DOI:
10.1039/C2RA20375H
(Paper)
RSC Adv., 2012,
2, 5172-5177
Oil removal from oily water systems using immobilized flaxseed gum gel beads
Received
29th February 2012
, Accepted 23rd March 2012
First published on 26th March 2012
Abstract
Experiments were conducted to evaluate the efficiency of immobilized flaxseed gum gel beads for oil removal from oily wastewater. The adsorption capacities achieved the highest level 0.55 g g−1 (oil/beads) under the following conditions: flaxseed gum content in beads 30 mg g−1, 20 °C, particle diameter of beads 2.0 mm and pH 7.5. Static adsorption kinetics showed that a pseudo-first-order rate equation described the experimental data most accurately (R2 ≥ 0.9112). Principal component analysis (PCA) showed that there are two main aspects influencing the adsorption capacities and describing 99.1% of the total variances. The results showed that immobilized flaxseed gum beads have better adsorption capacities than active carbon. This study provides valuable data for a new environmentally-friendly material for the oil removal from wastewater.
Introduction
Recently, water pollution caused by various oils has been highlighted all over the world. Oil pollution in water is considered to be a severe threat to fish, coral, marine birds, marine organisms, the landscape and even the whole ecosystem.1,6 Major industrial sources of oily water include petroleum refineries, metal manufacturing and machining, and food processors.9 It is of great importance to purify oily polluted water so that water resources can be reused and the ecosystem can be protected.
The best available technologies for oil removal include chemical treatment, gravity separation, sorbents, parallel-plate coalescers, gas floatation, cyclone separation, granular media filtration, cartridge filtration, microfiltration, membrane filtration and ultra filtration membranes.8,12 Among the main existing techniques to remove oil in water, the use of a sorbent seems to be fascinating because it can induce the separation of oil and water so that the oil can easily be removed. The adsorption process is the physical adhesion of polluting chemicals onto the surface of a solid. Nowadays, widely used sorbents can be classified into three classes: polymers, natural materials, and treated cellulosic materials. In recent years scientists have concentrated on adsorbents such as sawdust, walnut shell media,8, bentonite,10 and natural wool fibers.7
The utilization of immobilized flaxseed gum beads as an adsorbent takes advantage of alginate gel beads and the emulsion character of flaxseed gum. Alginic acid is a heteropolysaccharide composed of β-D-mannuronic acid and α-L-guluronic acid. The molecular weight of alginic acid is several tens of thousands to hundreds of thousands and it is nontoxic and has a high viscosity. Because of these physical and chemical characteristics, alginate gels with calcium ions have been used widely for immobilization. In the environmental field, alginate complexes, with several types of adsorbents such as activated carbon, chitosan, polyvinyl alcohol carboxylate and humic acid have been reported and their adsorption characteristics have been estimated.5
Flaxseed gum is a protein and heterogeneous polysaccharide composed of xylose, arabinose, glucose, galactose, galacturonic acid, rhamnose and fucose.3 It was reported to have emulsifying effects and has been used as a stabilizer for oily water systems.11 Flaxseed gum can adsorb oil from oily water systems, thus immobilized flaxseed gum beads can work as an adsorbent to remove oil from water. The use of biomaterials renders the adsorption process more environmentally-friendly.
The purpose of this work is to study the oil sorption in aqueous medium by immobilized flaxseed gum beads and to compare its performance with those of activated carbon. The effects of flaxseed gum content in the beads, type of oil, particle diameter, pH value, adsorption temperature and recycling capacity were studied. Adsorption kinetics and dynamic adsorption capacities were established and principal component analysis was carried out. Its reusability was also assessed. The results of the present study may provide valuable data for pilot-scale process design, as well as industrial scale-up applications for treating oily water using immobilized flaxseed gum beads.
