DOI:
10.1039/C2RA20436C
(Paper)
RSC Adv., 2012,
2, 6011-6017
Photo-induced electron transfer study of D-π-A sensitizers with different type of anchoring groups for dye-sensitized solar cells†
Received
9th March 2012
, Accepted 21st April 2012
First published on 25th April 2012
Abstract
A new D-π-A organic dye HY102 with a lateral anchoring group and two reference dyes HY102-1 (using cyanoacrylic acid as an electron acceptor and the anchoring group) and HY102-2 (containing both cyanoacrylic acid and lateral carboxylic acid) have been synthesized. The optical and electrochemical test results from the three different styles of photosensitizers show that the excited electrons of the novel dye HY102 with lateral carboxylic acid group most probably are injected into the CB of TiO2 through the electron acceptor moiety close to the TiO2 surface by spatial transfer, not through the lateral anchoring group of the carboxylic acid. Research into the photo-induced electron transfer of the novel sensitizers with lateral anchoring system is reasonable and crucial for further improving efficiencies by modifying the molecular structures.
Introduction
With increasing concerns about the energy crisis and accompanying environmental issues, the development of clean and sustainable energy technologies has been a subject of intense research to scientists. Dye-sensitized Solar Cells (DSCs) featuring low cost and easy fabrication when compared to the ubiquitous silicon solar cells have exhibited the highest efficiency up to 12% under laboratory conditions.1 D-π-A constructed organic dyes are known for their excellent high extinction molar coefficients, easy design and optimization of molecular structures, and simple preparation, making them attractive for DSCs.2 However, most of D-π-A organic dyes have a cyanoacrylic acid as the anchoring group. This has limited the fine-tuning of HOMO-LUMO levels due to the difficulty in changing the structure of the anchoring group and the electron acceptor part. With above consideration, previously, a new strategy to design organic dyes for DSCs has been developed, in which the anchoring group is separated from the electron acceptor groups of the dyes.3 The excited electrons in the new style of dyes are expected to be injected into the conduction band (CB) of TiO2 through the electron acceptor moiety rather than the anchoring group. However, the efficiency of this kind of dye is still lower than that of the state of the art organic dyes. In order to further investigate the effect of the lateral anchoring group on the performance of DSCs, a photo-induced electron transfer study of D-π-A sensitizers with a lateral anchoring group and a normal cyanoacrylic acid anchoring group is studied in this paper.
The traditional cyanoacrylic acid definitely plays a crucial role in TiO2 sensitization and electron injection and many studies have been performed to investigate the electron transfer process between the electron acceptor unit and the semiconductor.4,5 But, the electron injection process of the novel sensitizers with lateral anchoring groups is still under discussion. Therefore, to obtain a clearer understanding, we have studied the optical and electrochemical properties of lateral dyes compared with cyanoacrylic acid dyes. A new D-π-A organic dye HY102 with the lateral anchoring group and two reference of dyes HY102-1 using cyanoacrylic acid as electron acceptor and the anchoring group and HY102-2 containing both cyanoacrylic acid and lateral carboxylic acid were synthesized (Fig. 1). The results show that HY102 displays an obvious change in optical and redox properties due to the introduction of the stronger electron withdrawing unit, which plays a beneficial role on the light-harvesting properties of the dyes compared to two other reference dyes. It is concluded therefore that D-π-A organic near-infrared dyes can be achieved by introducing a lateral anchoring group into the dye framework. It is noteworthy that, according to our research, the novel lateral dye HY102 presents a poor photovoltaic performance, which is attributed to poor injection of excited electrons into the CB of TiO2 through the electron acceptor moiety being spatially close to the TiO2 surface, and not from the anchoring group more commonly observed in sensitizers with cyanoacrylic acid as an end-group, such as HY102-1 and HY102-2.
