 1.3) |
NPVI |
65 |
60 (± 0.9) |
58 (± 0.6) |
57 (± 0.9) |
55.4 (± 0.8) |
51.4 (± 0.5) |
47.2 (± 0.4) |
35.2 (± 0.4) |
30 (± 0.6) |
22.4 (± 0.8) |
14 (± 1.3) |
7.4 |
NPI |
50 |
25 (± 1.4) |
20.2 (± 0.7) |
10.2 (± 7.4) |
8 (± 0.6) |
4 (± 0.6) |
2.2 (± 0.4) |
0 |
0 |
0 |
0 |
NPIII |
50 |
36 (± 0.9) |
33 (± 0.9) |
28.4 (± 0.5) |
22.4 (± 0.5) |
16 (± 0.6) |
14.8 (± 0.4) |
12 (± 0.6) |
7.6 (± 1.0) |
2.8 (± 0.4) |
0 |
NPIV |
50 |
44 (± 0.9) |
35 (± 0.6) |
32.2 (± 0.7) |
26 (± 1.1) |
21 (± 0.6) |
18.4 (± 1.0) |
11.2 (± 0.4) |
8.8 (± 0.4) |
5.4 (± 0.5) |
0 |
NPVI |
50 |
45.2 (± 0.7) |
43.8 (± 0.7) |
42 (± 0.6) |
40.8 (± 0.4) |
37.2 (± 1.0) |
35.4 (± 0.5) |
31.8 (± 0.4) |
28 (± 0.6) |
22.8 (± 0.4) |
16.6 (± 0.5) |
At acidic pH, the free amino groups of chitosan nanoparticles might get protonated and became positively charged. These could strongly bind to the negatively charged mucus layer. The higher surface area of the nanoparticles was responsible for the observed enhanced mucoadhesive property exhibited by chitosan nanoparticles having a lower particle size. Sabitha et al.44 reported that chitosan-alginate microcapsules showed lower mucoadhesive properties in intestinal pH compared to gastric pH.
3.10.
Ex vivo mucoadhesive test
Table 4 shows the results of an ex vivo mucoadhesion test. The results are the mean value of five readings. It was observed that the detachment force increased with the decrease in particle size of the nanoparticles. The lower the particle size, the higher the surface area and hence the nanoparticles could strongly adhere to the mucosal surface. Rekha et al. reported that mucoadhesive properties of the succinyl chitosan particles were enhanced due to better penetration of smaller sized particles into the mucus layers.45
Table 4 Weight required to detach the membrane at different time intervalsa
Sample code |
Mass required to detach after 5 min/g |
Detachment force/dyne cm−2 |
Mass required to detach after 10 min/g |
Detachment force/dyne cm−2 |
Mass required to detach after 15 min/g |
Detachment force/dyne cm−2 |
Mass required to detach after 20 min/g |
Detachment force/dyne cm−2 |
Each value represents average of five readings, standard deviation in parenthesis.
|
NPI |
9.95 (± 0.05) |
3103.8 (± 12.7) |
16.5 (± 0.03) |
5147.0 (± 2.6) |
21.1 (± 0.03) |
6582 (± 7.6) |
21.8 (± 0.01) |
6800.4 (± 2.5) |
NPIII |
16.5 (± 0.01) |
5147.0 (± 2.6) |
19.6 (± 0.05) |
6124.1 (± 19.0) |
21.3 (± 0.01) |
6644.4 (± 2.5) |
22.0 (± 0.02) |
6862.8 (± 5.1) |
NPIV |
17.85 (± 0.05) |
5568.2 (± 12.7) |
20.2 (± 0.01) |
6301.3 (± 2.6) |
21.6 (± 0.04) |
6737.9 (± 10.2) |
22.2 (± 0.02) |
6925.1 (± 5.1) |
NPVI |
18.25 (± 0.02) |
5692.9 (± 5.1) |
22.1 (± 0.01) |
6893.9 (± 2.6) |
22.5 (± 0.03) |
7018.7 (± 7.6) |
23.5 (± 0.01) |
7330.7 (± 2.5) |
Conclusion
This work demonstrated the successful preparation of isoniazid loaded chitosan-MMT nanoparticles by ionic gelation followed by chemical crosslinking. Both the swelling and release of isoniazid from the nanoparticles were found to increase with an decrease in the pH of the medium and the size of the nanoparticles. The FTIR study indicated the interaction of the clay with the chitosan polymer. The exfoliation of MMT layers was examined by XRD and TEM. XRD results also showed the molecular level dispersion of isoniazid in the chitosan-MMT nanoparticles. SEM showed that the surface of the chitosan-MMT nanoparticles was less smooth compared to those of isoniazid loaded nanoparticles. Nanoparticles containing clay were less cytotoxic than clay-free nanoparticles. The lower the size of the nanoparticles, the higher the cytotoxicity. The mucoadhesivity of the nanoparticles was better in gastric pH and increased with the decrease in particle size.
