Selective oxidation of benzyl alcohol by two phase electrolysis using nitrate as mediator
Received
14th October 2011
, Accepted 24th December 2011
First published on 5th January 2012
Abstract
A convenient, selective and inexpensive two phase electrolysis method has been developed for preparation of benzaldehyde. Optimization studies have been carried out on an undivided beaker-type cell equipped with carbon anode and stainless steel cathode using a two-phase electrolytic medium made up of an aqueous solution of sodium nitrate with a minimum amount of HCl as supporting electrolyte and chloroform containing benzyl alcohol as organic phase at 30–34 °C. In order to oxidize one mole of benzyl alcohol to benzaldehyde 2 F of electricity was passed. Two-phase electrolysis results in high yield (95%) and high current efficiency (95%).
1. Introduction
Selective oxidation of alcohols is one of the most significant and elementary reactions in laboratory and industrial synthetic chemistry because the corresponding carbonyl compounds can be used as important and versatile intermediates for the synthesis of fine chemicals such as fragrances or food additives.1–4 Usually such selective oxidations involve the use of heavy metal compounds, as manganates, chromates and bromates. However, in a broader outlook such oxidizing reagents are hazardous, toxic and also show low selectivity. Moreover, such reagents are used in stoichiometric amounts, and it generates large quantities of waste whose treatment increases the cost of the overall process.5 Oxidation of alcohols with molecular oxygen using transition metal salts6–13 and polyoxometalates14,15 as catalysts is also reported. Nowadays green innovatory practices have great impact on organic synthesis as they create awareness on environmental issues. Thus, silica-supported reagents16 and carbon-supported platinum catalysts17 are reported in the literature as environmental benign reagents for the synthesis of aldehydes. Moreover techniques like electroorganic synthesis have also developed into an ecofriendly process and are more preferred because of the use of economically cheap reagents compared with the conventional redox reactions.
The present work focuses on the production of benzaldehyde through cheaper ways than the traditional methods as in the case of electrochemical oxidation of alcohols where N-oxyl (nitroxyl radicals) compound-mediated18–20 processes involve expensive reagents as mediators along with the catalyst. However such complexities are overcome by oxidation of alcohols involving nitrate mediated ion like redox catalyst which proceeds through an electrogenerated nitrate radical in two-phase electrolytic medium and being relatively cheap. This mediated system is useful for the oxidation of secondary alcohol to ketone when the electrolysis is carried out in homogeneous phase.21 At present there are very few reports available on the synthesis of fine chemicals by a two-phase electrolysis method22,23 and to our understanding nothing has ever been reported on the two-phase nitrate mediated selective oxidation in the literature.
Herein we report a simple method for the preparation of benzaldehyde from benzyl alcohol by two-phase electrolysis at 30–34 °C as shown in Scheme 1. The oxidation of benzyl alcohol, as a model substrate, was first investigated.
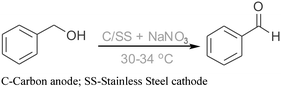 |
| Scheme 1 Selective oxidation of benzyl alcohol to benzaldehyde by two-phase electrolysis. | |
In electrochemical reaction, a nitrate ion undergoes preferably a one-electron oxidation24 and forms a nitrate radical in situ which is known to be an effective hydrogen abstractor.25 Finally, the nitrate radical can be regenerated after reaction with benzyl alcohol. This nitrate radical is responsible for the oxidation of alcohols into ketones/aldehydes. The principle is of increasing importance for the application of redox agents with regard to environmental protection, since large amounts of a product can be generated in a closed electrical circuit using only relatively small amounts of the redox reagent.
