DOI:
10.1039/C2RA20429K
(Paper)
RSC Adv., 2012,
2, 5983-5989
Medicinal plant acid-treatment for a healthier herb tea and recycling of the spent herb residue†
Received
8th March 2012
, Accepted 19th April 2012
First published on 25th April 2012
Abstract
Many kinds of medicinal plants are used in the treatment of disease, and a proportion of these plants grow tenaciously in soils contaminated with heavy metals. An example of one such medicinal plant is the herb Thlaspi rotundifolium L., which accumulates selenium (Se), and has anti-oxidation, anti-aging, anti-cancer and immunity-adjusting properties, but also simultaneously hyperaccumulates harmful metals such as Cd and Zn from the soil. An interesting model named ‘digging metals@plant then backfilling’ has been suggested, where the metals are removed from the plant by acid treatment, then the nano-pores imprinted with the heavy metals are backfilled from the drinking water. Experimental studies of this idea indicate that it can remove unwanted substances from the herb itself and also from the drinking water, ensuring the safety of the herb tea. Additionally, the Se normally embedded in the herb was not noticeably lost. Thus, this model provides a sustainable solution for the disposal of heavy metals using metal-accumulating plants.
Introduction
Since the earliest days, humans have used various natural plants to fight disease. The first Chinese medicinal herb book was written between 1152 and 1578. Medicinal plants often have anti-bacterial, anti-phlogistic, analgesic, anti-pyretic, anti-cancer, anti-aging and immune-regulating functions because they contain various bioactive components (BACs) such as polysaccharides,1,2 glycosides, polyphenols,3 vitamins, amino acids and flavonoids.4,5 In spite of the great advances in modern medicine during recent decades, medicinal plants still make an important contribution to health care. Over the past decade, interest in drugs derived from higher plants, especially the phytotherapeutic ones, has increased extensively. It is estimated that about 25% of all modern medicines are directly or indirectly derived from higher plants.6,7 The therapeutic effects of herbal medicines have been acknowledged by more and more countries.8–10 According to the WHO, because of poverty and a lack of access to modern medicine, about 65–80% of the world's population, who live in developing countries, depend upon plants for primary health care.11
Medicinal plants are found worldwide, many growing wild on roadside verges, hillsides, forests, and escarpments. From the mid-20th century, various industries such as processing, mining and manufacturing have developed significantly. The wanton depletion of resources and the discharge of industrial waste, sewage and noxious gases seriously threatens the environment.12,13 The environment in which these herbs grow has not escaped from pollution. Most medicinal herbs have a tenacious vitality, so that they can tolerate hostile environments, including soil and/or water contaminated by heavy metals. This is why a high proportion of wild herbs have been found to contain large accumulations of heavy metals and can also be used for phytoremediation of contaminated soil.14–17 For example, Egeria najas has the functions of heat-clearing, damp-inhibition, detumescence and detoxification, but it simultaneously accumulates arsenic (As); Arabis paniculata L. can reduce fever and clear heartburn, but it simultaneously absorbs Pb, Zn and Cd from the soil.18,19 A large number of the transport proteins within the plant, such as glutathione, phytochelatins, metallothioneins and enzymes that play key roles in absorption and transport, are also involved in the absorption, transport and binding of heavy metals from the roots to the stems and leaves.20,21 It is well known that an intake of heavy metals in humans causes lesions of the central nervous system, arteriosclerosis, cancer and a serious impairment of renal function.22–24 Owing to heavy metal accumulation by plants, medicinal herbs can retain their pharmacological activities but may increase health risks to users, particularly herbs such as ginseng and Pinellia ternate of which the roots are eaten. Another example is Thlaspi, which is widely distributed in Asia, Europe and North Africa.17 It has antibacterial, anti-inflammatory, heat-clearing and detoxifying functions, and an important feature of this herb is its ability to concentrate selenium (Se) from the soil. This provides the plant with additional properties— including anti-aging action, anti-cancer and immunity-adjusting features.25–27 Owing to its extraordinary pharmacological activity, the Thlaspi rotundifolium L. herb is cultivated on a large-scale in the Se capital—the Enshi Region of China (Fig. S1†, in the Supplementary Information). Unfortunately, T. rotundifolium can also accumulate large quantities of Cd and Zn from the soil14 (Fig. 1A). In order to use the medicinal herbs safely, it is essential to eliminate these metals from the plants (Fig. 1B).
