DOI:
10.1039/C2NR32505E
(Paper)
Nanoscale, 2013,
5, 275-283
Carrier-free nanoassemblies of a novel oxazolidinone compound FYL-67 display antimicrobial activity on methicillin-resistant Staphylococcus aureus
Received
30th August 2012
, Accepted 22nd October 2012
First published on 25th October 2012
Abstract
In this work, a novel oxazolidinone compound FYL-67 was synthesized, and the obtained FYL-67 could form nanoassemblies in aqueous solution by a self-assembly method without using any carrier, organic solvent, or surfactant. The prepared FYL-67 nanoassemblies had a particle size of 264.6 ± 4.3 nm. The FYL-67 nanoassemblies can be lyophilized into a powder form without any cryoprotector or excipient, and the re-dissolved FYL-67 nanoassemblies are stable and homogeneous. The in vitro release profile showed a significant difference between rapid release of free FYL-67 and much slower and sustained release of FYL-67 nanoassemblies. In vitro susceptibility tests were conducted in three strains of methicillin-susceptible Staphylococcus aureus (MSSA) and three strains of methicillin-resistant Staphylococcus aureus (MRSA), using linezolid as a positive control. FYL-67 nanoassemblies exhibited excellent in vitro activity, with a minimum inhibitory concentration (MIC) value of 0.5 μg mL−1 against MRSA. In the in vitro post-antibiotic effect (PAE) evaluation, FYL-67 nanoassemblies showed a more powerful effect than linezolid. Besides, in vitro cytotoxicity tests indicated that FYL-67 nanoassemblies had a very low cytotoxicity on HEK293 cells and L02 cells. Furthermore, in both MSSA and MRSA systemic infection mouse models, FYL-67 nanoassemblies showed a lower ED50 than linezolid. In a murine model of MRSA systemic infection, FYL-67 nanoassemblies displayed an ED50 of less than 4.0 mg kg−1, which is 2.3-fold better than that of linezolid. Our findings suggested that the FYL-67 nanoassemblies may be a potential drug candidate in MRSA therapy.
1. Introduction
Antimicrobial resistance in a whole range of bacteria, coupled with the failure of the pharmaceutical industry to develop innovative agents, is currently among the top global health challenges.1 In particular, infections caused by resistant Gram-positive cocci like methicillin-resistant Staphylococcus aureus (MRSA), penicillin-resistant Streptococcus pneumoniae (PRSP), and vancomycin-resistant Enterococcus faecalis (VRE) have become a major cause for concern in hospitals. Several new powerful compounds that are active against Gram-positive cocci have been made available in the last few years. For example, linezolid, the first oxazolidinone drug, was approved in 2000 by the Food and Drug Administration (FDA) for the treatment of multidrug resistant, Gram-positive bacterial infections.2 But, along with emerging clinical reports on linezolid-resistant strain infections of Staphylococcus aureus and Enterococcus faecium,3–5 there is evidence showing some of these toxicities such as adverse hematological effects, inhibition of mitochondrial protein synthesis and potential for result in serotonin syndrome.6 Thus, further studies focusing on novel drugs with improved potency and broad antibacterial spectrum are still urgent.
Solubility of drugs is a critical factor in drug research and development, which greatly affects the development of their clinical formulations.7,8 Approximately half of new active compounds have failed to be developed owing to their great hydrophobicity.9,10 As a fast developing field, nanotechnology gains increasing attention in drug delivery systems (DDSs), which provides an important method to overcome this problem of hydrophobic drugs.11,12 After a hydrophobic drug is rendered into nanoscale particles, it can be well dispersed in aqueous solution to form a stable and homogeneous solution for clinical administration.13,14 Furthermore, the nanoscale particles of drugs prolong their circulation time in vivo, which may improve its therapeutic effects.15–17 In recent years, a variety of materials have been investigated as carriers for nano-formulation of drugs and they have shown promising potential, including liposomes, synthetic polymers, natural polymers, and inorganic materials, however, few have penetrated into clinical practices because of safety, stability, and other problems.18–22 Therefore, novel methods or DDSs are still desired for developing nano-formulations of hydrophobic drugs.
In the present work, we aimed to synthesize a novel oxazolidinone compound FYL-67, and manipulated it into nanoassemblies by a self-assembly method without using any carrier, organic solvent, or surfactant. Besides, the characteristics of FYL-67 nanoassemblies were investigated in detail as a potential drug formulation, including particle size distribution, zeta potential, morphology, and in vitro release behavior. Furthermore, the in vitro susceptibility and the post-antibiotic effect (PAE) of FYL-67 nanoassemblies were investigated using methicillin-susceptible Staphylococcus aureus (MSSA) and MRSA. In addition, cytotoxicity tests were conducted using normal human cell lines. Subsequently, in vivo bactericidal activity tests were performed using a mouse systemic infection model. Our results indicated that the prepared FYL-67 nanoassemblies may have great potential application for MRSA therapy in clinic.
2. Materials and methods
2.1. Materials, cell lines, bacterial strains, and experimental animals
Linezolid (>99%, research compound; Airsea pharmaceutical Ltd, Taizhou, China), 3-(4,5-dimethyl-2-thiazolyl)-2,5-diphenyl-2H-tetrazolium bromide (methyl thiazolyl tetrazolium, MTT, Sigma, USA), and methanol (HPLC grade, Fisher Scientific, UK) were used without further purification. All the reagents used in this work were of analytic reagent (AR) grade and used as received.
