DOI:
10.1039/C2RA00048B
(Paper)
RSC Adv., 2012,
2, 4754-4758
Novel “turn-on” fluorescent chemodosimeters based on thioxanthen-9-thione for the selective detection of mercuric ions in aqueous media†
Received
8th January 2012
, Accepted 23rd February 2012
First published on 23rd February 2012
Abstract
Two Hg2+-selective chemodosimeters based on thioxanthen-9-thione (DP-TXT and BDPA-TXT) were synthesized and structurally characterized. The highly sensitive and selective chemodosimetric behaviors of thioxanthen-9-thione towards Hg2+ ions were investigated. Significant chromogenic and fluorogenic signaling occurred from the transformation of thioxanthen-9-thione to thioxanthen-9-one by Hg2+-induced selective desulfurization. Both of the two probes exhibit excellent Hg2+-specific fluorescence enhancement over various competitive cations in aqueous media.
Introduction
To date, fluorescence detection has become the dominant strategy for Hg2+ sensing owing to its operational simplicity, low cost, real time monitoring and high sensitivity.1–6 Our increasing awareness of the deleterious effects of mercury exposure has sparked interest in the development of novel fluorescent probes for detecting Hg2+. As known, there are a number of sophisticated systems for the optical detection of Hg2+ ions,7–20 and many of the small molecule-derived fluorescence sensors for Hg2+ reported so far compose of two components: a recognition moiety containing nitrogen/sulfur atoms and a fluorophore that signals the change of absorption/fluorescence upon exposure to Hg2+.21–28 As a result, Hg2+-triggered desulfurization and transformation of thioamide,29,30 thiourea31–33 and thione34,35 have been successfully utilized. For instance, Czarnik et al. reported that a chemodosimeter based on thioamide substituted anthracene could give 56-fold fluorescence enhancement by Hg2+ addition.36 However, in some cases interference from other metal ions can affect the selectivity, and only a handful of fluorescent sensors displayed good water solubility, so the search for readily accessible fluorescent Hg2+ probes with good water solubility as well as high sensitivity and selectivity is still a challenging task. Herein, we report two thioxanthen-9-thione derivatives, DP-TXT and BDPA-TXT (Scheme 1), can be used as highly sensitive and selective chemodosimeters for Hg2+ ions in aqueous media.
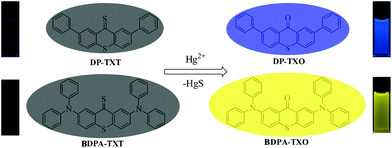 |
| Scheme 1 Fluorescence changes in the presence of Hg2+ and proposed sensing mechanism: desulfurization of DP-TXT and BDPA-TXT promoted by Hg2+. | |
When the carbonyl group in thioxanthen-9-one was replaced by a thiocarbonyl group, there existed obvious fluorescence quenching which was attributed to the heavy atom effect and photo-induced electron transfer (PET) properties of S atoms.35 Hence, we designed and synthesized two thioxanthen-9-thione based chemodosimeters. Addition of Hg2+ resulted in significant fluorescence enhancement by desulfurization of thione. For modification of the optical properties of compound DP-TXO, compound BDPA-TXO possessing diphenylamine group was synthesized via Buchwald–Hartwig amination (Scheme 2). As expected, compound BDPA-TXO exhibited a bathochromic-shift both in UV-vis and fluorescence spectra due to the enhanced intramolecular charge transfer (ICT) process.
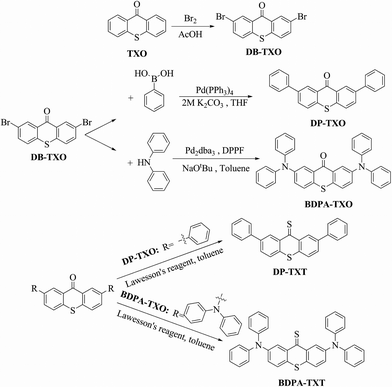 |
| Scheme 2 Synthesis of chemodosimeters DP-TXT and BDPA-TXT. | |
Experimental
Materials
Toluene and tetrahydrofuran (THF) were pre-dried over 4 Å molecular sieves and distilled under an argon atmosphere from sodium and benzophenone immediately prior to use. Unless otherwise stated, all reagents were obtained from commercial suppliers and were used without further purification.
