DOI:
10.1039/C2RA01233B
(Paper)
RSC Adv., 2012,
2, 2073-2079
Received
2nd December 2011
, Accepted 5th December 2011
First published on 13th January 2012
Abstract
Ru immobilized Al2O3 pellets were synthesized as a catalyst for hydrogen generation in NaBH4 solutions. Under the Ru/Al2O3 catalysis, the hydrogen generation rate from the hydrolysis and methanolysis of NaBH4 in alkaline solution was explored as a function of NaBH4 concentration and temperature. Meanwhile, the borate byproducts derived from the NaBH4 hydrolysis were characterized by XRD and NMR. The results showed that the hydrogen generation rate depended on NaBH4 concentration for the hydrolysis, but did not for the methanolysis. 10 wt% NaBH4 hydrolysis containing 1 wt% NaOH catalyzed with 0.5 g Ru/Al2O3 could generate hydrogen with a rate of about 65.5 ml min−1 g−1. The productivity and activation energy of hydrogen generation from the NaBH4 hydrolysis were 93% and 41.8 kJ mol−1, and from the NaBH4 methanolysis were 97% and 51.0 kJ mol−1, respectively. The XRD and NMR analyses showed that the species from the hydrolysis byproducts varied with the NaBH4 concentration could be the borax (Na2B4O7·H2O) and sodium borate hydrate (NaBO2·H2O).
1. Introduction
Air pollution from burning substances containing hydrocarbons and fossil-fuels has deteriorated sharply in decades. Novel clean and renewable energy sources are being developed for solving this problem. It is well known that the hydrogen is the preferred fuel source for providing clean energy through proton exchange membrane (PEM) fuel cells. Hydrogen gas is an anodic fuel for PEM fuel cells in which the only byproduct is water. The controllable generation and purity of hydrogen has made the hydrolysis of chemical hydrides widely studied.1 Among the chemical hydrides, NaBH4 contains a theoretical hydrogen content of 10.8 wt% and has many merits including stability, nonflammable in alkaline solution and recycled byproducts.2–4 The self-hydrolysis of NaBH4 could release hydrogen spontaneously in water:5–8 | NaBH4 + 2H2O → 4H2 + NaBO2 + Heat (−300 kJ mol−1) | (1) |
However, NaBH4 often needs a suitable catalyst to encourage the hydrogen generation in alkaline solution. Numerous catalysts, such as noble metal Ru,9–11Rh,12,13Pt,14,15PtRu,16 and transition metal Co,17,18Ni19,20 and Fe21 have been investigated. Among metal elements, ruthenium (Ru) is the most efficient catalyst to liberate the hydrogen form NaBH4 solutions; Brown and Brown have observed that those metallic elements exerted a catalytic effect on NaBH4 hydrolysis with an order: Ru, Rh > Pt > Co > Ni > Os > Ir > Fe ≫ Pd.7
On the other hand, the hydrolysis of borohydride is not efficient at low temperature which may be inconvenient for automotive and portable applications in high latitude regions. Lo et al.22 reported that NaBH4 could generate hydrogen in methanol at low temperatures (253–323 K). NaBH4 is not only soluble in water (0.55 g g−1 of H2O at 298 K), but also soluble in low molecular weight alcohols (0.164 g g−1 of CH3OH at 293 K);23–25 4 moles hydrogen were generated from 1 mole NaBH4 methanolysis:
| NaBH4 + 4CH3OH → 4H2 + NaB(OCH3)4 | (2) |
Since the composite concept of catalysts is appropriate for easy solid–liquid separation, the controllable initiation or halt of hydrogen generation is therefore highly appreciated in practical applications. Based on the high efficiency of using Ru to catalytically liberate the hydrogen from alkaline NaBH4, this study used an Al2O3 pellet to immobilize the metallic Ru. Meanwhile, through the Ru/Al2O3 catalysis, the properties of NaBH4 hydrolysis and methanolysis were compared, including the effects of NaBH4 concentrations, temperatures (273–303 K), and solvents (water and methanol). In addition, the characterization of borate byproduct collected after NaBH4 hydrolysis was provided by using XRD and NMR analyses.