2. Experimental section
2.1 Representative electrochemical procedure for nitrate mediated oxidation
An undivided beaker-type glass cell (120 mL capacity) equipped with a magnetic stirrer was used for the electrolysis. Benzyl alcohol (1.045 g, 10 mmol) dissolved in 20 mL chloroform was transferred to an undivided electrolytic cell. Deionised water was used for preparing sodium nitrate solution. An aqueous solution (60 mL) of sodium nitrate (0.5 g, 0.83%) containing 0.37 M HCl was added to it. The aqueous upper phase acted as the supporting electrolyte and nitrate radical source. Carbon anode and stainless steel cathode of 15 cm2 area were placed in the aqueous phase without touching the organic phase but very close to the interphasial region. The organic phase alone was stirred with a magnetic stirrer at a rate of 50 rpm in such a way that the organic layer did not touch the electrodes. Chloroform was periodically added in order to maintain the original volume of the organic phase. The temperature of the electrochemical cell contents was maintained at 30–34 °C throughout the electrolysis. The undivided cell was connected with a dc power supply (Fig. 1).
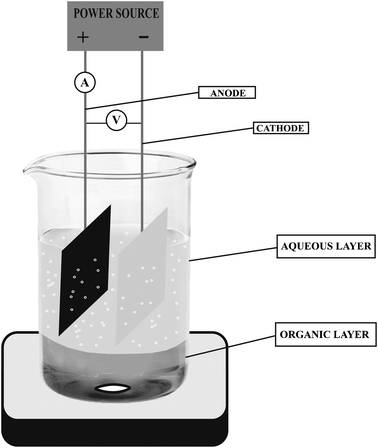 |
| Fig. 1 Electrochemical reaction setup. | |
The electrolysis was conducted galvanostatically at a current density of 50 mA cm−2 until a quantity of 2 F of electricity was passed. An aliquot was drawn periodically from the organic phase and was monitored by HPLC using a Shimadzu LC-8 A column (250 mm × 4.6 mm) as the stationary phase. The eluent consisted of acetonitrile/water (80
:
20) at a flow rate of 1 mL min−1. Samples were analysed at a wavelength of 254 nm with a UV detector. Authentic samples of benzaldehyde and benzoic acid were used to calculate the peak area of the corresponding experimental product for yield calculation.
After completion of the electrolysis, the lower organic phase was separated; washed with water (2 × 25 mL), dried over anhydrous Na2SO4 and the solvent was removed by distillation. HPLC analysis of the residue indicated the presence of 95% benzaldehyde (1.0 g) along with 4% unconverted benzyl alcohol.
3. Results and discussion
3.1 Electrochemical nitrate mediated oxidation of benzyl alcohol
Two-phase electrolysis has a distinct advantage over conventional homogeneous26 and emulsion electrolysis in practical electroorganic synthesis. In homogeneous systems, lower selectivity is observed due to over-oxidation of the substrate on the surface of the electrode leading to a mixture of products. In emulsion electrolysis, electrode passivation leads to high current density and consumption of high energy. In the above two systems, the spent mediator may not be easily separated.
In two-phase electrolysis systems, the reactive species formed by electrolytic oxidation of a mediator in the aqueous phase reacts with the substrate selectively to give the products at the interphasial region of the organic and aqueous phase. After completion of the electrolysis, a usual work up of the organic layer affords the product.
In the selective oxidation of benzyl alcohol by two-phase electrolysis, the following parameters were studied and the results are reported.
3.2 Effect of current density
The reactions were carried out at different current densities from 45 to 75 mA cm−2 by passing 2 F of electricity (Fig. 2). At low current density it takes a long time to carry out oxidation of benzyl alcohol. At higher current densities cell voltage increased at the end of the reaction compared to the initial stage. It reflects that the yield of benzaldehyde decreased along with current efficiency. Hence, the optimum current density for the selective oxidation of benzyl alcohol is 50 mA cm−2 (750 mA).