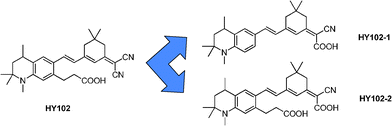 |
| Fig. 1 Molecular structures of HY102, HY102-1 and HY102-2. | |
Results and discussion
Synthesis
Tetrahydroquinoline derivatives 1–5 and D1 (Scheme 1) were synthesized in our previous publication.3Scheme 2 shows the synthetic routes of compounds 6–8 and D2 according to the already reported procedures.6 Intermediates 9 and A2 were prepared following the reported procedure.7 Finally, the Knoevenagel condensation reactions were performed to get compounds 9 and A2 through the aldehydes D1 and D2 compounds respectively then the hydrolysis reaction gave the target dyes HY102, HY102-1 and HY102-2(Scheme 3). The detailed synthetic procedures are described in the Supporting Information†.
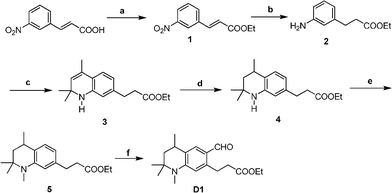 |
| Scheme 1 Synthetic routes of mediates 1-D1. Reagents and conditions: (a) EtOH, concentrated H2SO4, reflux overnight, 95%; (b) EtOH, Pd/C (5%), 4 h, 89%; (c) acetone, p-TsOH, cyclohexane, 80–90 °C, 8–10 h, 22%; (d) Raney-Ni, H2, 1 MPa, 130 °C, 99%; (e) (CH3)2SO4, benzene, reflux overnight, 68%; (f) DMF/POCl3, 55 °C, 6 h, 76%. | |
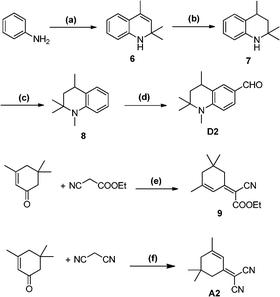 |
| Scheme 2 Synthetic routes of compounds 6-D2, 9 and A2. Reagents and conditions: (a) acetone, p-TsOH, cyclohexane, 80–90 °C, 8–10 h; (b) Raney-Ni, H2, 1 MPa, 130 °C; (c) (CH3)2SO4, benzene, reflux overnight; (d) DMF/POCl3, 55 °C, 6 h, 76%. (e)CH3COONH4, CH3COOH; (f)CH3CN, piperidine. | |
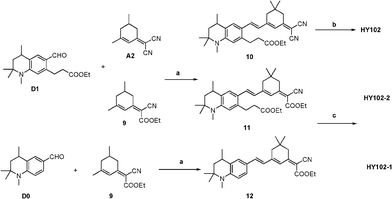 |
| Scheme 3 Synthetic routes of dyes HY102, HY102-1 and HY102-2. Reagents and conditions: (a) CH3CN, piperidine, reflux, over night; (b) CH3CH2OH, LiOH/H2O, room temperature; (c) CH3CH2OH, LiOH/H2O, 50 °C, 1 h. | |
Photophysical properties
Absorption spectra of the dyes HY102, HY102-1 and HY102-2 in CH2Cl2 solutions are shown in Fig. 2. The characteristic data are collected in Table 1. Three compounds HY102, HY102-1 and HY102-2 all exhibit a broad absorption band in the visible region, appearing at 533 nm, 514 nm and 485 nm, respectively. Compared with HY102-1, the maximum absorption of HY102 was red-shifted by 19 nm because of the introduction of the stronger electron withdrawing group (2-methylenemalononitrile) as the acceptor part. It can be concluded that the novel D-π-A organic dyes with the lateral anchoring group are more prone to adjust the absorption spectra into the near infrared region than conventional D-π-A organic dyes by modifying the electron acceptor and anchoring part. Generally, this red shift is anticipated to increase the photocurrent of DSCs. However, the sensitizer HY102-2, which has an additional lateral part than HY102-1, has dramatically blue shifted the absorption spectrum and given a remarkably decreased molar extinction coefficient, only 2415 M−1 cm−1 at 485 nm. The poor light harvesting ability of HY102-2 may be caused by the decrease of coplanarity between the electron donor and the electron acceptor in the ground-state as well as in the excited-state due to the existence of two carboxyl acid groups.