Apparatus
1H NMR and 13C NMR spectra were recorded on Brucker AM-400 spectrometers. Chemical shifts were reported in ppm relative to a tetramethylsilane (TMS) standard in CDCl3 or DMSO-d6. Mass spectra (MS) were obtained on a Waters LCT Premier XE spectrometer. UV-vis absorption spectra were performed on a Varian Cray 500 spectrophotometer and fluorescence spectra were recorded on a Varian Cray Eclipse. Quantum yields of samples (1.0 × 10−5 M in aqueous media) were recorded on a Horiba Fluoromax-4 with a calibrated intergrating sphere system. The melting points were measured on an X4 Micro-melting point apparatus.
Synthesis of 2,7-dibromothioxanthen-9-one (DB-TXO)
To a solution of thioxanthone (5.0 g, 23.6 mmol) in glacial acetic acid (45 mL) was added bromine (10 mL) dropwise over 20 min. The solution was stirred continuously and heated at reflux temperature for 20 h, after which half of the solvent volume was removed by distillation. The reaction mixture was then allowed to cool then poured over crushed ice. The resulting yellow precipitate was collected by filtration and washed with saturated sodium bicarbonate, a 20% aqueous solution of sodium bisulfite, and finally with water. The solid was then dried under vacuum and recrystallized twice from toluene to afford a bright yellow solid (5.1 g, 13.9 mmol, yield: 59%). m.p. 263–266 °C. 1H NMR (400 MHz, CDCl3), δ (ppm): 7.47 (d, J = 8.4 Hz, 2H), 7.74 (d, J = 8.4 Hz, 2H), 8.74 (s, 2H). HR-MS (ESI): calculated for C13H6Br2OS [M + H]+ 368.8584, found 368.8580.
Synthesis of 2,7-diphenylthioxanthen-9-one (DP-TXO)
Phenylboronic acid (0.54 g, 4.4 mmol) and compound DB-TXO (0.736 g, 2.0 mmol) were mixed in THF (15 mL). 10 mL of 2 M aqueous potassium carbonate solution was added, and the mixture was stirred with magnetic stirring. After added tetrakis(triphenylphsosphine)palladium (0.022 g, 0.02 mmol), the reaction solution was heated at reflux temperature for 12 h under the atmosphere of nitrogen. Then the mixture was cooled to room temperature, evaporated under reduced pressure and extracted with dichloromethane (3 × 10 mL). The organic portion was washed with brine and water, and then dried by anhydrous MgSO4. The solvent was evaporated, and the residue was purified by column chromatography with petroleum ether–dichloromethane (2
:
1, v/v) as the eluent to afford a yellow solid (0.61 g, 1.7 mmol, yield: 85%). m.p. 200–203 °C. 1H NMR (400 MHz, CDCl3), δ (ppm): 7.41 (t, J = 7.4 Hz, 2H), 7.50 (t, J = 7.4 Hz, 4H), 7.68 (d, J = 8.4 Hz, 2H), 7.73 (d, J = 7.8 Hz, 4H), 7.90 (dd, J = 8.4 Hz, 2H), 8.89 (d, J = 2.1 Hz, 2H). 13C NMR (100 MHz, CDCl3) δ (ppm): 126.67, 127.14, 127.94, 129.04, 129.43, 131.10, 135.99, 139.41, 139.51, 180.02. HR-MS (ESI): calculated for C25H16OS [M + H]+ 365.1000, found 365.1000.
Synthesis of 2,7-diphenylthioxanthen-9-thione (DP-TXT)
Compound DP-TXO (0.364 g, 1.0 mmol) and Lawesson's reagent (0.405 g, 1.0 mmol) were dissolved in toluene (15 mL) in a 50 mL round-bottom flask. Then the reaction mixture was refluxed for 3 h under the atmosphere of nitrogen. The mixture was concentrated under vacuum, purified by silica gel column chromatography with petroleum ether/dichloromethane (3
:
1, v/v) as eluent to afford a tan solid (0.3 g, 0.8 mmol, yield: 80%). m.p. 210–212 °C. 1H NMR (400 MHz, CDCl3), δ (ppm): 7.40 (t, J = 7.3 Hz, 2H), 7.50 (t, J = 7.6 Hz, 4H), 7.67 (d, J = 8.3 Hz, 2H), 7.71 (d, J = 7.3 Hz, 4H), 7.88 (dd, J = 8.3 Hz, 2H), 9.29 (d, J = 1.8 Hz, 2H). 13C NMR (100 MHz, CDCl3) δ (ppm): 126.60, 127.21, 127.93, 129.03, 130.57, 130.77, 131.61, 137.70, 139.60,140.04, 210.73. HR-MS (ESI): calculated for C25H16S2 [M + H]+ 381.0772, found 381.0773.