2. Materials and methods
2.1 Materials
Sodium borohydride (NaBH4, 95%, Riedel-de Haën), sodium hydroxide (NaOH, 99%, Riedel-de Haën), sodium metaborate tetrahydrate (NaBO2·4H2O, 98%, Alfa Aesar), ruthenium(III) chloride hydrate (RuCl3·nH2O, Aldrich) and methanol (CH3OH, ECHO) were all analytical grade and were used without further purification. Al2O3 pellets (Alfa Aesar) pre-calcined at 873 K for 6 h were used as the support for immobilizing Ru; the calcined Al2O3 was mixed with an appropriate quantity of RuCl3·nH2O dissolved in distilled water, and then soaked at room temperature for 24 h. Afterwards, the Ru/Al2O3 precursor was filtered and then loaded in oven at 393 K with N2 atmosphere to remove water. Finally, the dried precursor was calcined at 823 K for 3 h and reduced in hydrogen (40% H2/Ar) at 973 K for 6 h to obtain the Al2O3 supported zerovalent Ru(0) catalysts.
2.2
Hydrogen generation
The hydrogen generation experiment was performed in a three necked flask in which the dissolved NaBH4 and NaOH were mixed with the catalyst in 15 ml water. The hydrogen generation rate and activity energy using the Ru/Al2O3 catalysts were determined by recording the volume of hydrogen released periodically. The temperature of the water bath was maintained with a stability of ± 0.1 °C using a thermostatic circulator.
2.3 Characterization of the Ru/Al2O3 catalysts and spent NaBH4
The surface morphology of the Ru/Al2O3 catalysts was examined using a scanning electron microscope (SEM, JOEL JXA-840, HITACHI S4100), whereas the elemental compositions on the catalyst surface were analyzed by the attached energy dispersive spectrometer (EDS, LINKS AN10000/85S). The crystallization phase of byproducts from spent NaBH4 after hydrogen generation was probed by X-ray powder diffraction obtained at room temperature using a Rigaku RX III powder diffractometer with a Cu Kα radiation. The scanning speed is 0.08 degree per 1 s counting time, while the generator tension was 40 kV/30 mA. Moreover, the chemistry of spent NaBH4 was measured with a nuclear magnetic resonance (NMR) which was recorded in D2O at 500 MHz on a Bruker AV-500 NMR spectrometer.
3. Results and discussion
Fig. 1 indicates that the size of the Ru/Al2O3 catalyst is about a millimetre in thickness and the surface morphology under higher magnification shows the coverage with Ru flakes. The EDS analysis of the catalysts listed in Table 1 suggests that about 5 wt% of the Ru was coated on the surface of the Al2O3. The hydrogen generation from NaBH4 hydrolysis and methanolysis using Ru/Al2O3 catalysts without alkaline were compared in Fig. 2. According to eqn (1) and (2), 1 mole NaBH4 hydrolysis/methanolysis would generate 4 moles hydrogen gas. The volumes of hydrogen generated with different solvents in 1.0 wt% NaBH4 without NaOH at 298 K are almost linearly proportional to the reaction time which indicated their stable catalytic activity. Before the reaction finished, the rate of hydrogen generation decreased due to the low concentration of NaBH4. The final volumes of hydrogen generated by NaBH4 hydrolysis and methanolysis were similar. The hydrogen productivity was calculated according to the following equation, where Vr is the real volume of hydrogen, Vt is the theoretical volume of hydrogen, and WNaBH4 and MNaBH4 are the weight and molecular weight of NaBH4, respectively: | Productivity (%) = (Vr/Vt) × 100 | (3) |
| Vt = (WNaBH4/MNaBH4) × (4/1) × 24.5 (L mol−1 at 298 K) | (4) |
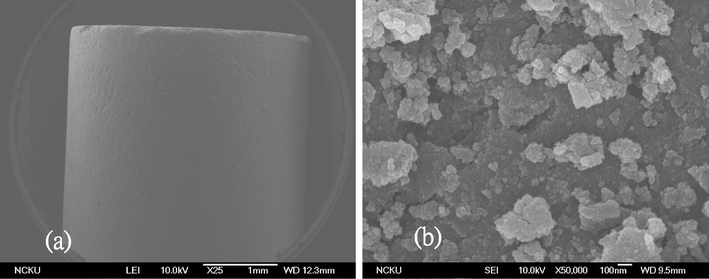 |
| Fig. 1
Scanning electron micrographs of Ru/Al2O3 catalysts (a) 25×, (b)50 000×. | |
Table 1 Surface compositions present in the Ru/Al2O3 catalysts by EDS
Element |
Weight (%) |
Atomic (%) |
Pt is sputtered on sample prior to SEM/EDS analysis to increase the electric conductivity of the sample.