Table 1 Absorption and electrochemical properties of HY102, HY102-1 and HY102-2
From the absorption spectra of HY102, HY102-1 and HY102-2 on the TiO2 surface, the maximum absorptions were all red-shifted compared to that in solution, 7 nm, 22 nm and 17 nm, respectively, as shown in Fig. 3. The red shifts of spectra for these dyes could be caused by well-known solvent effects. In addition, the tendency to J-aggregation of dye molecules onto the TiO2 surface may also contribute to this red shift.8 Obviously, the absorption region of HY102, HY102-1 and HY102-2 on TiO2 are broader than that those in solution, from 400 nm–700 nm, which is an advantageous spectral property for light harvesting of the solar spectrum. This result indicates that bathochromically shifted absorption spectra for all three dyes on the TiO2 surface were favorable for light harvesting and high photocurrent.
Electrochemical properties
The electrochemical properties of sensitizers HY102, HY102-1 and HY102-2 were investigated in CH2Cl2 containing 0.1 M TBAPF6 as the electrolyte at room temperature. The electrochemical data are summarized in Table 1. The oxidation potentials (Eox) corresponding to the HOMO levels of the dyes can be obtained by cyclic voltammetry measurements, the excited state oxidation potential (Eox*) corresponding to the LUMO levels of the dyes were calculated by Eox − E0-0, where E0-0 is the zero-zero energy of the dyes estimated from the onset point of absorption spectra on TiO2. In these dyes, the novel lateral sensitizer HY102 with a stronger electron withdrawing unit (2-methylenemalononitrile) instead of cyanoacrylic acid as the electron acceptor shows positive shifts at both HOMO and LUMO levels compared to the reference dyes. Furthermore, the relatively strong influence of the stronger electron acceptor on the LUMO level of HY102 makes the E0-0 value decrease, and consequently results in a red shift of the absorption spectrum. As shown in Table 1, the excited state oxidation potentials of all these dyes are much more negative than the conduction band edge of TiO2, which is located at ca. −0.5 V (vs NHE), and the ground state oxidation potentials of these dyes, are more positive than the iodine redox potential value (+0.4 V vs NHE). As a result, a sufficient thermodynamic driving force for the efficient electron injection to TiO2 film and the dye regeneration reaction from iodine redox couple can be provided.9
To gain the further insight into the molecular structure and electron distribution of these three dyes at the frontier and nearby molecular orbitals, we performed quantum-chemical calculations on the dyes HY102, HY102-1 and HY102-2 using DFT calculations with the Gaussian 03 program. The calculations were carried out with B3LYP under 6-31+G(d) basis set, given the negligible effect of salvation on the electronic structure. The molecular structure and electron distributions of the HOMO and LUMO levels of three dyes are shown in Fig. 4. We observed that HOMO-LUMO excitation induced by light irradiation could move the electron distribution from the whole molecule to the cyanoacrylic acid moieties for HY102-1. For the dyes HY102 and HY102-2 containing the lateral carboxylic acid, the electron distributions were located totally at the electron acceptor part, nothing at the lateral anchoring unit. The reason for this phenomenon may be due mainly to the flexible lateral carboxylic acid group incorporated into the framework by single bonds, which cannot participate in the π-conjugated system and attract electrons. As a result, according to theoretical calculations, providing a similar molecular orbital geometry when anchored to TiO2, the excited electron for the lateral dye HY102 on TiO2 should be injected into the conduction band of TiO2 mainly through the acceptor part, not the lateral carboxylic acid.