Synthesis of 2,7-bis(diphenylamino)thioxanthen-9-one (BDPA-TXO)37,38
Tris(dibenzylideneacetone)dipalladium(0) (Pd2dba3) (0.055 g, 0.06 mmol) and 1,1′-bis(diphenylphosphino)ferrocene (DPPF) (0.066 g, 0.12 mmol) were dissolved in toluene under argon and degassed by two freeze-pump-thaw cycles. This activated catalyst was then added to a degassed mixture of compound DB-TXO (0.5 g, 1.36 mmol), diphenylamine (0.506 g, 2.99 mmol) and sodium tert-butoxide (NaOtBu, 0.366 g, 3.81 mmol) in toluene. The mixture was stirred for 15 h at 90 °C. After the mixture was cooled to room temperature, the reaction was quenched by adding 5 mL of methanol, diluted with diethyl ether, and washed twice with brine and water, respectively. The combined aqueous phase was extracted twice with diethyl ether. The combined organic phase was then dried with MgSO4, filtered, and the solvent evaporated under reduced pressure. The residue was purified by column chromatography on silica gel with petroleum ether–dichloromethane (2
:
1, v/v) as the eluent to afford a yellow solid (0.360 g, 0.66 mmol, yield: 49%). m.p. 250–251 °C. 1H NMR (400 MHz, DMSO-d6), δ (ppm): 7.10 (d, J = 8.1 Hz, 8H), 7.14 (d, J = 7.4 Hz, 4H), 7.34–7.38 (m, 8H), 7.39 (d, J = 2.7 Hz, 2H), 7.75 (d, J = 8.8 Hz, 2H), 7.89 (d, J = 2.6 Hz, 2H). 13C NMR (100 MHz, CDCl3) δ (ppm): 122.57, 123.59, 124.60, 126.89, 128.19, 129.52, 129.82, 130.24, 146.55, 147.16, 179.07. HR-MS (ESI): calculated for C37H26N2OS [M + H]+ 547.1844, found 547.1847.
Synthesis of 2,7-bis(diphenylamino)thioxanthen-9-thione (BDPA-TXT)
Compound BDPA-TXO (0.109 g, 0.2 mmol) and Lawesson's reagent (0.081 g, 0.2 mmol) were dissolved in toluene (10 mL) in a 50 mL round-bottom flask. Then the reaction mixture was refluxed for 3 h under the atmosphere of nitrogen. The mixture was concentrated under vacuum, purified by silica gel column chromatography with petroleum ether–dichloromethane (1
:
1, v/v) as the eluent to afford a dark purple solid (0.086 g, 0.15 mmol, yield: 76%). m.p. 238–239 °C. 1H NMR (400 MHz, DMSO-d6), δ (ppm): 7.10 (d, J = 7.5 Hz, 8H), 7.13 (d, J = 7.3 Hz, 4H), 7.35 (t, J = 8.2 Hz, 8H), 7.41 (d, J = 8.8 Hz, 2H), 7.81 (d, J = 8.8 Hz, 2H), 8.52 (d, J = 2.6 Hz, 2H). 13C NMR (100 MHz, CDCl3) δ (ppm): 123.59, 124.56, 125.29, 125.83, 126.82, 127.80, 129.50, 138.34, 147.07, 208.16. HR-MS (ESI): calculated for C37H26N2S2 [M + H]+ 563.1616, found 563.1615.