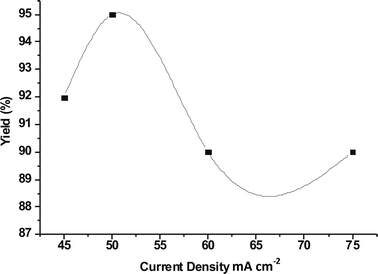 |
| Fig. 2 Effect of current density. | |
3.3 Effect of charge passed on the oxidation of benzyl alcohol
When 2 F of electricity was passed (optimum condition), there was maximum current efficiency (95%) leading to the selective oxidation of 95% of benzyl alcohol to benzaldehyde. Even when the electrolysis was carried out with more than the required amount of charge (F), there was only an increase in the time duration with no significant increase in the yield. This is evident from the drastic decrease of current efficiency (S.No. 3, Table 1) when 3 F of electricity was passed to oxidize benzyl alcohol to benzaldehyde though the product yield was not changed much.
Table 1 Effect of charge passed
S.No |
Charge passed (F) |
Benzaldehyde yield (%) |
Current efficiency (%) |
Two-phase electrolysis conditions: Current density, 50 mA cm−2; electrodes, carbon/carbon; temperature, 30 to 34 °C; stirring rate, 50 rpm; aqueous layer, 3.3% NaNO3 + 0.37 M HCl (60 mL); organic layer, benzyl alcohol (10 mmol) dissolved in chloroform (20 mL). |
1 |
2.0 |
95 |
95 |
2 |
2.5 |
94 |
77 |
3 |
3.0 |
94 |
64 |
3.4 Effect of nitrate mediator concentration
Sodium nitrate was used as mediator for the selective oxidation of benzyl alcohol in an undivided beaker-type cell at a temperature of 30–34 °C. The yield of benzaldehyde did not vary with the increase of nitrate concentration from 0.83 to 3.3%. It shows that 0.83% of mediator concentration is enough for the formation of nitrate radical species. This radical species reacted instantly at interphasial region of organic and aqueous phase for the selective oxidation of benzyl alcohol. Eventually, the mediator did not induce the product (benzaldehyde) for further oxidation.
3.5 Effect of acid
Acid presence is another key parameter in the redox mediated oxidations especially at higher density. Studies were conducted to observe the effect of acids on the selective oxidation of benzyl alcohol. The two-phase electrolysis was attempted with various acid media such as H2SO4, HNO3 and HClO4. It was observed that all other acids performed equally well, but HCl was preferred due to its low cost.
Other studies were conducted to observe the effect of acid concentration on the selective oxidation of benzyl alcohol. There was no increase in the yield of benzaldehyde with increase of concentration of HCl. It was observed that selective oxidation of benzyl alcohol was favored with the use of a lower acid concentration of around 0.37 M HCl. Hence the acid may be used as a supporting electrolyte to improve the conductivity.
3.6 Effect of solvent
Among the solvents used for selective oxidation of benzyl alcohol, carbon tetrachloride yielded benzaldehyde (96%) in a very little higher amount in comparison to other solvents (Table 2). It was sidelined since it is expensive and environmentally not benign. Similarly acetonitrile which is miscible with water forms a homogenous phase with electrolyte leading to higher cell voltage (3.5 V) and eventually higher energy consumption which also poses problems in the separation of spent mediator for recycling purposes. Therefore the ideal choice was chloroform for the two-phase electrolysis.
Table 2 Effect of solvent
S.No. |
Solvent |
Benzaldehyde yield (%) |
Current efficiency (%) |
Homogeneous phase (67% water–33% acetonitrile). Two-phase electrolysis conditions: current density, 50 mA cm−2; amount of electricity, 2 F; electrodes, carbon/carbon; temperature, 30 to 34 °C; stirring rate, 50 rpm; aqueous layer, 0.83% NaNO3 + 0.37 M HCl (60 mL); organic layer, benzyl alcohol (10 mmol) dissolved in various solvents mentioned in Table 2 (20 mL). |
1 |
CH2Cl2 |
90 |
90 |
2 |
CHCl3 |
95 |
95 |
3 |
CCl4 |
96 |
96 |
4 |
CH3CNa |
95 |
95 |
3.7 Effect of various electrode materials
Table 3 lists various types of electrode pairs employed in selective oxidation of benzyl alcohol by the two-phase electrolysis method. Platinum/platinum, platinum/stainless steel, carbon/carbon and carbon/stainless steel electrode materials were used in electrolysis and it was found that all four electrode pairs have more or less the same efficiency. From the practical point of view, carbon/stainless steel electrodes were preferred because of their easy availability and low cost.