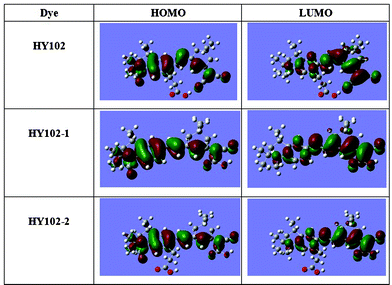 |
| Fig. 4 Calculated isodensity surface plots and molecular orbital energy diagram of the HOMO and LUMO for HY102, HY102-1 and HY102-2. | |
Photovoltaic performance
The dye-sensitized solar cells were constructed by using the three dyes as a sensitizer and nanocrystalline anatase TiO2 as the semiconductor material. The device performance parameters under AM 1.5 illumination are collected in Table 2. Fig. 5 and Fig. 7 show the photocurrent-voltage (J-V) curves and the incident photon-to-current conversion efficiencies (IPCE) of selected cells, respectively. The optimized evaluation conditions are determined to be 2 × 10−4 M of dye in CH2Cl2 solution, 2 × 10−4 M chenodeoxycholic acid (CDCA) as a coadsorbent, and the electrolyte is 0.6 M 1,2-dimethyl-3-n-propylimidazolium iodide (DMPII)/0.06 M LiI/0.04 M I2/0.4 M 4-tert-butylpyridine(TBP) in acetonitrile for HY102-1. Noticeably, the optimized test condition for HY102 and HY102-2 were different from HY102-1, which were determined to be 2 × 10−4 M of dye in CH2Cl2 solution and the electrolyte is 0.6 M DMPII/0.5 M LiI/0.02 M I2/0.1 M tetrabutyl ammonium iodide (TBAI) in acetonitrile
:
valeronitrile (AN
:
VN) = 85
:
15. Under the optimized test condition, the HY102-1-sensitized solar cell showed the best efficiency at 7.0% of all three dyes, with a Jsc of 13.96 mA cm−2, a Voc of 0.710 V, and an FF of 0.71. However, the lateral dye HY102-sensitized solar cell rendered an efficiency of 2.7% with Jsc of 7.55 mA cm−2, Voc of 0.54 V, and FF of 0.66. The photovoltaic performance is evidently decreased in the lateral dye HY102 compared with HY102-1 as a result of the poor electron injection efficiency from the excited dye to the conduction band of TiO2. Such a result was further investigated and discussed through the study of the mechanism of the electron injection to the conduction band of TiO2 for the DSCs based on the novel lateral sensitizer HY102 relative to HY102-1 and HY102-2.
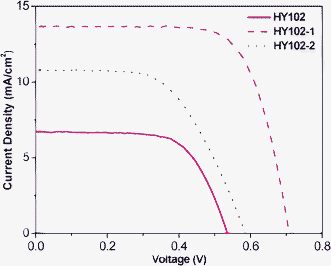 |
| Fig. 5
J-V curves of DSCs based on HY102, HY102-1 and HY102-2 dyes under optimized work conditions. | |
Table 2 Photovoltaic performance of DSCs based on HY102, HY102-1 and HY102-2 dyesa
Dyes |
Solvents |
J
sc (mA/cm2) |
V
oc (mV) |
FF
|
η (%) |
Irradiated light: AM 1.5 G (100 mW cm−2); Working area: 0.159 cm2; Electrolyte: 0.6 M DMPII, 0.06 M LiI, 0.04 M I2, 0.4 M TBP in acetonitrile for HY102-1 and 0.6 M DMPII/0.5 M LiI/0.02 M I2/0.1 M TBAI in AN:VN = 85:15 for HY102 and HY102-2.
|
HY102
|
CH2Cl2 |
7.55 |
540 |
0.66 |
2.7 |
HY102-1
|
CH2Cl2 |
13.96 |
710 |
0.71 |
7.0 |
HY102-2
|
CH2Cl2 |
10.80 |
581 |
0.57 |
3.6 |
Coadsorbent of CDCA is often utilized to suppress the dye aggregation by coadsorption with sensitizer on TiO2 surface , thus improving the photovoltaic performance.10 It has been reported11 that tetrahydroquinoline dyes easily aggregate on TiO2 film. Therefore, CDCA was added into the three dye solution and expected to give a better DSC performance. The sensitized results show that only the conventional dye HY102-1 could be adsorbed on the TiO2 surface perfectly with CDCA. But the lateral dyes HY102 and HY102-2 cannot be loaded onto TiO2 film in the presence of CDCA. This result implies that CDCA has much stronger competitive adsorption ability on the TiO2 surface than the lateral sensitizers. It is suggested that the sensitizers with lateral anchoring group adsorb to the TiO2 surface at a slower rate compared to common D-π-A organic dyes.