Two strains of MRSA (ATCC 33591 and ATCC 43300), three isolates of MRSA (MSAU 0016, 09L075 and 09N120), four strains of MSSA (ATCC 29213, ATCC 25923, ATCC 19636, and ATCC 6538), and two isolates of MSSA (09U035 and 09O077) were used in the present studies. MRSA and MSSA strains were obtained from the American Type Culture Collection (Manassas, Va., USA), and MRSA and MSSA isolates were obtained from hospitals or academic institutions. Mueller-Hinton (MH) broth (Qingdao Hopo Biol-technolog Co., Ltd. China) supplemented with calcium (25 mg L−1) and magnesium (12.5 mg L−1) was used for susceptibility tests and killing curve experiments. Colony counts were determined with MH agar plates (Qingdao Hopo).
HEK293 cells were purchased from the ATCC and were grown in Dulbecco's modified Eagle's medium (DMEM, Gibco, USA) supplemented with 10% fetal bovine serum (FBS, Gibco, USA). The normal human hepatocyte cell line L02 was purchased from the Cell Bank of the Chinese Academy of Sciences (Shanghai, China) and grown in Roswell Park Memorial Institute 1640 medium (RPMI 1640, Gibco, USA) supplemented with 10% fetal bovine serum (FBS, Gibco, USA). The cell cultures were maintained in a 37 °C incubator with a humidified 5% CO2 atmosphere.
C57BL/6 mice, weighing 20–22 g, were used for in vivo bactericidal activity tests. Animals were purchased from the Laboratory Animal Center of Sichuan University. Animals were housed at a controlled temperature of 20–22 °C, relative humidity of 50–60% and 12 h light–dark cycles. Animals were provided with standard laboratory chow and tap water ad libitum. All the animals would be in quarantine for a week before treatment. All animal procedures were performed following the protocol approved by the Institutional Animal Care and Treatment Committee of Sichuan University (Chengdu, P.R. China). All mice were treated humanely throughout the experimental period.
2.2. Synthesis and characterization of FYL-67
FYL-67 was synthesized according to Fig. 1, and the detailed procedures are as follows.
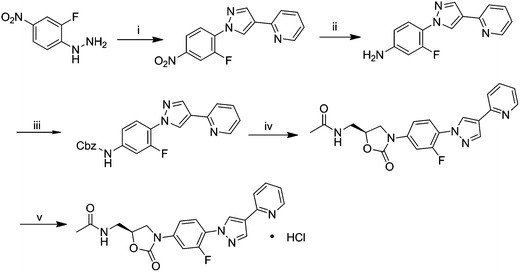 |
| Fig. 1 Synthetic route of the novel compound FYL-67. (i) 2-(pyridin-2-yl)malonaldehyde, p-TsOH (cat.), ethanol, reflux, 2 h; (ii) Fe, HCl, 95% ethanol, 1 h; (iii) Cbz–Cl, K2CO3, CH2Cl2, 2 h; (iv) (S)-1-acetamido-3-chloropropan-2-yl acetate, LiOt-Bu, THF, r.t.; (v) HCL (g), acetone, ethyl ether. | |
2.2.1. Synthesis of 2-(1-(2-fluoro-4-nitrophenyl)-1H-pyrazol-4-yl)pyridine.
The mixture of (2-fluoro-4-nitrophenyl)hydrazine (700 mg) and 2-(pyridin-2-yl) malonaldehyde (610 mg) dissolved in anhydrous ethanol (30 mL) was put in a single-neck flask. Then 4-methylbenzensulphonic acid (70 mg) was added with magnetic stirring. The above resultant was heated at reflux for 2 hours and then cooled to room temperature. The brown precipitate was filtered out and dried in a vacuum to obtain the title compound (993 mg) with a yield of 85.4%. 1H-NMR (400 MHz, DMSO-d6): δ 9.09 (s, 1H), 8.69 (d, J = 5.2 Hz, 1H), 8.62 (s, 1H), 8.50 (dd, J = 11.6 Hz, J = 2.4 Hz), 8.30–8.21 (m, 2H), 7.50–7.46 (m, 2H), 7.11 (d, J = 8.8 Hz, 1H).
2.2.2. Synthesis of 3-fluoro-4-(4-(pyridin-2-yl)-1H-pyrazol-1-yl)aniline.
2-(1-(2-Fluoro-4-nitrophenyl)-1H-pyrazol-4-yl)pyridine (900 mg) was dissolved in 95% ethanol (25 mL). The clear solution was heated to reflux and 4 N HCl (0.66 mL) was added in one portion. Then Fe powder (886.5 mg) was added gradually in 15 minutes. The reaction was monitored by thin liquid chromatography. After complete transformation of the starting material, the reactant was buffered to pH 10 with saturated sodium carbonate solution in an ice bath. The thus obtained dark slurry was filtered by suction. The filtering cake was washed using ethyl acetate (10 mL) three times. The combined organic phase was stripped off to afford the light brown title compound (540 mg) in a yield of 67.2%. 1H-NMR (400 MHz, DMSO-d6): δ 9.89 (s, 2H), 9.48 (s, 1H), 8.88 (s, 1H), 8.73 (d, J = 6 Hz, 1H), 8.58–8.54 (m, 1H), 8.46 (d, J = 8.4 Hz, 1H), 7.84 (t, J = 6.8 Hz, 1H), 7.66 (t, J = 8.4 Hz, 1H), 7.04 (d, J = 12.8 Hz, 1H), 6.95 (d, J = 8.8 Hz, 1H).
