DOI:
10.1039/C1JM14232A
(Communication)
J. Mater. Chem., 2012,
22, 60-63
Synthesis and characteristics of a novel, high-nitrogen, heat-resistant, insensitive material (NOG2Tz)†
Received
29th August 2011
, Accepted 25th October 2011
First published on 14th November 2011
Abstract
The novel, high-nitrogen, energetic compound, 3,6-bis(3-nitro-1,2,4-oxadiazole-5-guanyl)-1,2,4,5-tetrazine (NOG2Tz, 8, N 53.08%), was synthesised and fully characterised. Thermal decomposition kinetics and several thermodynamic parameters were obtained under non-isothermal conditions by DSC. NOG2Tz exhibits a high density and was calculated to have favourable detonation properties. It is thermally stable (Tdec. 328 °C) and remarkably insensitive to impact.
High-nitrogen, energetic compounds offer distinct advantages over conventional carbon-based energetic compounds, such as exhibiting a high enthalpy of formation and, in recent years, thermal stability. Molecules with high nitrogen, but low hydrogen and carbon content allow a good oxygen balance to be achieved more easily.1 For example, the static, shock, and friction sensitivities of some high-nitrogen compounds are relatively low.2 The enhanced safety of energetic materials, which is related to transportation, handling, and processing from production to end-use, is more important than better energetic characteristics. The superiority of high-nitrogen compounds may allow them to be used in potential applications such as gas generation, propellants, insensitive-high-pyrotechnics and other new energetic materials.1a,4b
1,2,4,5-Tetrazine rings have been extensively studied as energetic moieties due to their high-energy N–N and C–N bonds, as well as their great amount of ring strain.3 Incorporation of a tetrazine ring into a compound is also a known strategy for increasing thermal stability.4 Several tetrazine derivatives that display particularly interesting properties for explosives or pyrotechnics are the following: diazido-s-tetrazine (DAT, 1),4abis(tetrazolylamino)-s-tetrazine (BTATz, 2),4b,c bis (nitroguanyl)-s-tetrazine (NQ2Tz, 3)4d and its salts, 3,3′-azobis(6-amino-s-tetrazine) (DAAT, 4)4e and its oxide derivatives, 3,6-diamino-s-tetrazine-2,5-dioxide (LAX-112, 5),4b,f and 3-amino-(6-nitroamino)-s-tetrazine (ANAT, 6)4g and its salts. Please see the ESI† for the structures of the compounds.
1,2,4-Oxadiazole is an isomer of furazan, calculated to have a high positive enthalpy of formation.5 We have prepared a series of compounds containing 3-nitro-1,2,4-oxadiazole heterocyclic rings from 3-nitro-5-guanidino-1,2,4-oxadiazole (NOG).5 The results show that the introduction of a 3-nitro-1,2,4-oxadiazole ring to explosives simultaneously increases the thermal stability and decreases the sensitivity. 3,6-Bis(3-nitro-1,2,4-oxadiazole-5-guanyl)-1,2,4,5-tetrazine (NOG2Tz, 8) was prepared by the reaction of NOG and 3,6-bis(3,5-dimethylpyrazol-1-yl)-1,2,4,5-tetrazine (BT).6 The thermostability and impact sensitivity of 8 were exceedingly satisfactory.
In our preliminary experiments the sodium salt of NOG was prepared in situ using sodium methylate in methanol at 0 °C for 30 min.4d BT was added in portions with stirring for 1 h at 0 °C, then 2 h stirring at room temperature (Scheme 1). The reaction mixture was quenched with ice water and an acidic workup resulted in the precipitation of product 7, in which the nitro group was substituted by a methoxy group. BT remained unchanged in the reaction mixture. Comparing the Tdec. of 7 (260 °C) with that of NOG (290 °C)5 provides further evidence that electron-withdrawing groups located at the C(3) position of the 1,2,4-oxadiazole ring enhance the thermal stability of the compound.5,7 The structure of 7 was confirmed by X-ray single diffraction analysis (Fig. 1 and ESI†).