Results and discussion
Synthesis and characterization
The synthetic route of DP-TXT and BDPA-TXT is shown in Scheme 2. Bromination of thioxanthen-9-one was carried out according to literature procedures39 to afford a bright yellow solid DB-TXO in 59% yield through recrystallization twice from toluene. It was necessary that one of the reagent bromines should be in large excess for a reaction time of 20 h. Buchwald–Hartwig amination proceeded under the conditions of Pd2dba3 (catalyst), DPPF (ligand), NaOtBu (base) and toluene solutions, and the catalyst and ligand were activated under argon and degassed by two freeze-pump-thaw cycles prior to the addition of other reagents. As for the thionation procedure, it was performed in refluxing toluene with Lawesson's reagent under an atmosphere of nitrogen. The structures of DP-TXT, BDPA-TXT and other intermediate products were confirmed by 1H NMR, 13C NMR, and ESI-MS (Fig. S1–S14 in the ESI†). As for the solvent choosing principles of DP-TXT and BDPA-TXT for the optical detection of Hg2+, acetonitrile (CH3CN) and dimethyl sulfoxide (DMSO) are both water-miscible and are low-toxicity organic solvents, so they provide opportunities for optical detection in aqueous media. What's more, DP-TXT and BDPA-TXT show good solubility in CH3CN and DMSO, respectively.
Colorimetric response of chemodosimeters DP-TXT and BDPA-TXT
As shown in Fig. 1a and Fig. 2, among the various metal ions tested, only Hg2+ significantly affected the absorption behavior of DP-TXT. The absorption band was blue shifted from 487 nm to 397 nm with the addition of Hg2+ in CH3CN–H2O (5
:
5, v/v). The solution color changed from orange to colorless, which allowed a naked-eye detection of Hg2+. As for BDPA-TXT, the absorption band also exhibited a dramatic blue shift upon addition of Hg2+ in DMSO–H2O (9
:
1, v/v), and it displayed an obvious colorimetric response from purple to yellow (Fig. 1b and Fig. 3). All these results above suggest that chemodosimeters DP-TXT and BDPA-TXT can serve as “naked-eye” indicators for Hg2+.
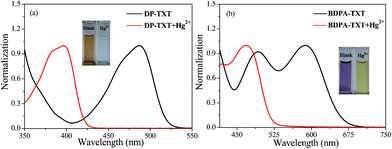 |
| Fig. 1 (a) UV-vis spectra of DP-TXT in the absence (black line) and presence (red line) of Hg2+ in CH3CN–H2O (5 : 5, v/v). Inset: Color change of DP-TXT upon addition of Hg2+. (b) UV-vis spectra of BDPA-TXT in the absence (black line) and presence (red line) of Hg2+ in DMSO–H2O (9 : 1, v/v). Inset: Color change of BDPA-TXT upon addition of Hg2+. | |
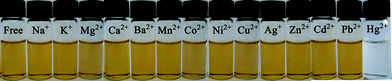 |
| Fig. 2 Color changes of DP-TXT towards various metal ions. | |
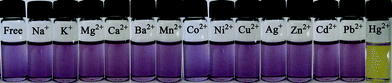 |
| Fig. 3 Color changes of BDPA-TXT towards various metal ions. | |
Fluorescence spectra of chemodosimeter DP-TXT in the presence of Hg2+
DP-TXT exhibited almost no emission centered on 460 nm in 50% aqueous acetonitrile. Upon interaction with Hg2+ ions, however, the fluorescence intensity at 460 nm increased 40-fold and the solution color under illumination with a UV lamp changed from dark to bright blue (Fig. 4), and the absolute fluorescence quantum yield varied dramatically from 1.3% to 21.7%. Furthermore, the fluorescence intensity as a function of Hg2+ concentration shows a near-linear relationship (Fig. S15 in the ESI†). As a consequence, the detection limit reached 21 nM.
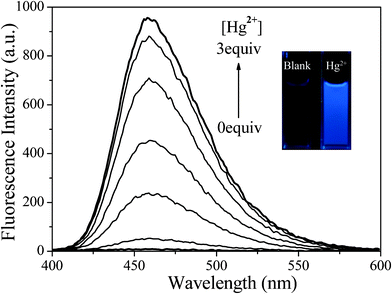 |
| Fig. 4 Fluorescence spectra of DP-TXT (1.0 × 10−5 M) in the presence of Hg2+ (0–3.0 × 10−5 M) in CH3CN–H2O (5 : 5, v/v), λex = 309 nm. Inset: Fluorescence change of DP-TXT upon addition of Hg2+. | |
The Hg2+-selective signaling process of compound DP-TXT was also examined by 1H NMR titration experiment (Fig. S16 in the ESI†). Upon treatment of DP-TXT with 3 equiv. of Hg2+, the resonances of DP-TXT were completely transformed to those of DP-TXO. As shown in Fig. S16,† the resonances of the thioxanthen-9-thione moiety were upfield shifted (from 9.29 to 8.89 ppm for the protons at the ortho position of the thiocarbonyl of DP-TXT), while the resonances for other protons did not significantly change. Analogous to DP-TXT, the peak of the protons at the ortho position of the thiocarbonyl of BDPA-TXT also upfield shifted from 8.52 to 7.89 ppm (Fig. S17 in the ESI†).