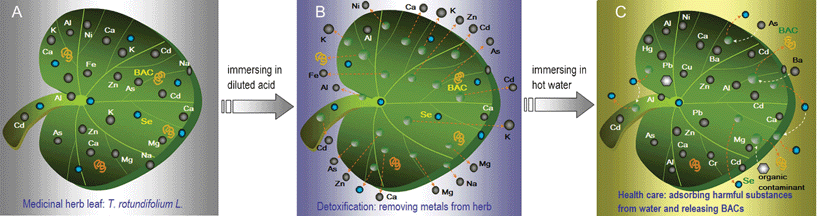 |
| Fig. 1 Cartoon illustration for T. rotundifolium embedding As, BACs and various metals (A), their release causing the formation of metal-imprinting hollows/pores under immersion in acid media (B) and then backfilling and sorption of harmful substances existing in drinking water (C). | |
Results
Effect of acid-treatment on the herb morphology
Heavy metals are often extracted from hyper-accumulator plants by fierce treatment such as incineration or immersion in acid, alkali or diethyl thiocarbamate.28 In order to minimize the loss of BACs from the T. rotundifolium herb, a mild treatment—dilute acid treatment—was assessed. When the herb was treated with 0.1 M hydrochloric acid, 0.5 M citric and 0.5 M acetic acids respectively, there was no significant difference for release of the same metal from the herb, for example 0.25, 0.40 and 0.16 mg l−1 As released, 4.36, 5.54 and 5.63 mg l−1 Se, 106, 90.6 and 56.9 mg l−1 Zn, 31.7, 27.1 and 14.3 mg l−1 Cd and so on (Fig. 2). The amount of As, Se and metals Zn, Cd, Mg, Ca, Al, Na and K removed from the herb was calculated to be 37, 33 and 31 mg g−1 with the above three acid media.
Equal to or more than 0.1 M hydrochloric acid and longer than 5 min of the immersion time caused the constant maximum of the metals Zn, Cd, Mg, Ca, Al and K desorbed from the T. rotundifolium herb (Fig. S2, S3†). Also, the release of Se from the herb always remains at a low level (Fig. 2, Fig. S2, S3†). The acid treatment caused the herb to change in appearance from gray to yellow (Fig. S4†). From SEM images of the herb powder (Fig. 3), the raw herb appears in various shapes of fragments (Fig. 3A) with the rough surface (Fig. 3B) attaching lots of irregular pellicle-peeling (Fig. 3C). The acid treatment made the herb surface smooth (Fig. 3F), where many hollows/nano pores were formed (Fig. 3E–F). In addition, plenty of the fiber pipes were exposed in the acid-treated herb (Fig. 3D). It may be attributed to the fact that 0.1 M hydrochloric acid removed inorganic salts and small organic compounds filling into fiber pipes and metals embedding in the plant fiber surface.
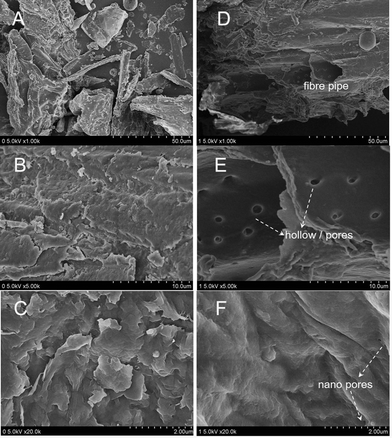 |
| Fig. 3 SEM images of the raw (A to C) and acid-treating (D to F) T. rotundifolium herb. | |
Release of heavy metals from the herb causing formation of pores <20 nm
As, and ten other metals, was found in the T. rotundifolium herb (Fig. 4A). The sum of As, Zn, Cd, Ni and Mn approached 6.3 mg g−1, occupying 8.4% of the total metals (75.0 mg g−1) embedded in the raw herb. After the acid treatment, the total desorption of metals was 63.3 mg g−1, occupying 80% of the total metals. The concentration of Zn was reduced from 5082 to 816 mg l−1, and Cd from 969 to 123 mg l−1, while As, Mg, Ca and K were reduced by over 84%. However, the desorption of Mn was only 40%, and 54% for Ni and Al.