Acknowledgements
University grant commission (UGC) is acknowledged for financial support.
References
- R. Riva, H. Ragelle, A. Rieux, N. Duhem, C. Jéroˆme and V. Préat, Adv. Polym. Sci., 2011, 244, 19–44 CrossRef.
- M. Z. Hussein, M. A. Yarmo, M. Z. H. A. Rahman, Z. Zainal and A. A. S. Liang, Malay. J. Analyt. Sci., 2001, 7, 35–40 Search PubMed.
- W. Tiyaboonchai, Naresuan University J., 2003, 11, 51–66 Search PubMed.
- M. Prabharan, J. Biomater. Appl., 2008, 23, 5–32 CrossRef.
- Y. Shirosakia, K. Tsurua, S. Hayakawaa, A. Osakaa, M. A. Lopes, J. D. Santos and M. H. Fernandes, Biomaterials, 2005, 26, 485–493 CrossRef.
- J. P. Venter, A. F. Kotźe, R. A. Velty and M. Rinaudo, Int. J. Pharm., 2006, 313, 36–42 CrossRef CAS.
- E. B. Denkbas and R. M. Ottenbrite, J. Bioact. Compat. Polym., 2006, 21, 351–368 CrossRef CAS.
- P. S. Pourshahab, K. Gilani, E. Moazeni, H. Eslahi, M. R. Fazeli and H. Jamalifar, J. Microencapsulation, 2011, 28, 605–613 CrossRef CAS.
- N. Ahmed, M. Michelin-Jamois, H. Fessi and A. Elaissari, Soft Matter, 2012, 8, 2554–2564 RSC.
- G. V. Joshi, B. D. Kevadiya, H. A. Patel, H. C. Bajaj and R. V. Jasra, Int. J. Pharm., 2009, 374, 53–57 CrossRef CAS.
- S. Selvaraj, N. Saravanakumar, J. Karthikeyan, D. D. Evangeline, D. Lathamary and N. N. Rajendran, Der Pharmacia Lettre, 2010, 2, 420–431 CAS.
- A. Grenha, C. I. Grainger, L. A. Dailey, B. Seijo, G. P. Martin, C. R. Lópeza and B. Forbes, Eur. J. Pharm. Sci., 2007, 31, 73–84 CrossRef CAS.
- A Mahapatro and D. K. Singh, J. Nanobiotechnol., 2011, 9, 1–11 CrossRef.
- Y. Wu, W. Yang, C. Wang, J. Hu and S. Fu, Int. J. Pharm., 2005, 295, 235–245 CrossRef CAS.
- J. P. Zheng, L. Luan, H. Y. Wang, L. F. Xi and K. D. Yao, Appl. Clay Sci., 2007, 36, 297–301 CrossRef CAS.
- F. H. Lin, Y. H. Lee, C. H. Jian, J. M. Wong, M. J. Shieh and C. Y. Wang, Biomaterials, 2002, 23, 1981–1987 CrossRef CAS.
- Y. Dong and S. S. Feng, Biomaterials, 2005, 26, 6068–6076 CrossRef CAS.
- K. C. Gupta and M. N. V. R. Kumar, Biomaterials, 2000, 21, 1115–1119 CrossRef CAS.
- A. Tripathi, R. Gupta and S. A. Saraf, Int. J. Pharm. Tech. Res., 2010, 2, 2116–2123 CAS.
- N. Devi and T. K. Maji, Drug Dev. Ind. Pharm., 2010, 36, 56–63 CrossRef CAS.