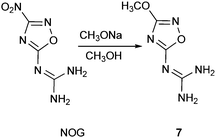 |
| Scheme 1 Synthesis of 7. | |
 |
| Fig. 1 The crystal structure of 7. | |
Another soft nucleophile, strong base, NaH, in DMF was used to obtain 8 (Scheme 2). After three re-crystallizations from DMSO, 8 was obtained as pure, red, needle-shaped crystals. The X-ray analysis shows that 8 has a planar geometry (Fig. 2 and ESI†). Therefore, –NH2protons in 8 have different chemical shifts at 9.27 and 8.74 ppm. The X-ray crystal structure analysis of 8 confirms the structure to be the bis-DMSO solvate of 8 with a density of 1.573 g cm−3. The molecules form planar sheets in the crystal. This graphite-like structure would lead to a high density of the neat material. Indeed, a calculated density of 1.812 g cm−3 was determined. The distance between two adjacent molecules was 5.75 Å in the same layer and the distance between the two layers was 8.875 Å. The DMSO solvate is easily removed by freeze-drying, treating with boiling water for 2 h or repeatedly washing with water. The DSC and impact sensitivity evaluations were conducted on 8 without DMSO.
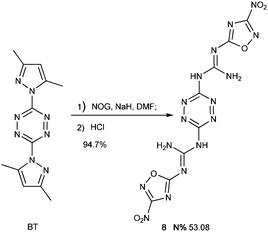 |
| Scheme 2 Synthesis of 8. | |
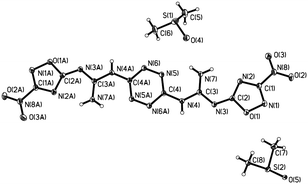 |
| Fig. 2 The crystal structure of 8·2DMSO. | |
The thermal stability of 8 was evaluated with a differential scanning calorimeter (DSC) (Fig. 3 and ESI†). 8 decomposed at 328 °C (peak) indicating that it has higher thermal stability than NQ2Tz (Tdec. 269 °C),4j and is comparable to that of 1,3,5-triamino-2,4,6-trinitrobenzene, TATB (Tdec. 324 °C).8 This shows that the introduction of 3-nitro-1,2,4-oxadiazole to explosive molecules can increase their thermal stability. The TGA analysis of 8 shows the total weight loss is 92.03% over one decomposition phase (Fig. 4).
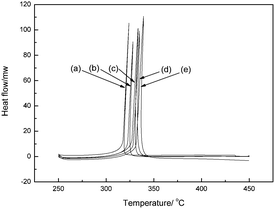 |
| Fig. 3
DSC curves at various heating rates of 8 from left to right, (a), (b), (c), (d), and (e) is 5, 10, 15, 20, and 25 °C min−1, respectively. | |
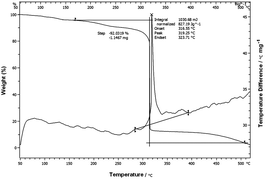 |
| Fig. 4
TGA of 8. | |
Multiple heating methods (Kissinger's method9 and Ozawa's method10) were employed in order to obtain the following thermodynamic parameters for the exothermic decomposition reaction of 8: apparent activation energy (E), pre-exponential factor (A), extrapolated onset temperature (T0), activation entropy (ΔS≠), activation enthalpy (ΔH≠), activation Gibbs free energy (ΔG≠) and critical temperature of thermal explosion (Tb). The Kissinger equation (eqn (1)), Ozawa equation (eqn (2)) and other relevant equations (eqn (3)–(7)) (ref. 11) are as follows:
| 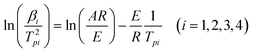 | (1) |
| 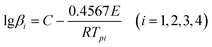 | (2) |
|  | (3) |
| Tpi = Tp0 + b′βi + c′βi2 + d′β3i + e′β4i (i = 1, 2, 3, 4) | (4) |
| 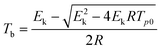 | (5) |
where
β is the linear heating rate,
Tp is the peak temperature,
A is the pre-exponential constant,
R is the gas constant,
kB is the Boltzmann constant,
h is the Planck constant,
E is the apparent activation energy and
C is a constant.
From the results listed in Table 1, we can see that the apparent activation energy obtained by Kissinger's method agrees well with that obtained by Ozawa's method leading to very close linear correlation coefficients (r), which indicates that the results are credible. 8 possesses an excellent heat-resistant ability, with a Tb of 339.9 °C. All the data provide the basis for the evaluation of the thermal stability of the compound.
Entry |
β
a/°C min−1 |
T
p
b/°C |
Kissinger's method |
Ozawa's method |
T
0
h/°C |
T
b
i/°C |
Thermodynamic parameters at T0 |
E
K
c/kJ mol−1 |
lg (A/s−1)d |
r
K
e
|
E
O
f/kJ mol−1 |
r
O
g/ |
ΔS≠j/J K−1 mol−1 |
ΔH≠k/kJ K−1 mol−1 |
ΔG≠l/kJ K−1 mol−1 |
The linear heating rate.
