DOI:
10.1039/C1RA00344E
(Paper)
RSC Adv., 2012,
2, 1577-1584
Highly flexible and stable aptamer-caged nanoparticles for control of thrombin activity†
Received
24th June 2011
, Accepted 2nd November 2011
First published on 22nd December 2011
Abstract
In this paper, we have demonstrated that the thymine linker length number (Tn, n = 0–60) and stem pair number (Pm, m = 0–16) in the terminal of thrombin binding aptamers (TBAs) have a strong impact on the flexibility and stability of TBA-modified gold nanoparticles (TBA–Au NPs) and thus the binding strength and inhibitory potency toward thrombin. The anticoagulation of TBA–Au NPs increased with an increase in the linker length from T0 to T30 due to an increase in the flexibility of G-quadruplexes of TBAs on the Au NP surfaces (TBA-Tn–Au NPs). The inhibition of TBA-PmT15–Au NPs increased with an increase in the Pm from P0 to P8 as a result of the increase in the rigidity and the stability of G-quadruplexes of the TBAs on the Au NP surfaces. The best results were observed for multivalent TBA–Au NPs conjugates—TBA15/TBA29-P8T15–Au NPs—which exhibited ultra-high binding affinity toward thrombin (Kd = 8.86 × 10−12 M) and thus extremely high anticoagulant (inhibitory) potency because of their particularly flexible and stable conformation and multivalency. Compared to the case without inhibitors, their measured thrombin clotting time (TCT) was 296 times longer, whereas for TBA15 alone it was only 3.9 times longer. From the dosage dependence of the TCT delay, we further demonstrated the anticoagulation ability of our TBA15/TBA29-P8T15–Au NPs was much better than the commercial drugs (argatroban and hirudin). Moreover, the Au NPs modified with TBA with photocleavable (PC) units allow a reversal in the activity of TBAPC–Au NPs via near-UV light-inducement of TBA release from Au NPs. We believe that our described techniques can be used widely to modify NPs with other anticoagulant DNA or RNA aptamers towards different proteins such as factor IX, activated protein C, and factor VIIa.
1. Introduction
Aptamers are short, single-stranded oligonucleotides that fold into a specific three-dimensional structure to specifically bind targets, which can range from ions and small organic or inorganic molecules to biomacromolecules and living cells.1–5 In comparison to antibodies, aptamers are cheaper, easier to synthesize and highly stable.6–9 Over the past decade, molecular functional aptamers and the integration of aptamers into nanomaterials has become a new interdisciplinary field that aims at providing new hybrid systems for sensors, cancer cell targeting and therapy.8,10–17 Such multifunctional aptamers exhibit unique optical, electrochemical and magnetic properties, polyvalent interactions, and extraordinary intracellular stability, allowing them to be used as probes, inhibitors or drug delivery systems for target analytes or cells.18–21
Thrombin plays an indispensable role in the coagulation cascade, converting soluble fibrinogen into insoluble strands of fibrin and catalysing many other coagulation-related reactions, including direct activation of protein C, platelets and feedback activation of pro-cofactors V and VIII.22–24 Thus, thrombin is a common target for anticoagulants and antithrombotics.25,26 Anticoagulant drugs that are direct thrombin inhibitors (DTIs), such as heparin, warfarin and bivalirudin, are often used to modulate blood coagulation.27–31 However, they have side effects such as excessive bleeding, thrombocytopenia, narrow therapeutic windows and highly variable dose–response relationships among individuals.32–35 As an alternative, aptamers have been shown to be potential hemostasis-factor-specific anticoagulants, with advantages of high affinity, non-immunogenicity, and nontoxicity.36,37 Two well-known thrombin-binding aptamers (TBAs) possess 15 bases (denoted as TBA15) and 29 bases (denoted as TBA29) that bind to exosite 1 and exosite 2 with dissociation constant (Kd) values of about 100 and 0.5 nM, respectively.38–40 TBA15, which interacts with fibrinogen-binding exosite 1, exhibits the enzymatic-inhibitory functions required for thrombin-mediated coagulation; however, because of its insufficient binding affinity, large amounts of it are needed to achieve the desired anticoagulant response.
Modifications of aptamer that included 4-thio-2′-deoxyuridine,41 locked nucleic acid,42 unlocked nucleic acid monomers,43 2′-O-fluoro,44 2′-deoxy-isoguanosine,45 2′-O-methyl-RNA nucleotides,46 phosphorothioate internucleoside linkages,47 partial inversion polarity,48 or changes of loop size,49 sequence,50 and terminal extension of oligonucleotides (< 8 mer)51 and poly(ethylene glycol) (PEG)52 have been employed to increasing affinity or resistance. Few of these modifications (phosphothioate, 2′-O-methyl, 2′-O-fluoro, etc.), can provide good resistance against enzymatic hydrolysis, while toxicity due to over dose of these non-natural compounds becomes a serious problem. Conjugation of PEG leads to an increase in the stability of aptamer and also facilitates clinical delivery. However, PEG reduces binding affinity between the PEG–aptamers and thrombin molecules.
Recently, several studies have demonstrated that a linear assembly of TBA15, TBA29, and engineering dendritic aptamers improves thrombin inhibition via multivalent interactions.53–59 Low flexibility of assembly aptamers and low rigidity of G-quadruplex structures of TBAs leads to limiting their inhibitory potencies. Recently, we have reported TBA29-conjugated gold nanoparticles (TBA29–Au NPs) provide a 82-fold improvement in anticoagulant potency via thrombin-mediated coagulation made possible by steric blocking and a high binding affinity toward thrombin in solutions that mimic biological plasma.60 We also used TBA–Au NPs with a self-assembled arranged monolayer (SAAM) to enhance the binding affinity of TBAs toward thrombin.61 More recently, we further incorporated sulfated galactose acid into TBA–Au NPs and demonstrated that it enables effective inhibition of thrombin activity toward fibrinogen.62 Although these TBA–Au NPs are ultra-stable in plasma, the low flexibility and rigidity of the G-quadruplex structures of TABs on nanoparticles limits their inhibitory ability.
It has been suggested that the stability and rigidity of aptamers along with the flexibility of assembly aptamers were indispensable for interaction with targeting proteins.63–65 In this work, we systematically investigated the inhibitory abilities of TBA–Au NPs with various linker lengths and the stem pair numbers of TBAs toward thrombin (Scheme 1a and 1b). We also prepared multivalent Au NP-conjugations of TBA15 and TBA29 on to Au NPs to increase the binding affinity, thereby improving its anticoagulant activity (Scheme 1c). Finally, we demonstrated that Au NPs modified with “caged” TBA with photocleavable (PC) units (2-nitrobenzyl group) allow a reversal in the activity of TBAs–Au NPs via inducement by near-UV light, which breaks a C–O bond releasing a 5′-phosphorylated TBA strand (Scheme 2).