2. Materials and experiments
2.1. Materials
Flaxseed was purchased from the medicinal materials market of Harbin, China. After being washed and dried in the shade, the flaxseed was pulverized to a homogeneous size by a disintegrator (HX-200A, Yong-Kang Hardware and Medical Instrument Company, China) and then sieved (30–40 mesh). Flaxseed sample was defatted by n-hexane extraction in a Soxhlet extractor at 80 °C for 3 h. After defatting, n-hexane was removed at 50 °C under reduced pressure using a rotary evaporator. Twenty-one percent (w/w) of oil was removed. Sodium alginate was purchased from Phoenix Company, China. Double-distilled water was used in all experiments. All other reagents were of analytical grade. The following oils were used in the study:
(1) Standard (light) mineral oil marketed by Bailingwei Scientific Company, China.
(2) Soybean oil, a vegetable oil manufactured in China.
(3) Frying oil, it is cooking frying oil, mainly animal oil, used in local restaurants. There is one percent more of C 18
:
2 and C 18
:
3 trans fatty acids in frying oil than in raw oil.
2.2. Experiments
2.2.1. Extraction of flaxseed gum.
For flaxseed gum extraction, a 250 W, 20 kHz ultrasonic generator (KQ-250DB, Kushan Company of acoustics, China) was used. Three parameters can be set in the ultrasonic generator: temperature, power and time. The defatted flaxseed powder (5.0 g) and double-distilled water (100 mL) were added into a flask, and placed into the ultrasonic cleaning bath. The extraction was performed under previously determined optimal conditions (45 °C, 250 W power for 30 min). The solution was centrifuged at a 9392 × g for 15 min, and two phases were obtained: a solid residue which is the flaxseed sample and supernatants. The supernatants were collected and evaporated to dry at 50 °C under reduced pressure using a rotary evaporator. The dry aqueous extracts were weighed. The extraction process was repeated three times.
2.2.2. Immobilization of flaxseed gum.
Defined concentration of flaxseed gum solution was immobilized on sodium alginate support. Dry flaxseed gum (0.3 to 0.8 g) was dissolved in 10 mL double-distilled water and 10 mL 4.0% (w/v) sodium alginate was added. The mixture was blended by a magnetic stirrer apparatus at 120 rpm for 30 min and immobilized at 4 °C for 3.5 h. Beads were formed by dropwise injection of the mixture into 50 mL 0.1 M calcium chloride (Fig. 1). To remove the unimmobilized part, the beads were washed with water and 0.1 M sodium citrate (pH = 4.8). The beads were then transferred into a 0.1 M calcium chloride solution prior to use.4 The immobilized flaxseed gum beads contain: flaxseed aqueous extracts (1.5–4.0%), 4.0% sodium alginate and water. Water contents of beads were all above 90%.
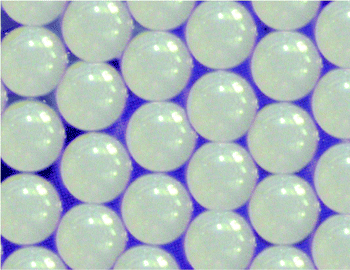 |
| Fig. 1 Macroscopic view of immobilized flaxseed gum gel beads. | |
2.2.3 Removal of oil from water using immobilized flaxseed gum beads.
The oily water was prepared with an oil volume fraction of 10% (v/v) by adding 5.0 g oil to make the 10 mL water in total. 5 g of immobilized flaxseed gum beads were added to oily water in a 50 mL beaker. The beaker was shaken on a flat-bed orbital shaker (model HZQ-X100, Donglian Company, Harbin, China) for 3–21 h at the desired temperatures (25–55 °C). 5 g of immobilized flaxseed gum beads were added to 5 g oil a 50 mL beaker. Beads and oil were separated by a suction funnel.
All the experiments were carried out in triplicate in this study. The results reported are means ± standard deviation of the triplicate data.