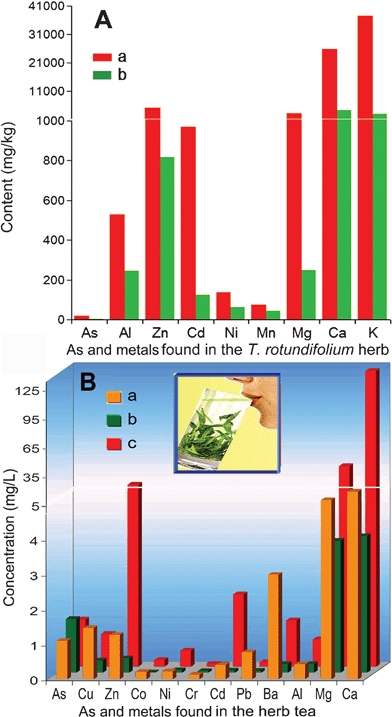 |
| Fig. 4 Distribution of As and metal content (A) embedded in the raw (a) and acid-treating herb (b) and variation of As and metal concentration (B) found in the raw water (a), the medicinal tea immersed in 1% of the acid-treated herb (b) and that immersed in 1% of the raw herb (c). | |
From the cumulative surface area (CSA) curve 2 (Fig. 5A), 0.057 m2 g−1 of <20 nm pores were present in the T. rotundifolium herb treated with 0.1 M hydrochloric acid. The CSA of >20 nm pores occupies only 4% of all of the nano-pores identified. An example of the 30–50 nm pores is shown in Fig. 3E. The cumulative pore volume (CPV) of <20 nm pores in the acid-treated herb was measured at 1.24 × 10−4 cm3 g−1 from curve 2 (Fig. 5B). However, no <20 nm pores were found in the raw herb from curve 1. From curve 3, all 1.6–20 nm pores disappeared, with the CVA of these accounting for only 2.3% of all nano-pores identified in this sample.
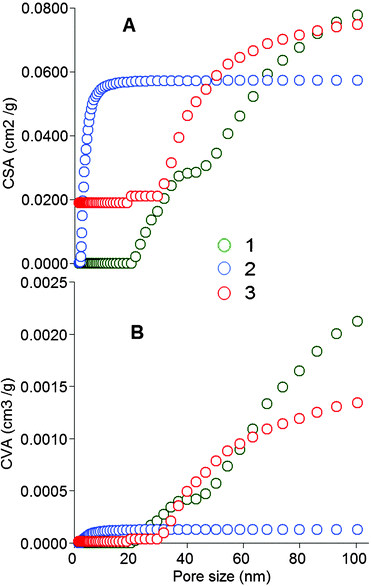 |
| Fig. 5 CSA (A) and CVA (B) of nano pores (<100 nm). 1: Raw T. rotundifolium, 2: the acid-treated herb, 3: the same as 2 after immersion into the heavy metal solution. | |
Alteration of BACs embedded in the acid-treated herb
In addition to the metals, approximately 1700 mg l−1 total organic carbon (TOC) was released whenever the raw herb was immersed in deionized water or in the acid solutions (Fig. S5†). A variety of BACs are contained in the T. rotundifolium herb, including nutritious polysaccharides, catechols and amino acids, and medicinal Se. After acid treatment, Se release decreased by 16%, from 0.567 to 0.476 mg g−1, polysaccharide release increased from 23 to 27 mg g−1, catechol release decreased from 8 to 3.8 mg g−1 and free amino acid released decreased from 3.4 to 1.6 mg g−1.
Capture of harmful substances with the acid-treated herb
A medicinal herb is regularly immersed in 70–80 °C hot water to form a herb tea as treatment for a disease. However, the water used to immerse a herb, such as surface or underground water, often contains various metal ions including Na+, K+, Mg2+, Ca2+, Fe2+ and Al3+. Especially in the last decade, the intemperate exploitation, mining and smelting of nonferrous metals has caused the pollution of many bodies of water by heavy metals. An example of this is the heavy metal pollution occurring in the Xiangjiang and Longjiang Rivers, which caused a problem with the drinking water quality around the basins in 2009 and 2012.13,29 A large bloom of blue-green algae, together with the highly toxic microcystins (MCs) and pesticides occurring in Taihu Lake, caused the water quality to deteriorate severely in 2007, resulting in cessation of the municipal water supply in the Wuxi area.12,30 It is inevitable that there will be some contamination of drinking water with metals and organic contaminants due to geological erosion, sewage intrusion and rainfall. Certainly, the combination of these harmful substances causes disease, while hard water leads to arteriosclerosis and calculus.31–33 Therefore it is essential to remove these contaminants from the drinking water. The acid-treated T. rotundifolium herb exhibited a high adsorption capacity to harmful substances. From the results shown in Fig. 4B, the in situ adsorption of metals clearly increased, particularly Cu2+, Zn2+, Co2+, Ni2+, Cr3+, Cd2+, Pb2+, Ba2+, Ca2+ and Mg2+ with the removal rates ranging from 61.4% to 93.3%, while 42% of Al3+ was adsorbed, although As(III) was seldom adsorbed. The in situ adsorption of metals reached 2.6 mg per gram of the acid-treated T. rotundifolium herb, while the untreated raw herb adsorbed only 36% Cu2+, 41% Cr3+, 85% Pb2+ and 56% Ba2+via surface complexation. The raw herb was ineffective for As(III), Zn2+, Ni2+, Cd2+, Al3+, Mg2+, Ca2+ and Co2+, and it also released large quantities of metals into the hot immersion water (Fig. 4B)—for example, Ni and Al were increased two-fold, Mg 3-fold, Cd 4-fold, Ca 6-fold and Zn 9-fold by comparison with the water itself. From curves 1 and 2 in Fig. 5A, the CSA of the acid-treated herb is less than that of the raw herb (0.087 m2 g−1). From curve 3, <1.6 nm pores were formed with 0.0189 m2 g−1 of CSA, and pores sized from 1.6 to 20 nm almost disappeared. The CPV of <20 nm pores in the acid-treated herb adsorbing metals was 1.32 × 10−5 cm3 g−1 (Fig. 5B).