Fluorescence spectra of chemodosimeter BDPA-TXT in the presence of Hg2+
The fluorescent changes of BDPA-TXT towards different concentrations of Hg2+ were also examined in DMSO–H2O (9
:
1, v/v). Compared to DP-TXT, the addition of Hg2+ to BDPA-TXT revealed an emission band at 570 nm which bathochromically shifted 110 nm owing to the two diphenylamine moieties introducing as donor groups. A 43-fold fluorescent enhancement was obtained at 570 nm and the solution color varied from nearly dark to bright yellow by using hand-held UV light irradiation (Fig. 5). The absolute fluorescence quantum yields in the absence and presence of Hg2+ were measured to be 0.8% and 9.6%, respectively. The detection limit for Hg2+ with chemodosimeter BDPA-TXT was determined to be 75 nM (Fig. S18 in the ESI†).
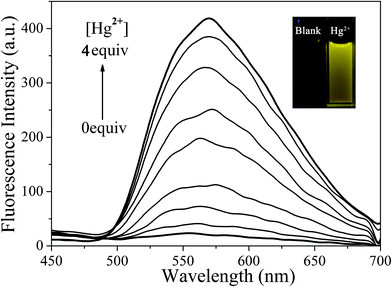 |
| Fig. 5 Fluorescence spectra of BDPA-TXT (1.0 × 10−5 M) in the presence of Hg2+ (0–4.0 × 10−5 M) in DMSO–H2O (9 : 1, v/v), λex = 357 nm. Inset: Fluorescence change of BDPA-TXT upon addition of Hg2+. | |
Moreover, mass spectra demonstrated the proposed Hg2+-induced conversion process by revealing a diagnostic peak at m/z = 547.1847 of BDPA-TXO, instead of the peak at m/z = 563.1615 that would be expected for the molecular ion of BDPA-TXT (Fig. S19 in the ESI†). HRMS (ESI) experiments for DP-TXT also confirmed the Hg2+-triggered desulfurization process (Fig. S20 in the ESI†).
Selective fluorescence response of chemodosimeters DP-TXT and BDPA-TXT
The fluorescence responses of chemodosimeters DP-TXT and BDPA-TXT towards various other metal ions including alkali (Na+, K+), alkaline earth (Mg2+, Ca2+, Ba2+), and transition-metal ions (Mn2+, Co2+, Ni2+, Cu2+, Ag+, Zn2+, Cd2+, Pb2+) were examined, and there is no significant influence on the emission color of DP-TXT except for Hg2+ (Fig. 6a and Fig. 7). Enhancing efficiency, defined as I/I0 (I0 and I represent the fluorescence intensity of DP-TXT in the absence and in the presence of metal ions at 460 nm, respectively), for Hg2+ was calculated to be more than 40, whereas the ratio of I/I0 varied in a limited range from 0.97 (Cu2+) to 1.29 (Ag+) for the other metal ions (Fig. S21a in the ESI†).