The peak temperature.
The apparent activation energy by Kissinger's method.
The pre-exponential constant.
The linear correlation coefficient by Kissinger's method.
The apparent activation energy by Ozawa's method.
The linear correlation coefficient by Ozawa's method.
The extrapolated onset temperature.
The critical temperature of thermal explosion.
Activation entropy.
Activation enthalpy.
Activation Gibbs free energy.
|
1 |
5 |
323.89 |
91.926 |
14.579 |
0.99 |
92.654 |
0.99 |
329.47 |
339.92 |
33.35 |
89.19 |
78.20 |
2 |
10 |
328.09 |
3 |
15 |
333.48 |
4 |
20 |
335.02 |
5 |
25 |
339.22 |
To investigate the energetic properties of 8, experiments and calculations were performed, the results of which are listed in Table 2. Drop hammer tests were used to determine the impact sensitivity of 8, which was >70 J. This value shows less sensitivity than TATB (IS: 50 J).8 This result shows that 8 is a good candidate for being an insensitive high explosive (IHE). Detonation performance of 8 is calculated based on the single crystal density and the theoretical heat of formation using MOPAC/PM6 (Table 2 and ESI†).
Table 2 Physical properties of 8 and other relevant compounds
Entry |
Compd. |
T
m
a/°C |
T
dec.
b/°C |
Density c/g cm−3 |
ΔHf0d/kJ mol−1 |
IS
e |
P
f/GPa |
D
g/m s−1 |
Melting point.
Thermal degradation (DSC, 10 °C min−1).
Single crystal density.
Heat of formation (MOPAC PW6).
Impact sensitivity (BAM drop hammer).
Detonation pressure.
Detonation velocity.
Calculated (B3LYP/6-31G*).
Ref. 4j; the impact sensitivity is 65 cm.
Ref. 8.
|
1 |
NOG
|
— |
290 (peak) |
1.766 |
235 |
>40 J |
28.16 |
8013 |
2 |
NOG2Tz (8) |
— |
329 (peak) |
1.812h |
957 |
>70 J |
28.52 |
8002 |
3 |
NQ2Tz
i |
— |
269 (peak) |
1.70 |
389 |
32.5 J |
26.0 |
7840 |
4 |
TATB
j |
324 |
324 |
1.93 |
−140 |
50 J |
31.15 |
8114 |
Conclusions
In summary, the new, energetic, high-nitrogen compound, NOG2Tz (8) (N 53.08%), was synthesised by condensation of NOG and BT in high yield (94.7%). It is very stable at high temperatures (Tdec. 328 °C, Tb 339.9 °C) and has excellent impact insensitivity, which should allow it to be used under severe thermal and impact conditions. With its excellent intrinsic properties, NOG2Tz is an attractive, stable, high-nitrogen candidate suitable for practical applications as a heat-resistant energetic material. It was also shown that the introduction of 3-nitro-1,2,4-oxadiazole rings can significantly enhance the thermal stability. We are continuing to expand our research on 3-nitro-1,2,4-oxadiazole and the applications of energetic compounds.
Acknowledgements
This research was financially supported by the China National Defense Fundamental Research Program (973 program, 613830101-3) and the Beijing Institute of Technology, and partly by NSFC (20972016).
Notes and references
-
(a) M. B. Talawar, R. Sivabalan, T. Mukundan, H. Muthurajan, A. K. Sikder, B. R. Gandhe and A. S. Rao, J. Hazard. Mater., 2009, 161, 589–607 CrossRef CAS;
(b) Y. Zhou, X.-P. Long, X. Wang, Y.-J. Shu and A.-M. Tian, Chin. J. Energ. Mater., 2006, 14, 315–320 CAS;
(c) S. Yang, S. Xu, H. Huang, W. Zhang and X. Zhang, Prog. Chem., 2008, 20, 526–536 CAS.
-
(a) T. M. KlapÖke, F. A. Martin and J. Stierstorfer, Angew. Chem., Int. Ed., 2008, 50, 4227–4229 Search PubMed;
(b) M.-H. V. Huynh, M. A. Hiskey, E. L. Hartline, D. P. Montoya and R. Gilardi, Angew. Chem., Int. Ed., 2004, 43, 4924–4928 CrossRef CAS;
(c) C. Ye, H. Gao, J. A. Boatz, G. W. Drake, B. Twamley and J. M. Shreeve, Angew. Chem., Int. Ed., 2006, 45, 7262–7265 CrossRef CAS;
(d) Y.-H. Joo and J. M. Shreeve, Angew. Chem., Int. Ed., 2009, 48, 564–567 CrossRef CAS;
(e) M. H. V. Huynh, M. A. Hiskey, J. G. Archuleta and E. L. Roemer, Angew. Chem., Int. Ed., 2005, 44, 737–739 CrossRef CAS;
(f) G.-H. Tao, B. Twamley and J. M. Shreeve, J. Mater. Chem., 2009, 19, 5850–5854 RSC.