The effect of two kinds of drinking water used for immersion of the herbs on the adsorption of metals was investigated (Table 1). The As(III), Ca2+, Mg2+ and Cr3+ in the underground water were substantially adsorbed by the acid-treated herb, but Zn2+ was only reduced by 10%. The water hardness, in proportion to total metals but excluding Na+ and K+, was reduced by nearly half. However, the raw herb caused a two-fold increase in water hardness, with Zn increasing from 0.298 up to 5.03 mg l−1, which is more than the guideline value (3 mg l−1) in “Guidelines for Drinking-water Quality” (v-GDWQ).34 With the untreated raw herb, the concentration of Cd in the water increased from 0 to 0.85 mg l−1, which is 283 times the v-GDWQ (0.003 mg l−1),34 Ni increased from 0 to 0.232 mg l−1, which is 3.3 times more than v-GDWQ (0.07 mg l−1),34 Pb increased from 0 to 0.026 mg l−1 ,which is 2.6 times more than v-GDWQ (0.01 mg l−1),34 Mg increased from 23.5 to 46.2 mg l−1 and Ca from 121 to 204 mg l−1 (Table 1). The acid-treated herb adsorbed Ca2+ from the cellar water so that the water hardness was reduced by half, while in contrast the raw herb caused a 7-fold increase in water hardness, i.e. from 20.8 to 154 mg l−1.
Table 1 Change of As and metal concentration in the herb tea containing 1% T. rotundifolium immersed for 20 min at 75 °C
Drinking water |
Chemical elements |
Found, mg l−1 |
Raw water |
Acid-treated herb tea |
Raw herb tea |
Characterized by sum of the metals except for Na and K.
|
Underground water |
Zn |
0.298 |
0.267 |
5.03 |
Pb |
0 |
0 |
0.026 |
Cr |
0.041 |
0 |
0 |
Cd |
0 |
0.028 |
0.843 |
Ni |
0 |
0.031 |
0.232 |
Ba |
0 |
0.01 |
0.034 |
Mg |
23.5 |
18.2 |
46.2 |
Ca |
121 |
62.4 |
204 |
As |
0.018 |
0 |
0 |
Water hardness |
145 |
80.9 |
256 |
Cellar water |
Zn |
0 |
0.369 |
8.85 |
Cd |
0 |
0.034 |
1.20 |
Ni |
0 |
0 |
0.209 |
Al |
0.017 |
0.128 |
0.289 |
Mg |
1.54 |
1.47 |
22.3 |
Ca |
19.2 |
9.95 |
121 |
Na |
11.6 |
20.3 |
35.3 |
K |
7.13 |
11.9 |
162 |
Water hardnessa |
20.8 |
11.9 |
154 |
In addition, the acid-treated T. rotundifolium herb adsorbed organic contaminants e.g. petisides and MCs from drinking water. From the results shown in Fig. 6, the removal rates of water-soluble dimethoate (5 mg l−1) and MC-LR (50 μg l−1) and the hydrophobic parathion-methyl (4 mg l−1) (chemical structure in Fig. S6†) reached 38, 38 and 63%, which are higher than those found with the raw herb, i.e. 30% for dimethoate, 0 for MC-LR and 58% for parathion-methyl.