- N. Devi and T.K. Maji, AAPS PharmSciTech, 2009, 10, 1412–1419 CrossRef CAS.
- R. Cassano, S. Trombino, T. Ferrarelli, M. V. Mauro, C. Giraldi, M. Manconi, A. M. Fadda and N. Picci, Society for Biomat., 201110.1002/jbm.a.33302 1-7. Search PubMed.
- A. Hussain, V. Saikia and A. M. Ramteke, Free Radicals and Antioxidants, 2012, 2, 8–11 CrossRef.
- F. Denizot and R. Lang, J. Immunol. Methods, 1986, 89, 271–277 CrossRef CAS.
- C. M. Lehr, J. A. Bowstra, J. J. Tukker and H. E. Junginger, J. Controlled Release, 1990, 13, 51–62 CrossRef CAS.
- B. R. Gandhi and J. R. Robinson, Ind. J. Pharm. Sci., 1988, 50, 145–152 Search PubMed.
-
G. J. Bourke, L. E. Daly and J. McGilvary, 3rd ed.;Blackwell Scientific Publication,Oxford, 2009.
- C. G. da Trindade Neto, A. LP. Fernandes, A. IB. Santos, W. A. Morais, M. VM. Navarro, T. NC. Dantas, M. R. Pereira and J. LC. Fonseca, Polym. Int., 2005, 54, 659–666 CrossRef CAS.
- N. F. Atta, A. Galal and R. A. Ahmed, Int. J. Electrochem. Sci., 2011, 6, 5097–5113 CAS.
- I. F. Amaral, P. L. Granja and M. A. Barbosa, J. Biomater. Sci., Polym. Ed., 2005, 16, 1575–1593 CrossRef CAS.
- B. K. Deka and T. K. Maji, Compos. Sci. Technol., 2010, 70, 1755–1761 CrossRef CAS.
- E. Fukuoka, M. Makita and S. Yamamura, Chem. Pharm. Bull., 1993, 41, 2166–2171 CrossRef CAS.
- Z. Zong, Y. Kimura, M. Takahashi and H. Yamane, Polymer, 2000, 41, 899–906 CrossRef CAS.
- A. Bahari, M. Asgharzadeh and M. Najafikhah, Afr. J. Pure Appl. Chem., 2011, 5, 429–435 CAS.
- W. H. Lu, G. J. Zho and Z. H. Xue, Forestry Studies in China, 2006, 8, 35–40 CrossRef CAS.
- S. C. Angadi, L. S. Manjeshwar and T. M. Aminabhavi, Int. J. Biol. Macromol., 2010, 47, 171–179 CrossRef CAS.
- X. Wang, Y. Du and J. Luo, Nanotecnology, 2008, 19, 1–7 CAS.
- N. V. Gupta and H. G. Shivakumar, Trop. J. Pharm. Res., 2010, 9, 257–264 CrossRef CAS.
- K. C. Gupta and M. N. Ravi Kumar, J. Mater. Sci.: Mater. Med., 2001, 12, 753–759 CrossRef CAS.
- U. Guliyeva, F. Uner, S. Özsoy and R. Haziroğlu, Eur. J. Pharm. Biopharm., 2006, 62, 17–25 CrossRef CAS.
- C. Bceker, J. B. Dressman, G. L. Amidam, H. E. Junginger, S. Kopp, K. K. Midha, V. P. Shah, S. Stavchansky and D. M. Barends, J. Pharm. Sci., 2007, 96, 522–531 CrossRef.
- S. M. O'Sullivan, J. A. Woods and N. M. O'Brien, Br. J. Nutr., 2004, 91, 757–764 CrossRef CAS.
- N. A. Monteiro-Riviere, A. O. Inman, Y. Y. Wang and R. J. Nemanich, Nanomed.: Nanotechnol., Biol. Med., 2005, 1, 293–299 CrossRef CAS.
- P. Sabitha, J. V. Ratna and K. R. Reddy, International Journal of Chemical Technology, 2010, 2, 88–98 CrossRef CAS.
- M. R. Rekha and C. P. Sharma, Trends Biomater. Artif. Organs, 2008, 21, 107–115 Search PubMed.
|
This journal is © The Royal Society of Chemistry 2012 |
Click here to see how this site uses Cookies. View our privacy policy here.