-
(a) G. Clavier and P. Audebert, Chem. Rev., 2008, 80, 3299–3314 Search PubMed;
(b) P. F. Pagoria, G. S. Lee, A. R. Mitchell and R. D. Schmidt, Thermochim. Acta, 2002, 384, 187–204 CrossRef CAS;
(c) A. K. Sikder and N. Sikder, J. Hazard. Mater., 2004, A112, 1–15 CrossRef.
-
(a) M. H. V. Huynh, M. A. Hiskey, J. G. Archuleta, E. L. Roemer and R. Gilardi, Angew. Chem., Int. Ed., 2004, 43, 5658–5661 CrossRef CAS;
(b) D. E. Chavez and M. A. Hiskey, J. Energ. Mater., 1999, 17, 357–377 CrossRef CAS;
(c) A. Saikia, R. Sivabalan, B. G. Polke, G. M. Gore, A. Singh, A. S. Rao and A. K. Sikder, J. Hazard. Mater., 2009, 170, 306–313 CrossRef CAS;
(d) D. E. Chavez, M. A. Hiskey and R. D. Gilardi, Org. Lett., 2004, 6, 2889–2891 CrossRef CAS;
(e) D. E. Chavez, M. A. Hiskey and R. D. Gilardi, Angew. Chem., Int. Ed., 2000, 39, 1791–1793 CrossRef CAS;
(f) M. D. Coburn, M. A. Hiskey, K.-Y. Lee, D. G. Ott and M. M. Stinecipher, J. Heterocycl. Chem., 1993, 30, 1593–1595 CrossRef CAS;
(g) H. Gao, R. Wang, B. Twamley, M. A. Hiskey and J. M. Shreeve, Chem. Commun., 2006, 4007–4009 RSC;
(h) D. E. Chavez and M. A. Hiskey, J. Heterocycl. Chem., 1998, 35, 1329–1332 CrossRef CAS;
(i) M. H. V. Huynh, M. A. Hiskey, D. Chavez, D. L. Naud and R. D. Gilardi, J. Am. Chem. Soc., 2005, 127, 12537–12543 CrossRef CAS;
(j) D. E. Chavez, B. C. Tappan, M. A. Hiskey, S. F. Son, H. Harry, D. Montoya and S. Hagelberg, Propellants, Explos., Pyrotech., 2005, 30, 412–417 CrossRef CAS;
(k) D. E. Chavez and R. D. Gilardi, J. Energ. Mater., 2009, 27, 80–87 CrossRef.
-
Z. Fu, R. Su, Y. Wang, Y.-F. Wang, W. Zeng, N. Xiao, Y. Wu, Z. Zhou, J. Chen and F.-X. Chen, Synthesis and characteristics of insensitive energetic 3-nitro-1,2,4-oxadiazoles, submitted.
- Y. Li, A. Asadi and D. M. Perrin, J. Fluorine Chem., 2009, 130, 377–382 CrossRef CAS.
- S. Buscemi, A. Pace, I. Pibiri and N. Vivona, J. Org. Chem., 2003, 68, 605–608 CrossRef CAS.
- Y. Zhang, Y. Huang, D. A. Parrish and J. M. Shreeve, J. Mater. Chem., 2011, 21, 6891–6897 RSC.
- H. E. Kissinger, Anal. Chem., 1957, 29, 1702–1706 CrossRef CAS.
- T. Ozawa, Bull. Chem. Soc. Jpn., 1965, 38, 1881–1886 CrossRef CAS.
-
(a) L.-Y. Zhang, K.-Z. Xu, J.-R. Song, F.-Q. Zhao, S.-Y. Heng and Y.-Y. Wang, Chin. J. Inorg. Chem., 2010, 26, 899–904 CAS;
(b) F. Zhao, L. Xue, X. Xing, R. Hu, Z. Zhou, H. Gao, J. Yi, S. Xu and Q. Pei, Scientia Sin. Chim., 2010, 40, 1430–1443 Search PubMed.
|
This journal is © The Royal Society of Chemistry 2012 |
Click here to see how this site uses Cookies. View our privacy policy here.