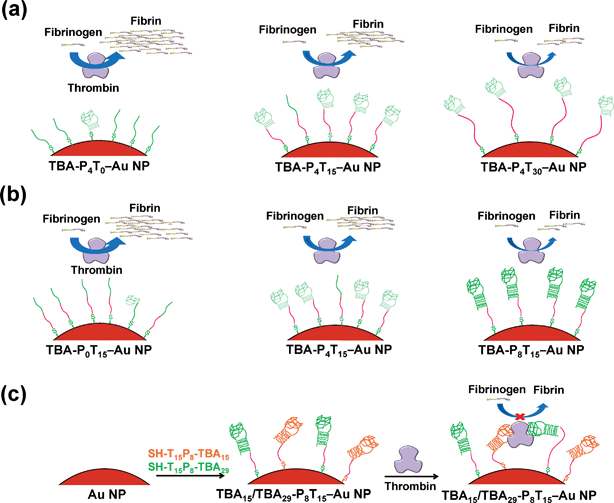 |
| Scheme 1 Schematic representation of the binding and enzymatic inhibition of (a) effect of linker-length with TBA-P4Tn–Au NPs, (b) effect of stem pair with TBA-PmT15–Au NPs, and (c) multivalent TBA15/TBA29-P8T15–Au NPs toward thrombin. | |
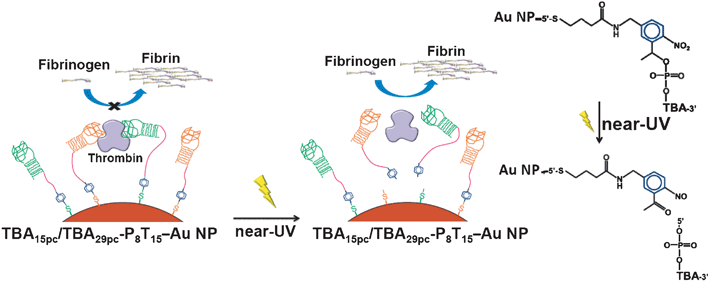 |
| Scheme 2 Schematic representation of reversible inhibitory function of the TBA15pc/TBA29pc-P8T15–Au NPs by irradiation of near-UV light. | |
2. Experimental
2.1 Materials
Calcium chloride (CaCl2), magnesium chloride (MgCl2), potassium chloride (KCl), sodium chloride (NaCl), tris(hydroxymethyl)aminomethane (Tris), trisodium citrate and hydrochloric acid (HCl) were purchased from Mallinckrodt Baker (Phillipsburg, NJ, USA). Human α-thrombin (≥ 1000 NIH units/mg proteins), fibrinogen and bovine serum albumin (BSA) were obtained from Sigma (St. Louis, MO, USA). The anticoagulant drugs argatroban and hirudin were purchased from Sigma. The argatroban (100 μM) or hirudin (100 μM) was prepared in biological buffer (25 mM Tris-HCl (pH 7.4), 150 mM NaCl, 5.0 mM KCl, 1.0 mM MgCl2, and 1.0 mM CaCl2). Reagents used for measuring thrombin clotting time (TCT) were purchased from Helena Laboratories (Victoria, Australia). Oligonucleotides and thiol-modified oligonucleotides listed in Table 1 were purchased from Integrated DNA Technologies (Coralville, IA, USA). All other reagents used in this study were purchased from Aldrich (Milwaukee, WI, USA).
Table 1 DNA sequences of TBAs, TBApc and rDNA used in this study
Name |
Sequencea |
TBA/Au NPb |
The underline was used denote the stem pairs.
Number of TBA molecules per Au NP.
|
TBA15-P0Tn |
5′-Tn GGT TGG TGT GGT TGG-3′ |
— |
TBA29-P4Tn |
5′-Tn AGT CCG TGG TAG GGC AGG TTG GGG TGA CT-3′ |
— |
rDNA-Tn |
5′-Tn GCT GAC TAC ACG CTG TCA TTA-3′ |
— |
TBA15-P0T0-SH |
5′-SH-GGT TGG TGT GGT TGG-3′ |
120 |
TBA15-P0T15-SH |
5′-SH-T15 GGT TGG TGT GGT TGG-3′ |
110 |
TBA15-P0T30-SH |
5′-SH-T30 GGT TGG TGT GGT TGG-3′ |
75 |
TBA15-P0T45-SH |
5′-SH-T45 GGT TGG TGT GGT TGG-3′ |
66 |
TBA15-P0T60-SH |
5′-SH-T60 GGT TGG TGT GGT TGG-3′ |
53 |
TBA29-P4T0-SH |
5′-SH-![[A with combining low line]](https://www.rsc.org/images/entities/char_0041_0332.gif) ![[G with combining low line]](https://www.rsc.org/images/entities/char_0047_0332.gif) CG TGG TAG GGC AGG TTG GGG T![[G with combining low line]](https://www.rsc.org/images/entities/char_0047_0332.gif) ![[C with combining low line]](https://www.rsc.org/images/entities/char_0043_0332.gif) -3′ |
99 |
TBA29-P4T15-SH |
5′-SH-T15 ![[A with combining low line]](https://www.rsc.org/images/entities/char_0041_0332.gif) ![[G with combining low line]](https://www.rsc.org/images/entities/char_0047_0332.gif) CG TGG TAG GGC AGG TTG GGG T![[G with combining low line]](https://www.rsc.org/images/entities/char_0047_0332.gif) ![[C with combining low line]](https://www.rsc.org/images/entities/char_0043_0332.gif) -3′ |
88 |
TBA29-P4T30-SH |
5′-SH-T30 ![[A with combining low line]](https://www.rsc.org/images/entities/char_0041_0332.gif) ![[G with combining low line]](https://www.rsc.org/images/entities/char_0047_0332.gif) CG TGG TAG GGC AGG TTG GGG T![[G with combining low line]](https://www.rsc.org/images/entities/char_0047_0332.gif) ![[C with combining low line]](https://www.rsc.org/images/entities/char_0043_0332.gif) -3′ |
70 |
TBA29-P4T45-SH |
5′-SH-T45 ![[A with combining low line]](https://www.rsc.org/images/entities/char_0041_0332.gif) ![[G with combining low line]](https://www.rsc.org/images/entities/char_0047_0332.gif) CG TGG TAG GGC AGG TTG GGG T![[G with combining low line]](https://www.rsc.org/images/entities/char_0047_0332.gif) ![[C with combining low line]](https://www.rsc.org/images/entities/char_0043_0332.gif) -3′ |
65 |
TBA29-P4T60-SH |
5′-SH-T60 ![[A with combining low line]](https://www.rsc.org/images/entities/char_0041_0332.gif) ![[G with combining low line]](https://www.rsc.org/images/entities/char_0047_0332.gif) CG TGG TAG GGC AGG TTG GGG T![[G with combining low line]](https://www.rsc.org/images/entities/char_0047_0332.gif) ![[C with combining low line]](https://www.rsc.org/images/entities/char_0043_0332.gif) -3′ |
52 |
TBA15-P4T15-SH |
5′-SH-T15 ![[A with combining low line]](https://www.rsc.org/images/entities/char_0041_0332.gif) ![[G with combining low line]](https://www.rsc.org/images/entities/char_0047_0332.gif) CG TGG TTG GTG TGG TTG GGG T![[G with combining low line]](https://www.rsc.org/images/entities/char_0047_0332.gif) ![[C with combining low line]](https://www.rsc.org/images/entities/char_0043_0332.gif) -3′ |
90 |
TBA15-P8T15-SH |
5′-SH-T15 ![[A with combining low line]](https://www.rsc.org/images/entities/char_0041_0332.gif) ![[T with combining low line]](https://www.rsc.org/images/entities/char_0054_0332.gif) ![[T with combining low line]](https://www.rsc.org/images/entities/char_0054_0332.gif) ![[A with combining low line]](https://www.rsc.org/images/entities/char_0041_0332.gif) ![[T with combining low line]](https://www.rsc.org/images/entities/char_0054_0332.gif) C GTG GTT GGT GTG GTT GGG GT ![[A with combining low line]](https://www.rsc.org/images/entities/char_0041_0332.gif) ![[C with combining low line]](https://www.rsc.org/images/entities/char_0043_0332.gif) ![[A with combining low line]](https://www.rsc.org/images/entities/char_0041_0332.gif) ![[G with combining low line]](https://www.rsc.org/images/entities/char_0047_0332.gif) -3′ |
70 |
TBA15-P16T15-SH |