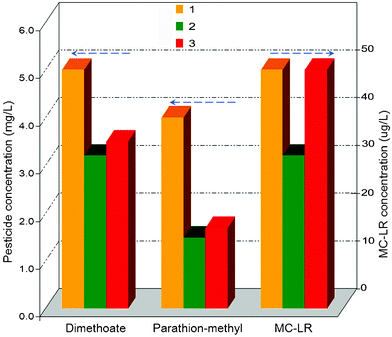 |
| Fig. 6 Dimethoate, parathion-methyl and MC-LR adsorbing to T. rotundifolium herb, which were mixed into: 1—raw water; 2—the same as 1 with 1% acid-treated herb and 3—with 1% raw herb. | |
Recycling disposal of the spent herb
Since heavy metal concentrations are often less than 1 mg l−1 in common drinking water, it is well known that the herb is often immersed two or three times to release the BACs entirely. Each immersion may transfer metal ions from the drinking water to the acid-treated herb at a level of 0.85 to 6.2 mg g−1 (Table 1), which is much less than the total amounts of metals removed from the herb (Fig. 4A). It remains possible for the spent herb to be reused for treatment of metal-contaminated water.35 From the results shown in Fig. 7, the spent herb still adsorbed 3.3 mg g−1 metal ions from a metal-contaminated water sample, especially Zn2+, Pb2+, Co2+, Cd2+, Ni2+, Ba2+, Cr3+, Cu2+ and Al3+. Five cycles indicated that the spent herb adsorbed a relatively constant amount of metals, ranging from 3.1 to 3.5 mg g−1, from the same metal-contaminated water.
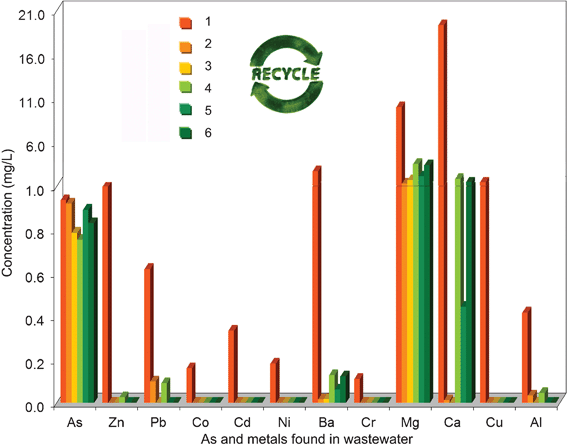 |
| Fig. 7 Change of As(III) and metal ion (Zn2+, Pb2+, Co2+, Cd2+, Ni2+, Ba2+, Cr3+, Mg2+, Ca2+, Cu2+ and Al3+) concentration in the metal-contaminated water (1) by adsorption with the spent herb residue in five cycles (2 to 6). | |
Many micro/nano hollows or pores were formed by acid-treatment (Fig. 3), which could increase exposure of the ligand groups that will potentially bind the metal ions. The exposure of the fiber tubes could also enhance the complexation of metal ions. The CSA and CVA curves confirmed that the nano-pores were present36 after the metals in the herb were removed by acid treatment (Fig. 5A). The acid-treatment seldom affected the IR peak locations of polar organic groups, e.g. –OH/–NH at 3420 cm−1, C
O at 1750 cm−1 and C–O, C–N or C–S from 1070 to 1380 cm−1 (Fig. S7†). After the acid-treated herb was immersed in a solution containing heavy metals, a portion of nano-pores, particularly those <20 nm, were filled with the hydrated metal ions, as illustrated in Fig. 1C.
From measurement of the BACs released from the T. rotundifolium herb, it is clear that the acid treatment did not cause a great loss of Se or polysaccharides, but half of the catechol and free amino acids were lost. It is possible that the basic/ionic organic compounds dissolved more readily in the acidic media. Thus, the acid-treatment may be unsuitable for medicinal herbs containing basic or ionic medicinal BACs such as alkaloids or polyphenols.
From changes of the CSA and CVA (Fig. 5A, B), surface complexation is unlikely to be the primary interaction of metals with the acid-treated herb. The capture of heavy metals from the hot water may be due to the open nano-pores being backfilled (Fig. 1B, C). The disappearance of the 1.6–20 nm pores in the acid-treated herb confirmed the successful backfilling with metal ions (Fig. 5B). The backfilling rate of the <20 nm pores' CSA was 37% (Fig. 5A) and that of the <20 nm pores' CPV 27% (Fig. 5B). Based on this, a new model was suggested named ‘digging metals@plant then backfilling (DMPtB)’. The backfilling may occur for all similarly sized metal ions. For example, the Na- and Ca-imprinted pores were backfilled with Sn2+, Cd2+ or Ca2+ itself. By analogy, the Mg-, Fe- and Zn-imprinted ones were filled with Cr3+, Co2+, Ni2+, Cu2+, Al3+ or themselves, and the K-imprinted ones were filled with Pb2+, Ba2+ or Hg2+ (Fig. 1C). From the results shown in Table 1, the raw herb clearly increased the concentration of heavy metals in the herb tea to higher levels than recommended in the v-GDWQ,37 resulting in the coexistence of toxicity and drug efficacy, colloquially known as ‘drinking poison to quench thirst’. The acid-treated herb exhibited a slightly higher adsorption capacity for organic phosphorus pesticides—dimethoate and parathion-methyl (Fig. S6†)—than the raw herb (Fig. 7). This may be due to their surface interaction with the herb (Fig. 1C). In addition, a cyclic seven-residue nonribosomal peptide, MC-LR produced by several species of cyanobacteria,38,39 is sometimes present in drinking water,12 but it was seldom adsorbed by the raw herb, although up to 38% was captured by the acid-treated herb. This may be due to MC-LR backfilling into the empty hollows/pores. In summary, the acid-treated herb eliminated the toxic heavy metals from the herb itself, and was also capable of removing harmful substances from drinking water to ensure safe herb use.