Table 3 Effect of various electrode materials
S.No. |
Electrode materials (anode/cathode) |
Benzaldehyde yield (%) |
Current efficiency (%) |
Pt Platinum; C Carbon; SS Stainless Steel. Two-phase electrolysis conditions: current density, 50 mA cm−2; amount of electricity, 2 F; electrodes, electrode pairs mentioned in Table 3; Temperature, 30 to 34 °C; stirring rate, 50 rpm; aqueous layer, 0.83% NaNO3 + 0.37 M HCl (60 mL); organic layer, benzyl alcohol (10 mmol) dissolved in chloroform (20 mL). |
1 |
C/C |
95 |
95 |
2 |
C/SS |
95 |
95 |
3 |
Pt/Pt |
92 |
92 |
4 |
Pt/SS |
91 |
91 |
3.8 Reusability of spent mediator
The most attractive features of mediated electrosynthesis are the use of nonstoichiometric quantities of redox mediator and its recycling. This method is economically attractive and environmental friendly since recycling of electrolyte results in a ‘zero effluent’ process. A few reactions were conducted for the selective oxidation of benzyl alcohol under optimized conditions with continuous recycling of the regenerated nitrate mediator. Almost identical yields (95–92%) were obtained through recycling the oxidant.
The two-phase electrolysis was also attempted with various nitrate mediators such as ammonium nitrate and potassium nitrate. It was observed that all other nitrates performed equally well, but sodium nitrate was preferred due to its low cost.
As a result, the plausible mechanism for the nitrate mediated selective oxidation of benzyl alcohol to benzaldehyde by the two-phase electrolysis method is given in Scheme 2.21
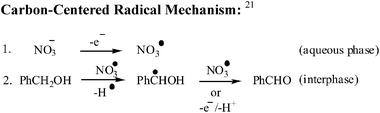 |
| Scheme 2 Catalytic cycle. | |
Conclusions
In conclusion, the two-phase electrolysis method involving the nitrate mediated selective oxidation of benzyl alcohol comprises a novel, efficient and alternative method over the conventional chemical method. The reactions were carried out under mild conditions with a very simple electrochemical setup of carbon anode and stainless steel cathode. Besides, it had several advantages such as the absence of secondary products, low cost of production, selective oxidation, high current efficiency, high yield and recycling of spent mediator. Purity of the product was also consistent during recycling. Thus it seems that this scheme is amenable for scale up as a pollution free process.
Acknowledgements
The authors thank Dr M. Anbu Kulandainathan, Dr K. Kulangiappar, Electro Organic Division, Central Electrochemical Research Institute, Karaikudi-630006, India, and Mr J. Sebastian Raja, Institute of Physical Chemistry ‘Rocasolano’, Department of Biophysical Chemistry, Madrid, Spain-28006, for their generous support and encouragement to carry out this work. One of the authors (C. Christopher) thanks Dr P. S. Joseph, Principal, Thanthai Hans Roever College, Perambalur-621212, India, for being generous and understanding in granting research leave.
Notes and references
-
A. Sheldon and J. K. Kochi, Metal Catalysed Oxidations of Organic Compounds, Academic Press, London, 1981 Search PubMed.
-
M. Hudlicky, Oxidations in Organic Chemistry, ACS Monograph Series, ACS, Washington, DC, 1990 Search PubMed.
- S. S. Stahl, Angew. Chem., Int. Ed., 2004, 43, 3400 CrossRef CAS.