2.2.4. Immobilized flaxseed gum beads recovery.
After the saturated adsorption, the recovery of beads was tested. The beads were washed by double-distilled water, then 10.0 g beads were washed with 10.0 mL n-hexane. In order to quantify the remaining oil in it and calculate the recovery rate, the n-hexane was collected and evaporated to dry at 50 °C under reduced pressure using a rotary evaporator. The leftover oil was weighed.
2.5.1. Adsorption kinetics.
The adsorption kinetic curves of oil on immobilized flaxseed gum gel beads were studied by mixing 10 mL oily water containing 5.0 g oil with pre-weighed amounts of 5.0 g wet gel beads in flasks. These flasks were shaken in a constant temperature swing bed (70 rpm) at 40 °C. During this process, adsorption was preformed at certain time intervals until equilibration was reached.
Furthermore, the pseudo-first-order equation by Lagergren and the pseudo-second-order equation was used to evaluate the effect of adsorption time.
The pseudo-first-order equation: dQt/dt = K1(Qe − Qt)
The pseudo-second-order equation: dQt/dt = K2(Qe − Qt)2
where Qe and Qt were the adsorption quantity (g/g) at equilibrium and time t (min), respectively, K1 and K2 were the equilibrium adsorption rate constant in the above two models.2
2.6. Dynamic adsorption capacities
Dynamic adsorption and desorption experiments for oily water solution were carried out on glass columns (12 mm × 500 mm) wet-packed with 10 g (wet weight) of the immobilized flaxseed gum gel beads. The oily water solution was loaded into the top of the glass column and flowed through the glass column at a flow rate of 1 mL min−1. After sorption, the beads were removed by filtration and the oily water emulsion was condensed to a final volume to remove water at 50 °C under reduced pressure using a rotary evaporator. So the oil left was weighted.
The following equations were used to quantify the adsorption of oil in water by immobilized flaxseed gum gel beads.
Adsorption evaluation: Qe = (C0 − Ce)Vi/W
where Qe was the adsorption capacity at adsorption equilibrium (g/g); C0 and Ce were the concentrations of oil in the oily water before and after adsorption by gel beads, respectively (mg mL−1).
Vi was the volume of the initial sample solution (mL) and W was the weight of gel beads (g).2
3. Results and discussion
3.1. Optimization of oil removal from oily water using immobilized flaxseed gum gel beads
3.1.1. Effect of flaxseed gum content.
The adsorption capacities of immobilized flaxseed gum gel beads on different flaxseed gum content were studied at 40 °C, in 5 h adsorption, 6.0 pH (Fig. 2). Adsorption capacity was the weight of oil from oily water removed by unit weight of sorbent. The control one which is beads without flaxseed gum showed an adsorption of 0.12 (g g−1). From Fig. 2, we can see that adsorption capacities reach a peak of 0.48 g g−1 for each 1.0 g beads containing 30 mg flaxseed gum, which is four times more than the control. The results indicated that the interstice among immobilized sodium alginate beads can provide large surface area and filtrate oil from water. The immobilization of flaxseed gum dramatically increases the adsorption capacities due to its emulsifying effects. Above that ratio rigidity of beads was decreased highly, so adsorption capacities dropped significantly. The results indicated that the content of flaxseed gum has a positive relationship with adsorption capacities. But when the content of flaxseed gum exceeded 30 mg g−1, it is harder to form the gel beads. So the flaxseed gum content in beads was chosen as 30 mg g−1.
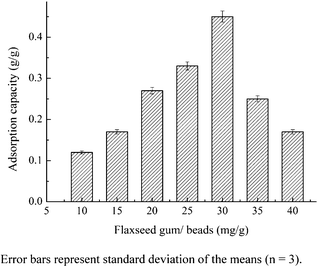 |
| Fig. 2 The optimization of adsorption capacities of immobilized flaxseed gum gel beads on different flaxseed content. | |
3.1.2. Time and different type of oil.
The adsorption capacities of immobilized flaxseed gum beads for different time lengths and different type of oil at 40 °C, 30 mg g−1 flaxseed gum/bead, pH value 6.0 are shown in Fig. 3. Among the three tested oil, soybean oil showed the highest adsorption capacities while the mineral oil showed the least, which correlated with the physical and chemical properties of the gel beads and the chemical features of the oil. Soybean oil is a kind of vegetable oil and flaxseed gum is extracted from plants. They are more similar in the original source. So flaxseed gum gel beads have better adsorption capacities in soybean oil.