Conclusions
Heavy metal pollution not only severely damage normal plant growth and metabolism, but also the bioaccumulation of these metals poses a grave risk to human health. Even so, many people depend on medicinal plants for treatment against disease,39 especially among ethnic tribes and in remote mountainous regions and undeveloped countryside. A few people even take herb tea as a tonic and for general daily fluid intake. This work indicates that the amount of harmful metals extracted from the raw herb in hot water is much higher than the concentration found in drinking water. The 0.1 M hydrochloric acid treatment removed 80% of metals from the T. rotundifolium herb and 0.057 m2 g−1 of <20 nm pores were left simultaneously. The acid-treated T. rotundifolium herb obviously adsorbed harmful substances from the herb tea, which is much better than the raw herb. In addition, the spent herb residue may be recycled as a cost-effective sorbent for remediation of metal-contaminated water with a metal-adsorbing capacity of over 3.1 mg g−1. Actually, not only herbs, but tea leaves, coffee and tobacco also accumulate harmful metals.40 The DMPtB provides a feasible solution for safe use of these edible metal-accumulating plants.
Experimental
Treatment of the T. rotundifolium herb
Entire dried herb samples of T. rotundifolium were provided by the Enshi Tengyun Agricultural Sciences and Technology Development Co., Ltd, Xintang Town, Enshi, China. The samples were washed with distilled water to remove any impurity attached to the surface of the herb. After drying at room temperature, the plant was milled to powder it. After the powder was passed through a 150 μm sieve and collected in a drier, 5 g of the herb powder was mixed with 100 ml of 0.1 M hydrochloric acid for 5–10 min, stirring constantly, then centrifuged. After centrifugation, the concentration of various metals and TOC in the supernatant was determined. The pellet was washed with distilled water, then dried for 12 h at 60 °C before being used for acid treatment.
Characterization of structure and morphology
The raw and acid-treated herb powders were photographed and their morphologies were characterized by scanning electronic microscopy (SEM) (Model Quanta 200 FEG, FEI Co., USA). Their infrared spectra were measured with a Fourier transform infrared spectrometer (Model NICOLET 5700, ThermoElectron Co., USA) to examine any changes in the surface ligand structure. The size and surface area of any micro-pores in the herb powders were determined with a surface area and porosimetry analyzer (Model ASAP2020, Micromeritics Co., USA).
Release of BACs and adsorption of harmful substances
Three types of water samples were used to immerse the herb and make herb tea: municipal water with various metals added; underground water sampled from Huaibei area of Anhui Province, China, which is a very hard water used as the local drinking water; and cellular water sampled from the Huanxian countryside of Gansu Province, China, into which 5.0 mg l−1 dimethoate (CAS No. 61515, content 99.3%, Aladdin Reagents, Shanghai), 4.0 mg l−1 parathion-methyl (CAS No. 298000, content 99%, Aladdin Reagents, Shanghai) and 50 μg l−1 MC-LR (CAS No. 101043-37-2, 95–99%, Genbank Co., Taiwan) were added. Conventionally, the herb is immersed in 70–80 °C hot water to release the BACs. Thus, 0.5 g of the raw or the acid-treated herb powder was immersed in 50 ml of each of these three waters and stirred for 20 min in a 75 °C water bath. After cooling, the liquids were centrifuged to obtain the yellow supernatant, i.e. the herb tea.
Recycling of the spent herb residue
The herb waste was collected after production of the herb tea and treated with 100 ml of 0.1 M hydrochloric acid per five gram of herb residue, stirred for 5–10 min, then centrifuged. The supernatant was discarded, and the pellet was washed with distilled water. After drying for 12 h at 60 °C, 1% of the dry herb waste was added to the metal-contaminated water and the mixture adjusted to pH 5 with dilute sodium hydroxide. The mixture was stirred for 20 min at room temperature, then centrifuged. Metals in the supernatant were determined. The herb pellet was reused four times using the same method, i.e. five cycles were carried out.