5′-SH-T15 ![[A with combining low line]](https://www.rsc.org/images/entities/char_0041_0332.gif) ![[T with combining low line]](https://www.rsc.org/images/entities/char_0054_0332.gif) ![[C with combining low line]](https://www.rsc.org/images/entities/char_0043_0332.gif) ![[T with combining low line]](https://www.rsc.org/images/entities/char_0054_0332.gif) ![[C with combining low line]](https://www.rsc.org/images/entities/char_0043_0332.gif) ![[T with combining low line]](https://www.rsc.org/images/entities/char_0054_0332.gif) ![[G with combining low line]](https://www.rsc.org/images/entities/char_0047_0332.gif) ![[A with combining low line]](https://www.rsc.org/images/entities/char_0041_0332.gif) ![[A with combining low line]](https://www.rsc.org/images/entities/char_0041_0332.gif) ![[G with combining low line]](https://www.rsc.org/images/entities/char_0047_0332.gif) CG TGG TTG GTG TGG TTG GGG T![[G with combining low line]](https://www.rsc.org/images/entities/char_0047_0332.gif) ![[C with combining low line]](https://www.rsc.org/images/entities/char_0043_0332.gif) ![[T with combining low line]](https://www.rsc.org/images/entities/char_0054_0332.gif) ![[T with combining low line]](https://www.rsc.org/images/entities/char_0054_0332.gif) ![[C with combining low line]](https://www.rsc.org/images/entities/char_0043_0332.gif) ![[A with combining low line]](https://www.rsc.org/images/entities/char_0041_0332.gif) ![[G with combining low line]](https://www.rsc.org/images/entities/char_0047_0332.gif) ![[A with combining low line]](https://www.rsc.org/images/entities/char_0041_0332.gif) ![[G with combining low line]](https://www.rsc.org/images/entities/char_0047_0332.gif) ![[A with combining low line]](https://www.rsc.org/images/entities/char_0041_0332.gif) -3′ |
55 |
TBA29-P0T15-SH |
5′-SH-T15 CGT GGT AGG GCA GGT TGG GGT -3′ |
118 |
TBA29-P8T15-SH |
5′-SH-T15 ![[A with combining low line]](https://www.rsc.org/images/entities/char_0041_0332.gif) ![[T with combining low line]](https://www.rsc.org/images/entities/char_0054_0332.gif) ![[T with combining low line]](https://www.rsc.org/images/entities/char_0054_0332.gif) ![[A with combining low line]](https://www.rsc.org/images/entities/char_0041_0332.gif) ![[T with combining low line]](https://www.rsc.org/images/entities/char_0054_0332.gif) C GTG GTA GGG CAG GTT GGG GT ![[A with combining low line]](https://www.rsc.org/images/entities/char_0041_0332.gif) ![[C with combining low line]](https://www.rsc.org/images/entities/char_0043_0332.gif) ![[A with combining low line]](https://www.rsc.org/images/entities/char_0041_0332.gif) ![[G with combining low line]](https://www.rsc.org/images/entities/char_0047_0332.gif) -3′ |
70 |
TBA29-P16T15-SH |
5′-SH-T15 ![[A with combining low line]](https://www.rsc.org/images/entities/char_0041_0332.gif) ![[T with combining low line]](https://www.rsc.org/images/entities/char_0054_0332.gif) ![[C with combining low line]](https://www.rsc.org/images/entities/char_0043_0332.gif) ![[T with combining low line]](https://www.rsc.org/images/entities/char_0054_0332.gif) ![[C with combining low line]](https://www.rsc.org/images/entities/char_0043_0332.gif) ![[T with combining low line]](https://www.rsc.org/images/entities/char_0054_0332.gif) ![[G with combining low line]](https://www.rsc.org/images/entities/char_0047_0332.gif) ![[A with combining low line]](https://www.rsc.org/images/entities/char_0041_0332.gif) ![[A with combining low line]](https://www.rsc.org/images/entities/char_0041_0332.gif) ![[G with combining low line]](https://www.rsc.org/images/entities/char_0047_0332.gif) CG TGG TAG GGC AGG TTG GGG T![[G with combining low line]](https://www.rsc.org/images/entities/char_0047_0332.gif) ![[C with combining low line]](https://www.rsc.org/images/entities/char_0043_0332.gif) ![[T with combining low line]](https://www.rsc.org/images/entities/char_0054_0332.gif) ![[T with combining low line]](https://www.rsc.org/images/entities/char_0054_0332.gif) ![[C with combining low line]](https://www.rsc.org/images/entities/char_0043_0332.gif) ![[A with combining low line]](https://www.rsc.org/images/entities/char_0041_0332.gif) ![[G with combining low line]](https://www.rsc.org/images/entities/char_0047_0332.gif) ![[A with combining low line]](https://www.rsc.org/images/entities/char_0041_0332.gif) ![[G with combining low line]](https://www.rsc.org/images/entities/char_0047_0332.gif) ![[A with combining low line]](https://www.rsc.org/images/entities/char_0041_0332.gif) -3′ |
55 |
TBA15pc-P8T15-SH |
5′-SH-pc-T15 ![[A with combining low line]](https://www.rsc.org/images/entities/char_0041_0332.gif) ![[T with combining low line]](https://www.rsc.org/images/entities/char_0054_0332.gif) ![[T with combining low line]](https://www.rsc.org/images/entities/char_0054_0332.gif) ![[A with combining low line]](https://www.rsc.org/images/entities/char_0041_0332.gif) ![[T with combining low line]](https://www.rsc.org/images/entities/char_0054_0332.gif) C GTG GTT GGT GTG GTT GGG GT ![[A with combining low line]](https://www.rsc.org/images/entities/char_0041_0332.gif) ![[C with combining low line]](https://www.rsc.org/images/entities/char_0043_0332.gif) ![[A with combining low line]](https://www.rsc.org/images/entities/char_0041_0332.gif) ![[G with combining low line]](https://www.rsc.org/images/entities/char_0047_0332.gif) -3′ |
60 |
TBA29pc-P8T15-SH |
5′-SH-pc-T15 ![[A with combining low line]](https://www.rsc.org/images/entities/char_0041_0332.gif) ![[T with combining low line]](https://www.rsc.org/images/entities/char_0054_0332.gif) ![[T with combining low line]](https://www.rsc.org/images/entities/char_0054_0332.gif) ![[A with combining low line]](https://www.rsc.org/images/entities/char_0041_0332.gif) ![[T with combining low line]](https://www.rsc.org/images/entities/char_0054_0332.gif) C GTG GTA GGG CAG GTT GGG GT ![[A with combining low line]](https://www.rsc.org/images/entities/char_0041_0332.gif) ![[C with combining low line]](https://www.rsc.org/images/entities/char_0043_0332.gif) ![[A with combining low line]](https://www.rsc.org/images/entities/char_0041_0332.gif) ![[G with combining low line]](https://www.rsc.org/images/entities/char_0047_0332.gif) -3′ |
60 |
rDNA-T15-SH |
5′-SH-T15GCT GAC TAC ACG CTG TCA TTA-3′ |
105 |
2.2 Synthesis of Au NPs
Spherical Au NPs (13.3 nm) were prepared by reduction of AuCl4− with citrate. Aqueous 1 mM NaAuCl4 (500 μL) was added all at once into 4 mM trisodium citrate (50 mL) in a round-bottom flask fitted with a reflux condenser.66,67 The solution was boiled for 8 min whilst stirring (during which it changed color from pale yellow to deep red), cooled to room temperature, and kept at 4 °C until use. The size of the Au NPs were determined by transmission electron microscopy (TEM: H7100, Hitachi High-Technologies Corporation, Tokyo, Japan); the NPs appeared to be nearly monodisperse, with an average size of 13.3 ±0.8 nm. The absorption of the Au NP solution was measured by microplate reader (Synergy 2 Multi-Mode Microplate Reader, Biotek Instruments, Winooski, VT). The particle concentration of Au NPs (15 nM) was determined from Beer's law using an extinction coefficient of ca. 2.0 × 108 M−1 cm−1 at 520 nm and an NP size of 13.3 nm.68