|
O |
44.71 |
62.84 |
Al
|
42.05 |
35.05 |
Ru
|
5.43 |
1.21 |
Pt
a |
7.81 |
0.90 |
The hydrogen productivities of the hydrolysis and methanolysis of NaBH4 are 93% and 97%, respectively. Furthermore, the initial hydrogen generation rates (ml min−1 g−1Ru/Al2O3 cat.) of the NaBH4 hydrolysis and methanolysis independently calculated by the mathematical linear regression are 34.9 and 204.3 ml min−1 g−1Ru/Al2O3 cat., respectively. Both the productivity and the hydrogen generation rate of NaBH4 methanolysis without NaOH addition were better than those for NaBH4 hydrolysis.
3.1 The effect of NaBH4 concentration
The effect of NaBH4 concentration on the hydrogen generation rate at 298 K using 0.5 g Ru/Al2O3 catalysts was shown in Fig. 3. As the NaBH4 concentration increased to 7.5 wt%, the generation rate could be increased to 64.5 ml min−1 g−1 (Fig. 3 (a)); however, the generation rate decreased from 65.5 to 31 ml min−1 g−1 (Fig. 3 (b)) with increasing the NaBH4 from 10 to 30 wt%. Fig. 4 shows viscosities and temperatures as a function of NaBH4 concentration. The excessive NaBH4 (more than 10 wt%) would increase the solution viscosity, but did not significantly change the solution temperature. Solution viscosity affecting the hydrogen generation from NaBH4 hydrolysis has been previously reported in the literature.17,25,26 A higher viscosity solution, due to the excessive NaBH4, was shown to inevitably retard the aqueous mass transfer and reduce the hydrolysis efficiency. Fig. 5 shows the hydrogen generation rates of methanolysis which almost maintained at around 6 ml min−1 g−1 as the NaBH4 concentration ranged from 1 to 10 wt%. Accordingly, Fig. 6 compares the hydrogen generation rate of NaBH4 hydrolysis with that of methanolysis as a function of NaBH4 concentration in NaOH solution. The hydrogen generation obviously depended on the NaBH4 concentration for the hydrolysis, but did not for the methanolysis. Meanwhile, the NaOH played the role of stabilizer for NaBH4 hydrolysis which greatly reduced the hydrogen generation rate of NaBH4 methanolysis from 204.3 to 6 ml min−1 g−1. NaBH4 methanolysis has been known to be a good first-order reaction, of which the rate constant would be remarkably reduced by the trace amount of alkaline.24
 |