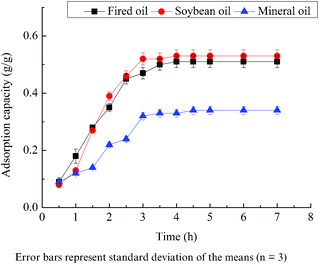 |
| Fig. 3 The optimization of adsorption capacities of immobilized flaxseed gum gel beads on different time and different type. | |
Adsorption capacities showed positive time dependency. From 1 h to 3 h, the adsorption capacities increased dramatically and reached a plateau at 3 h with a maximum adsorption capacity of 0.52 for soybean oil. Therefore, 3 h was sufficient to reach adsorption equilibrium.
3.1.3. Particle diameter, pH value and adsorption temperature.
Four different particle sizes of 1.0, 2.0, 3.0 and 4.0 mm were investigated (Fig. 4A). The adsorption capacities increased as the particle size decreased from 4.0 to 2.0 mm, and then decreased at 1.0 mm. Oil droplets can permeate the interstice among sodium alginate gum gel network.
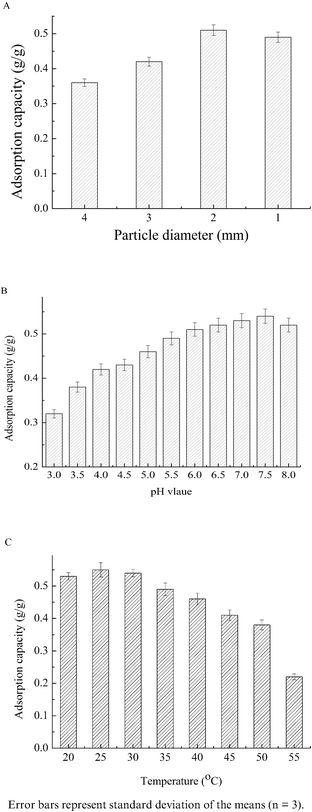 |
| Fig. 4 The optimization of adsorption capacities of immobilized flaxseed gum gel beads on particle diameter, pH value and incubation temperature. | |
Adsorption capacities increased with pH value from 3.0 up to 7.5 and maximum adsorption of 0.54 mg g−1 was obtained at pH 7.5 (Fig. 4B). In alkaline conditions it is more difficult to form beads. So the adsorption capacities dropped at 7.5 pH. The optimal adsorption temperature was 25 °C (Fig. 4C) . Higher temperature lowered the hardness of the beads and rendered the immobilization more difficult. So the adsorption capacities were relatively low.
3.1.4. Recycling capacity.
The adsorption capacities of immobilized flaxseed gum beads on recycling capacity are shown in Fig. 5. The optimal conditions were: 30 mg g−1 flaxseed extracts/bead, at 3 h and 25 °C, 2.0 mm particle diameter and pH 7.5. The results of reusability are presented with the highest adsorption capacities of the first run being assigned the value of 100% activity. We can see that after four times the adsorption capacities dropped 50%, so the recycling capacity were four. The oil reusability of the gel beads is mainly due to two mechanisms: firstly, the sodium alginate gel bead is very porous and the interstice can stock free oil droplets. This part of the oil in the beads can be removed by water. Secondly, the beads have a very large surface area available for adsorption by immobilized flaxseed gum. This kind of oil can be removed by n-hexane.