2.3 Preparation of TBA–Au NPs
Thiol-modified TBAs were attached to the Au NPs by a modified procedure.69 5′-Thiol-modified oligonucleotides received in the disulfide form HOCH3(CH2)5S-S-3′-oligo reacted directly with the NPs, attaching both the HO(CH2)6S and oligo-S units onto the NP surface. Aliquots of aqueous Au NP solutions (15 nM, 990 μL) in 1.5 mL tubes were mixed with thiol-TBA15 (150 μM, 5 μL) and thiol-TBA29 (150 μM, 5 μL), after 2 h it was prepared under salt aging in the presence of 200 mM NaCl, reacted over 16 h. Salt aging resulted in greater numbers of TBA molecules bound to the Au NPs.70 The mixtures were centrifuged at a relative centrifugal force (RCF) of 30
000g for 25 min to remove unattached thiol oligonucleotides. The supernatants were removed and the oily precipitates were washed with Tris-HCl (pH 7.4, 5.0 mM, 1.0 mL). For determination of the number of TBA molecules per Au NP, the amount of TBA in the supernatant after centrifugation was measured using OliGreen (OG) reagent.71 After 3 centrifuge/wash cycles, the colloids (TBA–Au NPs) were resuspended separately in Tris-HCl (pH 7.4, 5.0 mM, 1.0 mL). The ξ (zeta) potentials and hydrodynamic particle sizes of the TBA–Au NPs were measured on a Zetasizer 3000HS analyzer (Malvern Instruments, Malvern, U. K.).
2.4 Measurement of thrombin clotting time
TCT tests were performed to examine the potency of anticoagulation for the TBA–Au NPs by the common pathway of coagulation. Human plasma (200 μL) was pre-incubated at 37 °C with a 2
:
1 mixture of each of the oligonucleotides (100 μL) (TBA, TBA–Au NP or commercial anticoagulant drugs) in biological buffer [25 mM Tris-HCl (pH 7.4), 150 mM NaCl, 5.0 mM KCl, 1.0 mM MgCl2, and 1.0 mM CaCl2] in the presence of BSA (100 μM) for 180 s. Then pre-incubated thrombin (37 °C, 100 μL, 20 nM) was added to initiate the clotting cascade, and time-course light-scattering measurements were performed at 680 nm to the point of saturation. The TCT was taken at the point where the scattering signal was half-way between the lowest and highest points. Measurements were repeated 3 times; each set of experiments was performed using a single batch of plasma.
2.5 Binding constant
The dissociation constant (Kd) of the TBA–Au NPs with thrombin was determined by mixing aliquots of each TBA–Au NP solution (1.0 pM) separately with different concentrations of thrombin (0–10 pM) for 15 min to form thrombin/TBA–Au NP complexes. The solution was centrifuged (RCF 30
000g) for 20 min. Unbound thrombin in the supernatant was diluted with biological buffer and quantified using fibrinogen and 56 nm Au NPs as we described previously.72 Fibrinogen was added to a solution of 56 nm Au NPs, causing their ready conjugation into 56 nm Fib–Au NPs via electrostatic and hydrophobic interactions.73 Thrombin was then introduced in the presence of excess fibrinogen, inducing formation of insoluble fibrillar fibrin–Au NP agglutinates via polymerization of unconjugated and conjugated fibrinogen. The mixture was centrifuged and the absorbance of the supernatants was measured at 532 nm. For estimation of the binding properties of TBA–Au NPs, saturation binding data were processed further with the Scatchard equation
|
N
Thr/[Free-Thr] = Nmax/Kd − NThr/Kd
| (1) |
where
NThr is the number of thrombin molecules bound to the TBA–
Au NPs at equilibrium,
Nmax is the apparent maximum number of
binding sites, [Free-Thr] is the free thrombin concentration at equilibrium, and
Kd is the dissociation constant. The values of
Kd and
Nmax were calculated from the slope and intercept of the linear plot of
NThr/[Free–Thr]
versus NThr.
2.6 Reverse of thrombin activity
The reverse of the inhibited reaction was performed by photocleavable TBA15PC/TBA29PC-P8T15 modified Au NPs (TBA15pc/TBA29pc-P8T15–Au NPs) which can reverse thrombin activity via irradiation with near-UV light (365 nm) to cleave the 2-nitrobenzyl PC linker of the terminal-modified TBA.74,75 TBA15pc/TBA29pc-P8T15–Au NPs were prepared as described for the TBA–Au NPs. Aliquots of 2-fold diluted plasma with physiological buffer (500 μL) containing TBA15pc/TBA29pc-P8T15–Au NPs (2.5 nM) and thrombin (5.0 nM), were subsequently irradiated by 365 nm UV light (FWHM ∼50 nm, 20 mW cm−2) for 30 min. Time-course light-scattering measurements for determination of TCT were then performed.
3. Results and discussion
3.1. Effects of linker length of TBA-Tn–Au NPs.
Several DNA bioconjugated Au NPs were prepared through Au–S bonding by separately mixing 15 nM Au NP (diameter: 13.3 ±0.6 nm) solutions with one of the 2.0 μM 5′-thiol-modified oligonucleotides (TBA15, TBA29, and random-sequence control DNA). We performed five linker length (T0, T15, T30, T45, and T60) to test the effect of the distance between nanoparticle and aptamer toward the inhibitory efficiency. For simplicity, we denoted the Apt–Au NPs having Tn-linker and Pm-stem pair of aptamer molecules on Au NP as TBA-PmTn–Au NPs; TBA29-P4T15–Au NPs represent the TBA29 with 4-stem pair number and 15-mer thymidine on its' terminal. Please note the reported structure of TBA29 has 4 stem pair number (P4), while TBA15 does not.38–40 To investigate the effect of linker length and stem pair number of TBA on the inhibition of thrombin, we performed typical TCT tests using mixtures of thrombin (5.0 nM) and 2-fold diluted plasma sample in the presence and absence of the following DNA strands: TBA15-P0Tn (100 nM), TBA29-P4Tn (100 nM), TBA15-P0T0–Au NPs (0.83 nM), TBA15-P0T15–Au NPs (0.91 nM), TBA15-P0T30–Au NPs (1.30 nM), TBA15-P0T45–Au NPs (1.52 nM), TBA15-P0T60–Au NPs (1.89 nM), TBA29-P4T0–Au NPs (1.00 nM), TBA29-P4T15–Au NPs (1.14 nM), TBA29-P4T30–Au NPs (1.43 nM), TBA29-P4T45–Au NPs (1.54 nM) and TBA29-P4T60–Au NPs (1.92 nM). TCT is a common test performed in patients suspected of suffering from coagulopathy. The measurement of TCT is a common screening test for factors I, IIa, and XIII of the common pathways.76–78 The concentration of each of the oligonucleotides was held constant (100 nM) in these experiments. The numbers of TBA15 and TBA29 on each NP are listed in Table 1. We noted the decrease in the number of TBA density on Au NPs with the increase in the linker length from T0 to T60.