Under optimized test conditions for the lateral dyes HY102 and HY102-2, the electrolyte without TBP was used. To explain the TBP effect on DSC performance, the electrolytes with 0.08 M TBP and without TBP for DSCs based on HY102 were checked, and the performance of the solar cells are shown in Fig. 6. Upon the addition of TBP, although the Voc was improved, the Jsc of the solar cell decreased significantly from 6.36 to 3.23 mA cm−2 and thus η dropped from 2.1% to 1.0%. It has been reported12 that TBP gives a significant improvement of the open-circuit potential of the solar cell. Several mechanisms have been proposed to explain the observed effects. Among of them, Nazeeruddin et al. showed that the dark current, due to reduction of triiodide by electrons in TiO2, was suppressed by addition of TBP.13 This was attributed to a blocking effect in virtue of the adsorption of TBP on the active sites at the TiO2 surface. For our novel lateral dyes, the anchoring group and the electron injection group is separate. Firstly chemical bonding to TiO2 surface by the anchoring group of lateral carboxylic acid took place, and then electrons are injected through the electron acceptor unit of cyano-groups close to the TiO2 surface by proper spatial configurations. According to Nazeeruddin et al. the blocking effect due to adsorption of TBP on the TiO2 surface would hinder the electron acceptor from coming into close contact with the TiO2 surface, which would dramatically decrease the electron injection efficiency. As a result, the lowered Jsc for dyes HY102 and HY102-2 with lateral anchoring group is attributed to the addition of TBP in electrolyte.
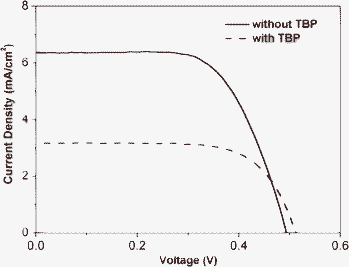 |
| Fig. 6
J-V curves of DSC based on HY102 dye with electrolyte without TBP (0.6 M DMPII/0.2 M LiI/0.02 M I2/0.1 M TBAI in AN:VN = 85:15) (solid line) and containing TBP(0.6 M DMPII/0.2 M LiI/0.02 M I2/0.1 M TBAI/0.08 M TBP in AN:VN = 85:15) (dash line). | |
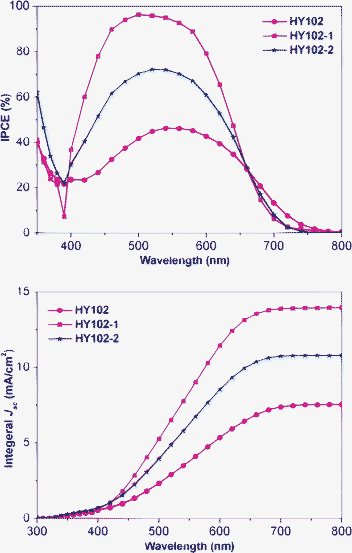 |
| Fig. 7 Spectral response of the photocurrent of DSC based on HY102, HY102-1 and HY102-2 dyes under optimized work conditions. | |
Fig. 7 shows the incident photon-to-current conversion efficiencies (IPCEs) data as a function of incident wavelength for DSCs based on three sensitizers. The IPCE spectra are consistent with the UV-vis absorption spectra on the TiO2 film. The integral current densities calculated from IPCE spectra for three dyes all match well with J-V test results (Fig. 7). IPCE action spectrum for DSC based on HY102 is broader than those of DSCs with HY102-1 and HY102-2, from 400 nm to 800 nm. The maximum IPCE value for the three DSCs increased in the order of HY102 < HY102-2 < HY102-1. As shown in Table 1, there are just minor differences between LUMO levels for the three dyes, but big differences in maximum IPCE values observed, which mainly arise from the different electron injection efficiencies caused by the spatial configurations of these dyes after loading onto the TiO2 surface, rather than the injection driving force. In other words, the electron injection efficiency follows the order of HY102 < HY102-2< HY102-1, consistent with the order of the maximum IPCE values. The poor electron injection efficiency of HY102 compared to HY102-1 is mainly due to the non-ideal electron injection mode. The spatial electron transfer mode in HY102 system is worse than electron injection through the chemical bond in HY102-1 system. For the dye HY102-2, although there are two carboxylic acid groups, only the lateral one acts as the anchoring group. The other located at the end-group role to inject electron to the TiO2 film is further from the TiO2 surface, and so makes little contribution to the electron injection efficiency compared to HY102.