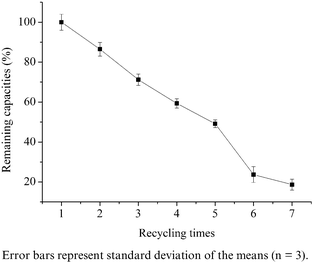 |
| Fig. 5 Effects of recycling times on the adsorption capacities of immobilized flaxseed gum beads. | |
3.2. Adsorption kinetics on immobilized flaxseed gum gel beads
In order to study the mechanism of adsorption and to determine the rate of the adsorption process, the adsorption kinetics data for immobilized flaxseed gum gel beads were analyzed by pseudo-first-order and pseudo-second-order equations. As shown in Table 1, the first-order equation described the experimental data more accurately (R2 ≥ 0.9112) for all the cases, in comparison with the second-order equation regarding the correlation coefficients simulation curve shown in Fig. 6.
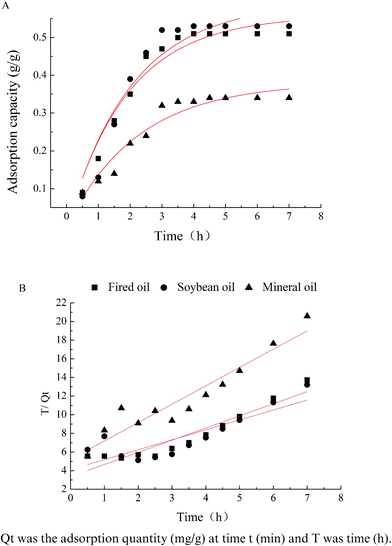 |
| Fig. 6 Pseudo-first-order simulation and pseudo-second-order simulation curve for removal oil by immobilized flaxseed gum gel beads. | |
Table 1 Kinetic parameters for the adsorption of three types of oil
Types of oil |
Pseudo-first-order kinetic model |
Pseudo-second-order kinetic model |
Qe, cal b (mg g−1) |
K1 (min−1) |
R2 |
Qe, cal b (mg g−1) |
K2 (g mg−1 min−1) |
R
2
|
Frying oil |
0.56 |
0.52 |
0.9529 |
0.79 |
0.18 |
0.8874 |
Soybean oil |
0.59 |
0.49 |
0.9112 |
0.94 |
0.27 |
0.6903 |
Mineral oil |
0.38 |
0.44 |
0.9333 |
0.51 |
0.74 |
0.8946 |
The results demonstrated that the adsorption of oil can fit the pseudo-first-order kinetic model and the adsorption mechanism might be a chemisorption process. Furthermore, immobilized flaxseed gum gel beads for exclusion of frying oil were most suitable for the kinetic model.
3.3. Dynamic adsorption capacities
To up-scale the adsorption process in a shorter time, dynamic adsorption for oil was investigated. The adsorption effect of gel beads was determined by surface adsorption and sieve classification. The bed volume (BV) of the resin was 14 mL and the packing length was 10.8 cm. Qe was calculated as the wet weight of gel beads varied from 5.0 g, 10.0 g, 15.0 g, 20.0 g, 25.0 g, 30.0 g, 35.0 g and 40.0 g. Qe was expressed as 0.52 ± 0.1 (g g−1) flaxseed gum gel beads. Data are means of three replicates ± standard deviation.