- M. Musawir, P. N. Davey, G. Kelly and I. V. Kozhevnikov, Chem. Commun., 2003, 1414 RSC.
- A. Jia, L. Lou, C. Zhang, Y. Zhang and S. Liu, J. Mol. Catal. A: Chem., 2009, 306, 123 CrossRef CAS.
- S. R. Reddy, S. Das and T. Punniyamurthy, Tetrahedron Lett., 2004, 45, 3561 CrossRef CAS.
- T. Iwahama, Y. Yoshino, T. Keitoku, S. Sakaguchi and Y. Ishii, J. Org. Chem., 2000, 65, 6502 CrossRef CAS.
- I. E. Marko, A. Guaiter, R. Dumeunier, K. Doda, F. Philippart, S. M. Brown and J. Ureh, Angew. Chem., Int. Ed., 2004, 43, 1588 CrossRef CAS.
- S. Velusamy, M. Ahamed and T. Punniyamurthy, Org. Lett., 2004, 6, 4821 CrossRef CAS.
- H. Egami, S. Onitsuka and T. Katsuki, Tetrahedron Lett., 2005, 46, 6049 CrossRef CAS.
- J. Martin, C. Martin, M. Faraj and J.-M. Bregeault, Nouv. J. Chim., 1984, 8, 141 CAS.
- M. J. Schultz, S. S. Hamilton, D. R. Jensen and M. S. Sigman, J. Org. Chem., 2005, 70, 3343 CrossRef CAS.
- A. Yoshida, Y. Takahashi, T. Ikeda, K. Azemoto and S. Naito, Catal. Today, 2011, 164, 332 CrossRef CAS.
- A. V. Biradar, M. K. Dongare and S. B. Umbarkar, Tetrahedron Lett., 2009, 50, 2885 CrossRef CAS.
- A. Bordoloi, S. Sahoo, F. Lefebvre and S. B. Halligudi, J. Catal., 2008, 259, 232 CrossRef CAS.
- C. Wiles, P. Watts and S. J. Haswell, Tetrahedron Lett., 2006, 47, 5261 CrossRef CAS.
- P. Korovchenko, C. Donze, P. Gallezot and M. Besson, Catal. Today, 2007, 121, 13 CrossRef CAS.
- D. Liaigre, T. Breton and E. I. Mustapha Belgsir, Electrochem. Commun., 2005, 7, 312 CrossRef CAS.
- J. Yusuke, M. S. Koichi and H. Tanaka, Tetrahedron Lett., 2005, 46, 8975 CrossRef.
- H. Tanaka, V. Kawakami, K. Goto and M. Kuroboshi, Tetrahedron Lett., 2001, 42, 445 CrossRef CAS.
- J. E. Leonard, P. C. Scholl and T. P. Steckel, Tetrahedron Lett., 1980, 21, 4695 CrossRef CAS.
- R. Sripriya, M. Chandrasekaran and M. Noel, J. Appl. Electrochem., 2008, 38, 597 CrossRef CAS.
- F. Raynal, R. Barhdadi, J. Périchon, A. Savall and M. Troupel, Adv. Synth. Catal., 2002, 344, 45 CrossRef CAS.
-
C. K. Mann and K. K. Barnes, Electrochemical Reactions in Nonaqueous Systems, Marcel Dekker, New York, NY, 1970, p. 511 Search PubMed.
- P. C. Scholl, S. E. Lentsch and M. R. Van De Mark, Tetrahedron, 1976, 32, 303 CrossRef CAS.
-
S. R. Forsyth and D. Pletcher, Extended Abstracts of the Ist International Symposium on Electroorganic Synthesis, Kurashiki, 1986, p. 35 Search PubMed.
|
This journal is © The Royal Society of Chemistry 2012 |