| Fig. 3 The effect of (a) low and (b) high concentrations of NaBH4 on hydrogen generation in the hydrolysis of NaBH4 containing 1 wt% NaOH at 298 K using 0.5 g Ru/Al2O3 catalysts. | |
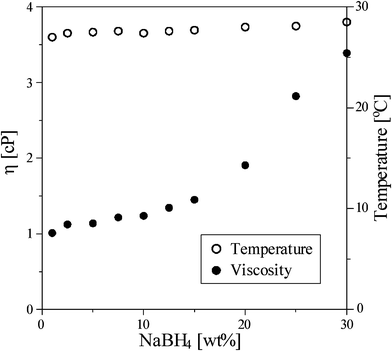 |
| Fig. 4 The solution viscosity and temperature as a function of NaBH4 concentration in water. | |
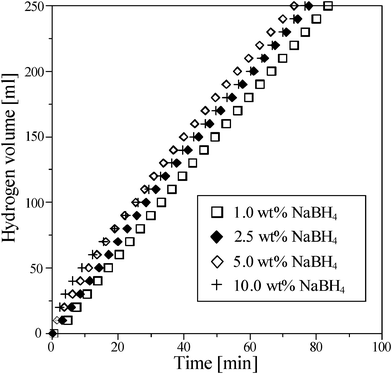 |
| Fig. 5 The effect of various concentrations of NaBH4 on hydrogen generation from the methanolysis of NaBH4 containing 1 wt% NaOH at 298 K using 0.5 g Ru/Al2O3 catalysts. | |
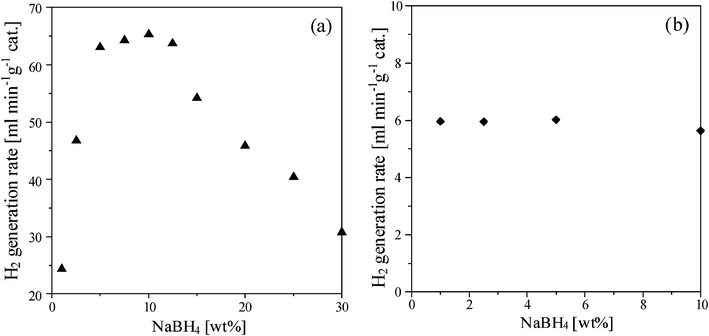 |
| Fig. 6 The rates of hydrogen generated by the (a) hydrolysis and (b) methanolysis of NaBH4 containing 1 wt% NaOH at 298 K using 0.5 g Ru/Al2O3 catalysts. | |
3.2 Effect of temperature
Fig. 7 shows effect of temperature in the range 278–308 K on the hydrogen generation rate of NaBH4 hydrolysis using the Ru/Al2O3 catalyst in alkaline solution. The hydrogen generation rate, that increased with increasing temperature, (Fig. 7 (a)) could be regarded as a zero-order reaction and could be written as: | 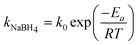 | (5) |
where kNaBH4 is the hydrogen generation rate (mol min−1 g−1) of NaBH4, k0 is the pre-exponential parameter (mol min−1 g−1), Ea is the activation energy of the reaction, R is the gas constant and T is reaction temperature in Kelvins.27
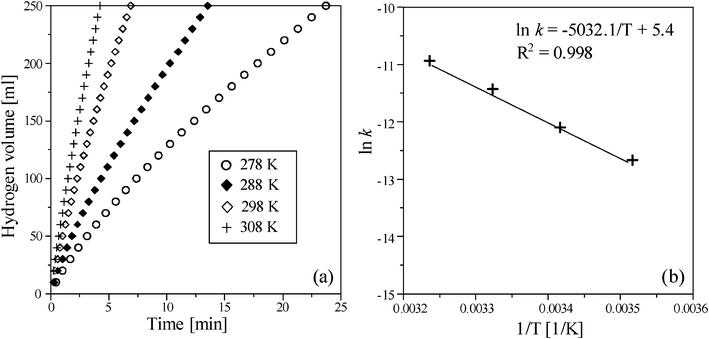 |
| Fig. 7 (a) The effect of temperature on the volume of hydrogen generation in NaBH4 hydrolysis and (b) the Arrhenius plot obtained from different temperatures in the presence of 12.5 wt% NaBH4 containing 1 wt% NaOH using 0.5 g Ru/Al2O3 catalysts. | |
Fig. 7 (b) shows the Arrhenius plot of NaBH4 hydrolysis in which the activation energy could be derived from the slope of ln k linearly reciprocal to absolute temperature (1/T). In addition, the hydrogen generation rate of NaBH4 methanolysis in 273–308 K [in Fig. 8 (a)] also represents a similar trend to that of NaBH4 hydrolysis. The calculated activation energies of the NaBH4 hydrolysis and methanolysis reactions were 41.8 and 51.0 kJ mol−1, respectively (Fig. 7 (b) and Fig. 8 (b)). Table 2 summarizes the metallic catalysts used for NaBH4 hydrolysis in the literature and suggests that the Ru/Al2O3 catalyst in this study exhibits lower activation energy and is highly efficient for use in hydrogen generation.