3.4. Principal component analysis
Principal component analysis (PCA) is a multivariate statistical technique used to identify important components or factors that explain most of the variances of a system.13 In our study, SPSS software 16.0.2 (IBM, US) was used to perform PCA. To evaluate the main factors during the oil removal process, effects of flaxseed content, different type of oil, adsorption time, particle diameter, pH value, adsorption temperature and reproducibility on adsorption capacities, were studied by PCA. Table 2 displays the correlation coefficient of the variables. For this study, a correlation coefficient that was greater than 0.80 (or 80%) was considered significant, which means the interaction between those two variables were significant. These significant variables have direct correlations with each other. It means when the variables are used together to elucidate the process, they have overlaps on the information of the subject. So the number of variables can be reduced by using an orthogonal transformation to convert a set of observations of correlated variables into a set of values of uncorrelated variables called principal components. In a PCA, the number of components is less than or equal to the number of original variables. In each component, there are eight variables in this study. In the study only two eigenvalues were larger than 1 (6.755 and 1.181), so two components were extracted. The first component accounted for about 84.4% and the second component accounted for about 14.7% of the total information in the data set. Therefore, the eight correlated variables can be described by the following two uncorrelated components; as they could describe 99.1% of the total variances:
Table 2 The correlation coefficient of the variables
Correlation |
Flaxseed content |
Frying oil |
Soybean oil |
Mineral oil |
Particle diameter |
pH |
Temperature |
Reproducibility |
Flaxseed content |
1.000 |
0.870 |
0.885 |
0.932 |
0.919 |
0.944 |
−0.650 |
−0.995 |
Frying oil |
0.870 |
1.000 |
0.999 |
0.971 |
0.605 |
0.697 |
−0.928 |
−0.857 |
Soybean oil |
0.885 |
0.999 |
1.000 |
0.974 |
0.629 |
0.714 |
−0.921 |
−0.871 |
Mineral oil |
0.932 |
0.971 |
0.974 |
1.000 |
0.730 |
0.832 |
−0.815 |
−0.938 |
Particle diameter |
0.919 |
0.605 |
0.629 |
0.730 |
1.000 |
0.969 |
−0.305 |
−0.922 |
pH |
0.944 |
0.697 |
0.714 |
0.832 |
0.969 |
1.000 |
−0.387 |
−0.965 |
Temperature |
−0.650 |
−0.928 |
−0.921 |
−0.815 |
−0.305 |
−0.387 |
1.000 |
0.614 |
Reproducibility |
−0.995 |
−0.857 |
−0.871 |
−0.938 |
−0.922 |
−0.965 |
0.614 |
1.000 |
PC1 = 0.983 flaxseed content + 0.944 fry oil −0.953 soybean oil + 0.982 mineral oil concentration + 0.982 particle diameter + 0.891 pH − 0.763 adsorption temperature + −0.979 reproducibility
PC2 = 0.169 flaxseed content −0.329 fry oil −0.303 soybean oil −0.126 mineral oil concentration + 0.539 particle diameter + 0.445 pH + 0.638 adsorption temperature −0.205 reproducibility
The coefficients in the equations were the eigenvectors. In the component matrix, component 1 (PC1) has a large correlation coefficient with all the variables except for adsorption temperature. Meanwhile, component 2 (PC2) has a large correlation coefficient with adsorption temperature. So component 1 contains the information about the seven variables, which represent the internal quality of bead and component 2 contains information about variable adsorption temperature. Thus, the eight variables can be described by two new components and a reduction of dimensionality was achieved.
3.5. Comparison between immobilized flaxseed gum beads and activated carbon.
We calculate the weight of immobilized flaxseed gum beads and activated carbon to remove 1.0 kg oil from oily water under the optimal condition above in the manuscript (Table 3 and Table 4). Removal 1.0 kg oil needs 1.82 kg flaxseed gum beads including 0.055 kg flaxseed gum and 0.036 kg sodium alginate. However, under the same conditions, removal of 1.0 kg oil from oily water required 2.17 kg powdered activated carbon. Water costs 0.01 RMB. Flaxseed costs 1.52 RMB. Sodium alginate and calcium chloride need 1.14 RMB. The total cost is 2.67 RMB. The electricity cost in immobilization process including: flat-bed orbital shaker, ultrasound extraction, centrifugalization and so on is 3.05 RMB. The water cost in the process of making beads is 0.03 RMB. So the total cost to remove 1.0 kg oil from oily water by immobilized flaxseed gum beads is 5.75 RMB. Under the same conditions, removal 1.0 kg oil from oil-in-water emulsion required 2.17 kg powdered activated carbon. The standard activated carbon costs 20 RMB per kg. So the standard cost for removal 1.0 kg oil by activated carbon was 43.4 RMB. It is also noted that immobilized flaxseed gum beads showed a better oil removal efficiency and much lower cost than activated carbon. Immobilized flaxseed gum beads may have higher porosity and surface area. It may be used as an alternative adsorbent for oil removal compared to the more costly standard activated carbon because of low cost and natural abundant resource.