![(a) Fluorescence spectra of chemodosimeter DP-TXT towards various metal ions in CH3CN–H2O (5 : 5, v/v). [DP-TXT] = 1.0 × 10−5 M, [Hg2+] = 3.0 × 10−5 M, [Mn+] = 1.0 × 10−4 M. (b) Fluorescence spectra of chemodosimeter BDPA-TXT towards various metal ions in DMSO–H2O (9 : 1, v/v). [BDPA-TXT] = 1.0 × 10−5 M, [Hg2+] = 4.0 × 10−5 M, [Mn+] = 1.0 × 10−4 M.](/image/article/2012/RA/c2ra00048b/c2ra00048b-f6.gif) |
| Fig. 6 (a) Fluorescence spectra of chemodosimeter DP-TXT towards various metal ions in CH3CN–H2O (5 : 5, v/v). [DP-TXT] = 1.0 × 10−5 M, [Hg2+] = 3.0 × 10−5 M, [Mn+] = 1.0 × 10−4 M. (b) Fluorescence spectra of chemodosimeter BDPA-TXT towards various metal ions in DMSO–H2O (9 : 1, v/v). [BDPA-TXT] = 1.0 × 10−5 M, [Hg2+] = 4.0 × 10−5 M, [Mn+] = 1.0 × 10−4 M. | |
 |
| Fig. 7 Fluorescence images of DP-TXT towards various metal ions. | |
Analogous to DP-TXT, no significant fluorescence changes of BDPA-TXT were observed in the presence of other metal ions in excess (Fig. 6b and Fig. 8). Addition of Ag+ resulted in weak fluorescent enhancement at 570 nm because of its weak thiophile ability. The enhancing efficiency of BDPA-TXT remained constant (≈1) after addition of other surveyed metal ions except Ag+ (6.28) and Hg2+ (43.41) (Fig. S21b in the ESI†).
 |
| Fig. 8 Fluorescence images of BDPA-TXT towards various metal ions. | |
Therefore, compounds DP-TXT and BDPA-TXT exhibited specific selectivity for Hg2+ over other examined metal ions in aqueous media.
Conclusions
In conclusion, two new fluorescent chemodosimeters based on thioxanthen-9-thione were developed for the selective detection of mercuric ions in aqueous media. Pronounced Hg2+-selective fluorogenic signaling behaviors were observed: dark to bright blue, dark to bright yellow under illumination with a UV lamp, respectively. Between the two chemodosimeters, DP-TXT exhibits the better sensitivity and selectivity properties with a lower detection limit and less interference from other metal ions. The recognition mechanism is attributed to Hg2+-triggered transformation of thioxanthen-9-thione to a thioxanthen-9-one derivative, and this kind of transformation could potentially be used for the design of other supramolecular systems that show sensitive and selective signaling behaviors for Hg2+ ions in aqueous environments. Furthermore, efforts will be focused on the sensing system's actual applicability, such as the feasibility of detecting real environmental samples in future research.
References
- D. T. Quang and J. S. Kim, Chem. Rev., 2010, 110, 6280 CrossRef CAS.
- X. L. Feng, L. B. Liu, S. Wang and D. B. Zhu, Chem. Soc. Rev., 2010, 39, 2411 RSC.
- E. M. Nolan and S. J. Lippard, J. Am. Chem. Soc., 2003, 125, 14270 CrossRef CAS.
- M. Kumar, R. Kumar and V. Bhalla, RSC Adv., 2011, 1, 1045 RSC.
- Y. Q. Wu, H. Jing, Z. S. Dong, Q. Zhao, H. Z. Wu and F. Y. Li, Inorg. Chem., 2011, 50, 7412 CrossRef CAS.
- L. L. An, Y. L. Tang, F. D. Feng, F. He and S. Wang, J. Mater. Chem., 2007, 17, 4147 RSC.
- R. K. Mahajan, R. Kaur, V. Bhalla, M. Kumar, T. Hattori and S. Miyano, Sens. Actuators, B, 2008, 130, 290 CrossRef.
- C. Chen, R. Y. Wang, L. Q. Guo, N. Y. Fu, H. J. Dong and Y. F. Yuan, Org. Lett., 2011, 13, 1162 CrossRef CAS.
- H. Yang, J. J. Qian, L. T. Li, Z. G. Zhou, D. R. Li, H. X. Wu and S. P. Yang, Inorg. Chim. Acta, 2010, 363, 1755 CrossRef CAS.
- Y. Liu, M. Y. Li, Q. Zhao, H. Z. Wu, K. W. Huang and F. Y. Li, Inorg. Chem., 2011, 50, 5969 CrossRef CAS.
- D. N. Lee, G. J. Kim and H. J. Kim, Tetrahedron Lett., 2009, 50, 4766 CrossRef CAS.
- J. W. Liu and Y. Lu, Angew. Chem., Int. Ed., 2007, 46, 7587 CrossRef CAS.
- X. F. Guo, X. H. Qian and L. H. Jia, J. Am. Chem. Soc., 2004, 126, 2272 CrossRef CAS.