2.2.3. Synthesis of benzyl(3-fluoro-4-(4-(pyridin-2-yl)-1H-pyrazol-1-yl)phenyl) carbamate.
3-Fluoro-4-(4-(pyridin-2-yl)-1H-pyrazol-1-yl)aniline (200 mg) was dissolved in dichloromethane (5 mL) and potassium carbonate (194.3 mg) was then added in one portion with magnetic stirring. The mixture was cooled in an ice-bath and benzyl carbonochloridate (0.99 mL) was added drop by drop for 30 minutes. The ice-bath was removed and the reactant was stirred at room temperature for 2 hours. Then the mixture was poured in the separation funnel and 10 mL of distilled water was added. The dichloromethane layer was separated. The water layer was extracted twice more with dichloromethane (5 mL). The combined organic layer was stripped off to afford the title compound (260 mg) with a yield of 95.1%. 1H-NMR (400 MHz, CDCl3): δ 8.60 (d, J = 4.8 Hz, 1H), 8.48 (d, J = 2 Hz, 1H), 8.21 (s, 1H), 7.82 (t, J = 8.8 Hz, 1H), 7.72–7.68 (m, 1H), 7.64 (d, J = 12.8 Hz, 1H), 7.54 (d, J = 8 Hz, 1H), 7.43–7.36 (m, 4H), 7.17–7.14 (m, 1H), 7.09 (dd, J = 8.8 Hz, J = 1.2 Hz, 1H), 6.86 (s, 1H), 5.23 (s, 1H).
2.2.4. Synthesis of (S)-N-((3-(3-fluoro-4-(4-(pyridin-2-yl)-1H-pyrazol-1-yl) phenyl)-2-oxooxazolidin-5-yl)methyl)acetamide.
Benzyl(3-fluoro-4-(4-(pyridin-2-yl)-1H-pyrazol-1-yl)phenyl) carbamate (150 mg) was dissolved in absolute tetrahydrofuran under a nitrogen atmosphere in an ice bath. After stirring for 5 minutes, (S)-1-acetamido-3-chloropropan-2-yl acetate (149.9 mg) was added. The reactant was stirred at room temperature for another 36 hours. Then a mixture of dichloromethane (10 mL), distilled water (10 mL) and glacial acetic acid (0.022 mL) was added in order. The dichloromethane phase was collected using a separation funnel. The water phase was extracted with dichloromethane (10 mL) for another 2 times. The organic layer was combined and dried with anhydrous sodium sulfate. After removal of the solvent, the residue was purified by flash chromatography and the title compound (58 mg) was obtained in a yield of 38.2%.
1H-NMR (400 MHz, CDCl3): δ 8.61 (d, J = 4 Hz, 1H), 8.52 (d, J = 6.8 Hz, 2.4H), 8.22 (s, 1H), 7.94 (t, J = 8.8 Hz, 1H), 7.77–7.69 (m, 2H), 7.55 (d, J = 8 Hz, 1H), 7.27–7.26 (m, 1H), 7.18–7.15 (m, 1H), 6.06 (t, J = 6 Hz, 1H), 4.86–4.80 (m, 1H), 4.11 (t, J = 9.2 Hz, 1H), 3.86–3.82 (m, 1H), 3.78–3.62 (m, 2H), 2.04 (s, 3H).
13C-NMR (DMSO-d6): δ 170.51, 154.47, 152.94, 151.26, 149.94, 139.70, 139.15, 137.43, 129.96, 125.61, 125.19, 123.42, 122.19, 120.38, 114.52, 106.68, 72.29, 47.70, 41.84, 22.91. ESI-MS m/z 418.08 (M + Na+).
2.2.5. Prepration of FYL-67.
25 mg of (S)-N-((3-(3-fluoro-4-(4-(pyridin-2-yl)-1H-pyrazol-1-yl) phenyl)-2-oxooxazolidin-5-yl)methyl)acetamide was put in a 25 mL round-bottom flask, and 10 mL of acetone was then added. After stirring for 5 minutes, the mixture turned transparent. Ethyl ether saturated with anhydrous hydrogen chloride was dropped in, and a white precipitate appeared. The collected yellowish powder was dried in a vacuum and 24.1 mg of powder was obtained with a yield of 88.3%.
1H-NMR (400 MHz, DMSO-d6) δ: 9.33 (s, 1H), 8.80 (s, 1H), 8.74 (d, J = 5.6 Hz, 1H), 8.45 (t, J = 7.2 Hz, 1H), 8.38–8.31 (m, 2H), 7.90 (t, J = 8.8 Hz, 1H), 7.81 (dd, J = 2.4 Hz, J = 16.4 Hz, 1H), 7.76 (t, J = 6.0 Hz, 1H); 7.55 (dd, J = 1.6 Hz, J = 8.8 Hz, 1H), 4.83–4.76 (m, 1H), 4.60 (br s, 1H), 4.20 (t, J = 8.8 Hz, 1H), 3.91–3.82 (m, 1H), 3.45 (t, J = 5.2 Hz, 2H), 1.85 (s, 3H); 13C-NMR (DMSO-d6) δ: 170.51, 154.47, 152.94, 151.26, 149.94, 139.70, 139.15, 137.43, 129.96, 125.61, 125.19, 123.42, 122.19, 120.38, 114.52, 106.68, 72.29, 47.70, 41.84, 22.91; HR-MS(TOF) m/z calcd for C20H18FN5O3 [M + Cl−]: 430.1082, found: 430.1085; for C20H18FN5O3 [M + H+]: 396.1472, found: 396.1472.
2.3. Preparation and characterization of FYL-67 nanoassemblies
FYL-67 nanoassemblies were prepared by a self-assembly method. Briefly, 10 mg of FYL-67 was directly added into 1 mL normal saline (NS) at room temperature. Due to the amphiphilic properties, FYL-67 was self-assembled into nanoparticles without using any carrier, organic solvent, or surfactant. The prepared FYL-67 nanoassemblies were lyophilized into a powder form for further applications.