Fig. 1 shows that in the TCT test, the presence of inhibitors prolong the TCT. The inhibition strengths of TBA and TBA–Au NPs toward thrombin were compared based on their TCT delay [(t − t0)/t0], where t0 and t are the TCT in the absence and presence, respectively, of inhibitors; with higher TCT delay representing stronger inhibition efficiency for anticoagulation. Of the two free aptamers, only TBA15 exhibited a significant inhibition effect of thrombin-induced coagulation. This result was expected because TBA15 targets the critical exosite 1, whereas TBA29 does not.38–40 In controlled experiments, the random DNA (rDNA) and rDNA modified Au NPs (rDNA–Au NPs) exhibit no inhibitory ability (data not shown). The ultra high anticoagulant potency of TBA29-P4Tn–Au NPs are a result of steric blocking effects and high binding affinity toward thrombin, and were two orders of magnitude higher than that of free TBA29.60 The length of the Tn-linker of TBA–Au NPs has a strong impact on their inhibitory ability while having little effect on that of free TBA. The little enhancement of free TBA15 with increasing Tn can be attributed to the increasing electrostatic interaction between thrombin and TBA15.51 The driving force of TBA and thrombin interaction is thought to be a combination of electrostatic interaction and molecular pattern recognition.38–40 The anticoagulation of TBA–Au NPs increased with an increase in the linker length from T0 to T30 and then started to decrease with a further increase in linker length. The increase in linker length will increase the flexibility and the stability of G-quadruplexes of TBAs (avoid formation of stretched, linear TBA structures) on the Au NP surfaces and therefore will improve the anticoagulant potency. Our reasoning of the phenomenon was supported by the fact that there was an increase in the hydrodynamic diameter with increasing linker length of TBA–Au NPs to T60 (Fig. 2a). When the linker length of TBA was longer than T30, there was a lowering of TBA density on Au NPs (Table 1) and possible formation of intermolecular G-quadruplexes of TBA, which resulted in the decrease of anticoagulation activity. The lower TBA density and formation of intermolecular G-quadruplexes were further supported by their electrophoretic mobility (Fig. 2c). The lower TBA density and/or formation of intermolecular G-quadruplexes both resulted in the decrease of surface charge of TBA–Au NPs and thus their decrease in the binding affinity to thrombin.
![The relative thrombin clotting time [(t − t0)/t0] in human plasma in the presence of TBA15-P0Tn, TBA29-P4Tn, TBA15-P0Tn–Au NPs or TBA29-P4Tn–Au NPs. The TCT is taken at the point where the scattering signal is half-way between the lowest and highest points. The TCT for human plasma in the absence and presence of inhibitors is represented by t0 and t, respectively. Coagulation was initiated by adding plasma to each thrombin sample, and then light scattering was monitored at 680 nm. The control sample contained only thrombin (5.0 nM) and plasma prepared in physiological buffer [25 mM Tris-HCl (pH 7.4), 150 mM NaCl, 5 mM KCl, 1 mM MgCl2, 1 mM CaCl2]. Error bars represent standard deviations from three repeated experiments.](/image/article/2012/RA/c1ra00344e/c1ra00344e-f1.gif) |
| Fig. 1 The relative thrombin clotting time [(t − t0)/t0] in human plasma in the presence of TBA15-P0Tn, TBA29-P4Tn, TBA15-P0Tn–Au NPs or TBA29-P4Tn–Au NPs. The TCT is taken at the point where the scattering signal is half-way between the lowest and highest points. The TCT for human plasma in the absence and presence of inhibitors is represented by t0 and t, respectively. Coagulation was initiated by adding plasma to each thrombin sample, and then light scattering was monitored at 680 nm. The control sample contained only thrombin (5.0 nM) and plasma prepared in physiological buffer [25 mM Tris-HCl (pH 7.4), 150 mM NaCl, 5 mM KCl, 1 mM MgCl2, 1 mM CaCl2]. Error bars represent standard deviations from three repeated experiments. | |
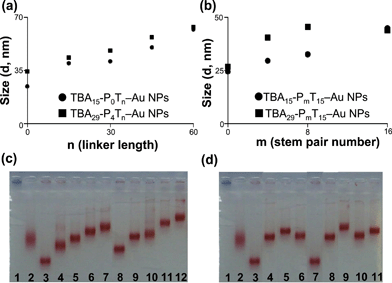 |
| Fig. 2 (a, b) Hydrodynamic diameters and (c, d) gel electrophoresis of various modified TBA-PmTn–Au NPs. Lanes in (c): (1) bare Au NPs, (2) BSA capping Au NPs, (3) TBA15-P0T0–Au NPs, (4) TBA15-P0T15–Au NPs, (5) TBA15-P0T30–Au NPs, (6) TBA15-P0T45–Au NPs, (7) TBA15-P0T60–Au NPs, (8) TBA29-P4T0–Au NPs, (9) TBA29-P4T15–Au NPs, (10) TBA29-P4T30–Au NPs, (11) TBA29-P4T45–Au NPs, (12) TBA29-P4T60–Au NPs. Lanes in (d): (1) bare Au NPs, (2) BSA capping Au NPs, (3) TBA15-P0T15–Au NPs, (4) TBA15-P4T15–Au NPs, (5) TBA15-P8T15–Au NPs, (6) TBA15-P16T15–Au NPs, (7) TBA29-P0T15–Au NPs, (8) TBA29-P4T15–Au NPs, (9) TBA29-P8T15–Au NPs, (10) TBA29-P16T15–Au NPs and (11) TBA15/TBA29-P8T15–Au NPs. (c, d) Gel electrophoresis of various modified Au NPs (50 nM) in a 1.5% agarose gel containing 0.5X TBE buffer with electric field of 20 V cm−1. Other conditions were the same as those described in Fig. 1. | |
3.2. Stem pair number of TBA-PmT15–Au NPs.
Fig. 3 displays the TCT delay in the presence of TBA15-PmT15 (100 nM), TBA29-PmT15 (100 nM), TBA15-P0T15–Au NPs (0.91 nM), TBA15-P4T15–Au NPs (1.11 nM), TBA15-P8T15–Au NPs (1.43 nM), TBA15-P16T15–Au NPs (1.82 nM), TBA29-P0T15–Au NPs (0.85 nM), TBA29-P4T15–Au NPs (1.14 nM), TBA29-P8T15–Au NPs (1.43 nM) or TBA29-P16T15–Au NPs (1.82 nM). The content of each of the oligonucleotides was held constant (100 nM) in these experiments. TBA15-PmT15 prolonging the stem pair number will stabilize the G-quadruplexes structure and increase the resistance of nuclease digestion in plasma and therefore increase their inhibitory potency. We noted the inhibition of TBA15-PmT15–Au NPs and TBA29-PmT15–Au NPs increased with increase in the stem pair number from P0 to P8 and then decreased. As a result of increase in the rigidity and the stability of G-quadruplexes of TBAs on the Au NP surfaces with increasing stem number, the anticoagulant potency increased. In addition, the increase of stem pair number leads to an increase in the distance of G-quadruplexes unit of TBA and thus increases their hydrodynamic diameter (Fig. 2b). The inhibitions of TBA15-P16T15–Au NPs/TBA29-P16T15–Au NPs (TCT delay 2.6/12.3 fold) were lower than TBA15-P8T15–Au NPs/TBA29-P8T15–Au NPs (TCT delay 4.2/18.2 fold), probably due to the relatively low TBA density (Table 1 and Fig. 2d) and unexpected intermolecular hybridization or G-quadruplexes. The electrophoretic mobility of TBA-P16T15–Au NPs was unexpectedly faster than TBA-h8T15–Au NPs.