Determinations
The concentrations of As, Se and the metals Zn, Cd, Ni, Mn, Mg, Ca, K, and Al in the raw and acid-treated herb powders were determined using a mobile X-ray fluorescence spectrometer (EPX-50, Innov-X System Co., USA). The concentrations of As, Se and the metals Zn, Pb, Cd, Mg, Ca, Cu, Al, Na and K released into the acid were determined directly with an inductively coupled plasma-optical emission system (ICP-OES) (Model Optima 2100DV, Perkin-Elmer, USA). Metals in the herb tea were also determined by this method.
The concentrations of TOC in the acid and the herb tea were determined using a total organic carbon analyzer (Model Elab-TOC, Suzhou Ailan Analysis Instrument Co., China).
The crude polysaccharide released into the herb tea was determined with phenol in the presence of sulfuric acid by spectrophotometry.41 The total amount of amino acids released into the herb tea was determined by spectrophotometry using ninhydrin.42
MC-LR in the herb tea was determined by the direct calibration of an enzyme-linked immunosorbent assay (dcELISA) with a Microcystins Plate Kit (96-Well Plate T detection limit > 0.01 ng ml−1, GenBank Co., Taiwan). A porous plate spectrophotometer (Model Power Wave XS2, BioTek Co., USA) was used.
The concentrations of dimethoate and parathion-methyl in the herb tea were determined by HPLC (Model LC-2000, Hitachi, Japan).43 Parathion-methyl was detected at a retention time of 8.7 min with a DAD detector at 280 nm. The flow phase consisted of CH3OH and H2O (v
:
v 70
:
30) at a flow rate of 0.7 ml min−1 with a 250 mm long column (Model Allsphere ODS-2.5 μm). The injection volume was 20 μl. Dimethoate was determined at 14.7 min at 210 nm with a CH3OH:H2O ratio of 30
:
70 (v
:
v) at a flow rate of 1 ml min−1, using the same column, injector and detector.
Acknowledgements
We would like to thank the Enshi Tengyun Agricultural Sciences and Technology Development Co. (Xintang Town, Enshi of China) who provided the T. rotundifolium herb and photographs of the herb plantation, and the Shanghai Institute of Quality Inspection and Technical Research of the National Food Quality Supervision and the Inspection Center of China for determining the concentrations of polysaccharide and catechol.
This work was supported financially by the Foundation of State Key Laboratory of Pollution Control and Resource Reuse of China (Tongji University) (Grant No. PCRRK11003).
References
- T. H. Xue and R. Roy, Science, 2003, 300, 740 CrossRef CAS.
- T. Villanueva, Nat. Rev. Cancer, 2010, 10, 664 CrossRef CAS.
- F. Alen-Ruiz, M. R. Perez-Gregorio, E. Martínez-Carballo, M. S. Garcia-Falcon and J. Simal-Gandara, Electron. J. Environ., Agric. Food Chem., 2008, 7, 3171 CAS.
- M. R. Perez-Gregorio, M. S. Garcia-Falcon, J. Simal-Gandara, A. S. A. Rodrigues and D. P. F. Almeida, J. Food Compos. Anal., 2010, 23, 592 CrossRef.
- A. S. Rodrigues, M. R. Perez-Gregorio, M. S. Garcia-Falcon, J. Simal-Gandara and D. P. F. Almeida, Food Chem., 2011, 124, 303 CrossRef CAS.
- C. M. Cragg, D. J. Newman and K. M. Snader, J. Nat. Prod., 1997, 60, 52 CrossRef.
- Y. Z. Shu, J. Nat. Prod., 1998, 61, 1053 CrossRef CAS.
- Y. Y. Tu, Nat. Med., 2011, 17, 1217 CrossRef CAS.
- T. K. Mutabingwa, D. Anthony, A. Heller, R. Hallett, J. Ahmed and C. Drakeley, Lancet, 2005, 365, 1474 CrossRef CAS.
- M. Wadman, Nature, 2009, 462, 711 CrossRef CAS.
- J. B. Calixto, Braz. J. Med. Biol. Res., 2000, 33, 179 CrossRef CAS.
- L. Guo, Science, 2007, 317, 1166 CrossRef CAS.
- Q. Zhang, Z. Li, G. Zeng, J. Li, Y. Fang and Q. Yuan, Environ. Monit. Assess., 2009, 152, 123 CrossRef CAS.
- N. Verbruggen, C. Hermans and H. Schat, New Phytol., 2009, 181, 759 CrossRef CAS.
- R. Barzanti, F. Ozino, M. Bazzicalupo, R. Gabbrielli and F. Galardi, Microb. Ecol., 2007, 53, 306 CrossRef CAS.