The particle size distribution and zeta potential of prepared FYL-67 nanoassemblies were determined by a Malvern Nano-ZS 90 laser particle size analyzer after equilibration for 10 min. All results were the mean of three test runs, and all data were expressed as the mean ± SD.
The morphological characteristics of the FYL-67 nanoassemblies were examined by a transmission electron microscope (TEM, H-6009IV, Hitachi, Japan). The FYL-67 nanoassemblies were diluted with distilled water and placed on a copper grid covered with nitrocellulose. The sample was negatively stained with phosphotungstic acid and dried at room temperature.
The concentration of FYL-67 was determined by a high performance liquid chromatography (HPLC) instrument (Waters Alliance 2695, Waters, Milford, MA, USA) and the sample was diluted before measurement. A solvent delivery system equipped with a column heater and a plus autosampler was used. Detection was carried out on a Waters 2996 detector. Chromatographic separations were performed on a reversed phase C18 column (4.6 × 150 mm to 5 μm, Chrosil). The column temperature was kept at 35 °C. Methanol/water (85/15, v/v) was used as an eluent at a flow rate of 1 mL min−1. The detection wavelength was 290 nm.
2.4.
In vitro release behavior of FYL-67 nanoassemblies
A modified dialysis method was employed to investigate the in vitro release behavior of FYL-67 nanoassemblies. Briefly, 500 μL of free FYL-67 or FYL-67 nanoparticles were placed in dialysis bags (the molecular mass cutoff is 3.0 kDa and the dialysis area is 1 cm2). The dialysis bags were incubated in 10 mL of PBS (pre-warmed to 37 °C, pH = 7.4) containing Tween 80 (0.5 wt%) at 37 °C with gentle shaking (100 rpm). At specific time points, all the release media were removed and replaced by pre-warmed fresh release media. After centrifugation, the supernatant of the removed release media was collected and stored at −20 °C until analysis. The released FYL-67 samples were quantified using HPLC. All results were the mean of three test runs, and all data were expressed as the mean ± SD.
2.5.
In vitro susceptibility test
The minimum inhibitory concentrations of the FYL-67 nanoassemblies against Gram-positive bacteria were tested using linezolid as a positive control. Minimum inhibitory concentration (MIC) values were determined using an agar dilution method according to the methods of National Committee for Clinical Laboratory Standards (NCCLS M7-M4, 4th ed.). A stock solution of FYL-67 nanoassemblies was prepared, which had a concentration of 320 μg mL−1. Serial 2-fold dilutions were prepared from the stock solution with sterile water and then 10-fold diluted with Mueller-Hinton (MH) agar medium to provide concentration in the range of 64–0.03125 μg mL−1. The tested organisms were grown in MH broth medium at 35 °C for 8 hours and were adjusted to the turbidity of the 0.5 McFarland standard. The bacterial suspensions were inoculated onto the drug-supplemented MH agar plates with a multipoint inoculator and incubated at 35 °C for 18 hours. The minimum bactericidal concentration (MBC), as defined by 99.9% reduction in viable bacteria relative to the initial inoculum, was determined by the method of broth dilution studies.23
2.6.
In vitro determination of the post-antibiotic effect (PAE)
In vitro PAEs were determined by the viable plate count method using Mueller-Hinton broth. 106 cfu mL−1 of logarithmic phase organisms were exposed for 1 h at 37 °C to four concentrations of linezolid or FYL-67 nanoassemblies (0.5×, 1×, 2×, 4× MIC). Then exposed bacteria were washed twice with phosphate-buffered saline (pH = 7.2) by centrifugation for 10 min at 2000g; controls were handled similarly. After drug removal, viable counts were plated hourly until visible regrowth had occurred. The following controls were included for each experiment: (i) a growth control, prepared and treated identically to the test solution but without exposure to antibiotic; and (ii) a residual antibiotic control, to which 1/1000 of the test antimicrobial concentration was added after centrifugation and washing. This last tube was included to ensure that, after centrifugation and washing, the residual drug in the tubes containing the treated organism did not affect the rate of growth.
The PAE was defined according to Craig & Gudmundsson as PAE = T – C, where T is the time required for the count of cfu in the test culture to increase 1 log 10 above the count observed immediately after drug removal, and C is the time required for the count of cfu in an untreated control culture to increase 1 log 10 above the count observed immediately after completion of the same procedure used on the test culture for drug removal.24
2.7.
In vitro cytotoxicity test
Cytotoxicity tests of FYL-67 nanoassemblies were performed on HEK293 cells and L02 cells. Cells were plated at a density of 1 × 104 cells per well in 100 μL of medium in 96-well plates and grown for 48 hours. The cells were then exposed to FYL-67 nanoassemblies for 48 hours. Then, viability of cells was measured using the MTT method. Briefly, the mean percentage of cell survival relative to that of untreated cells was estimated from data of six individual experiments, and all data were expressed as the mean ± SD. In cytotoxicity analysis, twenty percent inhibitory concentrations (IC20s) were determined graphically for every experiment, and mean values were calculated for the tabulation.
2.8.
In vivo bactericidal activity test
In vivo bactericidal activity of FYL-67 nanoassemblies was studied in a mouse systemic infection model, with linezolid as a control. Mice weighing 20–22 g were used in the study, with 10 mice in each group. A lethal systemic Staphylococcus aureus infection was given to the mice by the injection of 0.5 mL of an inoculum of Staphylococcus aureus 107 to 108 CFU mL−1via an intraperitoneal injection. FYL-67 nanoassemblies or linezolid were intravenously administered 1 h after infection. The ED50 was calculated 48 h after treatment by the method of Reed–Muench25 and using the Hill equation (GraphPad Prism 5.0; GraphPad Software, Inc., San Diego, CA, USA).