Table 3 Oil removal efficiency from the oil-in-water emulsions samples by adsorption on immobilized flaxseed gum beads and powdered activated carbon
Adsorbent |
Weight (g) |
Initial oil |
Final oil |
Oil removal |
Concentration (g mL−1) |
Concentration (g mL−1) |
(g g−1) |
Immobilized flaxseed gum beads |
5.0 |
0.5 |
0.23 |
0.55 |
Activated carbon |
5.0 |
0.5 |
0.27 |
0.46 |
Table 4 The cost for removal 1.0 kg oil from oil–water emulsions by immobilized flaxseed gum beads and powdered activated carbon
Adsorbent |
Weight (kg) |
Cost (RMB) |
Immobilized flaxseed gum beads |
Flaxseed gum |
0.055 |
1.52 |
Sodium alginate and calcium chloride |
0.036 |
1.14 |
Water |
1.73 |
0.01 |
Total beads |
1.82 |
2.67 |
Activated carbon |
Total |
2.17 |
43.4 |
Conclusions
Oil removal from oily water systems using immobilized flaxseed gum beads was optimized. Per gram, the beads can remove 0.55 g of oil with 30 mg g−1 flaxseed gum beads, at room temperature, with a particle diameter of 2.0 mm and pH of 7.5. The flaxseed gum beads can be reused resulting lower processing costs than traditional oil removal methods. The immobilized flaxseed gum beads method is an environmentally-friendly alternative technology to oil removal with activated carbon that could be potentially applied in the oily water industry.
References
- D.-B. Boylan and B. W. Tripp, Nature, 1971, 203, 44–47 CrossRef.
- C.-Y. Chen, Y.-G. Zu, Y.-J. Fu, M. Luo, C.-J. Zhao, W. Wang, B.-S. Zhao, J. Li and T. Efferth, Biochem. Eng. J., 2011, 56, 84–93 CrossRef CAS.
- H.-H. Chen, S.-Y. Xu and Z. Wang, J. Food Eng., 2006, 77, 295–303 CrossRef CAS.
- G. Fundueanu, C. Nastruzzi, A. Carpov, J. Desbrieres and M. Rinaudo, Biomaterials, 1999, 20, 1427–1435 CrossRef CAS.
- Y.-B. Lin, B. Fugetsu, N. Terui and S. Tanaka, J. Hazard. Mater., 2005, B120, 237–241 CrossRef.
- Y. Loya, Mar. Biol., 1975, 29, 177–185 CrossRef.
- V. Rajaković-Ognjanović, G. Aleksić and L.-j. Rajaković, J. Hazard. Mater., 2008, 154, 558–563 CrossRef.
- A. Srinivasan and T. Viraraghavan, Bioresour. Technol., 2008, 99, 8217–8220 CrossRef CAS.
- A. Srinivasan and T. Viraraghavan, Bioresour. Technol., 2010, 101, 6594–6600 CrossRef CAS.
- A. Shukla, Y.-H. Zhang, P. Dubey, L. J Margrave and S. S. Shukla, J. Hazard. Mater., 2002, B95, 137–152 CrossRef.
- Y. Wang, D. Li, L.-J. Wang and B. Adhikari, J. Food Eng., 2011, 104, 56–62 CrossRef CAS.
- J. Zhong, X. Sun and C. Wang, Sep. Purif. Technol., 2003, 32, 93–98 CrossRef CAS.
- Y. Ouyang, Water Res., 2005, 39, 2621–2635 CrossRef CAS.
|
This journal is © The Royal Society of Chemistry 2012 |
Click here to see how this site uses Cookies. View our privacy policy here.