The different binding modes between TiO2 surface and anchored dye are vital to achieve high quantum yields of electron injection into the semiconductor.14 The carboxylate functional groups of dyes play a crucial role in interfacial binding between the dye and semiconductor. The resonance FTIR technique provides a direct demonstration on binding mode of dyes on TiO2 surfaces. The FTIR spectra of our three dyes were measured as shown in Fig. 8. According to Deacon–Philips rule,15 the binding modes of the dye on TiO2 surface was determined by calculating the frequency separation, ΔV(Vas(COO−) - Vs(COO−)), between the asymmetric (Vas) and symmetric stretching (Vs) modes of the carboxylate unit. The ΔV values are in the order of unidentate > ionic form ≈ bidentate bridging > bidendate chelating. Yang et al has reported14 that bidentate bridging is suggested for a majority of binding modes between semiconductor surface and anchored dye in CH2Cl2 solution. When the dyes anchored to TiO2 surface in CH2Cl2, different ΔV values were obtained. The anchored three dyes HY102, HY102-1 and HY102-2 in CH2Cl2, showed different ΔV values of 231, 226, and 233 cm−1, respectively. One can conclude therefore that the binding modes of these dyes on the semiconductor surface should be the bidentate bridging configuration. The dyes HY102 and HY102-2 on TiO2 film have similar resonance FTIR absorption spectra. It can be deduced that the dye HY102-2 is supposed to anchor to TiO2 surface by lateral flexible carboxylic acid group like HY102. However, the peak of carbonyl group was not observed at 1700–1750 cm−1 for HY102-2. Considering the different ΔV value of HY102-2 compared with that of HY102-1, the bonding between HY102-2 and TiO2 film by the single lateral flexible carboxylic acid group is absolutely predominant. The other carboxylic acid group contained in the cyanoacrylic acid unit is most probably deprotonated in the vicinity of TiO2 surface, and injects electron to TiO2 film by spatial transfer.
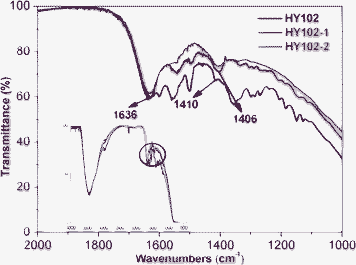 |
| Fig. 8 FTIR spectra of anchored HY102, HY102-1 and HY102-2 on TiO2via CH2Cl2. | |
To understand the lower Voc for the DSCs based on HY102 and HY102-2 compared to those of DSC with the dye HY102-1, we carried out Electrochemical Impedance Spectroscopy (EIS) analysis16 to study the interfacial charge transfer processes in DSCs. Fig. 9 shows the Nyquist plots for the DSCs with HY102, HY102-1 and HY102-2. The large semicircle represents the charge recombination arising from electrons in the TiO2 film recombination with I3− ion in the electrolyte. Under the same experimental condition the charge transfer resistance at the TiO2/dye/electrolyte interface increased from HY102 to HY102-2, and HY102-1 (Fig. 9). As previously mentioned, HY102-1 showed a larger Voc compared to HY102 and HY102-2 due to addition of TBP in the electrolyte. Since the TBP on the TiO2 surface acts as a blocking layer preventing the approach of I3− ions to the TiO2 surface, the recombination between the electrons in TiO2 and the holes in electrolyte could be more dramatically suppressed for HY102-1 than HY102 and HY102-2. Moreover, the anchoring group of the lateral carboxylic acid on the donor part for dyes HY102 and HY102-2 brings the donor unit close to the TiO2 surface, which is likely to induce electron recombination in the TiO2 film with the oxidized dye. As a result, the combination of poor charge transfer and possible electron recombination with oxidized dye leads to a lower Voc for DSCs sensitized by HY102 and HY102-2 compared to HY102-1.