2.9.
In vivo toxicity tests
To evaluate the possible side effects in FYL-67 nanoassembly-treated mice, all the animals were observed after administration of FYL-67 nanoassemblies, including the general conditions (the activity, energy, hair, feces, behavior pattern, and other clinical signs), body weight, and mortality. After sacrificing, various organs (heart, liver, spleen, lung, kidney, stomach, and intestine) were harvested and fixed in 4% paraformaldehyde in PBS. These tissues were sectioned, stained with haematoxylin–eosin (H&E), and observed by two pathologists in a blinded manner.
2.10. Statistical analysis
The statistic analysis was carried out using SPSS 15.0 software (Chicago, IL, USA). Comparisons of data were performed using one-way analysis of variance (ANOVA). A P value <0.05 on a 2-tailed test was considered statistically significant.
3. Results
3.1. Characterization of FYL-67 nanoassemblies
FYL-67 nanoparticles were prepared by a one-step self-assembly method without using any carrier, organic solvent, or surfactant, which is easy to produce and scale up. The average particle size of the prepared FLY-67 nanoparticles was 264.6 ± 4.3 nm with a polydisperse index (PDI) of 0.228 ± 0.016, and the zeta potential was 17.3 ± 1.8 mV (Fig. 2A and B). According to the particle size distribution spectrum shown in Fig. 2A, the FYL-67 nanoparticles had a narrow particle size distribution. Furthermore, because the FYL-67 nanoassemblies were a carrier-free system, drug loading (DL) and encapsulation efficiency (EE) of the obtained FYL-67 nanoassemblies were both 100%. The TEM image of FYL-67 nanoparticles is presented in Fig. 3A, which shows that FYL-67 nanoassemblies were of spherical shape in aqueous solution. The diameter of the FYL-67 nanoassemblies observed by TEM was in good agreement with the results of particle size analysis, which demonstrated that the prepared FYL-67 nanoparticles are stable and could be well-dispersed in aqueous solution. The appearance of prepared FYL-67 nanoparticles is presented in Fig. 3B. The clear solution of FYL-67 nanoassemblies could be observed (Fig. 3B, b). Besides, the FYL-67 nanoassemblies can be lyophilized into a powder form without any cryoprotector or excipient (Fig. 3B, c), and the re-dissolved FYL-67 nanoassemblies are stable and homogeneous (Fig. 3B, d).
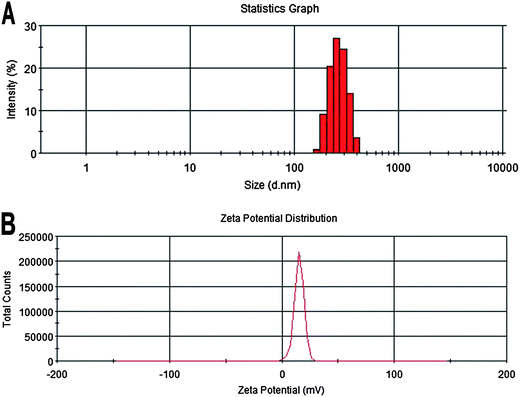 |
| Fig. 2 Particle size distribution (A) and zeta potential (B) of FYL-67 nanoassemblies. | |
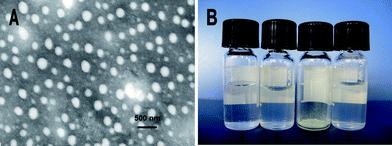 |
| Fig. 3 Characterization of FYL-67 nanoassemblies. (A): TEM image of FYL-67 nanoassemblies; (B): morphology of water (a), FYL-67 nanoassemblies (b), freeze-dried powder of FYL-67 nanoassemblies (c); and re-dispersed freeze-dried FYL-67 nanoassemblies (d). | |
3.2.
In vitro release behavior of FYL-67 nanoassemblies
In vitro release behavior of FYL-67 nanoassemblies was investigated using a dialysis method. Fig. 4 presents the in vitro release profiles of free FYL-67 and FYL-67 nanoassemblies. Compared with the release profile of free FYL-67, the cumulative release rate of FYL-67 nanoassemblies is much slower followed by a sustained release for more than one week. In the first 24 hours, 72.37 ± 5.78% of FYL-67 released from free FYL-67, whereas only 28.47 ± 0.57% of FYL-67 released from FYL-67 nanoassemblies into the outside media. At day 7 (168 hours), the cumulative release rate of free FYL-67 or FYL-67 nanoassemblies is 82.43 ± 4.53% or 55.85 ± 2.70%, respectively. A typical two-phase-release profile was observed in the FYL-67 nanoassemblies group, which showed a relatively rapid release in the first stage followed by a sustained and slow release over a prolonged time up to several weeks (Fig. 4).
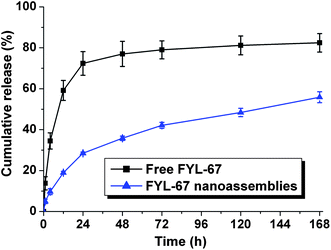 |
| Fig. 4
In vitro release behaviors of free FYL-67 and FYL-67 nanoassemblies. Error bars represent the standard deviation (n = 3). | |
3.3.
In vitro susceptibility tests
The in vitro activities of FYL-67 nanoassemblies were evaluated in MSSA (ATCC 29213, ATCC 25923, and ATCC 19636) and MRSA (ATCC 33591, ATCC 43300, and MSAU 0016), and the results are shown in Table 1. MIC values of FYL-67 nanoassemblies were 0.5–1 μg mL−1 or 0.5 μg mL−1 in MSSA or MRSA, respectively, whereas MIC values in the linezolid group were 1–2 μg mL−1 or 2 μg mL−1 in MSSA or MRSA, respectively. From Table 1, we found that FYL-67 nanoassemblies exhibited more potent activity against both MSSA and MRSA than linezolid (except in MSSA ATCC 29213, in which FYL-67 nanoassemblies showed an equal activity with linezolid).