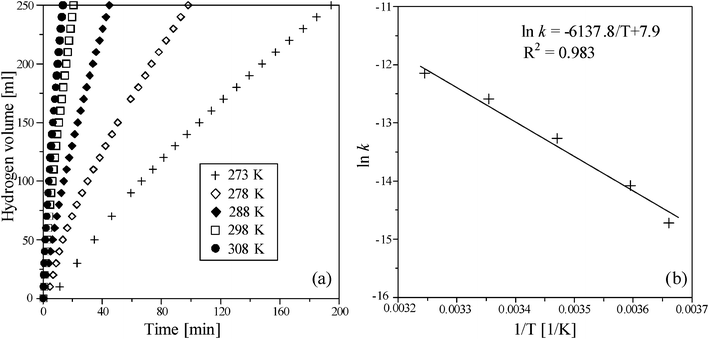 |
| Fig. 8 (a) The effect of temperature on volume of hydrogen generation in NaBH4 methanolysis and (b) the Arrhenius plot obtained from different temperatures in the presence of 10 wt% NaBH4 containing 1 wt% NaOH using 0.5 g Ru/Al2O3 catalysts. | |
Catalyst
|
Condition |
Activation energy (kJ mol−1) |
Reference |
[NaBH4] |
[NaOH] |
Co
|
0.1 mol dm3 |
2 mol dm3 |
75.0 |
Kaufman, 198528 |
Ni
|
|
|
71.0 |
|
RANEY® Ni |
|
|
63.0 |
|
Ru/LiCoO2 |
10.0 wt (%) |
5.0 wt (%) |
68.5 |
Liu, 200829 |
Ni–Ru nanocomposite |
5.0 wt (%) |
5.0 wt (%) |
52.7 |
Liu, 20095 |
Ru/IRA-400 |
20.0 wt (%) |
10.0 wt (%) |
47.0 |
Amendola, 20009 |
|
7.5 wt (%) |
1.0 wt (%) |
56.0 |
|
Ni powder Co powder |
10.0 M |
10.0 wt (%) |
62.7 |
Liu, 200619 |
RANEY® Ni |
10.0 M |
20.0 wt (%) |
41.9 |
|
RANEY® Co |
|
|
50.7 |
|
RANEY® Ni50Co50 |
|
|
53.7 |
|
|
|
|
52.5 |
|
Ru/Al2O3 |
12.5 wt (%) |
10 wt (%) |
14.8 |
This paper |
3.3 Characterization of byproducts after NaBH4 hydrolysis
It is worthy to provide the definite information about the characterization of byproducts in spent NaBH4 solution as its recycling and reuse after hydrogen generation is an issue. Fig. 9 shows the XRD analysis of white crystals collected in the solution after the NaBH4 hydrolysis (1 and 10 wt%) catalyzed with Ru/Al2O3. According to eqn (1), sodium metaborate (NaBO2) is the mainly byproduct after NaBH4 hydrolysis. As compared with the pure borax, however, the crystallization database fitting indicated that the byproduct probably belonged to the Na2B4O7·10H2O or Na2B4O5(OH)4·8H2O phase. White crystals were also found for the 12.5–30 wt% NaBH4 hydrolysis; as shown in Fig. 10, the diffraction peaks of the byproduct well fit with the sodium borate hydrate (NaBO2·2H2O). This result suggested that the byproduct compound from NaBH4 hydrolysis would depend on the NaBH4 concentration.
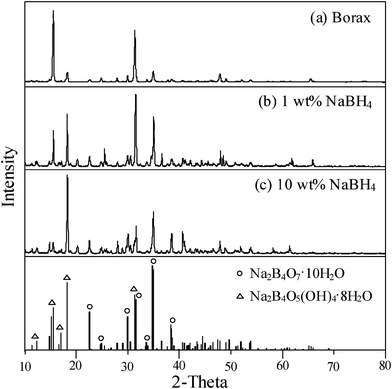 |
| Fig. 9
X-Ray diffraction patterns of (a) pure borax and byproducts after the hydrolysis of (b) 1wt% NaBH4 and (c) 10 wt% NaBH4. containing 1wt% NaOH using 0.5 g Ru/Al2O3 catalysts at 298 K. | |
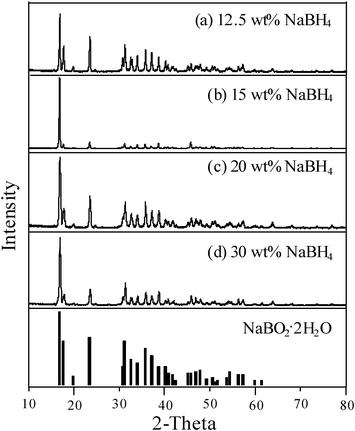 |
| Fig. 10
X-Ray diffraction patterns of byproducts after the hydrolysis of (a) 12.5 wt% NaBH4 (b) 15 wt% NaBH4, (c) 20 wt% NaBH4, and (c) 30 wt% NaBH4 containing 1 wt% NaOH using 0.5 g Ru/Al2O3 catalysts at 298 K. | |
In Fig. 11, the chemistry of the byproducts collected from NaBH4 hydrolysis with various concentrations is analyzed by 11B NMR spectra. Compared to the boron trifluoride diethyl etherate (BF3·C4H10O) the chemical shifts were referenced to the pure Na2B4O7·10H2O sample at −9.6 ppm and the pure NaBO2·4H2O at −15.5 ppm, respectively. A main signal corresponding to the byproduct from 10 wt% NaBH4 hydrolysis is observed at −8 ppm, which is relatively similar to the pure borax (Na2B4O7·10H2O). For the byproducts from higher NaBH4 concentrations (15–30 wt%), the shifts at around −16 ppm could be assigned to the sodium borate hydrate (NaBO2·4H2O). The chemistry of the byproducts from NaBH4 hydrolysis identified by the 11B NMR spectra was in good agreement with that of the XRD analysis.