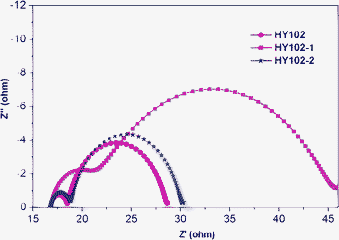 |
| Fig. 9 Electrochemical impedance spectra, scanned from 10−2 to 107 Hz at room temperature, for the devices based on HY102, HY102-1 and HY102-2, respectively: Nyquist plots; cells were measured at −0.7 V in the dark. The alternate current (AC) amplitude was set at 10 mV. | |
Possible photo-induced electron transfer of three different types of D-π-A sensitizers
Fig. 10 shows an ideal electron injection diagram for DSCs based on nanocrystalline TiO2 electrode, I−/I3− redox couple, and HY102, HY102-1 and HY102-2 as photosensitizers. HY102-1 (contains a cyanoacrylic acid that incorporates an anchoring group and acceptor part as one) adsorbed on the TiO2 surface and injected electron through carboxylic acid group with bidentate bridging mode. Unlike HY102-1, the lateral dye HY102 bonded to the TiO2 surface by bidentate bridging mode through the lateral flexible carboxylic acid group, but the electron was injected to TiO2 through the electron acceptor unit of cyano groups close to the TiO2 surface. HY102-2 which contains both a lateral flexible carboxylic acid group and a cyanoacrylic acid group shows similar anchoring and electron injection behavior as HY102, as discussed above.
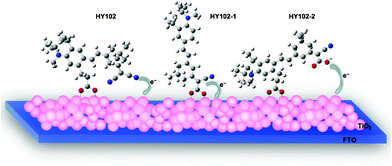 |
| Fig. 10 Schematic view of possible binding mode and electron injection for HY102, HY102-1 and HY102-2 on TiO2 surface. | |
Conclusions
In summary, we report one novel D-π-A organic dye HY102 with a lateral anchoring group and two reference dyes HY102-1 (containing cyanoacrylic acid group that combines electron acceptor and anchoring group together) and HY102-2 (containing both cyanoacrylic acid group and lateral carboxylic acid group). Optimized test results indicate that the HY102-1-sensitized solar cell shows the best efficiency of 7.0% among all three dyes, which is attributed to highly efficient electron injection by cyanoacrylic acid unit and less charge recombination on the TiO2 surface. The DSCs based on HY102 and HY102-2 exhibited decreased efficiencies of 3.6% and 2.7%, both lower Jsc and Voc compared with HY102-1. This is attributed mainly to the anchoring mode by the lateral anchoring group, which causes the electron donor moiety close to the TiO2 surface to enhance electron recombination, and makes the electron acceptor unit of cyanoacrylic acid move apart from the TiO2 surface decreasing the electron injection efficiency.
References
-
(a) M. Grätzel, Nature., 2001, 414, 338 CrossRef;
(b) M. K. Nazeeruddin, P. Pechy, T. Renouard, S. M. Zakeeruddin, R. Humphry-Baker, P. Comte, P. Liska, L. Cevey, E. Costa, V. Shklover, L. Spiccia, G. B. Deacon, C. A. Bignozzi and M. Grtäzel, J. Am. Chem. Soc., 2001, 123, 1613 CrossRef CAS;
(c) M. K. Nazeeruddin, F. De Angelis, S. Fantacci, A. Selloni, G. Viscardi, P. Liska, S. Ito, B. Takeru and M. Grätzel, J. Am. Chem. Soc., 2005, 127, 16835 CrossRef CAS;
(d) Y. Chiba, A. Islam, Y. Watanabe, R. Komiya, N. Koide and L. Han, Jpn. J. Appl. Phys., 2006, 45, 638 CrossRef;
(e) M. K. Nazeeruddin, T. Bessho, L. Cevey, S. Ito, C. Klein, F. De Angelis, S. Fantacci, P. Comte, P. Liska, H. Imai and M. Grätzel, J. Photochem. Photobiol. A., 2007, 185, 331 CrossRef CAS;
(f) F. Gao, Y. Wang, J. Zhang, D. Shi, M. Wang, R. Humphry-Baker, P. Wang, S. M. Zakeeruddin and M. Grätzel, Chem. Commun., 2008, 23, 2635 RSC;
(g) A. Hagfeldt, G. Boschloo, L. Sun, L. Kloo and H. Pettersson, Chem. Rev., 2010, 110, 6595–6663 CrossRef CAS.