![The relative thrombin clotting time [(t − t0)/t0] in human plasma in the presence of TBA-PmT15 or TBA-PmT15–Au NPs. The error bars represent the standard deviation of three repeated measurements. Other conditions were the same as those described in Fig. 1.](/image/article/2012/RA/c1ra00344e/c1ra00344e-f3.gif) |
| Fig. 3 The relative thrombin clotting time [(t − t0)/t0] in human plasma in the presence of TBA-PmT15 or TBA-PmT15–Au NPs. The error bars represent the standard deviation of three repeated measurements. Other conditions were the same as those described in Fig. 1. | |
3.3. Multivalent of TBA15/TBA29-P8T15–Au NPs
After optimization of linker length and stem pair number of TBA-PmTn–Au NPs, we further prepared multivalent Au NP bioconjugates (TBA15/TBA29-P8T15–Au NPs) to improve the inhibition of thrombin enzymatic activity. The TCT values for TBA15-P8T15 (100 nM), TBA15-P8T15–Au NPs (1.43 nM), TBA29-P8T15–Au NPs (1.43 nM) and TBA15/TBA29-P8T15–Au NPs (1.42 nM) are approximately 3.9, 4.2, 18.2 and 296 times longer than that would be in the absence of an inhibitor (Fig. 4). Our result reveals TBA15/TBA29-P8T15–Au NPs have an ultra strong potency on coagulation delay in this common pathway compared to TBA15 and monovalent TBA–Au NPs. We will emphasize that the TBA15-P0/TBA29-P4–Au NPs conjugates were used without modifying the sequence according to the reports,38–40 leading to only 19.3-times delay in TCT (data not shown). The multivalent interaction between the thrombin and TBA15/TBA29-P8T15–Au NPs induces interparticle cross-linking. The degree of aggregation of TBA15/TBA29-P8T15–Au NPs (1.0 nM) tends to increase with increasing thrombin concentration from 10 to 100 nM (ESI, Fig. S1†). Interaction of the TBA15/TBA29-P8T15–Au NPs with thrombin is strong: Kd = 8.86 × 10−12 M (ESI, Fig. S2†), two orders of magnitude higher than that for free TBA29 and one order of magnitude higher than that for a linear assembly of TBA15 and TBA29 and engineering dendritic aptamer.38–40,53–59 In addition, electrostatic interactions and steric blocking between the TBA15/TBA29-P8T15–Au NPs and thrombin are caused by the high density and negative charge of the aptamer units which cannot be excluded for stronger inhibitions. The stability of nucleic acids toward enzymes and nucleases is an important factor that affects their therapeutic and diagnostic applications. We investigated that the TCT delay for the TBA15/TBA29-P8T15–Au NPs remains almost constant after 48 h of incubation in the presence of human plasma (ESI, Fig. S3†). High local concentrations of salt in the highly negatively charged TBA15/TBA29-P8T15–Au NPs are known to cause enhanced resistance to nuclease digestion in plasma.79
![(a) Real-time monitoring of the light scattered during the coagulation of mixtures of thrombin, plasma and in the absence (A, control) and presence of inhibitors (B–E). (b) The relative thrombin clotting time [(t − t0)/t0] in human plasma in the presence of and in the absence (A, control) and presence of inhibitors (B–E). Other conditions were the same as those described in Fig. 1.](/image/article/2012/RA/c1ra00344e/c1ra00344e-f4.gif) |
| Fig. 4 (a) Real-time monitoring of the light scattered during the coagulation of mixtures of thrombin, plasma and in the absence (A, control) and presence of inhibitors (B–E). (b) The relative thrombin clotting time [(t − t0)/t0] in human plasma in the presence of and in the absence (A, control) and presence of inhibitors (B–E). Other conditions were the same as those described in Fig. 1. | |
We further compare our TBA15/TBA29-P8T15–Au NPs with commercial anticoagulant drugs of direct thrombin inhibitors (argatroban or hirudin) and our previous TBA29-P4T15–Au NPs in TCT assays (Fig. 5). The dosage dependence of TCT delay indicates the anticoagulation ability of TBA15/TBA29-P8T15–Au NPs was much better than the two commercial drugs and TBA29-P4T15–Au NPs. For example, TCT using TBA15/TBA29-P8T15–Au NPs (0.14 nM; [TBA] = 10 nM) was 961 ±11 s, which is much longer than that in the absence of the inhibitor (20 ±3 s), whereas the TCT time was prolonged to 58 ±4 s, 22± 3 s and 69± 4 s when using argatroban (10 nM), hirudin (10 nM) and TBA29-P4T15–Au NPs (0.11 nM; [TBA] = 10 nM), respectively.
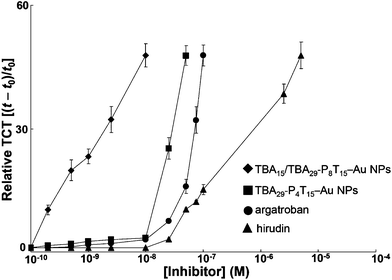 |
| Fig. 5 Dosage dependence of the delay of TCT for TBA15/TBA29-P8T15–Au NPs, TBA29-P4T15–Au NPs, argatroban or hirudin in the human plasma samples. The error bars represent the standard deviation of three repeated measurements. Other conditions were the same as those described in Fig. 1. | |
3.5. Reverse thrombin activity by irradiation of light
Reversibility of binding between inhibitors and coagulant factors is important for cardiopulmonary bypass.80–82 Thrombin is also important in the regulation of homeostasis and is associated with many types of cancer.83 Therefore, engineering probes that target thrombin and, moreover, whose function can be precisely regulated by photonic energy, can advance anticoagulation therapy for many diseases. Fig. 6 exhibits that, in the presence of TBA15/TBA29-P8T15–Au NPs and TBA15pc/TBA29pc-P8T15–Au NPs (both of them are 2.5 nM) inhibit the plasma clotting induced by thrombin (5 nM). The TBA15pc/TBA29pc-P8T15–Au NPs/thrombin complex irradiated with 365 nm UV light (365 nm, 20 mW cm−2, 30 min) can rapidly reverse the activity of thrombin. TBA15pc/TBA29pc-P8T15–Au NPs with near-UV light allowed the “caged” TBA15pc/TBA29pc units to be released from the Au NPs surface. We determined about 80% of the TBApc was released in to the solution after irradiation of near-UV light for 30 min. To minimize the damage caused by light to the animal tissue, for real animal system applications, we will use two photon irradiation in our future work.