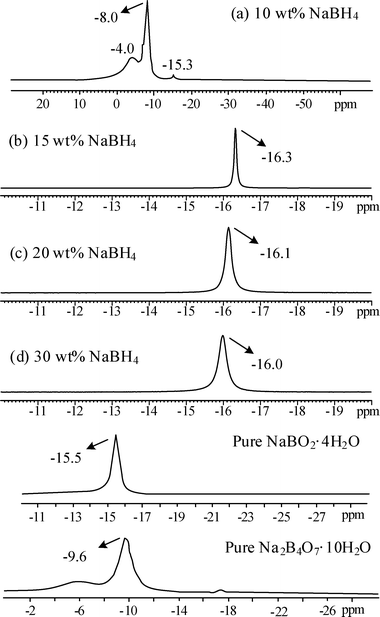 |
| Fig. 11
11B NMR spectra of byproducts after the hydrolysis of (a) 10 wt% (b) 15 wt% (c) 20 wt% (d) 30 wt% NaBH4 containing 1 wt% NaOH and 0.5 g Ru/Al2O3 catalysts. | |
4. Conclusion
This study confirmed that the productivity and hydrogen generation rates of the NaBH4 methanolysis (204.3 ml min−1g−1) without NaOH are better than those of the NaBH4 hydrolysis (34.9 ml min−1g−1). The productivities of hydrogen are 93% and 97% for NaBH4 hydrolysis and methanolysis, respectively. In alkaline solution (1 wt% NaOH), however, the hydrogen generation rate depended on the NaBH4 concentration for the NaBH4 hydrolysis, but did not for the NaBH4 methanolysis. By employing 0.5 g Ru/Al2O3 to catalyze the NaBH4 hydrolysis, the hydrogen generation rate increased from 24.6 to 65.5 ml min−1 g−1 as the NaBH4 concentration increased from 1 to 10 wt%, and decreased to 31 ml min−1g−1 with further increasing the NaBH4 to 30 wt%. Moreover, hydrogen generation rate could only be maintained at around 6 ml min−1 g−1 with increasing NaBH4 from 1 to 10 wt%. On the other hand, the activation energies of the NaBH4 hydrolysis and methanolysis catalyzed with Ru/Al2O3 were 41.8 kJ mol−1 and 51.0 kJ mol−1 at 273–303 K, respectively, which were lower than those reported in the literature. Both the XRD and NMR analyses showed that the byproduct compounds from NaBH4 hydrolysis depend on the NaBH4 concentration. The byproduct was borax (Na2B4O7·H2O) for low NaBH4 concentrations (10 wt%) and sodium borate hydrate (NaBO2·H2O) for high NaBH4 concentrations (15–30 wt%).
References
- A. J. Hung, S. F. Tsai, Y. Y. Hsu, J. R. Ku, Y. H. Chen and C. C. Yu, Int. J. Hydrogen Energy, 2008, 33, 6205 CrossRef CAS.
- U. B. Demirci and P. Miele, Energy Environ. Sci., 2009, 2, 627–637 CAS.
- C. L. Hsueh, C. H. Liu, B. H. Chen, C. Y. Chen, Y. C. Kuo, K. J. Hwang and J. R. Ku, Int. J. Hydrogen Energy, 2009, 34, 1717 CrossRef CAS.
- Z. P. Li, N. Morigazaki, B. H. Liu and S. Suda, J. Alloys Compd., 2003, 349, 232 CrossRef CAS.
- C. H. Liu, B. H. Chen, C. L. Hsueh, J. R. Ku, M. S. Jeng and F. Tsau, Int. J. Hydrogen Energy, 2009, 34, 2153 CrossRef CAS.