Table 1
In vitro antimicrobial activity of FYL-67 nanoassemblies against MRSA and MSSA
Organism |
Strains |
MIC (μg mL−1) |
FYL-67 |
Linezolid |
MSSA |
ATCC 29213 |
1 |
1 |
ATCC 25923 |
1 |
2 |
ATCC 19636 |
0.5 |
1 |
MRSA |
ATCC 33591 |
0.5 |
2 |
ATCC 43300 |
0.5 |
2 |
MSAU 0016 |
0.5 |
2 |
Furthermore, MBC and MIC values of FYL-67 nanoassemblies in MSSA (ATCC 25923, ATCC 6538, 09U035, 09O077) and MRSA (09L075, 09N120) were determined. As shown in Table 2, the MBC/MIC ratio of FYL-67 nanoassemblies is within two dilutions of the MIC (ranging from 1 to 2) in both MSSA and MRSA, suggesting that FYL-67 nanoassemblies may be considered to be “bactericidal”.
Table 2 MBC of FYL-67 nanoassemblies for MSSA and MRSA
Organism |
Strains |
MIC (μg mL−1) |
MBC (μg mL−1) |
MBC/MIC |
MSSA |
ATCC 25923 |
0.5 |
0.5 |
1 |
MSSA |
ATCC 6538 |
0.5 |
0.5 |
1 |
MSSA |
09U035 |
1 |
1 |
1 |
MSSA |
09O077 |
2 |
4 |
2 |
MRSA |
09L075 |
1 |
2 |
2 |
MRSA |
09N120 |
2 |
4 |
2 |
3.4.
In vitro PAEs of FYL-67 nanoassemblies
In vitro PAEs of FYL-67 nanoassemblies were determined using ATCC 25923 and ATCC 6538, and linezolid was used as a positive control. The results are summarized in Table 3. PAEs of FYL-67 nanoassemblies were between 1 and 3.6 h for ATCC 25923, and between 0 and 4 h for ATCC 6538, whereas those of linezolid were between 0 and 1.8 h for ATCC 25923, and between 0 and 2.2 h for ATCC 6538. FYL-67 nanoassemblies showed a longer PAE compared with linezolid, which indicated that FYL-67 nanoassemblies may have a higher antimicrobic activity than linezolid.
Table 3 PAE for FYL-67 nanoassemblies and linezolid
Compounds |
PAEa (h) |
ATCC 25923 |
ATCC 6538 |
Strains were exposed to 0.5–4 times the MIC of the test compounds (see text) for 1 h at 37 °C.
|
FYL-67 |
1–3.6 |
0–4 |
Linezolid |
0–1.8 |
0–2.2 |
3.5.
In vitro cytotoxicity of FYL-67 nanoassemblies
The cytotoxicity of FYL-67 nanoassemblies was evaluated by cell viability assay using HEK293 cells and L02 cells, and linezolid was used as a positive control. As shown in Table 4, IC20 of FYL-67 nanoassemblies on HEK293 cells or L02 cells was higher than 25 μM or 50 μM respectively, whereas that of linezolid was 2 μM or 5.9 μM. In comparison with linezolid, FYL-67 nanoassemblies exhibited lower mammalian cell toxicity. Therefore, the FYL-67 nanoassemblies could be regarded as a safe compound.
Table 4 Mean IC20 of FYL-67 nanoassemblies or linezolid in HEK293 cells and L02 cells after incubation for 48 h
Compounds |
IC20 in HEK293 cells |
IC20 in L02 cells |
FYL-67 |
>25 |
>50 |
Linezolid |
2 |
5.9 |
3.6.
In vivo antimicrobial in systemic infection mouse models
In vivo antimicrobial activity of FYL-67 nanoassemblies and linezolid was evaluated in MSSA (ATCC 25923) and MRSA (ATCC 33591) systemic infection mouse models, respectively. As shown in Table 5, in the MSSA systemic infection model, ED50 of FYL-67 nanoassemblies (2 mg kg−1) was significantly lower than that of linezolid (7.5 mg kg−1). Similar results were also found in the MRSA systemic infection model, in which ED50 of FYL-67 nanoassemblies was lower than 4 mg kg−1, versus 9.5 mg kg−1 in the linezolid group. FYL-67 nanoassemblies displayed an efficacy more than 3-fold or 2-fold higher than linezolid against MSSA or MRSA, respectively.
Table 5 Antibacterial efficacies (ED50) of FYL-67 nanoassemblies in mice systemic infection models
Compound |
Strain |
ED50a (mg per kg body weight) |
ED50 is the dose preventing death in 50% of the infected mice after i.v. injection.
|
FYL-67 |
ATCC 25923 |
2 |
ATCC 33591 |
<4 |
Linezolid |
ATCC 25923 |
7.5 |
ATCC 33591 |
9.5 |
3.7.
In vivo toxicity evaluation
We investigated the potential toxicity of the treatment. Body weights of mice were monitored every day. No obvious signs of adverse results were observed in body weight, behavior or feeding. Furthermore, H&E staining sections of heart, liver, kidney, spleen, and lung were observed under a microscope (Fig. 5). No histopathological changes were found in major organs after intravenous administration of FYL-67 nanoassemblies.