-
A. Catherine Hammett-Stabler, Herbal supplements: efficacy, toxicity, interactions with western drugs, and effects on clinical laboratory tests, John Wiley and Sons, New York, 2011, 353–368 Search PubMed.
- H. Fones, C. A. R. Davis, A. Rico, F. Fang and J. A. C. Smith, PLoS Pathog., 2010, 6, e1001093 Search PubMed.
- S. Clemens, D. M. Antosiewicz, J. M. Ward, D. P. Schachtman and J. I. Schroeder, Proc. Natl. Acad. Sci. U. S. A., 1998, 95, 12043 CrossRef CAS.
- E. W. Grill, E. L. Winnacker and M. H. Zenk, Science, 1985, 230, 674 CAS.
- N. S. Pence, P. B. Larsen and S. D. Ebbs, Proc. Natl. Acad. Sci. U. S. A., 2000, 97, 4956 CrossRef CAS.
- J. L. Hall and L. E. Williams, J. Exp. Bot., 2003, 54, 2601 CrossRef CAS.
- D. E. Salt, R. C. Prince, A. J. M. Baker, I. Raskin and I. J. Pickering, Environ. Sci. Technol., 1999, 33, 713 CrossRef CAS.
- S. Satarug, J. R. Baker, S. Urbenjapol, M. Haswell-Elkins, P. E. B. Reilly, D. J. Williams and M. R. Moore, Toxicol. Lett., 2003, 137, 65 CrossRef CAS.
- S. Tanwar, J. Jain, S. Verma and D. Solanki, Int. J. Pharm. Pharm. Sci., 2012, 4, 219 Search PubMed.
- M. P. Rayman, Lancet, 2000, 356, 233 CrossRef CAS.
- H. Tapiero, D. M. Townsend and K. D. Tew, Biomed. Pharmacother., 2003, 57, 134 CrossRef CAS.
- X. W. Zeng, L. Q. Ma, R. L. Qiu and Y. T. Tang, Environ. Exp. Bot., 2011, 70, 227 CrossRef CAS.
- C. Keller, C. Ludwig, F. Davoli and J. Wochele, Environ. Sci. Technol., 2005, 39, 3359 CrossRef CAS.
-
Q. Wang and F. Huang, River pollution level safe but rising, China Daily Search PubMed,http://www.chinadaily.com.cn/china/, accessed 2012-01-30.
- M. Yang, J. Yu, Z. Li, Z. Guo, M. Burch and T. F. Lin, Science, 2008, 319, 158 CrossRef CAS.
- H. F. Chiu, C. C. Chang, C. C. Chen and C. Y. Yang, J. Toxicol. Environ. Health, Part A, 2010, 74, 62 CrossRef.
- C. Nerbrand, L. Agréus, R. A. Lenner, R. A Nyberg and K. Svärdsudd, BMC Public Health, 2003, 3, 21–21 CrossRef.
- E. Rubenowitz, G. Axelsson and R. Rylander, Epidemiology, 1999, 10, 31 CrossRef CAS.
- World Health Organization (WHO), Guidelines for drinking-water quality, 4th ed., 2011,pp. 327–434.
- S. K. Prabhakaran, K. Vijayaraghavan and R. Balasubramanian, Ind. Eng. Chem. Res., 2009, 48, 2113 CrossRef CAS.
- J. Q. Liu and G. Wulff, J. Am. Chem. Soc., 2004, 126, 7452 CrossRef CAS.
- C. Djediat, M. Malecot, A. de Luze, C. Bernard and S. Puiseux-Dao, Toxicon, 2010, 55, 531 CrossRef CAS.
- C. Song, H. W. Gao and L. L. Wu, Toxicol. Sci., 2011, 122, 395 CrossRef CAS.
- R. Stone, Science, 2008, 319, 709 CrossRef CAS.
- R. J. O'Connor, Q. Li and W. E. Stephens, Tob. Control, 2010, 19, i47 CrossRef.
- A. K. Saha and C. F. Brewer, Carbohydr. Res., 1994, 254, 157 CrossRef.
- B. Starcher, Anal. Biochem., 2001, 292, 87 CrossRef.
- P. Salm, P. J. Taylor, D. Roberts and J. de. Silva, J. Chromatogr., B: Anal. Technol. Biomed. Life Sci., 2009, 877, 568 CrossRef CAS.
Footnote |
† Electronic Supplementary Information (ESI) available: figures S1–S7. See DOI: 10.1039/c2ra20429k/ |
|
This journal is © The Royal Society of Chemistry 2012 |
Click here to see how this site uses Cookies. View our privacy policy here.