- M. Kumar, R. Kumar and V. Bhalla, Chem. Commun., 2009, 45, 7384 RSC.
- M. Kadarkaraisamy, S. Thammavongkeo, P. N. Basa, G. Caple and A. G. Sykes, Org. Lett., 2011, 13, 2364 CrossRef CAS.
- L. Q. Guo, H. Hu, R. Q. Sun and G. N. Chen, Talanta, 2009, 79, 775 CrossRef CAS.
- J. S. Wu, W. M. Liu, J. C. Ge, H. Y. Zhang and P. F. Wang, Chem. Soc. Rev., 2011, 40, 3483 RSC.
- Q. Liu, J. J. Peng, L. N. Sun and F. Y. Li, ACS Nano, 2011, 5, 8040 CrossRef CAS.
- M. H. Yang, P. Thirupathi and K. H. Lee, Org. Lett., 2011, 13, 5028 CrossRef CAS.
- Q. Zhao, F. Y. Li and C. H. Huang, Chem. Soc. Rev., 2010, 39, 3007 RSC.
- W. Jiang and W. Wang, Chem. Commun., 2009, 45, 3913 RSC.
- W. Huang, D. Y. Wu and C. Y. Duan, Inorg. Chem. Commun., 2010, 13, 294 CrossRef CAS.
- H. G. Wang, Y. P. Li, S. F. Xu, Y. C. Li, C. Zhou, X. L. Fei, L. Sun, C. Q. Zhang, Y. X. Li, Q. B. Yang and X. Y. Xu, Org. Biomol. Chem., 2011, 9, 2850 CAS.
- N. Aksuner, B. Basaran, E. Henden, I. Yilmaz and A. Cukurovali, Dyes Pigm., 2011, 88, 143 CrossRef CAS.
- Y. K. Yang, K. J. Yook and J. Tae, J. Am. Chem. Soc., 2005, 127, 16760 CrossRef CAS.
- Z. K. Wu, Y. F. Zhang, J. S. Ma and G. Q. Yang, Inorg. Chem., 2006, 45, 3140 CrossRef CAS.
- L. Liu, G. X. Zhang, J. F. Xiang, D. Q. Zhang and D. B. Zhu, Org. Lett., 2008, 10, 4581 CrossRef CAS.
- H. N. Kim, M. H. Lee, H. J. Kim, J. S. Kim and J. Yoon, Chem. Soc. Rev., 2008, 37, 1465 RSC.
- K. C. Song, J. S. Kim, S. M. Park, K. C. Chung, S. Ahn and S. K. Chang, Org. Lett., 2006, 8, 3413 CrossRef CAS.
- W. M. Liu, L. W. Xu, H. Y. Zhang, J. J. You, X. L. Zhang, R. L. Sheng, H. P. Li, S. K. Wu and P. F. Wang, Org. Biomol. Chem., 2009, 7, 660 CAS.
- B. Liu and H. Tian, Chem. Commun., 2005, 41, 3156 RSC.
- J. S. Wu, I. C. Hwang, K. S. Kim and J. S. Kim, Org. Lett., 2007, 9, 907 CrossRef CAS.
- M. H. Lee, B. K. Cho, J. Yoon and J. S. Kim, Org. Lett., 2007, 9, 4515 CrossRef CAS.
- M. G. Choi, Y. H. Kim, J. E. Namgoong and S. K. Chang, Chem. Commun., 2009, 45, 3560 RSC.
- G. X. Zhang, D. Q. Zhang, S. W. Yin, X. D. Yang, Z. G. Shuai and D. B. Zhu, Chem. Commun., 2005, 41, 2161 RSC.
- M. Y. Chae and A. W. Czarnik, J. Am. Chem. Soc., 1992, 114, 9704 CrossRef CAS.
- N. Haberkorn, S. A. L. Weber, R. Berger and P. Theato, ACS Appl. Mater. Interfaces, 2010, 2, 1573 CAS.
- Y. Monguchi, K. Kitamoto, T. Ikawa, T. Maegawa and H. Sajiki, Adv. Synth. Catal., 2008, 350, 2767 CrossRef CAS.
- M. P. Coleman and M. K. Boyd, J. Org. Chem., 2002, 67, 7641 CrossRef CAS.
|
This journal is © The Royal Society of Chemistry 2012 |