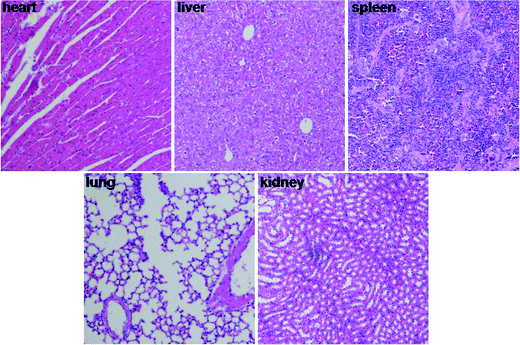 |
| Fig. 5 Photographs of major organs after intravenous administration of FYL-67 nanoassemblies (×200). | |
4. Discussion
Several observations were made in the present study concerning the synthesis, characterization, and antimicrobial activity of FYL-67 nanoassemblies in vitro and in vivo. A novel compound FYL-67 was synthesized, and FYL-67 could form nanoassemblies without using any carrier, organic solvent, or surfactant. The average particle size was 264.6 ± 4.3 nm with a PDI of 0.228 ± 0.016, and the particle size and nanostructure were also confirmed by TEM, which indicates that the prepared FYL-67 nanoassemblies were stable and well-dispersed in aqueous solutions. As no carrier was employed in the FYL-67 nanoassemblies system, DL and EE of the obtained FYL-67 nanoassemblies were both 100%. In in vitro drug release tests, a slower and sustained release behavior of FYL-67 nanoassemblies were observed, which was much different from the rapid release of free FYL-67. In cytotoxicity evaluation, a significant superiority of FYL-67 nanoassemblies was observed compared with linezolid. In addition, FYL-67 nanoassemblies had lower MIC values compared with linezolid in both MSSA and MRSA (except MSSA ATCC 29231). MBC/MIC ratios of FYL-67 nanoassemblies were all within two dilutions of the MIC in both MSSA and MRSA. Besides, PAE of FYL-67 nanoassemblies was also longer than that of linezolid. Furthermore, FYL-67 nanoassemblies showed a much lower ED50 than linezolid in both MSSA and MRSA systemic infection mouse models, and intravenous administration of FYL-67 nanoassemblies did not induce any significant toxic effect.
In the present work, a novel antimicrobial compound FYL-67 was synthesized. It is very interesting that FYL-67 could form nanoassemblies without using any carrier. The balance between hydrophobicity and hydrophilicity of the FYL-67 molecular structure may play a vital role in the formation of FYL-67 nanoassemblies. Besides, as we know, nanoparticles in aqueous solution always tend to aggregate due to their high surface/volume ratio. However, owing to the electrostatic interaction or stereospecific blockade, a stable nanoparticle suspension could be prepared. The zeta potential of FYL-67 nanoassemblies is 17.3 ± 1.8 mV, therefore electrostatic interactions may contribute to the stability of FYL-67 nanoassemblies.
In activity tests in vitro and in vivo, the prepared FYL-67 nanoassemblies were evaluated for their antibacterial activity against a panel of susceptible and resistant Gram-positive organisms. FYL-67 nanoassemblies exhibited potent activities compared with linezolid, with similar MIC values against MSSA ATCC 25923, and lower MIC values (0.5–2 μg mL−1) against the other two MSSA and all three MRSA. In addition, a longer PAE than linezolid was observed in the present study. The PAE refers to the time period after complete removal of an antibiotic, during which there is delayed regrowth of the bacteria. Therefore, this index is recognized as an important pharmacodynamic parameter.26
In the current study, both strains were preexposed to linezolid at 0.5–4 times the MIC, well below the clinical levels achievable in humans.27 After 1 h exposure to FYL-67 nanoassemblies at 0.5–4× MIC, the in vitro PAEs were longer against ATCC 25923 and ATCC 6538 compared with that of linezolid. These may partly explain the reason why a more potent in vivo antibiotic activity of FYL-67 nanoassemblies we observed. Furthermore, results of in vitro antibiotic activity (ratio of MBC to MIC) suggested that FYL-67 nanoassemblies may be considered to be bactericidal, while linezolid displays nonbactericidal activity in vitro against staphylococci. Besides, after FYL-67 was rendered into nanoscale particles, the circulation time of FYL-67 nanoassemblies may be increased, thus improved in vivo antibiotic activity of FYL-67 nanoassemblies. However, this study tested only 2 strains of MSSA and did not test concentrations of FYL-67 nanoassemblies higher than 4× MIC, which we predict would not have detected the maximal effect. Thus, it is very attractive to further test the PAEs of FYL-67 nanoassemblies in vitro and in vivo and analyze the possible mechanism in the future.
5. Conclusions
In this work, we synthesized a novel compound FYL-67 and rendered it into nanoassemblies without using any vehicle, organic solvent, or additive. The formed FYL-67 nanoassemblies were monodisperse with narrow particle size distribution, which could be lyophilized into a powder form, and re-dissolved into a stable and homogeneous solution. We observed a very low cytotoxicity of FYL-67 nanoassemblies on HEK293 cells and L02 cells. The in vitro release profile showed a much slower and sustained release of FYL-67 nanoassemblies compared with free FYL-67. Furthermore, in vitro and in vivo tests indicated that FYL-67 nanoassemblies had significantly higher antimicrobial activities than linezolid in both MSSA and MRSA. The above results suggested that the novel FYL-67 nanoassemblies could serve as a potential candidate for MRSA therapy in clinic.
Declaration of interest statement
The authors report no conflicts of interest. The authors alone are responsible for the content and writing of the paper.
Acknowledgements
This work was supported by the National Major Program of China during the 12th Five-Year Plan Period (2012ZX09103-101-036).