- H. I. Schlesingehr, H. C. Browna, A. E. Finholt, J. R. Gilbreath, H. R. Hoekstra and E. K. Hyde, J. Am. Chem. Soc., 1953, 75, 215 CrossRef.
- H. C. Brown and C. A. Brown, J. Am. Chem. Soc., 1962, 84, 1493–1494 CrossRef CAS.
- U. B. Demirci and F. Garin, J. Mol. Catal. A: Chemical, 2008, 279, 57 CrossRef CAS.
- S. C. Amendola, S. L. Sharp-Goldman, M. S. Janjua, N. C. Spencer, M. T. Kelly, P. J. Petillo and M. Binder, Int. J. Hydrogen Energy, 2000, 25, 969 CrossRef CAS.
- C. W. Chen, C. Y. Chen and Y. H. Huang, Int. J. Hydrogen Energy, 2009, 34, 2164 CrossRef CAS.
- Z. T. Xia and S. H. Chan, J. Power Sources, 2005, 152, 46 CrossRef CAS.
- Y. V. Larichev, O. V. Netskina, O. V. Komova and V. I. Simagina, Int. J. Hydrogen Energy, 2010, 35, 6501 CrossRef CAS.
- V. I. Simagina, P. A. Storozhenko, O. V. Netskina, O. V. Komova, G. V. Odegova, Y. V. Larichev, A. V. Ishchenko and A. M. Ozerova, Catal. Today, 2008, 138, 253 CrossRef CAS.
- Y. Kojima, K. Suzuki, K. Fukumoto, M. Sasaki, T. Yamamoto, Y. Kawai and H. Hayashi, Int. J. Hydrogen Energy, 2002, 27, 1029 CrossRef CAS.
- C. Wu, H. Zhang and B. Yi, Catal. Today, 2004, 93–95, 477 CrossRef CAS.
- P. Krishnan, K. L. Hsueh and S. D. Yim, Appl. Catal., B, 2007, 77, 206 CrossRef CAS.
- C. H. Liu, B. H. Chen, C. L. Hsueh, J. R. Ku, F. Tsau and K. J. Hwang, Appl. Catal., B, 2009, 91, 368 CrossRef CAS.
- S. U. Jeong, R. K. Kim, E. A. Cho, H. J. Kim, S. W. Nam, I. H. Oh, S. A. Hong and S. H. Kim, J. Power Sources, 2005, 144, 129 CrossRef CAS.
- B. H. Liu, Z. P. Li and S. Suda, J. Alloys Compd., 2006, 415, 288 CrossRef CAS.
- A. M. F. R. Pinto, D. S. Falcão, R. A. Silva and C. M. Rangel, Int. J. Hydrogen Energy, 2006, 31, 1341 CrossRef CAS.
- C. Cento, P. Dislon and P. P. Prosini, Int. J. Hydrogen Energy, 2009, 34, 4551 CrossRef CAS.
- C. F. Lo, K. Karan and B. R. Davis, Ind. Eng. Chem. Res., 2007, 46, 5478 CrossRef CAS.
- J. Hannauer, U. B. Demirci, G. Pastor, C. Geantet, J. M. Herrmannb and P. Miele, Energy Environ. Sci., 2010, 3, 1796–1803 CAS.
- R. E. Davis and J. A. Gottbrath, J. Am. Chem. Soc., 1962, 84, 895 CrossRef CAS.
- S. C. Amendola, S. L. Sharp-Goldman, M. S. Janjua, M. T. Kelly, P. J. Petillo and M. Binder, J. Power Sources, 2000, 85, 186 CrossRef CAS.
- C. H. Liu, B.H. Chen, C. L. Hsueh, J. R. Ku, M. S. Jeng and F. Tsau, Int. J. Hydrogen Energy, 2009, 34, 2153 CrossRef CAS.
- W. Ye, H. Zhang, D. Xu, L. Ma and B. Yi, J. Power Sources, 2007, 164, 544 CrossRef CAS.
- C. M. Kaufman and B. Sen, J. Chem. Soc., Dalton Trans., 1985, 307 RSC.
- Z. Liu, B. Guo, S. H. Chan, E. H. Tang and L. Hong, J. Power Sources, 2008, 176, 306 CrossRef CAS.
|
This journal is © The Royal Society of Chemistry 2012 |
Click here to see how this site uses Cookies. View our privacy policy here.