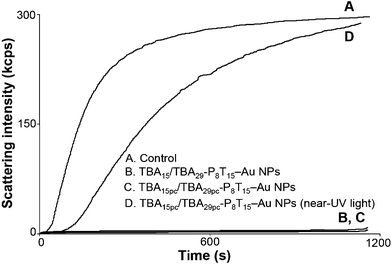 |
| Fig. 6 Reversible inhibitory function of the TBA15pc/TBA29pc-P8T15–Au NPs (2.5 nM) in plasma by irradiation of near-UV light. (A) thrombin (5 nM) is added to 2-fold diluted plasma immediately in the absence of any inhibitors, (B) 2.5 nM TBA15/TBA29-P8T15–Au NPs or (C) 2.5 nM TBA15pc/TBA29pc-P8T15–Au NPs incubated with thrombin and plasma, or (D) 2.5 nM TBA15pc/TBA29pc-P8T15–Au NPs incubated with thrombin were irradiated with near-UV light for 30 min and then reacted with plasma. Other conditions were the same as those described in Fig. 1. | |
4. Conclusions
We have demonstrated that TBA15/TBA29-P8T15–Au NPs display a high anticoagulant activity toward thrombin due to their highly flexible and stable conformation and multivalent binding. The TBA15/TBA29-P8T15–Au NPs interact strongly with thrombin, exhibiting a high binding affinity (Kd = 8.86 × 10−12 M) toward thrombin—over 100 times higher than that of monovalent TBA29, 10
000 times higher than that of TBA15, and at least 10 times higher than those that have been reported previously for fusion aptamers, dendritic aptamers, and TBA29–Au NPs.53–60 The TBA15/TBA29-P8T15–Au NPs exhibited a 296-fold longer TCT relative to that tested in the absence of any inhibitor, whereas for TBA15 alone, it was only 3.9 times longer. Moreover, the caged TBA15pc/TBA29pc-P8T15–Au NPs allow a reversal of thrombin activity via near-UV light irradiation. Thus, our newly developed light-controllable anticoagulant drug should exhibit high potencies in biomedical applications. We believe that our described techniques can be used widely to modify NPs with other DNA or RNA aptamers toward different proteins or cancer cells.
Acknowledgements
This study was supported by the National Science Council of Taiwan under contract NSC 99-2113-M-019-001-MY2 and the National Health Research Institutes Taiwan under contract NHRI-EX100-10047NI.
References
- G. Mayer, Angew. Chem., Int. Ed., 2009, 48, 2672 CrossRef CAS
.
- .S. Tombelli, M. Minunni and M. Mascini, Biomol. Eng., 2007, 24, 191 CrossRef
.
- T. Mairal, V. C. Özalp, P. L. Sánchez, M. Mir, I. Katakis and C. K. O'Sullivan, Anal. Bioanal. Chem., 2008, 390, 989 CrossRef CAS
.
- W. Mok and Y. Li, Sensors, 2008, 8, 7050 CrossRef CAS
.
- A. S. Barbas and R. R. White, Curr. Opin. Invest. Drugs, 2009, 10, 572 CAS
.
- Y. Du, B. Li and E. Wang, Bioanalytical Reviews, 2010, 1, 187 CrossRef
.
- M. Famulok, J. S. Hartig and G. Mayer, Chem. Rev., 2007, 107, 3715 CrossRef CAS
.
- A. D. Keefe, S. Pai and A. Ellington, Nat. Rev. Drug Discovery, 2010, 9, 537 CrossRef CAS
.
- S. D. Jayasena, Clin. Chem., 1999, 45, 1628 CAS
.
- J. H. Lee, M. V. Yigit, D. Mazumdar and Y. Lu, Adv. Drug Delivery Rev., 2010, 62, 592 CrossRef CAS
.
- A. B. Iliuk, L. Hu and W. A. Tao, Anal. Chem., 2011, 83, 4440 CrossRef CAS
.
- B. Soontornworajit and Y. Wang, Anal. Bioanal. Chem., 2011, 399, 1591 CrossRef CAS
.
- J. Zhou and J. J. Rossi, Oligonucleotides, 2011, 21, 1 CrossRef CAS
.
- A. S. Barbas, J. Mi, B. M. Clary and R. R. White, Future Oncol., 2010, 6, 1117 CrossRef CAS
.
- T.-C. Chiu and C.-C. Huang, Sensors, 2009, 9, 10356 CrossRef CAS
.
- K. Wang, M. You, Y. Chen, D. Han, Z. Zhu, J. Huang, K. Williams, C. J. Yang and W. Tan, Angew. Chem., Int. Ed., 2011, 50, 6098 CrossRef CAS
.
- J. Müller, T. Becher, J. Braunstein, P. Berdel, S. Gravius, F. Rohrbach, J. Oldenburg, G. Mayer and B. Pötzsch, Angew. Chem., Int. Ed., 2011, 50, 6075 CrossRef
.
- T. O. Pangburn, M. A. Petersen, B. Waybrant, M. M. Adil and E. Kokkoli, J. Biomech. Eng., 2009, 131, 074005-1 CrossRef
.
- Z. Wang and Y. Lu, J. Mater. Chem., 2009, 19, 1788 RSC
.
- X. Chen, Y.-F. Huang and W. Tan, J. Biomed. Nanotechnol., 2008, 4, 400 CrossRef CAS
.
- S. Balamurugan, A. Obubuafo, S. A. Soper and D. A. Spivak, Anal. Bioanal. Chem., 2008, 390, 1009 CrossRef CAS
.
- E. D. Cera, Mol. Aspects Med., 2008, 29, 203 CrossRef
.
- A. S. Wolberg, Blood Rev., 2007, 21, 131 CrossRef CAS
.
- L. G. Licari and J. P. Kovacic, Journal of Veterinary Emergency and Critical Care, 2009, 19, 11 CrossRef
.
- J. A. Huntington and J. Thromb, J. Thromb. Haemostasis, 2005, 3, 1861 CrossRef CAS
.
- J. A. Huntington and T. P. Baglin, Trends Pharmacol. Sci., 2003, 24, 589 CrossRef CAS
.
- J. W. Frederiksen, Ann. Thorac. Surg., 2000, 70, 1434 CrossRef CAS
.
- F. Szlam, D. Luan, D. Bolliger, A. D. Szlam, J. H. Levy, J. D. Varner and K. A. Tanaka, Thromb. Res., 2010, 125, 432 CrossRef CAS
.
- A. Schwienhorst, Cell. Mol. Life Sci., 2006, 63, 2773 CrossRef CAS
.
- B. Subramaniam and K. W. Park, Int. Anesthesiol. Clin., 2005, 43, 39 CrossRef
.
- J. Hirsh, M. O'Donnell and J. W. Eikelboom, Circulation, 2007, 116, 552 CrossRef CAS
.
- J. Hirsh, Thromb. Res., 2003, 109, S1 CrossRef CAS
.
- T. E. Warkentin and A. Greinacher, Ann. Thorac. Surg., 2003, 76, 2121 CrossRef
.
- S. Rangarajan, Haemophilia, 2011, 17, 90 CrossRef CAS
.
- P. Klement and J. Rak, Vnitr Lek., 2006, 52, 119 CAS
.
- S. M. Nimjee, C. P. Rusconi, R. A. Harrington and B. A. Sullenger, Trends Cardiovasc. Med., 2005, 15, 41 CrossRef CAS
.
- M. Famulok, Nat. Biotechnol., 2004, 22, 1373 CrossRef CAS
.
- L. C. Bock, L. C. Griffin, J. A. Latham, E. H. Vermaas and J. J. Toole, Nature, 1992, 355, 564 CrossRef CAS
.
- K. Padmanabhan, K. P. Padmanabhan, J. D. Ferrara, J. E. Sadler and A. Tulinsky, J. Biol. Chem., 1993, 268, 17651 CAS
.
- D. M. Tasset, M. F. Kubik and W. Steiner, J. Mol. Biol., 1997, 272, 688 CrossRef CAS
.