References
- K. K. Kumarasamy, M. A. Toleman, T. R. Walsh, J. Bagaria, F. Butt, R. Balakrishnan, U. Chaudhary, M. Doumith, C. G. Giske, S. Irfan, P. Krishnan, A. V. Kumar, S. Maharjan, S. Mushtaq, T. Noorie, D. L. Paterson, A. Pearson, C. Perry, R. Pike, B. Rao, U. Ray, J. B. Sarma, M. Sharma, E. Sheridan, M. A. Thirunarayan, J. Turton, S. Upadhyay, M. Warner, W. Welfare, D. M. Livermore and N. Woodford, Lancet Infect. Dis., 2010, 10, 597–602 CrossRef CAS.
- E. Rubinstein, R. Isturiz, H. C. Standiford, L. G. Smith, T. H. Oliphant, S. Cammarata, B. Hafkin, V. Le and J. Remington, Antimicrob. Agents Chemother., 2003, 47, 1824–1831 CrossRef CAS.
- R. D. Gonzales, P. C. Schreckenberger, M. B. Graham, S. Kelkar, K. DenBesten and J. P. Quinn, Lancet, 2001, 357, 1179 CrossRef CAS.
- R. E. Mendes, L. Deshpande, E. Rodriguez-Noriega, J. E. Ross, R. N. Jones and R. Morfin-Otero, J. Clin. Microbiol., 2010, 48, 3041–3043 CrossRef.
- S. Tsiodras, H. S. Gold, G. Sakoulas, G. M. Eliopoulos, C. Wennersten, L. Venkataraman, R. C. Moellering and M. J. Ferraro, Lancet, 2001, 358, 207–208 CrossRef CAS.
- D. J. Kuter and G. S. Tillotson, Pharmacotherapy, 2001, 21, 1010–1013 CrossRef CAS.
- B. Y. S. Kim, J. T. Rutka and W. C. W. Chan, N. Engl. J. Med., 2010, 363, 2434–2443 CrossRef CAS.
- O. C. Farokhzad and R. Langer, Adv. Drug Delivery Rev., 2006, 58, 1456–1459 CrossRef CAS.
- T. M. Allen and P. R. Cullis, Science, 2004, 303, 1818–1822 CrossRef CAS.
- W. Charman, H. Chan, B. Finnin and S. Charman, Drug Dev. Res., 1999, 46, 316–327 CrossRef CAS.
- K. L. Hennenfent and R. Govindan, Ann. Oncol., 2006, 17, 735–749 CrossRef CAS.
- V. Wagner, A. Dullaart, A.-K. Bock and A. Zweck, Nat. Biotechnol., 2006, 24, 1211–1217 CrossRef CAS.
- C. Y. Gong, X. W. Wei, X. H. Wang, Y. J. Wang, G. Guo, Y. Q. Mao, F. Luo and Z. Y. Qian, Nanotechnology, 2010, 21 CAS.
- C. Y. Gong, C. Wang, Y. J. Wang, Q. J. Wu, D. D. Zhang, F. Luo and Z. Y. Qian, Nanoscale, 2012, 4, 3095–3104 RSC.
- R. K. Jain and T. Stylianopoulos, Nat. Rev. Clin. Oncol., 2010, 7, 653–664 CrossRef CAS.
- L. Brannon-Peppas and J. O. Blanchette, Adv. Drug Delivery Rev., 2004, 56, 1649–1659 CrossRef CAS.
- B. Ozpolat, A. Sood and G. Lopez-Berestein, J. Intern. Med., 2010, 267, 44–53 CrossRef CAS.
- L. Li, F. Tang, H. Liu, T. Liu, N. Hao, D. Chen, X. Teng and J. He, ACS Nano, 2010 Search PubMed.
- C. Y. Gong, Y. J. Wang, X. H. Wang, X. W. Wei, Q. J. Wu, B. L. Wang, P. W. Dong, L. J. Chen, F. Luo and Z. Y. Qian, J. Nanopart. Res., 2011, 13, 721–731 CrossRef CAS.
- H. Kang, M. B. O'Donoghue, H. Liu and W. Tan, Chem. Commun., 2010, 46, 249–251 RSC.
- H. D. Han, L. S. Mangala, J. W. Lee, M. M. K. Shahzad, H. S. Kim, D. Shen, E. J. Nam, E. M. Mora, R. L. Stone and C. Lu, Clin. Cancer Res., 2010, 16, 3910–3922 CrossRef CAS.
- C. Y. Gong, B. Yang, Z. Y. Qian, X. Zhao, Q. J. Wu, X. R. Qi, Y. J. Wang, G. Guo, B. Kan and F. Luo, Nanomed. Nanotechnol. Biol. Med., 2011 Search PubMed.
- R. D. Pearson, R. T. Steigbigel, H. T. Davis and S. W. Chapman, Antimicrob. Agents Chemother., 1980, 18, 699–708 CrossRef CAS.
-
W. A. Craig and S. Gudmundsson, in Antibiotics in Laboratory Medicine, ed. V. Lorian, Williams and Wilkins, Baltimore, 4th edn, 1996, pp. 296–329 Search PubMed.
- L. J. Reed and H. Muench, Am. J. Epidemiol., 1938, 27, 493–497 Search PubMed.
-
W. A. Craig and S. Gudmundsson, in Antibiotics in Laboratory Medicine, ed. V. Lorian, Williams & Wilkins, Baltimore, 3rd edn, 1999, pp. 403–431 Search PubMed.
- G. W. Kaatz and S. M. Seo, Antimicrob. Agents Chemother., 1996, 40, 799–801 CAS.
Footnote |
† T. Yang and C. Gong contributed equally to this work and are co-first authors for this paper. |
|
This journal is © The Royal Society of Chemistry 2013 |
Click here to see how this site uses Cookies. View our privacy policy here.