- C. S. Karthikeyan, H. Wietasch and M. Thelakkat, Adv. Mater., 2007, 19, 1091–1095 CrossRef CAS.
- Y. Hao, X. Yang, J. Cong, H. Tian, A. Hagfeldt and L. Sun, Chem. Commun., 2009, 4031–4033 RSC.
- S. Yu, S. Ahmadi, M. Zuleta, H. Tian, K. Schulte, A. Pietzsch, F. Hennies, J. Weissenrieder, X. Yang and M. Göthelid, J. Chem. Phys., 2010, 133, 224704 CrossRef.
- M. Ziółek, I. Tacchini, M. Teresa Martínez, X. Yang, L. Sun and A. Douhal, Phys. Chem. Chem. Phys., 2011, 13, 4032–4044 RSC.
-
(a) R. Chen, X. Yang, H. Tian, X. Wang, A. Hagfeldt and L. Sun, Chem. Mater., 2007, 19, 4007 CrossRef CAS;
(b) R. Chen, X. Yang, H. Tian and L. Sun, J. Photochem. Photobiol. A, 2007, 189, 295 CrossRef CAS.
- K. H. Park, R. J. Twieg, R. Ravikiran, L. F. Rhodes, R. A. Shick, D. Yankelevich and A. Knoesen, Macromolecules., 2004, 37, 5163 CrossRef CAS.
-
(a) A. Hagfeldt and M. Grätzel, Chem. ReV., 1995, 95, 49 CrossRef CAS;
(b) K. Kalyanasundaram and M. Grätzel, Coord. Chem. Rev., 1998, 177, 347 CrossRef CAS.
- D. P. Hagberg, T. Edvinsson, T. Marinado, G. Boschloo, A. Hagfeldt and L. Sun, Chem. Commun., 2006, 2245–2247 RSC.
- J. H. Yum, S. J. Moon, R. Humphry-Baker, P. Walter, T. Geiger, F. Nüesch, M. Grätzel and M. K. Nazeeruddin, Nanotechnology., 2008, 19, 424005 CrossRef CAS.
- H. Tian, X. Yang, J. Cong, R. Chen, C. Teng, J. Liu, Y. Hao, L. Wang and L. Sun, Dyes Pigments., 2010, 84, 62–68 CrossRef CAS.
- G. Boschloo, L. Häggman and A. Hagfeldt, J. Phys. Chem. B., 2006, 110, 26 CrossRef.
- M. K. Nazeeruddin, A. Kay, I. Rodicio, R. Humphry-Baker, E. Müller, P. Liska, N. Vlachopoulos and M. Grätzel, J. Am. Chem. Soc., 1993, 115, 6382 CrossRef CAS.
- H. Tian, X. Yang, R. Chen, R. Zhang, A. Hagfeldt and L. Sun, J. Phys. Chem. C., 2008, 112, 11023–11033 CAS.
- G. B. Deacon and R. Phillips, J. Coord. Chem. Rev., 1980, 33, 227–250 CrossRef CAS.
-
(a) M. Adachi, M. Sakamoto, J. Jiu, Y. Ogata and S. Isoda, J. Chem. Phys. B., 2006, 110, 13872 CrossRef CAS;
(b) Q. Wang, J. Moser and M. Grätzel, J. Chem. Phys. B., 2005, 109, 14945 CrossRef CAS;
(c) Z. Wang, N. Koumura, Y. Cui, M. Takahashi, H. Sekiguchi, A. Mori, T. Kubo, A. Furube and K. Hara, Chem. Mater., 2008, 20, 3993 CrossRef CAS;
(d) D. Kuang, S. Uchida, R. Humphry-Baker, S. Zakeeruddin and M. Grätzel, Angew. Chem., Int. Ed., 2008, 47, 1923 CrossRef CAS.
Footnote |
† Electronic Supplementary Information (ESI) available: Details of the synthesis and characterization of HY102, HY102-1 and HY102-2, procedures of fabricating the DSCs, materials and methods of measurements and calculations. See DOI: 10.1039/c2ra20436c |
|
This journal is © The Royal Society of Chemistry 2012 |