- S. M. Raviv, A. Horváth, J. Aradi, Z. Bagoly, F. Fazakas, Z. Batta, L. Muszbek and J. Hársfalvi, J. Thromb. Haemostasis, 2008, 6, 1764 CrossRef CAS
.
- A. Virno, A. Randazzo, C. Giancola, M. Bucci, G. Cirino and L. Mayol, Bioorg. Med. Chem., 2007, 15, 5710 CrossRef CAS
.
- A. Pasternak, F. J. Hernandez, L. M. Rasmussen, B. Vester and J. Wengel, Nucleic Acids Res., 2011, 39, 1155 CrossRef CAS
.
- C. G. Peng and M. J. Damha, Nucleic Acids Res., 2007, 35, 4977 CrossRef CAS
.
- S. R. Nallagatla, B. Heuberger, A. Haque and C. Switzer, J. Comb. Chem., 2009, 11, 364 CrossRef CAS
.
- B. Saccà, L. Lacroix and J.-L. Mergny, Nucleic Acids Res., 2005, 33, 1182 CrossRef
.
- M. Zaitseva, D. Kaluzhny, A. Shchyolkina, O. Borisova, I. Smirnov and G. Pozmogova, Biophys. Chem., 2010, 146, 1 CrossRef CAS
.
- L. Martino, A. Virno, A. Randazzo, A. Virgilio, V. Esposito, C. Giancola, M. Bucci, G. Cirino and L. Mayol, Nucleic Acids Res., 2006, 34, 6653 CrossRef CAS
.
- I. Smirnov and R. H. Shafer, Biochemistry, 2000, 39, 1462 CrossRef CAS
.
- G. Zhou, X. Huang and Y. Qu, Biochem. Eng. J., 2010, 52, 117 CrossRef CAS
.
- M. C. R. Buff, F. Schäfer, B. Wulffen, J. Müller, B. Pötzsch, A. Heckel and G. Mayer, Nucleic Acids Res., 2010, 38, 2111 CrossRef CAS
.
- H. Dougan, D. M. Lyster, C. V. Vo, A. Stafford, J. I. Weitz and J. B. Hobbs, Nucl. Med. Biol., 2000, 27, 289 CrossRef CAS
.
- Y. Kim, D. M. Dennis, T. Morey, L. Yang and W. Tan, Chem.–Asian J., 2010, 5, 56 CrossRef CAS
.
- Y. Kim, Z. Cao and W. Tan, Proc. Natl. Acad. Sci. U. S. A., 2008, 105, 5664 CrossRef CAS
.
- J. Müller, D. Freitag, G. Mayer and B. Pötzsch, J. Thromb. Haemostasis, 2008, 6, 2105 CrossRef
.
- J. Müller, B. Wulffen, B. Pötzsch and G. Mayer, ChemBioChem, 2007, 8, 2223 CrossRef
.
- H. Hasegawa, K. Taira, K. Sode and K. Ikebukuro, Sensors, 2008, 8, 1090 CrossRef CAS
.
- G. Mayer, J. Müller, T. Mack, D. F. Freitag, T. Höver, B. Pötzsch and A. Heckel, ChemBioChem, 2009, 10, 654 CrossRef CAS
.
- B. L. Henry, B. H. Monien, P. E. Bock and U. R. Desai, J. Biol. Chem., 2007, 282, 31891 CrossRef CAS
.
- Y.-C. Shiang, C.-C. Huang, T.-H. Wang, C.-W. Chien and H.-T. Chang, Adv. Funct. Mater., 2010, 20, 3175 CrossRef CAS
.
- Y.-C. Shiang, C.-L. Hsu, C.-C. Huang and H.-T. Chang, Angew. Chem., Int. Ed., 2011, 50, 7660 CrossRef CAS
.
- C.-L. Hsu, H.-T. Chang, C.-T. Chen, S.-C. Wei, Y.-C. Shiang and C.-C. Huang, Chem.-Eur. J., 2011, 17, 10994 CrossRef CAS
.
- L. Tian and T. Heyduk, Biochemistry, 2009, 48, 264 CrossRef CAS
.
- H. Saneyoshi, S. Mazzini, A. Aviñό, G. Portella, C. González, M. Orozco, V. E. Marquez and R. Eritja, Nucleic Acids Res., 2009, 37, 5589 CrossRef CAS
.
- B. Pagano, L. Martino, A. Randazzo and C. Giancola, Biophys. J., 2008, 94, 562 CrossRef CAS
.
- B. V. Enüstün and J. Turkevich, J. Am. Chem. Soc., 1963, 85, 3317 CrossRef
.
- R. C. Mucic, J. J. Storhoff, C. A. Mirkin and R. L. Letsinger, J. Am. Chem. Soc., 1998, 120, 12674 CrossRef CAS
.
- S. Link and M. A. El-Sayed, J. Phys. Chem. B, 1999, 103, 8410 CrossRef CAS
.
- J. J. Storhoff, R. Elghanian, R. C. Mucic, C. A. Mirkin and R. L. Letsinger, J. Am. Chem. Soc., 1998, 120, 1959 CrossRef CAS
.
- S. J. Hurst, A. K. R. Lytton-Jean and C. A. Mirkin, Anal. Chem., 2006, 78, 8313 CrossRef CAS
.
- The Handbook—A Guide to Fluorescent Probes and Labeling Technologies, http://www.probes.com/handbook/sections/0803.html..
- C.-K. Chen, C.-C. Huang and H.-T. Chang, Biosens. Bioelectron., 2010, 25, 1922 CrossRef CAS
.
- S. H. Brewer, W. R. Glomm, M. C. Johnson, M. K. Knag and S. Franzen, Langmuir, 2005, 21, 9303 CrossRef CAS
.
- X. Bai, Z. Li, S. Jockusch, N. J. Turro and J. Ju, Proc. Natl. Acad. Sci. U. S. A., 2003, 100, 409 CrossRef CAS
.
- J. Olejnik, S. Sonar, E. Krzymañska-Olejnik and K. J. Rothschild, Proc. Natl. Acad. Sci. U. S. A., 1995, 92, 7590 CrossRef CAS
.
- G. Y. Shinowara and L. Rosenfeld, J. Lab. Clin. Med., 1951, 37, 303 CAS
.
- J. A. Penner, Am. J. Clin. Path., 1974, 61, 645 CAS
.
- J. A. Ginsberg, M. A. Crowther, R. H. White and T. L. Ortel, Hematology, 2001, 2001, 339 CrossRef
.
- D. S. Seferos, A. E. Prigodich, D. A. Giljohann, P. C. Patel and C. A. Mirkin, Nano Lett., 2009, 9, 308 CrossRef CAS
.
- S. M. Nimjee, J. R. Keys, G. A. Pitoc, G. Quick, C. P. Rusconi and B. A. Sullenger, Mol. Ther., 2006, 14, 408 CrossRef CAS
.
- Y. Kim, J. A. Phillips, H. Liu, H. Kang and W. Tan, Proc. Natl. Acad. Sci. U. S. A., 2009, 106, 6489 CrossRef CAS
.
- A. Heckel, M. C. R. Buff, M.-S. L. Raddatz, J. Müller, B. Pötzsch and G. Mayer, Angew. Chem., Int. Ed., 2006, 45, 6748 CrossRef CAS
.
- L. R. Zacharski, Cancer Lett., 2002, 186, 1 CrossRef CAS
.
Footnote |
† Electronic Supplementary Information (ESI) available: Fig. S1–S3. See DOI: 10.1039/c1ra00344e/ |
|
This journal is © The Royal Society of Chemistry 2012 |